- 1Institute of Human Movement Sciences and Sport, Department of Health Sciences and Technology, ETH Zurich, Zurich, Switzerland
- 2Cereneo, Center for Neurology and Rehabilitation, Vitznau, Switzerland
- 3Department of Neurology, University Hospital of Zurich, Zurich, Switzerland
- 4Fraunhofer Portugal AICOS, Porto, Portugal
- 5Division of Physiotherapy, Department of Neurobiology, Care Sciences and Society, Karolinska Institutet, Stockholm, Sweden
Aging is associated with a decline in physical functions, cognition and brain structure. Considering that human life is based on an inseparable physical-cognitive interplay, combined physical-cognitive training through exergames is a promising approach to counteract age-related impairments. The aim of this study was to assess the effects of an in-home multicomponent exergame training on [i] physical and cognitive functions and [ii] brain volume of older adults compared to a usual care control group. Thirty-seven healthy and independently living older adults aged 65 years and older were randomly assigned to an intervention (exergame training) or a control (usual care) group. Over 16 weeks, the participants of the intervention group absolved three home-based exergame sessions per week (à 30–40 min) including Tai Chi-inspired exercises, dancing and step-based cognitive games. The control participants continued with their normal daily living. Pre- and post-measurements included assessments of physical (gait parameters, functional muscle strength, balance, aerobic endurance) and cognitive (processing speed, short-term attention span, working memory, inhibition, mental flexibility) functions. T1-weighted magnetic resonance imaging was conducted to assess brain volume. Thirty-one participants (mean age = 73.9 ± 6.4 years, range = 65–90 years, 16 female) completed the study. Inhibition and working memory significantly improved post-intervention in favor of the intervention group [inhibition: F(1) = 2.537, p = 0.046, = 0.11, working memory: F(1) = 5.872, p = 0.015, = 0.02]. Two measures of short-term attentional span showed improvements after training in favor of the control group [F(1) = 4.309, p = 0.038, = 0.03, F(1) = 8.504, p = 0.004, = 0.04]. No significant training effects were evident for physical functions or brain volume. Both groups exhibited a significant decrease in gray matter volume of frontal areas and the hippocampus over time. The findings indicate a positive influence of exergame training on executive functioning. No improvements in physical functions or brain volume were evident in this study. Better adapted individualized training challenge and a longer training period are suggested. Further studies are needed that assess training-related structural brain plasticity and its effect on performance, daily life functioning and healthy aging.
Introduction
The worldwide population of older adults is growing fast and concurrently, life expectancy is prolonged leading to an increase of age-related impairments and diseases (1). These degenerative changes affect physical and cognitive functions resulting in impaired daily life functioning including mobility impairments which, in turn, reduce independence and psychological well-being as well as increase health care costs (2). Moreover, aging is accompanied by alterations in brain structure. Significant gray matter (GM) volume reduction in frontal, temporal, and subcortical areas are described in elderly with mild cognitive impairment or dementia as well as in healthy older adults (3). The diminished brain structures seem to be related to behavioral impairments and might translate to cognitive and/or physical dysfunctioning (4). However, a considerable inter-individual variation exists in age-related declines (5). Thus, other factors than age itself seem to influence functionality in older age, for example lifestyle and behavior (6). Human brain and body are plastic and adapt in response to experiences and stimulation (7). Also in higher age, experience-based plasticity can be observed on behavioral and neuronal level (8). Nevertheless, a knowledge gap exists regarding the optimal ways for influencing neuronal plasticity. To conclude, a strong need exists to support healthy aging by identifying effective strategies, which trigger experience-based (neuro)plasticity.
One behavioral strategy to promote healthy aging is applying physical exercise. Many studies have shown that physical activity has various positive effects on the human body (9). Physical exercise improves the cardiovascular and respiratory system, the metabolism, the immune system and body composition (10). Moreover, cross-sectional as well as longitudinal and intervention studies revealed positive effects of physical activity and exercising on psychological well-being and cognition of older adults (11, 12). On brain level, animal and human studies demonstrated positive effects on various brain structures, particularly a brain volume increase in the frontal cortex and the hippocampus, areas that are most affected during the aging process (13–15). Although the underlying mechanisms of these brain alterations are not yet fully understood and difficult to explore in vivo in humans, physical exercise is known to induce a cascade of cellular and molecular processes that support brain plasticity (16, 17). Changes in neurotransmitters and/or neurotrophic factors are supposed to play a critical role in training-related neuroplasticity (18, 19). For example, exercising increases the expression of neurotrophins such as vascular endothelial growth factor (VEGF), brain-derived neurotrophic factor (BDNF) or insulin-like growth factor 1 (IGF-1), which might trigger neuroplasticity (20). Synaptic changes e.g., strengthening of existing connections or building new synapses (synaptogenesis), the creation of new blood vessels (angiogenesis) and the development of new neurons (neurogenesis) and glia cells (gliagenesis) are discussed as underlying mechanisms for neuroplasticity (17, 21–25).
Besides physical exercise, challenging environments and cognitive training are also discussed to be able to enhance cognitive performance and positively change certain brain structures. The results of reviews and meta-analyses show that older adults can benefit from cognitive training (26, 27). Cognitive training seems to be effective in improving various aspects of cognitive functioning including memory, executive functions, attention, visuo-spatial skills and processing speed (28). Regarding neuronal measures, a positive influence on gray and white brain matter has been reported after cognitive training in older adults, mainly in frontal, temporal (including hippocampus), and parietal areas (29–33). Animal studies, with mice or rats raised in enriched environments (environments with various objects as toys, tunnels, and mirrors providing cognitive stimulation), showed altered brain structure and better cognitive performance (34–36). As underlying mechanism, neurogenesis, synaptogenesis and adaptations in astrocytes are discussed (34–37). Moreover, BDNF production has been shown to be increased by cognitive stimulation (37, 38).
Considering the significant impact of physical activity and cognitive training on several health aspects, it has been assumed that a combination of physical exercise with cognitive stimulation poses a promising training approach with superior benefits due to several reasons (39–44). First, most daily life activities require the simultaneous performance of cognitive and physical functions (45). Thus, combined physical-cognitive training has ecological validity and is close to daily life requirements. This might enhance transfer effects and perceived meaningfulness of training (43). Second, the inseparable physical-cognitive interplay of human life can be described also from a more biological perspective: Muscles and movements are controlled by the central nervous system, whereas feedback from peripheral structures as muscles and sensory organs influence brain activity (19). Combined training targets this interplay. Third, synergistic effects of combined training are assumed: Physical activity seems to trigger neuroplastic effects, but cognitive stimulation might be crucial to guide and consolidate these effects (46, 47).
A promising approach for interactive physical-cognitive training are so-called exergames. Exergames are digital games, which require bodily movements to play the game (48). In the virtual reality/game scenario, cognitive stimulation may be, more or less explicitly and specifically, embedded. Exergames are known to increase training motivation due to “gamification” of training leading to engaging and fun gameplay (49, 50). Depending on the applied technology, exergame training might be conducted in an in-home setting that overcomes accessibility barriers (51). In general, exergame training has been shown to improve both, cognitive functions (mainly executive functions) (52, 53) and physical functions (cardiovascular or musculoskeletal system) depending on the physical components and exercises that are integrated in the training (e.g., stepping or cycling) (54–56). Compared to traditional cognitive or physical training (e.g., aerobic training), exergaming seems to be equally or slightly more effective for cognitive enhancement in older adults (52, 53). On brain level, two studies assessed changes in brain structure after exergame training in older adults (57, 58). To our knowledge, however, no past research has investigated the effect of home-based exergame training, tailored to older adults' needs, on physical and cognitive functions and on brain structure of the elderly.
Considering the theoretical background from human movement science and neuropsychology together with the art of game design and the needs of older adults, the Active@Home exergame was developed. This exergame enables multicomponent training including strength, balance and cognitive training. Usability and feasibility of the newly developed exergame was tested previously (59). Results showed that the Active@Home exergame is a usable and feasible home-based training for older adults. Consequently, the next step in the development process is to conduct a study assessing the effectiveness of the exergame training.
The primary objective of this study was to assess the effects of this in-home multicomponent exergame training on physical and cognitive functions in healthy older adults. The secondary objective included the application of magnetic resonance imaging (MRI) and voxel-based morphometry (VBM) to evaluate the impact of exergame training on brain volume in healthy older adults. We hypothesized that the Active@Home exergame training would enhance physical and cognitive functions as well as positively influence brain volume compared to a passive control group in older adults.
Materials and Methods
Participants
Participants were recruited from June to August 2018 through public advertisements in local newspapers, senior organizations, physiotherapy and medical centers as well as supermarkets. All potential participants were screened for eligibility using the Mini Mental State Examination (MMSE) to assess cognitive status and a health questionnaire to assess anthropometric and health data and medical history. Participants fulfilling all of the following inclusion criteria were eligible for the study: (1) age ≥ 65 years, (2) living independently, (3) healthy by self-report, (4) able to stand at least for 10 minutes without assistance, (5) access to a TV with HDMI connection. Participants exhibiting at least one of the following criteria were excluded from the study: (1) cognitive impairments (MMSE ≤ 23 points), (2) mobility impairments that prevent training participation, (3) severe and uncontrolled health problems (e.g., recent cardiac infarction, uncontrolled diabetes or hypertension), (4) orthopedic disease that prevents training participation, (5) neurological disease (e.g., history of stroke or epilepsy, Parkinson's disease, Alzheimer disease or other forms of dementia), (6) acute severe, rapidly progressive or terminal illness, (7) intake of any psychoactive substances (e.g., neuroleptics, antidepressants), (8) active participation in a supervised physical or cognitive training. Consumption of nicotine or caffeine was not defined as an exclusion criterion, but participants were asked to consume the usual amount before measurements to avoid deprivations states. Regarding MRI measurements, participants with claustrophobia or magnetic metal pieces in the body were excluded.
Written informed consent was obtained from all participants before any measurements. The intended study sample size of 40 participants was determined by the number of available training/exergame systems. Participants received no payment or refund for traveling expenses but a detailed feedback on personal performance and general study outcomes at the end of the trial.
Study Design and Procedure
This randomized controlled trial (RCT) was a clinical phase III study (60) and used an experimental mixed design with time (pre, post) as within-subject factor and group (training, control) as between-subject factor.
The eligible participants were randomly allocated to either the intervention or the control group using a computer-based randomization tool (www.randomization.com). To counteract size imbalances of the groups, permutated block randomization method with block size of four was used (61). The allocation sequence was kept by an independent study investigator whereas participant enrollment and assignment were done by other study investigators. Blinding of investigators was not possible since they were responsible for both, conduction of measurements and supervision during the intervention period. Blinding of participants was not possible since a passive control group was used.
Assessments were performed at baseline (pre) and at the end (post) of the intervention phase within a timeframe of 2 weeks and were conducted at the cereneo (Swiss clinic and research institute at Vitznau, Lucerne, Switzerland). Pre- and post-measurements were conducted at approximately the same daytime for each participant. The intervention period lasted 16–18 weeks (a maximum of 2 weeks holiday interruption was allowed) and took place from July 2018 until December 2018.
The participants of the training group trained three times per week for 30–40 min during 4 months resulting in a total of 48 training sessions. Training frequency and duration followed previous exergame studies illustrating positive effects in older adults (40) and current recommendations for physical activity and fall prevention in elderly (62, 63). The training was conducted at participants' home. For installation and instruction, the study investigators visited participants at home. The training sessions were scheduled individually by participants considering a guideline of three training sessions per week and no more than one training session per day. Furthermore, participants received written recommendations how to progressively adapt the training challenge whereas a moderate training intensity was targeted (64). All training sessions were recorded by participants on a training diary. The participants of the control group were instructed to continue with their normal daily living. At study end, Active@Home training systems were provided to the control group members to enable a voluntary 4-month training period.
All participants (training and control group) were called biweekly to monitor for deviations in their usual patterns of physical and cognitive activities (on top of the study-related activity). Intense physical activity (e.g., running or playing tennis) was separated from moderate physical activity (e.g., walking or gardening). Examples for cognitive activities were playing chess, learning a language or using an app for playing games. Furthermore, participants in the training group were asked about potential safety issues during training and were supported in case technical or content related issues arose. A total of eight phone calls per participant were absolved.
All procedures were carried out in accordance with the Declaration of Helsinki and were approved by the ethics committee of Nordwest- und Zentralschweiz (EKNZ, 2018-00510). The study was registered at the Clinical Trial Register (www.clinicaltrials.gov, NCT03676452).
Training Intervention - Active@Home Exergame
The Active@Home exergame is a technology-based multicomponent in-home training for older adults to train strength, balance and cognition. It consists of the following three components: (1) Tai Chi-inspired exercises, (2) dancing, and (3) step-based cognitive games. Tai Chi-inspired exercises are a combination of lower-limb and core strength exercises with Tai Chi movement elements performed in three different stance positions (squat, plié, and lunge). Dancing exercises are based on dances such as Bachata, Disco Fox, Salsa, Waltz, Chachacha, and Jive and require motor components of balance, coordination, and agility (65). A total of 18 different step-based cognitive games target specific attentional and executive functions; e.g., selective and divided attention, interference control, cognitive flexibility, and working memory. By stepping forward, backward, and to the right or left side, the cognitive games are played and controlled. In the Active@Home exergame, physical and cognitive functions are trained in a combined approach where physical-cognitive interplay is required in all training components. For Tai Chi-inspired and dance exercises, the cognitive demands might be lower than for the step-based specific cognitive games but nevertheless, cognitive functions are required for movement imitation, control and coordination (66, 67). In contrast, the step-based games might be less physically demanding than Tai Chi-inspired and dance exercises, however, movements (steps) are required to play the games. The training content of the Active@Home exergame is based on current recommendations for exercising in older age (63, 68–70).
The hardware of the Active@Home exergame consists of four inertial measurement units (IMUs) providing both accelerometer and gyroscope assessments. For movement evaluation, participants wore the IMUs at wrists and ankles attached with a silicone slap band. Color and size of the silicon bracelet helped to distinguish the position/placement of each IMU. The IMUs were connected via Bluetooth to a HDMI dongle. This dongle was inserted into a television (TV) and provided the exergame software. The game interface was presented on the TV screen. By pointing the IMU on the right wrist horizontally to the TV screen, a “hand mouse” got activated for navigation through the game (see Figure 1).
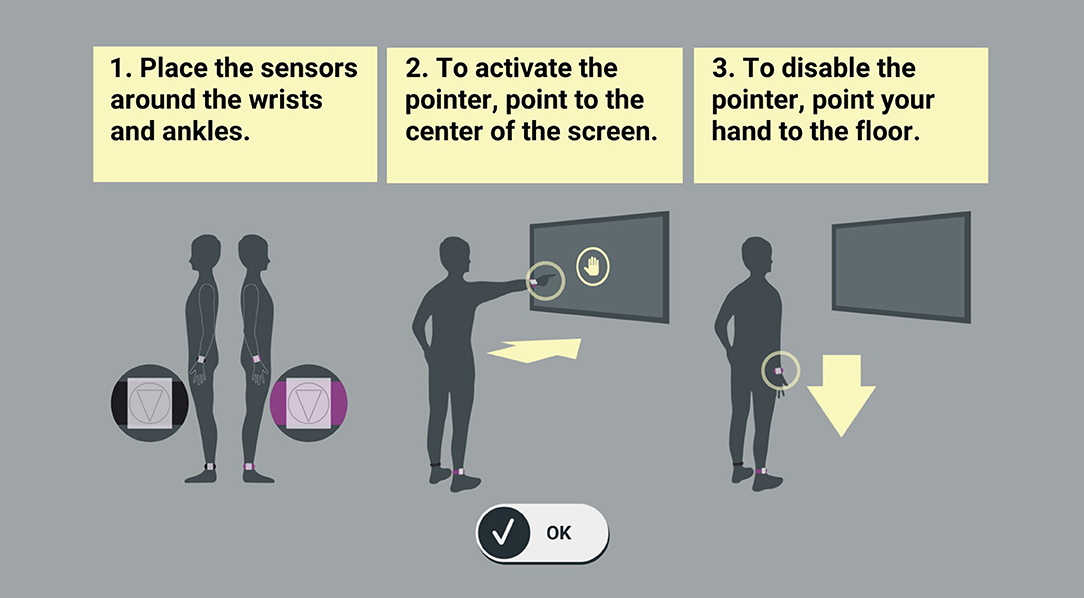
Figure 1. Set up and navigation in the Active@Home exergame. The hardware of the Active@Home exergame consists of four inertial measurement units (IMUs). For movement evaluation, participants wore the sensors at wrists and ankles. Color and size of the bracelet helped to distinguish the position of each IMU. The IMUs were connected via Bluetooth to a HDMI dongle. This dongle was inserted into a television and provided the exergame software. The game interface was presented on the TV screen. By pointing the IMU on the right wrist horizontally to the TV screen, a “hand mouse” got activated for navigation through the game.
The story of the exergame was about traveling in Europe and to train in several different cities (London, Paris, Amsterdam, Rome, Porto, and Zurich). Exercises were instructed by a cartoon-based instructor and were accentuated with background music (71). Moreover, the exergame implemented some basic training principles which are important for effective training such as feedback, optimal challenge and progression, and variety (72). A multimodal feedback system based on symbols, sounds, performances scores and a color code was included. To ensure optimal challenge and progression, several difficulty levels for the exercises were developed. Progression was reached through more complex or faster movements. Each training session included Tai Chi-inspired exercises, dancing and step-based cognitive games to an equal amount. It was recommended to play through all levels of one city before switching to the next city whereas the order of the cities was predefined based on progressive difficulty. Additionally, before changing the level, one level should have been trained at least in two separated sessions. The current difficulty level should always provide an optimal challenge avoiding under- or overload.
Outcome Measures
Assessments of Physical Functions
Gait analysis
Parameters of gait kinematics were measured using the wearable Physilog®5 (Gait Up Sàrl, Lausanne, Switzerland), which has been shown to reliably and validly measure gait performance (73). The Physilog®5s were clipped to the top of the right and left shoe (forefoot). Participants were required to walk a 10 m straight and flat distance six times consecutively to achieve a walkway of 60 m resulting in more than 20 gait cycles, ensuring reliable assessment of gait variability (74). Two walking conditions were used: (1) single-task walking (ST): participants were instructed to walk at preferred speed without talking; (2) dual-task walking (DT): participants were instructed to walk at preferred speed and simultaneously count backwards (cognitive task) in steps of seven from a randomly given number between 200 and 250. In this condition, participants were asked to count aloud and perform both tasks concurrently and not to prioritize one task above the other. This is a common method to measure multitasking capabilities.
For further analysis, data were transferred to the computer via USB port. Two walking cycles for initiation and termination and turns were discarded in order to analyse steady state walking. For this study, the focus was on the following parameters: speed [m/s], stride length [m], stride time [s], and minimal toe clearance [cm]. For each gait parameter, mean values of the left and right foot were determined for both walking conditions and coefficients of variation (CV) were calculated for all parameters: CV [%] = (standard deviation)/mean × 100. Dual-task costs (DTC) were calculated as a percentage of loss of the DT relative to the ST performance according to the formula: DTC [%] = (ST—DT)/ST × 100.
Short Physical Performance Battery (only balance test)
To assess balance, a subtest of the Short Physical Performance Battery (SPPB) was applied (Interclass Correlation Coefficient of 0.88–0.92) (75, 76). The balance test of the SPPB includes: standing in (1) feet side-by-side position, (2) semi-tandem stance, and (3) full-tandem stance. Each position should be held unsupported for 10 s. The performance was rated with a score ranging from zero (“not able to complete the tasks”) to four points (“good balance function”). In line with previous studies (77), we extended the balance test with two additional tasks to avoid ceiling effects. The first additional task was a 20 s single-leg stance (with preferred leg) where two points were achieved for reaching 20 s, one point for 10–20 s and zero points for <10 s. The second additional task was a single-leg stance (with preferred leg) with eyes closed where one point was assigned for every 5 s of successful task achievement. Three trials were conducted for each additional task whereas the best trial counted. For the extended version of the subtest, the maximum point score is unlimited with higher scores meaning better balance functioning. The total score of the extended subtest was calculated for the analysis.
Senior Fitness Test (two subtests)
Two subtests of the Senior Fitness Test (SFT) were used to assess functional lower body strength and aerobic endurance (Interclass Correlation Coefficient of 0.93–0.98) (78, 79). In the 30 s chair rises test, the participants started in a standing position in front of a chair with arms crossed in front of the chest and performed as many full “chair rises” as possible in 30 s. A full chair rise was defined as sitting down on the chair and standing up, ending in an upright position again. The number of completed full chair rises in 30 s was counted.
In the 2 min stepping test, the participants were asked to alternatingly step with both legs in place as many times as possible in 2 min while reaching a predefined individual height with the knees. This threshold height was calculated by means of the height from the floor to the middle of the thigh (midway between the iliac crest and the upper patella). For the analysis, the number of steps with the starting leg was counted during the 2 min whereas a step was valid when the knee was reaching the required height (marked with a tape on the wall).
Assessments of Cognitive Functions
Victoria Stroop Test
To assess response inhibition and interference control, the Victoria Stroop Test (VST) was used, a tool that validly and reliably measures executive functions (80). The VST compromises three parts: (1) VST 1: naming the color of dots (red, blue, green, or yellow), (2) VST 2: naming the color of neutral words (e.g., words like “when” or “hardly” colored in red, blue, green, or yellow), and (3) VST 3: naming the color of “color words” printed in incongruent colors (e.g., word = red while word color = blue, etc.). In VST 3, interfering information is provided which requires interference control and response inhibition; the fast and automatic response of reading the words has to be inhibited and a more effortful color-naming response has to be produced. Each part contains 24 stimuli. Performance time [s] was recorded for each task and errors were counted.
Trail Making Test
The valid and reliable Trail Making Test (TMT) was used to assess psychomotor speed and executive functioning (81). In the first part of the test (TMT A), participants had to connect randomly allocated, encircled numbers from 1 to 25 in ascending order as fast as possible. In the second part of the test (TMT B), the stimuli comprise encircled letters and numbers. The randomly allocated numbers and letters have to be connected in ascending numerical and alphabetical order alternatingly as fast as possible (e.g., 1 – A – 2 – B – 3 – C – …). TMT A measures visual exploration skills and psychomotor processing speed whereas TMT B additionally assesses cognitive flexibility. In both parts, a short practice session was conducted. Time [s] for completing the tasks was recorded and errors were counted.
Wechsler Memory Scale-Revised (two subtests)
Further cognitive performance was evaluated with two subtests of the Wechsler Memory Scale-Revised (WMS-R) (82). The first subtest of the WMS-R, the digit span forward task (later on called WMS-R forward), assessed the short-term attention span and information processing speed. Participants had to remember and repeat digit sequences, which were read aloud and monotonously by the tester, in the correct order. The second subtest of the WMS-R, the digit span backward task (later on called WMS-R backward), was used to evaluate working memory capacity. Participants had to repeat the digit sequences in reversed order. Span length covered two to eight digits whereas for each length, two trials were presented to the participant prior to increasing sequence length. For every correct replication of a digit sequence, one point was scored, summing up to a total point score for each subtest. Moreover, the length of the longest correctly repeated digit sequence was recorded as the maximum span.
MRI (Image) Acquisition and Preprocessing
MRI measurements were conducted at cereneo (Neurorehabilitation Clinic cereneo AG, Vitznau, Switzerland) with a 3T Philips Ingenia MRI scanner (Philips Healthcare, Best, The Netherlands) equipped with a 32-channel head coil. The whole-brain structural images were obtained using a three-dimensional T1-weighted spoiled gradient echo pulse sequence (180 slices, TR: 20 ms, TE: 2.3 ms, flip angle: 20°, FOV: 220 mm × 220 mm × 135 mm, matrix size: 224 × 192, resulting in a voxel size of 0.98 mm × 1.15 mm × 1.5 mm).
For pre-processing, we used the VBM12 toolbox (Voxel-Based Morphometry; Structural Brain Mapping Group, Department of Psychiatry, University of Jean, Germany) of SPM12 (Statistical Parametric Mapping; The Wellcome Trust Centre for Neuroimaging, Institute of Neurology, University College London, London, United Kingdom) running under MATLAB R2017b (The Mathworks, Natick, Massachusetts, USA). First, all images were visually checked for artifacts, structural abnormalities, and pathologies by a physician. In case of brain abnormalities, participants were excluded. Then, the standard VBM routines and default parameters were applied (83). The pre-processing procedure implemented in VBM consisted of a tissue classification and segmentation into gray matter (GM), white matter (WM) and cerebrospinal fluid (CSF), followed by high-dimensional spatial DARTEL (Diffeomorphic Anatomical Registration Through Exponentiated Lie Algebra) normalization into MNI (Montreal Neurological Institute) space (84). The spatially normalized GM volumes were then smoothed with an isotropic Gaussian kernel of 6 mm (for further analysis of the cerebellum) respective 10 mm (for further analysis of the frontal cortex and the hippocampus) full width at half maximum (FWHM). The normalized and smoothed GM images were used for statistical analysis. Additionally, whole-brain GM, WM and CSF volumes in native space were calculated and summed to yield the total intra-cranial volume. Moreover, bilateral hippocampal volume (sum of right and left hippocampus volume) was calculated based on HV toolbox (Hippocampal Volumetry; jung diagnostics GmbH, Germany) (85) in SPM12.
Statistical Analyses
Physical and Cognitive Data
The statistical analyses of the behavioral data were performed using SPSS 23.0 for Windows (SPSS Inc, Chicago, Illinois, USA), the R 3.5.2 software (The R Foundation for Statistical Computing, Vienna, Austria) and the package nparLD (86, 87). A p-value of p < 0.05 was considered significant. Per protocol analyses were performed. Adherence rate to the intervention was assessed with the training diary. For inclusion into analysis, a training adherence rate of 70% was predefined for the participants.
Descriptive statistics (median and interquartile range or frequencies) were reported for all data. The baseline group differences in the anthropometric data were tested using Mann Whitney U test or 2-dimensional (Person) Chi Square test. As common assumptions, in particular normality distribution of residuals and homogeneity of variance, were violated in most data, we used a rank-based non-parametric variance analysis (ANOVA-type statistic). The interaction effect of group (training, control) and time (pre, post) was investigated using the method described by Brunner and Langer (88) for non-parametric (rank-based) analysis of longitudinal data in factorial designs. Descriptions of how this approach works can be found elsewhere (88, 89). In case of a significant interaction effect, the Wilcoxon signed-rank test was used for post hoc analyses to investigate the within-group differences. For the ANOVA-type statistic, effect size was calculated by partial eta-square (), expressing the amount of variance explained in the dependent variables by the respective effect (90). For the post hoc analyses, effect size r according to Cohen (91) was reported. Effect size r = 0.10 indicates a small effect, r = 0.30 a medium effect, and r ≥ 0.50 a large effect. For exploratory reasons, within-group pre- vs. post-comparisons and effect sizes r have been calculated for all the functional measures.
MRI Data
For statistical analyses of the MRI data, the VBM12 toolbox was used (Voxel-Based Morphometry; Structural Brain Mapping Group, Department of Psychiatry, University of Jean, Germany) of SPM12 (Statistical Parametric Mapping; The Wellcome Trust Centre for Neuroimaging, Institute of Neurology, University College London, London, United Kingdom) running under MATLAB R2017b (The Mathworks, Natick, Massachusetts, USA). Following the preprocessing of MRI images, standard parametric procedures were used, as the residuals are most likely to be normally distributed once the segmented images have been smoothed. A two-way repeated measures ANOVA with group (training, control) as between-subject factor and time (pre, post) as within-subject factor was calculated for the preprocessed GM images for predefined regions of interest (ROI). These ROIs were the hippocampus, frontal lobe, and cerebellum, for which masks were taken in MNI space from the wfu-pickatlas (92). Total intra-cranial volume, age and sex were included as covariates of no interest in all the analyses to correct for brain volume differences related to age and sex. All analyses were thresholded with a family-wise error (FWE)-corrected voxel significance level of p < 0.05. In addition, the repeated measures ANOVA was conducted for total brain GM volume as well as for hippocampal volume (in mm3).
To determine differences in change of GM volume between the training and control group after intervention, the interaction effect of time x group was reported. Furthermore, the main effect of time was reported to evaluate GM volume changes over time in both groups (age-related atrophy). Effect sizes were calculated by partial eta-square () (90).
All data generated and analyzed during this study are included in this published article.
Results
Out of the 37 older adults who were randomized (training, control), 31 participants completed the 16-week intervention period (mean age = 73.9 ± 6.4 years, range = 65–90 years, 16 female). Figure 2 shows the study flow chart. Since the six drop-outs were equally distributed to both groups and clear reasons for not completing the study were known, these participants were excluded from analysis. The drop-outs (mean age = 73.5 ± 8.0 years, range = 65–83 years, 5 female) were comparable to the remaining participants regarding their characteristics (p > 0.05). Table 1 summarizes baseline demographic data and screening values of participants who were included in the analysis. The two groups differed at baseline in age (p = 0.005), fear of falling (p = 0.013), and self-evaluation of balance (p = 0.049). All participants in the training group adhered to 70% or more of the total training sessions.
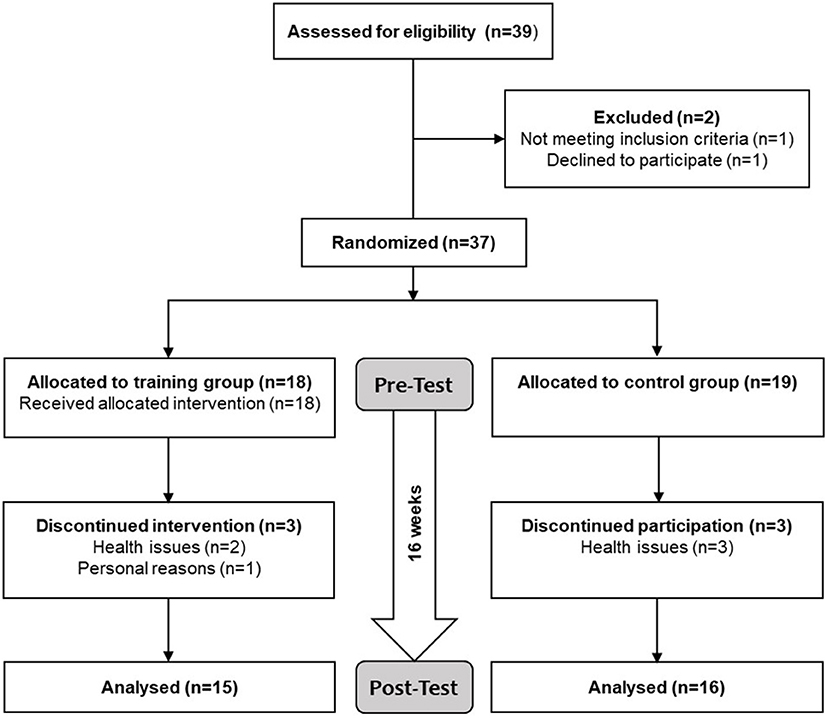
Figure 2. Study flow chart. Screening for eligibility included a health questionnaire and the Mini Mental Status Examination. Eligible participants were randomly assigned to either training or control group. The participants of the training group trained 3x/week à 30–40 min for 16 weeks while the participants of the control group continued with their normal daily living. Physical and cognitive functions as well as brain volume were assessed before and after the intervention period.
Physical and Cognitive Functions
The descriptive statistics (median values and interquartile ranges) of all physical and cognitive measures are presented in Tables 2, 3, which include the results from the non-parametric variance analysis. For the dual-task gait analysis, data of one participant from the training group had to be excluded due to a violation of the testing protocol (prioritizing one task above the other). Due to technical issues, for two participants, data of one foot were used instead of averaging data of left and right foot.
Non-parametric variance analysis showed no significant interaction effects for any of the physical function measures (p > 0.05) with the exception of an interaction effect in the 30 s chair rises test [F(1) = 5.076, p = 0.024, = 0.01]. Post hoc analyses revealed no significant within-group changes comparing pre- and post-measurements.
Regarding cognitive functions, a significant interaction effect was present in the VST 3 [F(1) = 2.537, p = 0.046, = 0.11]. Post hoc analyses revealed that the training group significantly improved in VST 3 after intervention (z = −3.051, p = 0.001, r = 0.56), whereas the control group did not (z = −1.885, p = 0.060, r = 0.33). Significant interaction effects were found in the WMS-R forward score [F(1) = 8.504, p = 0.004, = 0.04] and WMS-R forward span [F(1) = 4.309, p = 0.038, = 0.03]. Within-group pre-post comparisons showed that the control group significantly improved in WMS-R forward score (z = −2.268, p = 0.023, r = 0.40), whereas the training group did not (z = −1.409, p = 0.159, r = 0.26). A significant interaction effect was present in the WMS-R backward span [F(1) = 5.872, p = 0.015, = 0.02]. Post hoc analyses revealed that the training group performed significantly better in WMS-R backward span after the intervention (z = −2.126, p = 0.033, r = 0.39), whereas the control group did not (z = −0.432, p = 0.666, r = 0.08). Further exploratory analyses (within-group pre- vs. post-comparisons for all functional measures) are shown in the Supplementary Material.
Brain Volume
Eleven participants could not conduct MRI measurements or their MRI images could not be analyzed due to metal pieces in the body from surgeries (4 participants), claustrophobia (1 participant), intake of antidepressants (1 participant), loss of data (1 participant), rejection of MRI measurement due to personal reasons (2 participants) or dropping out from the study due to health-related issues (2 participants). No participant was excluded due to brain abnormalities or movement artifacts. MRI data of 12 participants of the training group and 14 participants of the control group were finally analyzed.
Repeated measures ANOVA did not reveal significant GM volume differences between the training and control group after intervention in the ROI analyses of the frontal cortex, the hippocampus and the cerebellum (significance level p < 0.05, FWE-corrected). Table 4 shows the significant main effects of time in frontal and hippocampal brain areas. Both groups showed a decrease in brain volume in these areas (right orbitofrontal cortex, right and left ventrolateral and ventromedial prefrontal cortex, left hippocampus) at the second measurement compared to the first.
For extracted GM volumes (total brain GM and hippocampal volume), further analyses did not show a significant time x group interaction or a significant main effect of time on the total brain GM volume or the hippocampal volume (see Table 5). For analysis of the hippocampal volume, one participant was considered as outlier with a value > +2 standard deviations and was replaced by the mean.
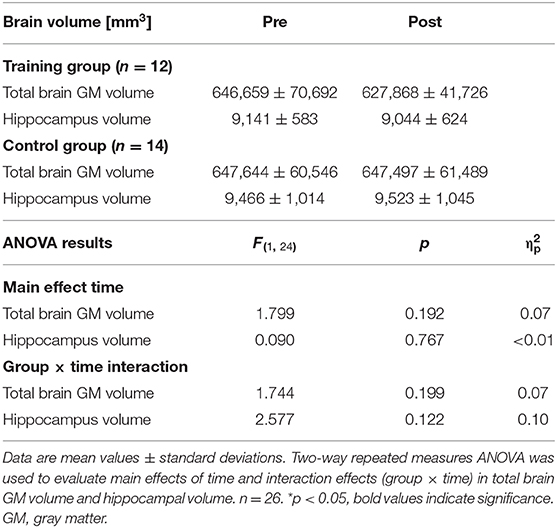
Table 5. Main effects of time and interaction effects (group x time) in total brain gray matter volume and hippocampal volume.
Other Outcomes
The subjectively reported activity, derived from the biweekly phone calls, were averaged to hours/week (h/w) over the study period. Participants of the training group reported an average cognitive activity (e.g., playing chess, learning a language, using an app) of 2.8 ± 0.7 h/w and 1.5 ± 0.5 h/w of intense physical activity (e.g., running or playing tennis) and 4.4 ± 0.6 h/w of moderate physical activity (e.g., walking or gardening). Participants of the control group reported to be on average 3.3 ± 0.6 h/w cognitively active and an intense physical activity of 1.4 ± 0.4 h/w and a moderate physical activity of 6.3 ± 1.1 h/w. Mann Whitney U tests showed no significant group differences in all three categories (p > 0.05).
During the intervention period, none of the participants suffered any adverse events related to the study intervention, especially no falls or injuries during measurements or training. Moreover, no fear of falling during exergame training was reported. Eight participants reported to have experienced a “critical moment” where they almost fell (e.g., tripping, slipping, swaying). Most “critical moments” occurred during strength exercises in a lunge position or during whole body rotations. Dizziness during training was reported by three participants (related to body rotations or exhaustion). Slight pain during training was stated by five participants whereas in two cases, the pain was related to the IMU attachment to the ankles with a wrong-size slap band. The other three participants reported slight knee and/or back pain.
Discussion
In this study, we investigated the impact of a newly developed home-based exergame training on physical functions, cognition and brain volume compared to usual care in healthy older adults. The training group completed a 4-month intervention with physical-cognitive training embedded in the exergame. Our results showed an improvement in higher-order cognitive functions (executive functions) after exergame training compared to non-training controls. No training-related improvements were evident in physical functions or brain volume. However, the analysis of brain volume showed a significant reduction of frontal and hippocampal brain volume over time in both groups. In the following, we will discuss these findings, put the results in the context of existing knowledge and draw consequences for future studies using similar approaches.
Training Effects on Cognitive Functions
Our results showed significant time x group interactions in favor of the training group for two measures of higher-order cognitive functions; inhibition and working memory. Inhibition is related to the capability to inhibit irrelevant information and responses, sometimes called interference control, as interfering information might trigger responses, which have to be controlled (93). Working memory means to memorize/maintain and manipulate information (“work” on them), also referred to as updating (93). Both cognitive skills are part of the executive functions, which are mainly controlled by frontal brain areas. Cognitive aging is accompanied by a decline, among others, in executive functions whereas a link can be hypothesized to the age-related changes in the frontal cortex (3). Executive functions play an important role in guiding through everyday life including problem-solving and planning and controlling of actions (93). Moreover, they contribute to safe gait as a higher risk of falling was observed in subjects with cognitive impairments including executive functioning deficits (94–98). Thus, the improvement of executive functioning in this study might be an outcome of high relevance as strengthened executive functions could enhance daily life performance of older adults.
Several other studies including combined physical-cognitive training as exergaming indicated that this training approach can boost cognitive functions, particularly executive functions (99–103). Thus, our study results are in line with the evidence provided by recent reviews and meta-analyses, which reported significant improvements in executive functions after exergame training (52, 53, 104). This might be related to the cognitive stimulation more or less explicitly embedded in the game scenario of exergames. But also coordinative exercises such as dancing as well as aerobic and strength exercises have been shown to improve cognitive functions (12, 105–111). In the Active@Home exergame, several components were included with rather high cognitive demands (step-based cognitive training, dancing, Tai Chi-inspired strength exercises). All components could have contributed to the improved cognitive functions in varying proportions and/or in synergistic interactions.
One executive function – mental flexibility – showed no significant time x group interaction in this intervention study which is in line with the results of a previous exergame study (101). Mental flexibility is related to the capability to switch between different tasks and to process and manipulate multiple information (93). Three possible explanations for the missing improvement should be mentioned: (a) The cognitive training was not challenging enough to train this cognitive function. (b) The improvement could not be assessed by the applied cognitive test supporting the need to use several cognitive tests to assess a cognitive outcome of interest as a latent construct behind the different tests (12). (c) Nevertheless, in further exploratory analyses comparing pre- vs. post-measurements within groups, the training group showed significant improvement in mental flexibility after the intervention, the control group did not. Thus, another explanation could be that the training effect was just not strong enough to get evident in a significant interaction effect.
A further, however rather surprisingly finding was the significant time x group interaction in the short-term attentional span in favor of the control group. As the control group was a passive control group and (physical and cognitive) activities beside of the activity due to the exergame intervention (in the training group) were assessed and comparable in both groups, activity-related improvements are likely to be excluded. Kent recently reviewed the WMS-R, of which the subtests to assess attentional span (WMS-R digit span forward) and working memory (WMS-R digit span backward) have been applied (112). Concerns have been expressed regarding the reliability and validity of this test battery, even though it is clinically used for over 75 years. Thus, caution might be required regarding the results measured with these subtests.
Training Effects on Physical Functions
Another rather surprising result of this study was that no significant improvements got evident for any of the measured physical functions (gait parameters, muscle strength, balance and endurance). Previous studies have reported improvements in several physical functions of older adults after exergame training (e.g., in cardiovascular or musculoskeletal system) depending on the physical components and exercises that are integrated in the training (e.g., stepping or cycling) (54–56, 113). In exergame studies with similar interventions than in ours, gait parameters as walking speed have been shown to be enhanced after exergame training, especially under dual-task walking (103, 114, 115). Exergaming is discussed to train dual-task abilities, which are important in daily life activities and for safe gait and fall prevention in older adults (116, 117). Even though no significant interaction effects were evident in this study for gait parameters, in further exploratory analyses of pre- vs. post-comparisons within groups, the training group showed significant improvements in several gait parameters (walking speed, stride length and cycle duration) under dual-task walking in line with previous research. One might speculate that the effects have not been strong enough to get evident in the results of the variance analysis. However, the control group individuals showed a baseline walking speed around 1.4 m/s and, thus, seem to be “high performers” compared to reference values of walking speed for older adults, meaning there is little room for improvement (118). The gait improvements within the training group might be related to the improved executive functioning together with their lower baseline values.
Even though the physical exercises incorporated in the Active@Home exergame are supposed to target balance and strength, no improvements were evident in these functions. For example, dancing has been shown to positively influence coordinative skills and balance, whereas strength training with exercises similar to the ones incorporated in our intervention has been demonstrated to be effective in older adults (119–122). Also after step-based games, requiring the execution of rapid and well-directed steps, improvements in balance have been reported (123, 124). Moreover, in a previous pilot study of the Active@Home exergame (59), heart rate measures during training showed moderate physical intensity (an average heart rate of about 60% of the estimated maximal heart rate of the elderly participants), which is recommended to improve cardiovascular fitness (68). Nevertheless, no enhancement in aerobic endurance was evident in this study. Three aspects should be discussed related to the missing improvements in endurance, strength and balance.
One assumption is that insensitive and unsuitable assessments have been used. Some of the applied tests were originally designed for moderate-performing older adults or for clinical setting. Thus, they might have not been able to assess a sufficient range of performance in healthy (high-performing) older adults and to detect subtle differences (e.g., due to ceiling effects). For example, the SPPB was developed to assess older adults with low functionality and to evaluate their risk of disability (75). The SFT was developed for early detection of decline in physical functions (78, 125). Moreover, to assess strength and endurance, functional measures (rising from a chair, stepping) were used. Other measurement methods might have been much more accurate (e.g., leg press or maximal oxygen consumption). Another assumption relates to the duration of the intervention. Even though other exergame studies showed improvements in physical functions after several weeks of training and a very comparable training amount (e.g., min/week), prolonged intervention period might have uncover (greater) training effects (113). A last assumption may relate to the training load. An individually adapted, optimal training load has been discussed to be crucial to maximize training benefits and improve motor skills and physical fitness (72, 126, 127). Moreover, due to training-related improvements in the course of training, the training load has to be continuously adapted. This continuous adaptation leads to a progressively increasing training load. Participants in this study have been instructed that the current training load respective the chosen difficulty level should always provide an optimal challenge avoiding under- or overload. The exergame included various difficulty levels of exercises arranged in a progressive order. However, the training load and its progressive adaptation were not monitored or supervised and based on subjective estimates of the participants rather than on objective measurements (e.g., percentage of the individual maximal heart rate or the individual one repetition maximum). We hypothesize that some participants may have trained under their optimal load due to difficulties with self-perception and -evaluation. Additionally, although the Active@Home exergame training should provide moderate intense physical exercises based on the heart rate measures during training in a pilot study (59), it might be suggested, that higher intensity levels would have been required for physical adaptations.
Structural Plasticity Effects
The study results revealed no increase in brain volume after exergame training. However, previous animal and human studies showed structural brain alternations after a variety of physical exercises in gray and white matter; for example after aerobic training (13, 24, 128, 129), after strength training (15), after coordinative exercises (130–134), after dancing (111, 121, 135) or after Tai Chi (136). Moreover, changes in brain structure have been reported after cognitive training (29–33, 137). To the best of our knowledge, only two studies have been conducted assessing the effects of exergame training on brain structure of older adults (57, 58). Ji and colleagues showed an increase in brain volume in fontal areas after Nintendo Wii training (57). Anderson-Hanley and colleagues reported similar findings after training with a specifically developed exergame (stationary bicycles with an interactive virtual component) (58). They also showed increased BDNF levels after exergame training. As exergame training combines physical exercise with cognitive stimulation, synergistic effects on neuroplasticity are assumed. Even though physical and cognitive training both seem to positively influence the brain, the underlying mechanisms might be different (36, 138). Indeed, physical activity has been shown to trigger cell proliferation of precursor cells and thereby inducing neurogenesis whereas cognitive stimulation seem to promote the survival and integration of the newborn cells into the functional networks (46, 47, 139). Based on these findings, the “facilitation-guidance-model” was proposed (46, 47): Physical exercise is assumed to facilitate neuroplastic processes while cognitive stimulation might guide the plastic changes. Although the Active@Home exergame incorporated combined physical-cognitive training, no positive influence on brain volume was evident after the 4-month intervention. Three potential reasons should be discussed.
First, most of the studies showing improvements in brain structure included longer intervention periods (6–12 months) (13, 15, 58, 111, 121, 133, 134). Hippocampal volume seems to be sensitive to the intervention duration: In a sample of older adults, an almost linear development in hippocampal volume enhancement was demonstrated after 6 and 12 months of aerobic training (13). In cognitive training studies, however intervention durations were around several weeks (29–33, 137). Thus, one might speculate that a longer training period with the Active@Home exergame could have led to effects on older adults' brain volume. Second, effects on the brain might have been evident, but they could probably not be measured by the applied measurement method. Other neuronal outcome measures might have been included (e.g., serum BDNF or IGF-1, diffusion tension imaging for white matter integrity and functional MRI). Third, the missing improvement in brain volume may relate to the training intensity. In general, moderate physical intensity is recommended for health benefits in older adults (corresponding to a heart rate of about 60–80% of the maximal heart rate) (68). An animal study by Ferris and colleagues showed that BDNF increase might be exercise intensity-dependent with higher intensity leading to higher expression (140). BDNF is known to trigger neuroplastic effects as synaptogenesis and neurogenesis (18, 141). Thus, it can speculated that higher physical training intensity would have shown (larger) positive effects. However, Ruscheweyh and colleagues compared outcomes of older adults engaged either in a medium- or a low-intense physical exercise to a no training control group (142). They found beneficial effects of physical activity independent of the intensity on cognitive functions and on frontal brain structure. Another study by Lövden and colleagues showed a positive effect on hippocampal volume after an exergame training with a very low physical intensity (slow walking) (143). Thus, the relationship between physical intensity and brain changes remains unclear. Nevertheless, indisputable is the fact that intense (metabolically demanding) exercises trigger adaptations in the cardiovascular and respiratory system, the metabolism and the immune system, and that, in turn, might have indirect effects on brain metabolism and processes (144, 145).
Moreover, this study revealed a significant time effect on brain volume. The ROI-analysis showed a reduction of frontal and hippocampal brain volume over time in both groups which has been observed in other interventional studies (32). This decrease in brain volume is consistent with numerous cross-sectional and longitudinal studies of aging (3, 146, 147). For example, Fjell and Waldhove reported an annual reduction of brain volume between 0.5 and 1.0% in most brain areas in older adults (3). Thus, this study result points to the brain degradation that seems to occur during normal aging.
Limitations
One of the limitations of this study was that only a passive rather than an active control group was included. Nevertheless, social contact was kept stable between the two groups as exergame training was conducted at participants' homes (no supervised training sessions) and participants of both groups were regularly called to assess activities and safety issues. Another study limitation might be related to the choice of outcome measurements. On one hand, more than one test could have been used to assess an outcome of interest, thereby evaluating an underlying latent construct and increasing validity (12). On the other hand, no measures of daily life functioning have been included (28), meaning that transfer effects to daily life activities, which are tremendously important, have not been assessed. Moreover, some applied measurement methods have been originally developed for clinical setting, thus limiting the sensitive assessment of high performance. Regarding neuronal outcome measures, other outcomes than gray matter brain volume (e.g., white matter microstructure, BDNF and IGF-1) could have been considered. Additionally, relationship (potential correlation) between brain alterations and changes in performance should be assessed in future studies to highlight the meaningfulness of neuronal changes. Considering the rather fit participants in the study sample, ceiling effects in performance might have restricted informative value. Moreover, attention should be paid regarding generalization of the study results to overall older adults, again due to the rather fit and high-performing participants in this study. Another limitation of this study might be related to the rather small sample size, which might have limited the detection of significant training effects. Finally, during the exergame training, individual and progressive adaptation of training load was neither monitored nor based on objective measures. Further studies, especially with technology-based interventions, should consider automatic load adaptation.
Conclusion
To prevent age-related decline and maintain brain health, continuous active interaction with environments that are demanding to sensory, motor and cognitive systems is necessary. Training-induced plasticity is evident on behavioral as well as on neuronal level also in higher age. Exergame training seems to be a motivating and promising option for simultaneous physical-cognitive training in older adults. Our study showed an improvement in higher-order cognitive functions (executive functions) after exergame training compared to the passive control group. While comparing our study results to the findings of other exergame studies, it got evident that this comparison is limited due to a large heterogeneity in exergame characteristics. Even though physical exercise is by definition a part of exergame training, the physical exercise component of an exergame might be very different in various exergames. One exergame training might be based on walking, running or cycling, another exergame training might require balance-shifts, stepping or jumping. Thereby, physical activity embedded in an exergame can be more or less intense respective metabolically demanding. The same heterogeneity can be found for cognitive stimulation incorporated in exergame training. Exergaming might require very basal but also rather specific and complex cognitive functions and processes. To summarize, a huge variety exists in physical and cognitive demands of exergame training. Therefore, benefits of exergame training might vary depending on the exergame characteristics. Especially regarding neuronal outcomes, different mechanisms might be triggered by exergames with various metabolic and cognitive demands. Further research is needed to assess the training conditions and requirements of exergame training to optimally enhance performance and brain structure. We suggest that regular physical-cognitive training with an individually adapted optimal training challenge might lead to both, greater improvements in cognitive and physical functions as well as measurable changes in brain structure.
Data Availability Statement
All datasets generated for this study are included in the article/Supplementary Material.
Ethics Statement
The studies involving human participants were reviewed and approved by Ethics committee of Nordwest- und Zentralschweiz (EKNZ, 2018-00510). The participants provided their written informed consent to participate in this study.
Author Contributions
MA, MF, and JP developed the research question under the lead of EB. The concept and design were established by MA, MF, and JP, while AS, EB, and AL acted as methodological council. MA, MF, and JP conducted data acquisition, analysis, and interpretation of the results with edition and improvements by AS, EB, and KL. KL supported the MRI data analysis and interpretation. LZ was the study physician checking all MRI images for structural abnormalities and pathologies. VG was mainly responsible for exergame development including algorithms for movement evaluation. During the study intervention, VG provided technical support. MA produced the first version of the manuscript. AS and EB substantially revised the manuscript to bring it to its current version. All authors have read and approved the final manuscript.
Funding
This work was partially supported by the AAL Active@Home project (www.aal-europe.eu/projects/activehome/), funded by the European Commission and co-funded by the Swiss Confederation represented by the State Secretariat for Education, Research and Innovation (SERI; agreement number 1315000595).
Conflict of Interest
The authors declare that the research was conducted in the absence of any commercial or financial relationships that could be construed as a potential conflict of interest.
Acknowledgments
The authors would like to thank all participants for their kindness and patience during the testing and training sessions. The authors would like to thank also all the national and international partners involved in the Active@Home project and exergame development. Moreover, the authors would like to thank Melanie Thalmann for supporting this study including participants' care and manuscript preparation.
Supplementary Material
The Supplementary Material for this article can be found online at: https://www.frontiersin.org/articles/10.3389/fmed.2019.00321/full#supplementary-material
References
1. He W, Goodkind D, Kowal P. An Aging World: 2015. International Publication Reports. Washington, DC: U.S. Census Bureau (2016).
2. Harada CN, Love MCN, Triebel KL. Normal cognitive aging. Clin Geriatr Med. (2013) 29:737–52. doi: 10.1016/j.cger.2013.07.002
3. Fjell AM, Walhovd KB. Structural brain changes in aging: courses, causes and cognitive consequences. Rev Neurosci. (2010) 21:187–222. doi: 10.1515/REVNEURO.2010.21.3.187
4. Reuter-Lorenz PA, Cooke KA. Neuropsychology of aging, past, present and future: contributions of Morris Moscovitch. Neuropsychologia. (2016) 90:117–24. doi: 10.1016/j.neuropsychologia.2016.06.018
5. Brehmer Y, Kalpouzos G, Wenger E, Lövdén M. Plasticity of brain and cognition in older adults. Psychol Res. (2014) 78:790–802. doi: 10.1007/s00426-014-0587-z
6. Hertzog C, Kramer AF, Wilson RS, Lindenberger U. Enrichment effects on adult cognitive development: can the functional capacity of older adults be preserved and enhanced? Psychol Sci Public Interest. (2008) 9:1–65. doi: 10.1111/j.1539-6053.2009.01034.x
7. Pascual-Leone A, Amedi A, Fregni F, Merabet LB. The plastic human brain cortex. Annu Rev Neurosci. (2005) 28:377–401. doi: 10.1146/annurev.neuro.27.070203.144216
8. Cai L, Chan JS, Yan JH, Peng K. Brain plasticity and motor practice in cognitive aging. Front. Aging Neurosci. (2014) 6:31. doi: 10.3389/fnagi.2014.00031
9. Warburton DE, Nicol CW, Bredin SS. Health benefits of physical activity: the evidence. Can Med Assoc J. (2006) 174:801–9. doi: 10.1503/cmaj.051351
10. Chang Y-K, Pan C-Y, Chen F-T, Tsai C-L, Huang C-C. Effect of resistance-exercise training on cognitive function in healthy older adults: a review. J Aging Phys Act. (2012) 20:497–517. doi: 10.1123/japa.20.4.497
11. Netz Y, Wu M-J, Becker BJ, Tenenbaum G. Physical activity and psychological well-being in advanced age: a meta-analysis of intervention studies. Psychol Aging. (2005) 20:272. doi: 10.1037/0882-7974.20.2.272
12. Kramer AF, Colcombe S. Fitness effects on the cognitive function of older adults: a meta-analytic study—revisited. Perspect. Psychol. Sci. (2018) 13:213–7. doi: 10.1177/1745691617707316
13. Erickson KI, Voss MW, Prakash RS, Basak C, Szabo A, Chaddock L, et al. Exercise training increases size of hippocampus and improves memory. Proc Natl Acad Sci USA. (2011) 108:3017–22. doi: 10.1073/pnas.1015950108
14. Bherer L, Erickson KI, Liu-Ambrose T. A review of the effects of physical activity and exercise on cognitive and brain functions in older adults. J Aging Res. (2013) 2013:657508. doi: 10.1155/2013/657508
15. Best JR, Chiu BK, Hsu CL, Nagamatsu LS, Liu-Ambrose T. Long-term effects of resistance exercise training on cognition and brain volume in older women: results from a randomized controlled trial. J Int Neuropsychol Soc. (2015) 21:745–56. doi: 10.1017/S1355617715000673
16. Voss MW, Nagamatsu LS, Liu-Ambrose T, Kramer AF. Exercise, brain, and cognition across the life span. J Appl Physiol. (2011) 111:1505–13. doi: 10.1152/japplphysiol.00210.2011
17. Thomas A, Dennis A, Bandettini PA, Johansen-Berg H. The effects of aerobic activity on brain structure. Front Psychol. (2012) 3:86. doi: 10.3389/fpsyg.2012.00086
18. Cotman CW, Berchtold NC, Christie L-A. Exercise builds brain health: key roles of growth factor cascades and inflammation. Trends Neurosci. (2007) 30:464–72. doi: 10.1016/j.tins.2007.06.011
19. Lista I, Sorrentino G. Biological mechanisms of physical activity in preventing cognitive decline. Cell Mol Neurobiol. (2010) 30:493–503. doi: 10.1007/s10571-009-9488-x
20. Knaepen K, Goekint M, Heyman EM, Meeusen R. Neuroplasticity—exercise-induced response of peripheral brain-derived neurotrophic factor. Sports Med. (2010) 40:765–801. doi: 10.2165/11534530-000000000-00000
21. Black JE, Isaacs KR, Anderson BJ, Alcantara AA, Greenough WT. Learning causes synaptogenesis, whereas motor activity causes angiogenesis, in cerebellar cortex of adult rats. Proc Natl Acad Sci USA. (1990) 87:5568–72. doi: 10.1073/pnas.87.14.5568
22. Van Praag H, Kempermann G, Gage FH. Running increases cell proliferation and neurogenesis in the adult mouse dentate gyrus. Nat Neurosci. (1999) 2:266. doi: 10.1038/6368
23. Eadie BD, Redila VA, Christie BR. Voluntary exercise alters the cytoarchitecture of the adult dentate gyrus by increasing cellular proliferation, dendritic complexity, and spine density. J Comp Neurol. (2005) 486:39–47. doi: 10.1002/cne.20493
24. Van Praag H, Shubert T, Zhao C, Gage FH. Exercise enhances learning and hippocampal neurogenesis in aged mice. J Neurosci. (2005) 25:8680–5. doi: 10.1523/JNEUROSCI.1731-05.2005
25. Pereira AC, Huddleston DE, Brickman AM, Sosunov AA, Hen R, McKhann GM, et al. An in vivo correlate of exercise-induced neurogenesis in the adult dentate gyrus. Proc Natl Acad Sci USA. (2007) 104:5638–43. doi: 10.1073/pnas.0611721104
26. Bediou B, Adams DM, Mayer RE, Tipton E, Green CS, Bavelier D. Meta-analysis of action video game impact on perceptual, attentional, and cognitive skills. Psychol Bull. (2018) 144:77. doi: 10.1037/bul0000130
27. Nguyen L, Murphy K, Andrews G. Cognitive and neural plasticity in old age: a systematic review of evidence from executive functions cognitive training. Ageing Res Rev. (2019) 53:100912. doi: 10.1016/j.arr.2019.100912
28. Reijnders J, van Heugten C, van Boxtel M. Cognitive interventions in healthy older adults and people with mild cognitive impairment: a systematic review. Ageing Res Rev. (2013) 12:263–75. doi: 10.1016/j.arr.2012.07.003
29. Engvig A, Fjell AM, Westlye LT, Moberget T, Sundseth Ø, Larsen VA, et al. Effects of memory training on cortical thickness in the elderly. Neuroimage. (2010) 52:1667–76. doi: 10.1016/j.neuroimage.2010.05.041
30. Lövdén M, Bodammer NC, Kühn S, Kaufmann J, Schütze H, Tempelmann C, et al. Experience-dependent plasticity of white-matter microstructure extends into old age. Neuropsychologia. (2010) 48:3878–83. doi: 10.1016/j.neuropsychologia.2010.08.026
31. Engvig A, Fjell AM, Westlye LT, Moberget T, Sundseth Ø, Larsen VA, et al. Memory training impacts short-term changes in aging white matter: a longitudinal diffusion tensor imaging study. Hum Brain Mapp. (2012) 33:2390–406. doi: 10.1002/hbm.21370
32. Lampit A, Hallock H, Suo C, Naismith SL, Valenzuela M. Cognitive training-induced short-term functional and long-term structural plastic change is related to gains in global cognition in healthy older adults: a pilot study. Front Aging Neurosci. (2015) 7:14. doi: 10.3389/fnagi.2015.00014
33. Kühn S, Lorenz RC, Weichenberger M, Becker M, Haesner M, O'Sullivan J, et al. Taking control! Structural and behavioural plasticity in response to game-based inhibition training in older adults. NeuroImage. (2017) 156:199–206. doi: 10.1016/j.neuroimage.2017.05.026
34. Brown J, Cooper-Kuhn CM, Kempermann G, Van Praag H, Winkler J, Gage FH, et al. Enriched environment and physical activity stimulate hippocampal but not olfactory bulb neurogenesis. Eur J Neurosci. (2003) 17:2042–6. doi: 10.1046/j.1460-9568.2003.02647.x
35. Ehninger D, Kempermann G. Regional effects of wheel running and environmental enrichment on cell genesis and microglia proliferation in the adult murine neocortex. Cerebral Cortex. (2003) 13:845–51. doi: 10.1093/cercor/13.8.845
36. Olson AK, Eadie BD, Ernst C, Christie BR. Environmental enrichment and voluntary exercise massively increase neurogenesis in the adult hippocampus via dissociable pathways. Hippocampus. (2006) 16:250–60. doi: 10.1002/hipo.20157
37. Wolf SA, Kronenberg G, Lehmann K, Blankenship A, Overall R, Staufenbiel M, et al. Cognitive and physical activity differently modulate disease progression in the amyloid precursor protein (APP)-23 model of Alzheimer's disease. Biol Psychiatry. (2006) 60:1314–23. doi: 10.1016/j.biopsych.2006.04.004
38. Korol DL, Gold PE, Scavuzzo CJ. Use it and boost it with physical and mental activity. Hippocampus. (2013) 23:1125–35. doi: 10.1002/hipo.22197
39. Law LL, Barnett F, Yau MK, Gray MA. Effects of combined cognitive and exercise interventions on cognition in older adults with and without cognitive impairment: a systematic review. Ageing Res Rev. (2014) 15:61–75. doi: 10.1016/j.arr.2014.02.008
40. Lauenroth A, Ioannidis AE, Teichmann B. Influence of combined physical and cognitive training on cognition: a systematic review. BMC Geriatr. (2016) 16:1. doi: 10.1186/s12877-016-0315-1
41. Levin O, Netz Y, Ziv G. The beneficial effects of different types of exercise interventions on motor and cognitive functions in older age: a systematic review. Eur Rev Aging Phys Activ. (2017) 14:20. doi: 10.1186/s11556-017-0189-z
42. Tait JL, Duckham RL, Milte CM, Main LC, Daly RM. Influence of sequential vs. simultaneous dual-task exercise training on cognitive function in older adults. Front Aging Neurosci. (2017) 9:368. doi: 10.3389/fnagi.2017.00368
43. Herold F, Hamacher D, Schega L, Mueller NG. Thinking while moving or moving while thinking–concepts of motor-cognitive training for cognitive performance enhancement. Front Aging Neurosci. (2018) 10:228. doi: 10.3389/fnagi.2018.00228
44. Joubert C, Chainay H. Aging brain: the effect of combined cognitive and physical training on cognition as compared to cognitive and physical training alone–a systematic review. Clin Interv Aging. (2018) 13:1267. doi: 10.2147/CIA.S165399
45. Schaefer S, Schumacher V. The interplay between cognitive and motor functioning in healthy older adults: findings from dual-task studies and suggestions for intervention. Gerontology. (2011) 57:239–46. doi: 10.1159/000322197
46. Fabel K, Kempermann G. Physical activity and the regulation of neurogenesis in the adult and aging brain. Neuromolecular Med. (2008) 10:59–66. doi: 10.1007/s12017-008-8031-4
47. Kempermann G, Fabel K, Ehninger D, Babu H, Leal-Galicia P, Garthe A, et al. Why and how physical activity promotes experience-induced brain plasticity. Front Neurosci. (2010) 4:189. doi: 10.3389/fnins.2010.00189
48. Benzing V, Schmidt M. Exergaming for children and adolescents: strengths, weaknesses, opportunities and threats. J Clin Med. (2018) 7:422. doi: 10.3390/jcm7110422
49. Brox E, Luque LF, Evertsen GJ, Hernández JEG. Exergames for elderly: social exergames to persuade seniors to increase physical activity. In Pervasive Computing Technologies for Healthcare (PervasiveHealth), 5th International Conference on: IEEE. Dublin (2011). p. 546–9.
50. Pirovano M, Surer E, Mainetti R, Lanzi PL, Borghese NA. Exergaming and rehabilitation: a methodology for the design of effective and safe therapeutic exergames. Entertain Comput. (2016) 14:55–65. doi: 10.1016/j.entcom.2015.10.002
51. Schutzer KA, Graves BS. Barriers and motivations to exercise in older adults. Prev Med. (2004) 39:1056–61. doi: 10.1016/j.ypmed.2004.04.003
52. Stanmore E, Stubbs B, Vancampfort D, de Bruin ED, Firth J. The effect of active video games on cognitive functioning in clinical and non-clinical populations: a meta-analysis of randomized controlled trials. Neurosci Biobehav Rev. (2017) 78:34–43. doi: 10.1016/j.neubiorev.2017.04.011
53. Stojan R, Voelcker-Rehage C. A systematic review on the cognitive benefits and neurophysiological correlates of exergaming in healthy older adults. J Clin Med. (2019) 8:734. doi: 10.3390/jcm8050734
54. Larsen LH, Schou L, Lund HH, Langberg H. The physical effect of exergames in healthy elderly—a systematic review. Games Health J. (2013) 2:205–12. doi: 10.1089/g4h.2013.0036
55. Street TD, Lacey SJ, Langdon RR. Gaming your way to health: a systematic review of exergaming programs to increase health and exercise behaviors in adults. Games Health J. (2017) 6:136–46. doi: 10.1089/g4h.2016.0102
56. Kappen DL, Mirza-Babaei P, Nacke LE. Older Adults' physical activity and exergames: a systematic review. Int J Hum Comput Interact. (2019) 35:1–28. doi: 10.1080/10447318.2018.1441253
57. Ji L, Zhang H, Potter GG, Zang Y-F, Steffens DC, Guo H, et al. Multiple neuroimaging measures for examining exercise-induced neuroplasticity in older adults: a quasi-experimental study. Front Aging Neurosci. (2017) 9:102. doi: 10.3389/fnagi.2017.00102
58. Anderson-Hanley C, Barcelos NM, Zimmerman EA, Gillen RW, Dunnam M, Cohen BD, et al. The Aerobic and Cognitive Exercise Study (ACES) for community-dwelling older adults with or at-risk for mild cognitive impairment (MCI): neuropsychological, neurobiological and neuroimaging outcomes of a randomized clinical trial. Front Aging Neurosci. (2018) 10:76. doi: 10.3389/fnagi.2018.00076
59. Adcock M, Thalmann M, Schättin A, Gennaro F, de Bruin ED. A pilot study of an in-home multicomponent exergame training for older adults: feasibility, usability and pre-post evaluation. Front. Aging Neurosci. (2019) 11:304. doi: 10.3389/fnagi.2019.00304
60. Campbell M, Fitzpatrick R, Haines A, Kinmonth AL, Sandercock P, Spiegelhalter D, et al. Framework for design and evaluation of complex interventions to improve health. BMJ. (2000) 321:694–6. doi: 10.1136/bmj.321.7262.694
61. Beller EM, Gebski V, Keech AC. Randomisation in clinical trials. Med J Aust. (2002) 177:565–7. doi: 10.5694/j.1326-5377.2002.tb04955.x
62. American Geriatrics Society BGS American Academy of Orthopaedic Surgeons Panel on Falls Prevention. Summary of the Updated American Geriatrics Society/British Geriatrics Society clinical practice guideline for prevention of falls in older persons. J Am Geriatr Soc. (2011) 59:148–57. doi: 10.1111/j.1532-5415.2010.03234.x
63. Sherrington C, Tiedemann A, Fairhall N, Close JC, Lord SR. Exercise to prevent falls in older adults: an updated meta-analysis and best practice recommendations. NSW Public Health Bull. (2011) 22:78–83. doi: 10.1071/NB10056
64. Brach M, Hauer K, Rotter L, Werres C, Korn O, Konrad R, et al. Modern principles of training in exergames for sedentary seniors: requirements and approaches for sport and exercise sciences. Int J Comput Sci Sport. (2011) 11:86–99. doi: 10.13140/RG.2.1.3762.2647
65. Shigematsu R, Chang M, Yabushita N, Sakai T, Nakagaichi M, Nho H, et al. Dance-based aerobic exercise may improve indices of falling risk in older women. Age Ageing. (2002) 31:261–6. doi: 10.1093/ageing/31.4.261
66. Wayne PM, Walsh JN, Taylor-Piliae RE, Wells RE, Papp KV, Donovan NJ, et al. Effect of Tai Chi on cognitive performance in older adults: systematic review and meta-analysis. J Am Geriatr Soc. (2014) 62:25–39. doi: 10.1111/jgs.12611
67. Dhami P, Moreno S, DeSouza JF. New framework for rehabilitation–fusion of cognitive and physical rehabilitation: the hope for dancing. Front Psychol. (2015) 5:1478. doi: 10.3389/fpsyg.2014.01478
68. Nelson ME, Rejeski WJ, Blair SN, Duncan PW, Judge JO, King AC, et al. Physical activity and public health in older adults: recommendation from the American College of Sports Medicine and the American Heart Association. Circulation. (2007) 116:1094. doi: 10.1249/mss.0b013e3180616aa2
69. Chodzko-Zajko WJ, Proctor DN, Singh MAF, Minson CT, Nigg CR, Salem GJ, et al. Exercise and physical activity for older adults. Med Sci Sports Exerc. (2009) 41:1510–30. doi: 10.1249/MSS.0b013e3181a0c95c
70. Bamidis P, Vivas A, Styliadis C, Frantzidis C, Klados M, Schlee W, et al. A review of physical and cognitive interventions in aging. Neurosci Biobehav Rev. (2014) 44:206–20. doi: 10.1016/j.neubiorev.2014.03.019
71. Tabei KI, Satoh M, Ogawa JI, Tokita T, Nakaguchi N, Nakao K, et al. Physical exercise with music reduces gray and white matter loss in the frontal cortex of elderly people: the Mihama-Kiho scan project. Front Aging Neurosci. (2017) 9:174. doi: 10.3389/fnagi.2017.00174
72. Healy AF, Kole JA, Bourne LE Jr. Training principles to advance expertise. Front Psychol. 5:131. doi: 10.3389/fpsyg.2014.00131
73. Dubost V, Kressig RW, Gonthier R, Herrmann FR, Aminian K, Najafi B, et al. Relationships between dual-task related changes in stride velocity and stride time variability in healthy older adults. Hum Mov Sci. (2006) 25:372–82. doi: 10.1016/j.humov.2006.03.004
74. Lindemann U, Najafi B, Zijlstra W, Hauer K, Muche R, Becker C, et al. Distance to achieve steady state walking speed in frail elderly persons. Gait Posture. (2008) 27:91–6. doi: 10.1016/j.gaitpost.2007.02.005
75. Guralnik JM, Ferrucci L, Pieper CF, Leveille SG, Markides KS, Ostir GV, et al. Lower extremity function and subsequent disability: consistency across studies, predictive models, and value of gait speed alone compared with the short physical performance battery. J Gerontol A Biol Sci Med Sci. (2000) 55:M221–31. doi: 10.1093/gerona/55.4.M221
76. Ostir GV, Volpato S, Fried LP, Chaves P, Guralnik JM. Reliability and sensitivity to change assessed for a summary measure of lower body function: results from the Women's Health and Aging Study. J Clin Epidemiol. (2002) 55:916–21. doi: 10.1016/S0895-4356(02)00436-5
77. Eggenberger P, Wolf M, Schumann M, de Bruin ED. Exergame and balance training modulate prefrontal brain activity during walking and enhance executive function in older adults. Front Aging Neurosci. (2016) 8:66. doi: 10.3389/fnagi.2016.00066
78. Rikli RE, Jones CJ. Development and validation of a functional fitness test for community-residing older adults. J Aging Phys Act. (1999) 7:129–61. doi: 10.1123/japa.7.2.129
79. Hesseberg K, Bentzen H, Bergland A. Reliability of the senior fitness test in community-dwelling older people with cognitive impairment. Physiother Res Int. (2015) 20:37–44. doi: 10.1002/pri.1594
80. Strauss E, Sherman EM, Spreen O. A Compendium of Neuropsychological Tests: Administration, Norms, and Commentary. (2006). American Chemical Society.
81. Bowie CR, Harvey PD. Administration and interpretation of the Trail Making Test. Nat Protoc. (2006) 1:2277–81. doi: 10.1038/nprot.2006.390
83. Ashburner J, Friston KJ. Voxel-based morphometry—the methods. Neuroimage. (2000) 11:805–21. doi: 10.1006/nimg.2000.0582
84. Ashburner J. A fast diffeomorphic image registration algorithm. Neuroimage. (2007) 38:95–113. doi: 10.1016/j.neuroimage.2007.07.007
85. Suppa P, Anker U, Spies L, Bopp I, Rüegger-Frey B, Klaghofer R, et al. Fully automated atlas-based hippocampal volumetry for detection of Alzheimer's disease in a memory clinic setting. J Alzheimer's Dis. (2015) 44:183–93. doi: 10.3233/JAD-141446
86. Noguchi K, Gel YR, Brunner E, Konietschke F. nparLD: an R software package for the nonparametric analysis of longitudinal data in factorial experiments. J Stat Softw. (2012) 50:1–23. doi: 10.18637/jss.v050.i12
87. Team RC. R: A Language and Environment for Statistical Computing. R Foundation for Statistical Computing, Vienna (2017) Available online at: https://www.R-project.org
88. Brunner EDS, Langer F. Nonparametric Analysis of Longitudinal Data in Factorial Experiments. New York, NY: Wiley (2002).
89. Brunner E, Puri ML. Nonparametric methods in factorial designs. Stat Papers. (2001) 42:1–52. doi: 10.1007/s003620000039
90. Lakens D. Calculating and reporting effect sizes to facilitate cumulative science: a practical primer for t-tests and ANOVAs. Front Psychol. (2013) 4:863. doi: 10.3389/fpsyg.2013.00863
91. Cohen J. Statistical Analysis for the Behavioral Sciences. Hillsdale, MI: Lawrance Erlbaum (1988).
92. Tzourio-Mazoyer N, Landeau B, Papathanassiou D, Crivello F, Etard O, Delcroix N, et al. Automated anatomical labeling of activations in SPM using a macroscopic anatomical parcellation of the MNI MRI single-subject brain. Neuroimage. (2002) 15:273–89. doi: 10.1006/nimg.2001.0978
93. Miyake A, Friedman NP, Emerson MJ, Witzki AH, Howerter A, Wager TD. The unity and diversity of executive functions and their contributions to complex “frontal lobe” tasks: a latent variable analysis. Cogn Psychol. (2000) 41:49–100. doi: 10.1006/cogp.1999.0734
94. Delbaere K, Close JC, Heim J, Sachdev PS, Brodaty H, Slavin MJ, et al. A multifactorial approach to understanding fall risk in older people. J Am Geriatr Soc. (2010) 58:1679–85. doi: 10.1111/j.1532-5415.2010.03017.x
95. Schoene D, Smith ST, Davies TA, Delbaere K, Lord SR. A Stroop Stepping Test (SST) using low-cost computer game technology discriminates between older fallers and non-fallers. Age Ageing. (2014) 43:285–9. doi: 10.1093/ageing/aft157
96. Blackwood J, Shubert T, Forgarty K, Chase C. Relationships between performance on assessments of executive function and fall risk screening measures in community-dwelling older adults. J Geriatr Phys Ther. (2016) 39:89–96. doi: 10.1519/JPT.0000000000000056
97. Caetano MJD, Menant JC, Schoene D, Pelicioni PHS, Sturnieks DL, Lord SR. Sensorimotor and cognitive predictors of impaired gait adaptability in older people. J Gerontol A Biol Sci Med Sci. (2017) 72:1257–63. doi: 10.1093/gerona/glw171
98. Mateen BA, Bussas M, Doogan C, Waller D, Saverino A, Kiraly FJ, et al. The Trail Making test: a study of its ability to predict falls in the acute neurological in-patient population. Clin Rehabil. (2018) 32:1396–405. doi: 10.1177/0269215518771127
99. Anderson-Hanley C, Arciero PJ, Brickman AM, Nimon JP, Okuma N, Westen SC, et al. Exergaming and older adult cognition a cluster randomized clinical trial. Am J Prev Med. (2012) 42:109–19. doi: 10.1016/j.amepre.2011.10.016
100. Maillot P, Perrot A, Hartley A. Effects of interactive physical-activity video-game training on physical and cognitive function in older adults. Psychol Aging. (2012) 27:589. doi: 10.1037/a0026268
101. Schoene D, Lord SR, Delbaere K, Severino C, Davies TA, Smith ST. A randomized controlled pilot study of home-based step training in older people using videogame technology. PLoS ONE. (2013) 8:e57734. doi: 10.1371/journal.pone.0057734
102. Eggenberger P, Schumacher V, Angst M, Theill N, de Bruin ED. Does multicomponent physical exercise with simultaneous cognitive training boost cognitive performance in older adults? A 6-month randomized controlled trial with a 1-year follow-up. Clin Interv Aging. (2015) 10:1335–49. doi: 10.2147/CIA.S87732
103. Gschwind YJ, Eichberg S, Ejupi A, de Rosario H, Kroll M, Marston HR, et al. ICT-based system to predict and prevent falls (iStoppFalls): results from an international multicenter randomized controlled trial. Eur Rev Aging Phys Act. (2015) 12:10. doi: 10.1186/s11556-015-0155-6
104. Ogawa EF, You T, Leveille SG. Potential benefits of exergaming for cognition and dual-task function in older adults: a systematic review. J Aging Phys Act. (2016) 24:332–6. doi: 10.1123/japa.2014-0267
105. Cassilhas RC, Viana VA, Grassmann V, Santos RT, Santos RF, Tufik S, et al. The impact of resistance exercise on the cognitive function of the elderly. Med Sci Sports Exerc. (2007) 39:1401–7. doi: 10.1249/mss.0b013e318060111f
106. Hillman CH, Erickson KI, Kramer AF. Be smart, exercise your heart: exercise effects on brain and cognition. Nat Rev Neurosci. (2008) 9:58. doi: 10.1038/nrn2298
107. Anderson-Hanley C, Nimon JP, Westen SC. Cognitive health benefits of strengthening exercise for community-dwelling older adults. J Clin Exp Neuropsychol. (2010) 32:996–1001. doi: 10.1080/13803391003662702
108. Smith PJ, Blumenthal JA, Hoffman BM, Cooper H, Strauman TA, Welsh-Bohmer K, et al. Aerobic exercise and neurocognitive performance: a meta-analytic review of randomized controlled trials. Psychosom Med. (2010) 72:239–52. doi: 10.1097/PSY.0b013e3181d14633
109. Voelcker-Rehage C, Godde B, Staudinger UM. Cardiovascular and coordination training differentially improve cognitive performance and neural processing in older adults. Front Hum Neurosci. (2011) 5:26. doi: 10.3389/fnhum.2011.00026
110. Nagamatsu LS, Handy TC, Hsu CL, Voss M, Liu-Ambrose T. Resistance training promotes cognitive and functional brain plasticity in seniors with probable mild cognitive impairment. Arch Intern Med. (2012) 172:666–8. doi: 10.1001/archinternmed.2012.379
111. Rehfeld K, Lüders A, Hökelmann A, Lessmann V, Kaufmann J, Brigadski T, et al. Dance training is superior to repetitive physical exercise in inducing brain plasticity in the elderly. PLoS ONE. (2018) 13:e0196636. doi: 10.1371/journal.pone.0196636
112. Kent P. The evolution of the Wechsler Memory Scale: a selective review. Appl Neuropsychol Adult. (2013) 20:277–91. doi: 10.1080/09084282.2012.689267
113. Howes SC, Charles DK, Marley J, Pedlow K, McDonough SM. Gaming for health: systematic review and meta-analysis of the physical and cognitive effects of active computer gaming in older adults. Phys Ther. (2017) 97:1122–37. doi: 10.1093/ptj/pzx088
114. Pichierri G, Murer K, de Bruin ED. A cognitive-motor intervention using a dance video game to enhance foot placement accuracy and gait under dual task conditions in older adults: a randomized controlled trial. BMC Geriatr. (2012) 12:74. doi: 10.1186/1471-2318-12-74
115. Schättin A, Arner R, Gennaro F, de Bruin ED. Adaptations of prefrontal brain activity, executive functions, and gait in healthy elderly following exergame and balance training: a randomized-controlled study. Front Aging Neurosci. (2016) 8:278. doi: 10.3389/fnagi.2016.00278
116. Segev-Jacubovski O, Herman T, Yogev-Seligmann G, Mirelman A, Giladi N, Hausdorff JM. The interplay between gait, falls and cognition: can cognitive therapy reduce fall risk? Expert Rev Neurother. (2011) 11:1057–75. doi: 10.1586/ern.11.69
117. Beurskens R, Bock O. Age-related deficits of dual-task walking: a review. Neural Plast. (2012) 2012:131608. doi: 10.1155/2012/131608
118. Bohannon RW, Andrews AW, Thomas MW. Walking speed: reference values and correlates for older adults. J Orthopaedic Sports Phys Ther. (1996) 24:86–90. doi: 10.2519/jospt.1996.24.2.86
119. Kattenstroth J-C, Kalisch T, Holt S, Tegenthoff M, Dinse HR. Six months of dance intervention enhances postural, sensorimotor, and cognitive performance in elderly without affecting cardio-respiratory functions. Front Aging Neurosci. (2013) 5:5. doi: 10.3389/fnagi.2013.00005
120. Hamacher D, Hamacher D, Rehfeld K, Hökelmann A, Schega L. The effect of a six-month dancing program on motor-cognitive dual-task performance in older adults. J Aging Phys Act. (2015) 23:647–52. doi: 10.1123/japa.2014-0067
121. Rehfeld K, Müller P, Aye N, Schmicker M, Dordevic M, Kaufmann J, et al. Dancing or fitness sport? The effects of two training programs on hippocampal plasticity and balance abilities in healthy seniors. Front. Hum Neurosci. (2017) 11:305. doi: 10.3389/fnhum.2017.00305
122. Liao Y-Y, Chen I-H, Wang R-Y. Effects of Kinect-based exergaming on frailty status and physical performance in prefrail and frail elderly: a randomized controlled trial. Sci Rep. (2019) 9:9353. doi: 10.1038/s41598-019-45767-y
123. Schoene D, Valenzuela T, Toson B, Delbaere K, Severino C, Garcia J, et al. Interactive cognitive-motor step training improves cognitive risk factors of falling in older adults - a randomized controlled trial. PLoS ONE. (2015) 10:e0145161. doi: 10.1371/journal.pone.0145161
124. Okubo Y, Schoene D, Lord SR. Step training improves reaction time, gait and balance and reduces falls in older people: a systematic review and meta-analysis. Br J Sports Med. (2016) 51:586–93. doi: 10.1136/bjsports-2015-095452
125. Jones CJ, Rikli RE. Measuring functional fitness in older adults. J Active Aging. (2002) 1:24–30.
126. Cress ME, Buchner DM, Prohaska T, Rimmer J, Brown M, Macera C, et al. Best practices for physical activity programs and behavior counseling in older adult populations. J Aging Phys Act. (2005) 13:61–74. doi: 10.1123/japa.13.1.61
127. Paterson DH, Jones GR, Rice CL. Ageing and physical activity: evidence to develop exercise recommendations for older adults. Appl Physiol Nutr Metab. (2007) 32:S69–108. doi: 10.1139/H07-111
128. Van Praag H, Christie BR, Sejnowski TJ, Gage FH. Running enhances neurogenesis, learning, and long-term potentiation in mice. Proc Natl Acad Sci USA. (1999) 96:13427–31. doi: 10.1073/pnas.96.23.13427
129. Colcombe SJ, Erickson KI, Scalf PE, Kim JS, Prakash R, McAuley E, et al. Aerobic exercise training increases brain volume in aging humans. J Gerontol Ser A Biol Sci Med Sci. (2006) 61:1166–70. doi: 10.1093/gerona/61.11.1166
130. Kleim JA, Barbay S, Cooper NR, Hogg TM, Reidel CN, Remple MS, et al. Motor learning-dependent synaptogenesis is localized to functionally reorganized motor cortex. Neurobiol Learn Mem. (2002) 77:63–77. doi: 10.1006/nlme.2000.4004
131. Kleim JA, Markham JA, Vij K, Freese JL, Ballard DH, Greenough WT. Motor learning induces astrocytic hypertrophy in the cerebellar cortex. Behav Brain Res. (2007) 178:244–9. doi: 10.1016/j.bbr.2006.12.022
132. Blumenfeld-Katzir T, Pasternak O, Dagan M, Assaf Y. Diffusion MRI of structural brain plasticity induced by a learning and memory task. PLoS ONE. (2011) 6:e20678. doi: 10.1371/journal.pone.0020678
133. Niemann C, Godde B, Staudinger U, Voelcker-Rehage C. Exercise-induced changes in basal ganglia volume and cognition in older adults. Neuroscience. (2014) 281:147–63. doi: 10.1016/j.neuroscience.2014.09.033
134. Niemann C, Godde B, Voelcker-Rehage C. Not only cardiovascular, but also coordinative exercise increases hippocampal volume in older adults. Front Aging Neurosci. (2014) 6:170. doi: 10.3389/fnagi.2014.00170
135. Müller P, Rehfeld K, Schmicker M, Hökelmann A, Dordevic M, Lessmann V, et al. Evolution of neuroplasticity in response to physical activity in old age: the case for dancing. Front Aging Neurosci. (2017) 9:56. doi: 10.3389/fnagi.2017.00056
136. Mortimer JA, Ding D, Borenstein AR, DeCarli C, Guo Q, Wu Y, et al. Changes in brain volume and cognition in a randomized trial of exercise and social interaction in a community-based sample of non-demented Chinese elders. J Alzheimer's Dis. (2012) 30:757–66. doi: 10.3233/JAD-2012-120079
137. Engvig A, Fjell AM, Westlye LT, Skaane NV, Dale AM, Holland D, et al. Effects of cognitive training on gray matter volumes in memory clinic patients with subjective memory impairment. J Alzheimer's Dis. (2014) 41:779–91. doi: 10.3233/JAD-131889
138. Suo C, Singh MF, Gates N, Wen W, Sachdev P, Brodaty H, et al. Therapeutically relevant structural and functional mechanisms triggered by physical and cognitive exercise. Mol Psychiatry. (2016) 21:1633. doi: 10.1038/mp.2016.19
139. Jessberger S, Gage FH. Stem-cell-associated structural and functional plasticity in the aging hippocampus. Psychol Aging. (2008) 23:684. doi: 10.1037/a0014188
140. Ferris LT, Williams JS, Shen C-L. The effect of acute exercise on serum brain-derived neurotrophic factor levels and cognitive function. Med Sci Sports Exerc. (2007) 39:728–34. doi: 10.1249/mss.0b013e31802f04c7
141. Vaynman S, Ying Z, Gomez-Pinilla F. Hippocampal BDNF mediates the efficacy of exercise on synaptic plasticity and cognition. Eur J Neurosci. (2004) 20:2580–90. doi: 10.1111/j.1460-9568.2004.03720.x
142. Ruscheweyh R, Willemer C, Krüger K, Duning T, Warnecke T, Sommer J, et al. Physical activity and memory functions: an interventional study. Neurobiol Aging. (2011) 32:1304–19. doi: 10.1016/j.neurobiolaging.2009.08.001
143. Lövdén M, Schaefer S, Noack H, Bodammer NC, Kühn S, Heinze HJ, et al. Spatial navigation training protects the hippocampus against age-related changes during early and late adulthood. Neurobiol Aging. (2012) 33:620.e9-22. doi: 10.1016/j.neurobiolaging.2011.02.013
144. Petersen AMW, Pedersen BK. The anti-inflammatory effect of exercise. J Appl Physiol. (2005) 98:1154–62. doi: 10.1152/japplphysiol.00164.2004
145. Pervaiz N, Hoffman-Goetz L. Freewheel training alters mouse hippocampal cytokines. Int J Sports Med. (2011) 32:889–95. doi: 10.1055/s-0031-1279780
146. Good CD, Johnsrude IS, Ashburner J, Henson RN, Friston KJ, Frackowiak RS. A voxel-based morphometric study of ageing in 465 normal adult human brains. Neuroimage. (2001) 14:21–36. doi: 10.1006/nimg.2001.0786
Keywords: physical-cognitive training, exergame, physical functions, cognition, brain volume, healthy aging
Citation: Adcock M, Fankhauser M, Post J, Lutz K, Zizlsperger L, Luft AR, Guimarães V, Schättin A and de Bruin ED (2020) Effects of an In-home Multicomponent Exergame Training on Physical Functions, Cognition, and Brain Volume of Older Adults: A Randomized Controlled Trial. Front. Med. 6:321. doi: 10.3389/fmed.2019.00321
Received: 24 September 2019; Accepted: 16 December 2019;
Published: 28 January 2020.
Edited by:
Jon Irazusta, University of the Basque Country, SpainReviewed by:
Marios Kyriazis, ELPIs Foundation for Indefinite Lifespans, CyprusLiang-Yu Chen, Taipei Veterans General Hospital, Taiwan
Copyright © 2020 Adcock, Fankhauser, Post, Lutz, Zizlsperger, Luft, Guimarães, Schättin and de Bruin. This is an open-access article distributed under the terms of the Creative Commons Attribution License (CC BY). The use, distribution or reproduction in other forums is permitted, provided the original author(s) and the copyright owner(s) are credited and that the original publication in this journal is cited, in accordance with accepted academic practice. No use, distribution or reproduction is permitted which does not comply with these terms.
*Correspondence: Manuela Adcock, bWFudWVsYS5vbWxpbkBoZXN0LmV0aHouY2g=