- 1Canadian Blood Services, Vancouver, BC, Canada
- 2Centre for Blood Research, University of British Columbia, Vancouver, BC, Canada
- 3Research and Development, Australian Red Cross Blood Service, Sydney, NSW, Australia
- 4Sydney Medical School, The University of Sydney, Sydney, NSW, Australia
Transfusions of platelets are an important cornerstone of medicine; however, recipients may be subject to risk of adverse events associated with the potential transmission of pathogens, especially bacteria. Pathogen inactivation (PI) technologies based on ultraviolet illumination have been developed in the last decades to mitigate this risk. This review discusses studies of platelet concentrates treated with the current generation of PI technologies to assess their impact on quality, PI capacity, safety, and clinical efficacy. Improved safety seems to come with the cost of reduced platelet functionality, and hence transfusion efficacy. In order to understand these negative impacts in more detail, several molecular analyses have identified signaling pathways linked to platelet function that are altered by PI. Because some of these biochemical alterations are similar to those seen arising in the context of routine platelet storage lesion development occurring during blood bank storage, we lack a complete picture of the contribution of PI treatment to impaired platelet functionality. A model generated using data from currently available publications places the signaling protein kinase p38 as a central player regulating a variety of mechanisms triggered in platelets by PI systems.
The Challenges of Platelet Transfusions
Platelets play an essential role in hemostasis, fibrinolysis, and vascular integrity, which are critical physiological processes to prevent and control bleeding (1–3). Platelet concentrates (PCs) are transfused to treat bleeding in thrombocytopenic, trauma, or surgery patients (4–6) as well as for prophylactic treatment of patients with hypoproliferative thrombocytopenia (7, 8). Over the last decades, development of improved therapies and the subsequent introduction of new transfusion guidelines have changed the practice of platelet transfusion (9, 10) which has, in turn, influenced the management of platelet inventories in the blood bank.
Additionally, the integrity and safety of platelet preparations could be compromised by the presence of pathogens, such as viruses, bacteria, and parasites (11). Serious complications or death due to bacterially contaminated units have been well documented, leading to several changes in the collection procedures, including stricter donor screening, improved skin disinfection methods and diversion of the first few milliliters of collected blood, and bacterial culture of PCs (12–16). However, the risk still exists, not only for undetected bacterial contamination but for the increasing number of emerging and re-emerging pathogens, particularly viruses for which screening tests may not be in place.
Finally, even with the use of pre-storage leukoreduction, the transfer of residual allogeneic donor leukocytes in PCs still occurs and can potentially cause adverse reactions in certain platelet recipients (17). All pathogen inactivation (PI) systems show inactivation capacity of these residual leukocytes (18, 19).
These challenges of platelet storage have led to the development and increasing implementation of PI technologies which are based on ultraviolet (UV) light-mediated damage of nucleic acids and subsequent inactivation of most pathogens as well as passenger white blood cells.
A Brief Overview of Current PI Systems
Currently, three PI systems to produce pathogen-reduced PCs are commercially available, utilizing UV in the presence or absence of a photosenzitizer. These technologies are extensively reviewed in the literature (20–29); therefore, only key points necessary for the context of this review are provided.
The INTERCEPT system (Cerus Corporation, Concord, CA, USA) uses amotosalen as photosensitzer in combination with UVA light (320–400 nm). Amotosalen penetrates the cellular membrane forming non-covalent links between pyrimidine residues in DNA and RNA. UV illumination induces a photochemical reaction that transforms the preexisting link into an irreversible covalent bond, preventing DNA replication and RNA transcription. Excess amotosalen and its photoproducts need to be removed by an in-line compound absorption device (30, 31).
The MIRASOL system (Terumo BCT, Lakewood, CO, USA) uses vitamin B2 (riboflavin) as the photosensitizer and UVA/UVB light (270–360 nm) (32, 33). In the presence of riboflavin, illumination generates free oxygen radicals causing irreversible damage to guanidine nucleotide bases. Riboflavin does not need to be removed following illumination as it is a common dietary element and generally considered to be safe.
The THERAFLEX-UV Platelets system (MacoPharma, Tourcoing, France) uses UVC light in combination with strong agitation which facilitates light penetration and does not require a photosensitizer. UVC acts directly on nucleic acids to induce pyrimidine dimers to block DNA replication (34, 35).
Pathogen-Reduced Platelet Products
Pathogen-reduced PCs can be obtained by direct treatment of platelet components using a PI system, or they can be derived by treating whole blood with the MIRASOL (36, 37) or potentially the INTERCEPT system once a current trial turns out to be successful followed by processing into the (platelet) components (Table 1).
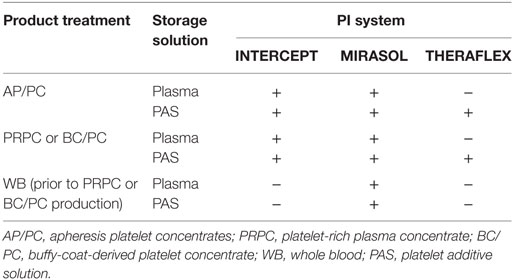
Table 1. Overview of pathogen inactivation (PI) treatment options to obtain pathogen-reduced platelet products.
It is noteworthy to point out that the THERAFLEX system require PCs produced in platelet additive solution (PAS) while the MIRASOL and INTERCEPT systems can treat PCs in plasma or PAS.
Ongoing Debate: Safety vs Efficacy of PI
More than a decade ago, the interest in PI prompted many large-scale discussions (38–40). The outcome of these deliberations included the provision of information required for implementation of PI systems such as implementation criteria, component specifications, licensing requirements, and the impact in blood product inventories, as well as clinical issues such as transfusion efficacy, risk management issues, and cost–benefit assessments. Since then, numerous studies have been conducted to provide answers to questions on product safety, clinical efficacy, and quality.
In order to assess inactivation efficacy, studies spiking pathogens relevant to blood transfusion into PCs prior to illumination have been performed (34, 41–44). All PI systems currently on the market have demonstrated effectiveness in inactivating most tested pathogens with moderate to highly effective inactivation capacities for several emerging viruses including West Nile virus (45), chikungunyah virus (46), Zika virus (47, 48), dengue virus (49), and hepatitis-E-virus (50). Additionally, a comparative study (51) revealed that HIV-1 can be similarly inactivated by MIRASOL and INTERCEPT, however, less efficient compared to other viruses due to its resistance to UV light. Furthermore, INTERCEPT demonstrated a higher inactivation capacity for bovine viral diarrhea virus and pseudorabies virus compared to MIRASOL while both technologies showed similar log reductions for hepatitis-A-virus and porcine parvovirus. However, due to their chemistry, PI systems are only able to target pathogens that contain nucleic acids and consequently they are ineffective against prions and transmission of variant Creutzfeldt–Jakob disease (52).
In order to demonstrate clinical efficacy, several large clinical trials using these PI systems have been conducted or are underway (22, 53) and extensive hemovigilance studies have also been undertaken. The main message is that PI treatment damages the platelets in many ways including alterations in membrane integrity, signaling pathways and in some capacity functionality of miRNAs, which results in reduced recovery and survival in healthy volunteers (54, 55). Similarly, shorter transfusion intervals have been observed in patients receiving PI-treated platelets, but these observations for the most part have not been associated with increased World Health Organization grade 2 or greater bleeding in patients receiving pathogen-reduced platelets, as hemostatic efficacy seems to be maintained (22, 26). Furthermore, some evidence points toward the fact that transfusion of PI-treated platelets does not affect mortality, the risk of clinically significant or severe bleeding, or the risk of a serious adverse event (AE) (56). However, as pointed out by Kaiser-Guignard and colleagues, the results of the published clinical studies should be interpreted with caution, and their characteristics and possible biases should be taken into account (22), such that interpretation of clinical outcome data cannot be generalized across different PI systems (22). A recent systematic review presented strong evidence that transfusion of PI-treated platelets appears to increase the risk of platelet refractoriness and the frequency of platelet transfusions (56).
The majority of contributions to investigations of PIs are in vitro quality studies. Multiple analyses have been conducted to monitor potential changes in the platelet quality following illumination with the three different PI systems in combination with products of different characteristics (see Table 1). These studies have typically measured common blood banking parameters, including metabolic activities, platelet activation, and platelet function to evaluate product quality, and to determine whether quality control requirements of the individual jurisdictions were met. Comparisons of different studies; however, are hampered by the fact that these measures are influenced by the type and proportion of the platelet storage medium. PI treatment of platelets in different PAS differentially alters platelet quality features (57). Recent studies with the riboflavin/UV system (MIRASOL) revealed that the quality of platelets is similar whether stored in plasma or PAS; however, transfusion of treated PCs in PAS led to fewer transfusion reactions (58). This observation is corroborated by the finding that PAS seems to have a protective effect on platelets upon illumination (59).
Based on these diverse studies, in recent years, many (individual) opinions have been published outlining the pros and cons of PI in light of safety and efficacy (20, 60–64). Ongoing discussions are guided by experiences from blood centers that have implemented PI (65–67).
Platelet Storage Lesion (PSL): A General Overview
Many studies measuring changes to platelet in vitro quality indicate that PI treatment accelerates the progression of the PSL. This term describes the sum of all the deleterious changes in platelet structure and function that arise from the time the blood is withdrawn from the donor to the time the platelets are transfused to the recipient (68–73). It is mainly explained by triggering platelet activation during preparation and handling of PCs, especially the heightened metabolic activity and activation-specific changes to surface glycoproteins observed in stored platelets (74). Transient derangement of platelet metabolism can be rescued by plasma replacement, resulting in improved morphology scores, stabilized osmotic recovery, and completely restored platelet secretory responses (75).
The Impact of PI on Platelet Functions
PLT Activation, Degranulation, and Protein Release
As mentioned above, the main feature of PSL seems to be platelet activation, which is commonly determined by the expression of P-selectin (CD62P) on the platelet surface, as a consequence of the release of the alpha-granule content. Many studies have shown that PI increases the surface expression of CD62P (58, 76–78).
Additional features of storage-mediated platelet activation are the increased phosphatidylserine (PS) externalization (79) and changes in the protein profile of platelet surface receptors (80, 81) which are further altered upon PI treatment (82).
Among other changes, the level of cytokines and chemokines also increases in the supernatant of the storage solution during platelet storage (83–85). Although some controversy continues in the literature (86), PI treatment appears to induce platelet degranulation, hence further increasing the levels of immune factors under some treatment conditions (86–91). The altered releasate composition may affect the immunomodulatory capacity of platelets. As a consequence of this accumulation, supernatants of MIRASOL PI-treated platelets can suppress LPS (lipopolysaccharide)-induced monocyte IL-12 production (92), as well as increase LPS-induced mononuclear cell production of IL-8 (93). A recent study has demonstrated that increased supernatant levels of pro-inflammatory molecules resulting from platelet granule release are associated with reactive oxygen species generation during storage (94). This finding is corroborated by an observed increased ROS production in MIRASOL PI-treated PCs (77, 95).
A brief summary is provided in Table 2 highlighting the changes of platelet storage features by the individual PI systems.
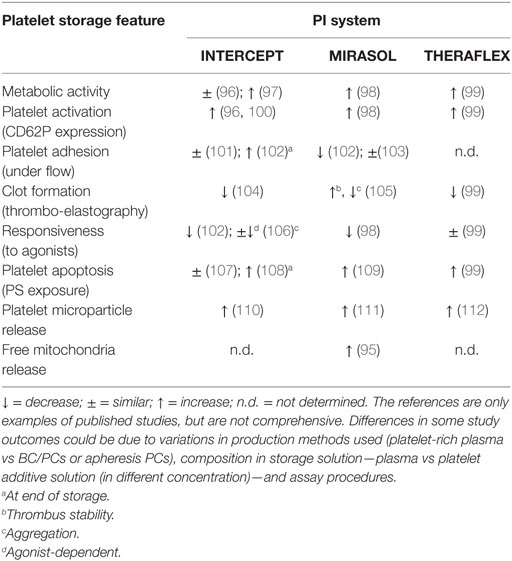
Table 2. Summary of impact of pathogen inactivation (PI) treatment on platelet features compared to untreated control.
Development of Platelet Apoptosis
There is an ongoing debate regarding the extent to which platelet activation and programmed cell death (apoptosis) in platelets overlap at the molecular level (113). Platelets contain most of the apoptotic machinery, including pro- and anti-apoptotic Bcl-protein family members as well as caspases (114). Activation of these pathways leads to microvesiculation with expression of PS in the outer layer of the platelet membrane (115). As PS exposure is believed to contribute to the development of inflammatory or immunomodulatory processes and ultimately regulates clearance of platelet from circulation, PS exposure monitored by annexin-V binding is commonly used to measure the development of platelet apoptosis.
Pathogen inactivation treatment also results in the externalization of PS (59, 116, 117). MIRASOL PI-treated PLTs exhibit an increased expression of proapoptotic proteins Bak and Bax, but not anti-apoptotic proteins Bcl-XL (109, 116). Additionally, MIRASOL PI-triggered activation of caspase cleavage leads to proteolytic cleavage of their respective substrate proteins (116). Similar results have recently been shown in INTERCEPT PI-treated platelets (118). However, these features are not prominent until later in storage (typically 5–7 days) and may only need to be considered in the context of extended platelet storage.
Microvesicle (MR) Release
Platelets are known to generate heterogeneous populations of cell-derived MVs (119). Platelet MVs have a bilayered phospholipid structure exposing procoagulant PS and expressing various membrane receptors, and they serve as cell-to-cell shuttles for bioactive molecules such as lipids, growth factors, microRNAs (miRNAs), and mitochondria (120). Further, the presence and quantity of MVs has been associated with the clinical severity of the atherosclerotic disease, diabetes, and cancer (6, 121). These features along with the observation that the number, function, and content of MVs in the components varies with age, gender, lipid, and hormone profiles of the blood donor (122) makes them one of the most discussed, controversial, and interesting topics in current blood banking and transfusion medicine (123). Different studies have demonstrated that all UV-based PI treatments increase the release of MVs from platelets compared to untreated controls (36, 95, 112, 124). To our knowledge, no study has directly addressed the impact of INTERCEPT on the release of MVs during PC storage; however, Kanzler et al. found a reduction of MVs in the platelet product immediate after INTERCEPT treatment (125).
Role of Platelets in Inflammation
Although once primarily recognized for their role in hemostasis and thrombosis, platelets have been increasingly recognized as a multipurpose cell. There is growing recognition of the critical role of platelets in inflammation and immune responses. Platelets release numerous inflammatory mediators such as RANTES or CD40L, modifying leukocyte and endothelial responses to a range of different inflammatory stimuli (88). Additionally, platelets form aggregates with leukocytes and form bridges between leukocytes and endothelium, largely mediated by platelet P-selectin. Through their interactions with monocytes, neutrophils, lymphocytes, and the endothelium, platelets are, therefore, important coordinators of inflammation and both innate and adaptive immune responses. As mentioned above, studies have shown that MIRASOL-treated platelets release such mediators (92, 93) and, therefore, might modulate inflammatory responses.
Mitochondria and Mitochondrial DNA (mtDNA) Release
Mitochondria are known as the powerhouse of cells and play a crucial role in maintaining platelet function throughout platelet storage (126). Mitochondria are released from activated platelets and upon hydrolysis of the mitochondrial membrane release mtDNA (127). MIRASOL-PI treatment also causes release of free mitochondria, mainly at the later stages of storage (95). Potentially associated with the mitochondria release, free mtDNA has been associated with AEs following platelet transfusion, and may be predictive of some types of AEs (128). mtDNA is a highly potent inflammatory trigger (128) that can be released from platelets during storage (129). Illumination of platelets with PI systems modifies mtDNA (129–131). Detection of PI-modified mtDNA using PCR assays can be used to monitor and confirm PI treatment (131). Furthermore, the relationship of mtDNA levels and AEs related to immunomodulation should also be considered; with a recent study showing an association between mtDNA and the incidence of respiratory distress posttransfusion (132).
MicroRNA
MicroRNAs are small (~20–24 nucleotides) RNA sequences generated by ribonucleases in the nucleus (by Drosha) and cytosol (by Dicer 1) through sequential enzymatic trimming of double stranded miRNA precursors. miRNAs are thought to fine tune gene expression through degradation of their mRNA targets (133). Although platelets are anucleate, high-throughput sequencing has revealed that human platelets harbor a complex array of miRNAs, which are key regulators of mRNA translation in different cell types (134). Activated platelets can deliver mRNA regulatory Argonaute-2 miRNA complexes to endothelial cells via MVs leading to modulation of cell function (135).
INTERCEPT, but not MIRASOL PI treatment has been shown to affect the platelet mRNA transcriptome (27, 136). However, miRNA synthesis and function were not affected and no cross-linking of miRNA-sized endogenous platelet RNA species was observed; rather miRNA levels were reduced (136, 137). Further, the reduction in the platelet miRNA levels induced by INTERCEPT correlated with platelet activation and an impaired platelet aggregation response to ADP (136). In contrast, a recent study presented by Arnason et al. (138) demonstrated that INTERCEPT treatment did not change the quality or significantly altered the miRNA profile of PCs. These controversial results prompted further investigations and as the clinical significance of MV-associated miRNAs is unknown, and speculation of a negative effect of PI-treated platelets including long-term consequences for recipients is as yet unwarranted. This is a relatively new area of research, and additional studies are required to fully understand the impact of PI treatment on miRNA synthesis and the resulting impact on platelet quality.
mRNA Levels and Protein Synthesis
Although anucleate, platelets have the capacity to synthesize biologically relevant proteins that are regulated via gene expression programs at the translational level in response to physiological stimuli (139–141). Recent studies have demonstrated that levels of specific mRNA species are reduced following MIRASOL PI treatment while others are less affected (142). Subsequent studies have revealed that this observation is mirrored in the platelet translatome, demonstrating that platelets are still capable of synthesizing proteins following PI treatment, suggesting that they may possess mechanism(s) to protect their mRNA from damage by the PI treatment (143). The clinical relevance of this finding, however, is still unknown.
Impact of PI Treatment on Platelet Lipidomics
Although the application of lipidomics to platelet biology is still in its infancy, seminal studies have shaped our knowledge of how lipids regulate key aspects of platelet biology, including aggregation, shape change, coagulation, and degranulation, as well as how lipids generated by platelets influence other cells, such as leukocytes and the vascular wall, and thus how they regulate hemostasis, vascular integrity, and inflammation, as well as contribute to pathologies, including arterial/deep vein thrombosis, and atherosclerosis (144). Mapping the human platelet lipidome revealed cytosolic phospholipase A2 as a regulator of mitochondrial bioenergetics during activation (145). A recent study has demonstrated that psoralen and UV light increased the order of lipid phases by covalent modification of phospholipids, thereby inhibiting membrane recruitment of effector kinases such as BTK and Akt and consequently affecting GPVI- and PAR1-mediated signal transduction (99).
Further Investigations toward Understanding the Molecular Mechanisms of PI-Induced Platelet Alteration: From Proteomics to Signaling
A variety of untargeted proteomic approaches have been used to assess the impact of PI systems on platelets (146–148). The effect of the PI treatment on the proteome appears to be different according to the particular technology. A comparative analysis of proteomic data revealed that MIRASOL seems to impact proteins involved mainly in platelet adhesion and shape change while INTERCEPT affects proteins of intracellular platelet activation pathways and THERAFLEX influences proteins linked to platelet shape change and aggregation (149). These conclusions are based on a relatively small number of studies and further analyses are required for verification.
A more targeted approach using a phospho-kinase antibody-based array demonstrated that a variety of kinases were activated by MIRASOL PI treatment (150). p38MAPK plays a central role in MIRASOL PI-mediated signaling by regulating a variety of platelet features, such as apoptosis (109), mitochondrial function, and release of free and MV-encapsulated mitochondria (95). The INTERCEPT system also triggers p38MAPK activation in platelets, and the phosphorylation of the p38MAPK substrate Tace is directly linked to GPIb cleavage possibly explaining the reduced adhesion of those platelets under flow conditions (118). The role of p38MAPK in mediating PI-triggered signaling linked to features of PSL is supported by studies demonstrating a regulatory role of p38MAPK in regulating PSL development (151) and platelet in vivo recovery and survival in mouse models (152). This body of work suggests that similar signaling pathways are activated by both of these PI systems as modeled in Figure 1. Although only a few studies to date have investigated the signaling aspect in platelets, it could be hypothesized that p38MAPK activation in response to the stress associated with the PI treatment may have a regulatory role in platelet life span (153) as inhibition of this protein leads to decreased apoptosis (109, 118).
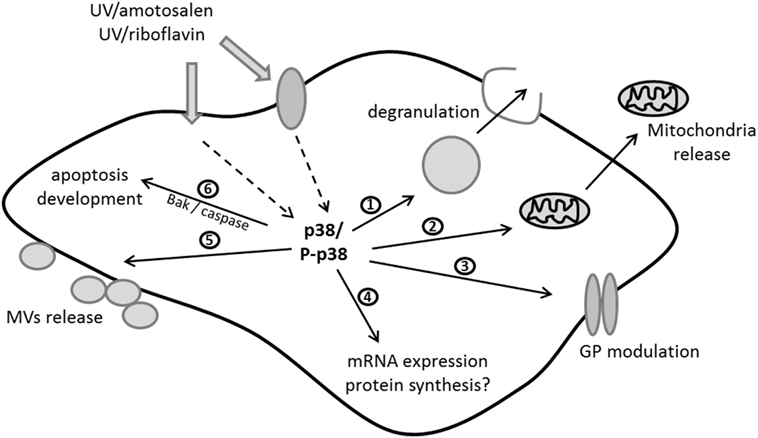
Figure 1. Current molecular model of signaling triggered by ultraviolet (UV)/riboflavin (MIRASOL) and UV/amotosalen (INTERCEPT) in platelets: UV can penetrate either directly or via surface/receptor proteins to activate p38MAPK kinase as one of the central players in the signaling cascade. Thus far it has been shown that p38 activation/phosphorylation (P-p38) is involved in regulating (1) degranulation, (2) release of free mitochondria, (3) the modulation of glycoproteins (GPs), (4) the expression levels of mRNAs and potentially protein synthesis, (5) microvesicle (MVs) release, and (6) the development of apoptosis via proapoptotic protein expression and caspase activation. This figure was modified from Ref. (150).
Conclusion and Future Directions
Although there are numerous studies in the literature assessing the impact of UV-based PI systems on platelet in vitro and in vivo function, only a few conclusions can be drawn. All technologies seem to accelerate the development of some form of the PSL but this likely results through different modes of action; therefore, it is likely that many divergent, as well as overlapping molecular mechanisms are triggered. Most of the functional studies conducted to decipher the role of signaling pathways in PI-treated platelets have been carried out using the INTERCEPT and MIRASOL system and thus the effects of the THERAFLEX system remain relatively unknown. However, it is clear that PI-treated platelets are different to untreated platelets, and the differences may go some way toward explaining some of the clinical observations following transfusion of PI-treated platelets. Proteomic analyses and in future other -omics approaches such as metabolomics (154) will likely shed more light into the specific effects of PI treatment. Additional targeted approaches will guide the formulation of signaling models, which may ultimately identify pathways known to impact platelet function upon illumination, and provide potential (protein) markers to assist with the fine-tuning of these technologies. We need to keep in mind, however, that the PI treatment does not only affect platelets per se, these procedures trigger the release of MVs, proteins, and nucleic acids in to the storage medium which also gets transfused. Whether any of these components will have deleterious effects on the recipients remains to be determined even though the initial clinical studies do not show significant clinical effects from PI treatment of PCs.
Author Contributions
All authors contributed to this manuscript and approved the final version for submission.
Conflict of Interest Statement
The authors declare that the research was conducted in the absence of any commercial or financial relationships that could be construed as a potential conflict of interest.
Acknowledgments
The authors received research funding from Cerus, Terumo, and MacoPharma. This study was supported in part by a grant from Health Canada and Canadian Blood Services. The views expressed herein do not necessarily represent the view of the federal government. The Australian governments fund the Australian Red Cross Blood Service to provide blood, blood products, and services to the Australian community.
References
1. Packham MA. Role of platelets in thrombosis and hemostasis. Can J Physiol Pharmacol (1994) 72:278–84. doi:10.1139/y94-043
2. Ni H, Freedman J. Platelets in hemostasis and thrombosis: role of integrins and their ligands. Transfus Apher Sci (2003) 28:257–64. doi:10.1016/S1473-0502(03)00044-2
3. Wang Y, Andrews M, Yang Y, Lang S, Jin JW, Cameron-Vendrig A, et al. Platelets in thrombosis and hemostasis: old topic with new mechanisms. Cardiovasc Hematol Disord Drug Targets (2012) 12:126–32. doi:10.2174/1871529X11202020126
4. McCullough J. Overview of platelet transfusion. Semin Hematol (2010) 47:235–42. doi:10.1053/j.seminhematol.2010.04.001
5. McQuilten ZK, Crighton G, Engelbrecht S, Gotmaker R, Brunskill SJ, Murphy MF, et al. Transfusion interventions in critical bleeding requiring massive transfusion: a systematic review. Transfus Med Rev (2015) 29:127–37. doi:10.1016/j.tmrv.2015.01.001
6. Burnouf T, Elemary M, Radosevic J, Seghatchian J, Goubran H. Platelet transfusion in thrombocytopenic cancer patients: sometimes justified but likely insidious. Transfus Apher Sci (2017) 56:305–9. doi:10.1016/j.transci.2017.05.016
7. Slichter SJ. New thoughts on the correct dosing of prophylactic platelet transfusions to prevent bleeding. Curr Opin Hematol (2011) 18:427–35. doi:10.1097/MOH.0b013e32834babf4
8. Estcourt L, Stanworth S, Doree C, Hopewell S, Murphy MF, Tinmouth A, et al. Prophylactic platelet transfusion for prevention of bleeding in patients with haematological disorders after chemotherapy and stem cell transplantation. Cochrane Database Syst Rev (2012) 5:CD004269. doi:10.1002/14651858.CD004269.pub3
9. Annen K, Olson JE. Optimizing platelet transfusions. Curr Opin Hematol (2015) 22:559–64. doi:10.1097/MOH.0000000000000188
10. Kumar A, Mhaskar R, Grossman BJ, Kaufman RM, Tobian AA, Kleinman S, et al. Platelet transfusion: a systematic review of the clinical evidence. Transfusion (2015) 55:1116–27; quiz 1115. doi:10.1111/trf.12943
11. Morel P, Deschaseaux M, Bertrand X, Naegelen C, Thouverez M, Talon D. [Detection of bacterial contamination in platelet concentrates: perspectives]. Transfus Clin Biol (2002) 9:250–7. doi:10.1016/S1246-7820(02)00252-5
12. Dodd RY. Bacterial contamination and transfusion safety: experience in the United States. Transfus Clin Biol (2003) 10:6–9. doi:10.1016/S1246-7820(02)00277-X
13. Hillyer CD, Josephson CD, Blajchman MA, Vostal JG, Epstein JS, Goodman JL. Bacterial contamination of blood components: risks, strategies, and regulation: joint ASH and AABB educational session in transfusion medicine. Hematology Am Soc Hematol Educ Program (2003):575–89. doi:10.1182/asheducation-2003.1.575
14. Blajchman MA, Beckers EA, Dickmeiss E, Lin L, Moore G, Muylle L. Bacterial detection of platelets: current problems and possible resolutions. Transfus Med Rev (2005) 19:259–72. doi:10.1016/j.tmrv.2005.05.002
15. Alter HJ, Klein HG. The hazards of blood transfusion in historical perspective. Blood (2008) 112:2617–26. doi:10.1182/blood-2008-07-077370
16. Mathai J. Problem of bacterial contamination in platelet concentrates. Transfus Apher Sci (2009) 41:139–44. doi:10.1016/j.transci.2009.07.012
17. Garraud O, Tariket S, Sut C, Haddad A, Aloui C, Chakroun T, et al. Transfusion as an inflammation hit: knowns and unknowns. Front Immunol (2016) 7:534. doi:10.3389/fimmu.2016.00534
18. Fast LD, DiLeone G, Marschner S. Inactivation of human white blood cells in platelet products after pathogen reduction technology treatment in comparison to gamma irradiation. Transfusion (2011) 51:1397–404. doi:10.1111/j.1537-2995.2010.02984.x
19. Pohler P, Muller M, Winkler C, Schaudien D, Sewald K, Müller TH, et al. Pathogen reduction by ultraviolet C light effectively inactivates human white blood cells in platelet products. Transfusion (2015) 55:337–47. doi:10.1111/trf.12836
20. Lozano M, Cid J. Pathogen inactivation: coming of age. Curr Opin Hematol (2013) 20:540–5. doi:10.1097/MOH.0b013e328365a18f
21. Prowse CV. Component pathogen inactivation: a critical review. Vox Sang (2013) 104:183–99. doi:10.1111/j.1423-0410.2012.01662.x
22. Kaiser-Guignard J, Canellini G, Lion N, Abonnenc M, Osselaer JC, Tissot JD. The clinical and biological impact of new pathogen inactivation technologies on platelet concentrates. Blood Rev (2014) 28:235–41. doi:10.1016/j.blre.2014.07.005
23. Schlenke P. Pathogen inactivation technologies for cellular blood components: an update. Transfus Med Hemother (2014) 41:309–25. doi:10.1159/000365646
24. Kleinman S. Pathogen inactivation: emerging indications. Curr Opin Hematol (2015) 22:547–53. doi:10.1097/MOH.0000000000000186
25. Salunkhe V, van der Meer PF, de Korte D, Seghatchian J, Gutiérrez L. Development of blood transfusion product pathogen reduction treatments: a review of methods, current applications and demands. Transfus Apher Sci (2015) 52:19–34. doi:10.1016/j.transci.2014.12.016
26. Devine DV, Schubert P. Pathogen inactivation technologies: the advent of pathogen-reduced blood components to reduce blood safety risk. Hematol Oncol Clin North Am (2016) 30:609–17. doi:10.1016/j.hoc.2016.01.005
27. Osman A, Hitzler WE, Provost P. Peculiarities of studying the effects of pathogen reduction technologies on platelets. Proteomics Clin Appl (2016) 10:805–15. doi:10.1002/prca.201500124
28. Ramsey G. Hemostatic efficacy of pathogen-inactivated blood components. Semin Thromb Hemost (2016) 42:172–82. doi:10.1055/s-0035-1564845
29. Osman A, Hitzler WE, Provost P. The platelets’ perspective to pathogen reduction technologies. Platelets (2017) 29(2):140–7. doi:10.1080/09537104.2017.1293806
30. Irsch J, Lin L. Pathogen inactivation of platelet and plasma blood components for transfusion using the INTERCEPT blood system. Transfus Med Hemother (2011) 38:19–31. doi:10.1159/000323937
31. Irsch J, Seghatchian J. Update on pathogen inactivation treatment of plasma, with the INTERCEPT blood system: current position on methodological, clinical and regulatory aspects. Transfus Apher Sci (2015) 52:240–4. doi:10.1016/j.transci.2015.02.013
32. Goodrich RP, Doane S, Reddy HL. Design and development of a method for the reduction of infectious pathogen load and inactivation of white blood cells in whole blood products. Biologicals (2010) 38:20–30. doi:10.1016/j.biologicals.2009.10.016
33. Marschner S, Goodrich R. Pathogen reduction technology treatment of platelets, plasma and whole blood using riboflavin and UV light. Transfus Med Hemother (2011) 38:8–18. doi:10.1159/000324160
34. Mohr H, Steil L, Gravemann U, Thiele T, Hammer E, Greinacher A, et al. A novel approach to pathogen reduction in platelet concentrates using short-wave ultraviolet light. Transfusion (2009) 49:2612–24. doi:10.1111/j.1537-2995.2009.02334.x
35. Seghatchian J, Tolksdorf F. Characteristics of the THERAFLEX UV-platelets pathogen inactivation system – an update. Transfus Apher Sci (2012) 46:221–9. doi:10.1016/j.transci.2012.01.008
36. Solheim BG. Pathogen reduction of blood components. Transfus Apher Sci (2008) 39:75–82. doi:10.1016/j.transci.2008.05.003
37. Allain JP, Goodrich R. Pathogen reduction of whole blood: utility and feasibility. Transfus Med (2017) 27(Suppl 5):320–6. doi:10.1111/tme.12456
38. Klein HG, Anderson D, Bernardi MJ, Cable R, Carey W, Hoch JS. Pathogen inactivation: making decisions about new technologies. Report of a consensus conference. Transfusion (2007) 47:2338–47. doi:10.1111/j.1537-2995.2007.01512.x
39. Webert KE, Cserti CM, Hannon J, Lin Y, Pavenski K, Pendergrast JM, et al. Proceedings of a consensus conference: pathogen inactivation-making decisions about new technologies. Transfus Med Rev (2008) 22:1–34. doi:10.1016/j.tmrv.2007.09.001
40. Klein HG, Glynn SA, Ness PM, Blajchman MA; NHLBI Working Group on Research Opportunities for the Pathogen Reduction/Inactivation of Blood Components. Research opportunities for pathogen reduction/inactivation of blood components: summary of an NHLBI workshop. Transfusion (2009) 49:1262–8. doi:10.1111/j.1537-2995.2009.02210.x
41. Keil SD, Bengrine A, Bowen R, Marschner S, Hovenga N, Rouse L, et al. Inactivation of viruses in platelet and plasma products using a riboflavin-and-UV-based photochemical treatment. Transfusion (2015) 55:1736–44. doi:10.1111/trf.13030
42. Keil SD, Hovenga N, Gilmour D, Marschner S, Goodrich R. Treatment of platelet products with riboflavin and UV light: effectiveness against high titer bacterial contamination. J Vis Exp (2015) 102:e52820. doi:10.3791/52820
43. Gowland P, Fontana S, Stolz M, Andina N, Niederhauser C. Parvovirus B19 passive transmission by transfusion of intercept(R) blood system-treated platelet concentrate. Transfus Med Hemother (2016) 43:198–202. doi:10.1159/000445195
44. Taha M, Culibrk B, Kalab M, Schubert P, Yi QL, Goodrich R, et al. Efficiency of riboflavin and ultraviolet light treatment against high levels of biofilm-derived Staphylococcus epidermidis in buffy coat platelet concentrates. Vox Sang (2017) 112:408–16. doi:10.1111/vox.12519
45. Ruane PH, Edrich R, Gampp D, Keil SD, Leonard RL, Goodrich RP. Photochemical inactivation of selected viruses and bacteria in platelet concentrates using riboflavin and light. Transfusion (2004) 44:877–85. doi:10.1111/j.1537-2995.2004.03355.x
46. Vanlandingham DL, Keil SD, Horne KM, Pyles R, Goodrich RP, Higgs S. Photochemical inactivation of chikungunya virus in plasma and platelets using the Mirasol pathogen reduction technology system. Transfusion (2013) 53:284–90. doi:10.1111/j.1537-2995.2012.03717.x
47. Fryk JJ, Marks DC, Hobson-Peters J, Watterson D, Hall RA, Young PR, et al. Reduction of Zika virus infectivity in platelet concentrates after treatment with ultraviolet C light and in plasma after treatment with methylene blue and visible light. Transfusion (2017) 57:2677–82. doi:10.1111/trf.14256
48. Santa Maria F, Laughhunn A, Lanteri MC, Aubry M, Musso D, Stassinopoulos A. Inactivation of zika virus in platelet components using amotosalen and ultraviolet A illumination. Transfusion (2017) 57:2016–25. doi:10.1111/trf.14161
49. Faddy HM, Fryk JJ, Watterson D, Young PR, Modhiran N, Muller DA, et al. Riboflavin and ultraviolet light: impact on dengue virus infectivity. Vox Sang (2016) 111:235–41. doi:10.1111/vox.12414
50. Owada T, Kaneko M, Matsumoto C, Sobata R, Igarashi M, Suzuki K, et al. Establishment of culture systems for genotypes 3 and 4 hepatitis E virus (HEV) obtained from human blood and application of HEV inactivation using a pathogen reduction technology system. Transfusion (2014) 54:2820–7. doi:10.1111/trf.12686
51. Kwon SY, Kim IS, Bae JE, Kang JW, Cho YJ, Cho NS, et al. Pathogen inactivation efficacy of Mirasol PRT system and intercept blood system for non-leucoreduced platelet-rich plasma-derived platelets suspended in plasma. Vox Sang (2014) 107:254–60. doi:10.1111/vox.12158
52. Lescoutra-Etchegaray N, Sumian C, Culeux A, Durand V, Gurgel PV, Deslys JP, et al. Removal of exogenous prion infectivity in leukoreduced red blood cells unit by a specific filter designed for human transfusion. Transfusion (2014) 54:1037–45. doi:10.1111/trf.12420
53. McClaskey J, Xu M, Snyder EL, Tormey CA. Clinical trials for pathogen reduction in transfusion medicine: a review. Transfus Apher Sci (2009) 41:217–25. doi:10.1016/j.transci.2009.09.008
54. Snyder E, Raife T, Lin L, Cimino G, Metzel P, Rheinschmidt M, et al. Recovery and life span of 111indium-radiolabeled platelets treated with pathogen inactivation with amotosalen HCl (S-59) and ultraviolet A light. Transfusion (2004) 44:1732–40. doi:10.1111/j.0041-1132.2004.04145.x
55. AuBuchon JP, Herschel L, Roger J, Taylor H, Whitley P, Li J, et al. Efficacy of apheresis platelets treated with riboflavin and ultraviolet light for pathogen reduction. Transfusion (2005) 45:1335–41. doi:10.1111/j.1537-2995.2005.00202.x
56. Estcourt LJ, Malouf R, Hopewell S, Trivella M, Doree C, Stanworth SJ, et al. Pathogen-reduced platelets for the prevention of bleeding. Cochrane Database Syst Rev (2017) 7:CD009072. doi:10.1002/14651858.CD009072.pub3
57. Leitner GC, List J, Horvath M, Eichelberger B, Panzer S, Jilma-Stohlawetz P. Additive solutions differentially affect metabolic and functional parameters of platelet concentrates. Vox Sang (2016) 110:20–6. doi:10.1111/vox.12317
58. Ignatova AA, Karpova OV, Trakhtman PE, Rumiantsev SA, Panteleev MA. Functional characteristics and clinical effectiveness of platelet concentrates treated with riboflavin and ultraviolet light in plasma and in platelet additive solution. Vox Sang (2016) 110:244–52. doi:10.1111/vox.12364
59. van der Meer PF, Bontekoe IJ, Daal BB, de Korte D. Riboflavin and UV light treatment of platelets: a protective effect of platelet additive solution? Transfusion (2015) 55:1900–8. doi:10.1111/trf.13033
60. Hervig T, Seghatchian J, Apelseth TO. Current debate on pathogen inactivation of platelet concentrates – to use or not to use? Transfus Apher Sci (2010) 43:411–4. doi:10.1016/j.transci.2010.10.012
61. Lozano M, Cid J. Analysis of reasons for not implementing pathogen inactivation for platelet concentrates. Transfus Clin Biol (2013) 20:158–64. doi:10.1016/j.tracli.2013.02.017
62. Hess JR, Pagano MB, Barbeau JD, Johannson PI. Will pathogen reduction of blood components harm more people than it helps in developed countries? Transfusion (2016) 56:1236–41. doi:10.1111/trf.13512
63. Lozano M, Cid J. Platelet concentrates: balancing between efficacy and safety? Presse Med (2016) 45:e289–98. doi:10.1016/j.lpm.2016.06.020
64. Magron A, Laugier J, Provost P, Boilard E. Pathogen reduction technologies: the pros and cons for platelet transfusion. Platelets (2017) 29(1):2–8. doi:10.1080/09537104.2017.1306046
65. Murphy WG. Pathogen reduction: state of reflection in Ireland. Transfus Clin Biol (2011) 18:488–90. doi:10.1016/j.tracli.2011.05.004
66. Jimenez-Marco T, Mercant C, Lliteras E, Cózar M, Girona-Llobera E. Practical issues that should be considered when planning the implementation of pathogen reduction technology for plateletpheresis. Transfus Apher Sci (2015) 52:84–93. doi:10.1016/j.transci.2014.12.004
67. Devine DV. Implementation of pathogen inactivation technology: how to make the best decisions? Transfusion (2017) 57:1109–11. doi:10.1111/trf.14117
68. Seghatchian J, Krailadsiri P. The platelet storage lesion. Transfus Med Rev (1997) 11:130–44. doi:10.1053/tm.1997.0110130
69. Thon JN, Schubert P, Devine DV. Platelet storage lesion: a new understanding from a proteomic perspective. Transfus Med Rev (2008) 22:268–79. doi:10.1016/j.tmrv.2008.05.004
70. Shrivastava M. The platelet storage lesion. Transfus Apher Sci (2009) 41:105–13. doi:10.1016/j.transci.2009.07.002
71. Devine DV, Serrano K. The platelet storage lesion. Clin Lab Med (2010) 30:475–87. doi:10.1016/j.cll.2010.02.002
72. Bennett JS. Shedding new light on the platelet storage lesion. Arterioscler Thromb Vasc Biol (2016) 36:1715–6. doi:10.1161/ATVBAHA.116.308095
73. Tissot JD, Bardyn M, Sonego G, Abonnenc M, Prudent M. The storage lesions: from past to future. Transfus Clin Biol (2017) 24:277–84. doi:10.1016/j.tracli.2017.05.012
74. Bode AP. Platelet activation may explain the storage lesion in platelet concentrates. Blood Cells (1990) 16:109–25; discussion 125–6.
75. Rinder HM, Snyder EL, Tracey JB, Dincecco D, Wang C, Baril L, et al. Reversibility of severe metabolic stress in stored platelets after in vitro plasma rescue or in vivo transfusion: restoration of secretory function and maintenance of platelet survival. Transfusion (2003) 43:1230–7. doi:10.1046/j.1537-2995.2003.00484.x
76. Johnson L, Winter KM, Reid S, Hartkopf-Theis T, Marschner S, Goodrich RP, et al. The effect of pathogen reduction technology (Mirasol) on platelet quality when treated in additive solution with low plasma carryover. Vox Sang (2011) 101:208–14. doi:10.1111/j.1423-0410.2011.01477.x
77. Johnson L, Marks D. Treatment of platelet concentrates with the Mirasol pathogen inactivation system modulates platelet oxidative stress and NF-kappaB activation. Transfus Med Hemother (2015) 42:167–73. doi:10.1159/000403245
78. Tynngård N, Trinks M, Berlin G. In vitro function of platelets treated with ultraviolet C light for pathogen inactivation: a comparative study with nonirradiated and gamma-irradiated platelets. Transfusion (2015) 55:1169–77. doi:10.1111/trf.12963
79. Shapira S, Friedman Z, Shapiro H, Presseizen K, Radnay J, Ellis MH. The effect of storage on the expression of platelet membrane phosphatidylserine and the subsequent impacton the coagulant function of stored platelets. Transfusion (2000) 40:1257–63. doi:10.1046/j.1537-2995.2000.40101257.x
80. Thon JN, Devine DV. Translation of glycoprotein IIIa in stored blood platelets. Transfusion (2007) 47:2260–70. doi:10.1111/j.1537-2995.2007.01455.x
81. Rijkers M, van der Meer PF, Bontekoe IJ, Daal BB, de Korte D, Leebeek FW, et al. Evaluation of the role of the GPIb-IX-V receptor complex in development of the platelet storage lesion. Vox Sang (2016) 111:247–56. doi:10.1111/vox.12416
82. Terada C, Mori J, Okazaki H, Satake M, Tadokoro K. Effects of riboflavin and ultraviolet light treatment on platelet thrombus formation on collagen via integrin alphaIIbbeta3 activation. Transfusion (2014) 54:1808–16. doi:10.1111/trf.12566
83. Rinder HM, Snyder EL. Activation of platelet concentrate during preparation and storage. Blood Cells (1992) 18:445–56; discussion 457–60.
84. Rinder HM, Snyder EL, Bonan JL, Napychank PA, Malkus H, Smith BR. Activation in stored platelet concentrates: correlation between membrane expression of P-selectin, glycoprotein IIb/IIIa, and beta-thromboglobulin release. Transfusion (1993) 33:25–9. doi:10.1046/j.1537-2995.1993.33193142305.x
85. Aye MT, Palmer DS, Giulivi A, Hashemi S. Effect of filtration of platelet concentrates on the accumulation of cytokines and platelet release factors during storage. Transfusion (1995) 35:117–24. doi:10.1046/j.1537-2995.1995.35295125733.x
86. Apelseth T, Hervig T. Comments on “Release of immune modulation factors from platelet concentrates during storage after photochemical pathogen inactivation treatment”. Transfusion (2009) 49:603–4; author reply 604–5. doi:10.1111/j.1537-2995.2008.02047.x
87. Apelseth TO, Hervig TA, Wentzel-Larsen T, Bruserud O. Cytokine accumulation in photochemically treated and gamma-irradiated platelet concentrates during storage. Transfusion (2006) 46:800–10. doi:10.1111/j.1537-2995.2006.00800.x
88. Cognasse F, Osselaer JC, Payrat JM, Chavarin P, Corash L, Garraud O. Release of immune modulation factors from platelet concentrates during storage after photochemical pathogen inactivation treatment. Transfusion (2008) 48:809–13. doi:10.1111/j.1537-2995.2008.01655.x
89. Picker SM, Steisel A, Gathof BS. Evaluation of white blood cell- and platelet-derived cytokine accumulation in MIRASOL-PRT-treated platelets. Transfus Med Hemother (2009) 36:114–20. doi:10.1159/000203359
90. Tauszig ME, Picker SM, Gathof BS. Platelet derived cytokine accumulation in platelet concentrates treated for pathogen reduction. Transfus Apher Sci (2012) 46:33–7. doi:10.1016/j.transci.2011.10.025
91. Sandgren P, Berlin G, Tynngård N. Treatment of platelet concentrates with ultraviolet C light for pathogen reduction increases cytokine accumulation. Transfusion (2016) 56:1377–83. doi:10.1111/trf.13601
92. Loh YS, Dean MM, Johnson L, Marks DC. Treatment of platelets with riboflavin and ultraviolet light mediates complement activation and suppresses monocyte interleukin-12 production in whole blood. Vox Sang (2015) 109:327–35. doi:10.1111/vox.12283
93. Loh YS, Johnson L, Kwok M, Marks DC. Pathogen reduction treatment alters the immunomodulatory capacity of buffy coat-derived platelet concentrates. Transfusion (2014) 54:577–84. doi:10.1111/trf.12320
94. Ghasemzadeh M, Hosseini E. Platelet granule release is associated with reactive oxygen species generation during platelet storage: a direct link between platelet pro-inflammatory and oxidation states. Thromb Res (2017) 156:101–4. doi:10.1016/j.thromres.2017.06.016
95. Chen Z, Schubert P, Bakkour S, Culibrk B, Busch MP, Devine DV. p38 mitogen-activated protein kinase regulates mitochondrial function and microvesicle release in riboflavin- and ultraviolet light-treated apheresis platelet concentrates. Transfusion (2017) 57:1199–207. doi:10.1111/trf.14035
96. van Rhenen DJ, Vermeij J, Mayaudon V, Hind C, Lin L, Corash L. Functional characteristics of S-59 photochemically treated platelet concentrates derived from buffy coats. Vox Sang (2000) 79:206–14. doi:10.1046/j.1423-0410.2000.7940206.x
97. Picker SM, Speer R, Gathof BS. Functional characteristics of buffy-coat PLTs photochemically treated with amotosalen-HCl for pathogen inactivation. Transfusion (2004) 44:320–9. doi:10.1111/j.1537-2995.2003.00590.x
98. Schubert P, Culibrk B, Coupland D, Scammell K, Gyongyossy-Issa M, Devine DV. Riboflavin and ultraviolet light treatment potentiates vasodilator-stimulated phosphoprotein Ser-239 phosphorylation in platelet concentrates during storage. Transfusion (2012) 52:397–408. doi:10.1111/j.1537-2995.2011.03287.x
99. Van Aelst B, Devloo R, Vandekerckhove P, Compernolle V, Feys HB. Ultraviolet C light pathogen inactivation treatment of platelet concentrates preserves integrin activation but affects thrombus formation kinetics on collagen in vitro. Transfusion (2015) 55:2404–14. doi:10.1111/trf.13137
100. Apelseth TØ, Bruserud Ø, Wentzel-Larsen T, Bakken AM, Bjørsvik S, Hervig T. In vitro evaluation of metabolic changes and residual platelet responsiveness in photochemical treated and gamma-irradiated single-donor platelet concentrates during long-term storage. Transfusion (2007) 47:653–65. doi:10.1111/j.1537-2995.2007.01167.x
101. Lozano M, Galan A, Mazzara R, Corash L, Escolar G. Leukoreduced buffy coat-derived platelet concentrates photochemically treated with amotosalen HCl and ultraviolet A light stored up to 7 days: assessment of hemostatic function under flow conditions. Transfusion (2007) 47:666–71. doi:10.1111/j.1537-2995.2007.01169.x
102. Picker SM, Schneider V, Gathof BS. Platelet function assessed by shear-induced deposition of split triple-dose apheresis concentrates treated with pathogen reduction technologies. Transfusion (2009) 49:1224–32. doi:10.1111/j.1537-2995.2009.02092.x
103. Li J, Lockerbie O, de Korte D, Rice J, McLean R, Goodrich RP. Evaluation of platelet mitochondria integrity after treatment with Mirasol pathogen reduction technology. Transfusion (2005) 45:920–6. doi:10.1111/j.1537-2995.2005.04381.x
104. Tynngård N, Johansson BM, Lindahl TL, Berlin G, Hansson M. Effects of intercept pathogen inactivation on platelet function as analysed by free oscillation rheometry. Transfus Apher Sci (2008) 38:85–8. doi:10.1016/j.transci.2007.12.012
105. Terada C, Shiba M, Nagai T, Satake M. Effects of riboflavin and ultraviolet light treatment on platelet thrombus formation and thrombus stability on collagen. Transfusion (2017) 57:1772–80. doi:10.1111/trf.14114
106. Abonnenc M, Sonego G, Kaiser-Guignard J, Crettaz D, Prudent M, Tissot JD, et al. In vitro evaluation of pathogen-inactivated buffy coat-derived platelet concentrates during storage: psoralen-based photochemical treatment step-by-step. Blood Transfus (2015) 13:255–64. doi:10.2450/2014.0082-14
107. Jansen GA, van Vliet HH, Vermeij H, Beckers EA, Leebeek FW, Sonneveld P, et al. Functional characteristics of photochemically treated platelets. Transfusion (2004) 44:313–9. doi:10.1111/j.1537-2995.2003.00588.x
108. Picker SM, Oustianskaia L, Schneider V, Gathof BS. Functional characteristics of apheresis-derived platelets treated with ultraviolet light combined with either amotosalen-HCl (S-59) or riboflavin (vitamin B2) for pathogen-reduction. Vox Sang (2009) 97:26–33. doi:10.1111/j.1423-0410.2009.01176.x
109. Chen Z, Schubert P, Culibrk B, Devine DV. p38MAPK is involved in apoptosis development in apheresis platelet concentrates after riboflavin and ultraviolet light treatment. Transfusion (2015) 55:848–57. doi:10.1111/trf.12905
110. Diquattro M, De Francisci G, Bonaccorso R, Tagliavia AM, Marcatti M, Palma B, et al. Evaluation of amotosalem treated platelets over 7 days of storage with an automated cytometry assay panel. Int J Lab Hematol (2013) 35:637–43. doi:10.1111/ijlh.12102
111. Ostrowski SR, Bochsen L, Salado-Jimena JA, Ullum H, Reynaerts I, Goodrich RP, et al. In vitro cell quality of buffy coat platelets in additive solution treated with pathogen reduction technology. Transfusion (2010) 50:2210–9. doi:10.1111/j.1537-2995.2010.02681.x
112. Johnson L, Hyland R, Tan S, Tolksdorf F, Sumian C, Seltsam A, et al. In vitro quality of platelets with low plasma carryover treated with ultraviolet C light for pathogen inactivation. Transfus Med Hemother (2016) 43:190–7. doi:10.1159/000441830
113. Seghatchian J, Krailadsiri P. Platelet storage lesion and apoptosis: are they related? Transfus Apher Sci (2001) 24:103–5. doi:10.1016/S0955-3886(00)00134-X
114. Lebois M, Josefsson EC. Regulation of platelet lifespan by apoptosis. Platelets (2016) 27:497–504. doi:10.3109/09537104.2016.1161739
115. Leytin V. Apoptosis in the anucleate platelet. Blood Rev (2012) 26:51–63. doi:10.1016/j.blre.2011.10.002
116. Reid S, Johnson L, Woodland N, Marks DC. Pathogen reduction treatment of buffy coat platelet concentrates in additive solution induces proapoptotic signaling. Transfusion (2012) 52:2094–103. doi:10.1111/j.1537-2995.2011.03558.x
117. Bashir S, Cookson P, Wiltshire M, Hawkins L, Sonoda L, Thomas S, et al. Pathogen inactivation of platelets using ultraviolet C light: effect on in vitro function and recovery and survival of platelets. Transfusion (2015) 53:990–1000. doi:10.1111/j.1537-2995.2012.03854.x
118. Stivala S, Gobbato S, Infanti L, Reiner MF, Bonetti N, Meyer SC, et al. Amotosalen/ultraviolet A pathogen inactivation technology reduces platelet activatability, induces apoptosis and accelerates clearance. Haematologica (2017) 102:1650–60. doi:10.3324/haematol.2017.164137
119. Burnouf T, Goubran HA, Chou ML, Devos D, Radosevic M. Platelet microparticles: detection and assessment of their paradoxical functional roles in disease and regenerative medicine. Blood Rev (2014) 28:155–66. doi:10.1016/j.blre.2014.04.002
120. Marcoux G, Duchez AC, Rousseau M, Lévesque T, Boudreau LH, Thibault L, et al. Microparticle and mitochondrial release during extended storage of different types of platelet concentrates. Platelets (2017) 28:272–80. doi:10.1080/09537104.2016.1218455
121. Badimon L, Suades R, Fuentes E, Palomo I, Padró T. Role of platelet-derived microvesicles as crosstalk mediators in atherothrombosis and future pharmacology targets: a link between inflammation, atherosclerosis, and thrombosis. Front Pharmacol (2016) 7:293. doi:10.3389/fphar.2016.00293
122. Enjeti AK, Ariyarajah A, D’Crus A, Seldon M, Lincz LF. Circulating microvesicle number, function and small RNA content vary with age, gender, smoking status, lipid and hormone profiles. Thromb Res (2017) 156:65–72. doi:10.1016/j.thromres.2017.04.019
123. Burnouf T, Chou ML, Goubran H, Cognasse F, Garraud O, Seghatchian J. An overview of the role of microparticles/microvesicles in blood components: are they clinically beneficial or harmful? Transfus Apher Sci (2015) 53:137–45. doi:10.1016/j.transci.2015.10.010
124. Maurer-Spurej E, Larsen R, Labrie A, Heaton A, Chipperfield K. Microparticle content of platelet concentrates is predicted by donor microparticles and is altered by production methods and stress. Transfus Apher Sci (2016) 55:35–43. doi:10.1016/j.transci.2016.07.010
125. Kanzler P, Mahoney A, Leitner G, Witt V, Maurer-Spurej E. Microparticle detection to guide platelet management for the reduction of platelet refractoriness in children – a study proposal. Transfus Apher Sci (2017) 56:39–44. doi:10.1016/j.transci.2016.12.016
126. Hayashi T, Tanaka S, Hori Y, Hirayama F, Sato EF, Inoue M. Role of mitochondria in the maintenance of platelet function during in vitro storage. Transfus Med (2011) 21:166–74. doi:10.1111/j.1365-3148.2010.01065.x
127. Boudreau LH, Duchez AC, Cloutier N, Soulet D, Martin N, Bollinger J, et al. Platelets release mitochondria serving as substrate for bactericidal group IIA-secreted phospholipase A2 to promote inflammation. Blood (2014) 124:2173–83. doi:10.1182/blood-2014-05-573543
128. Cognasse F, Aloui C, Anh Nguyen K, Hamzeh-Cognasse H, Fagan J, Arthaud CA, et al. Platelet components associated with adverse reactions: predictive value of mitochondrial DNA relative to biological response modifiers. Transfusion (2016) 56:497–504. doi:10.1111/trf.13373
129. Bakkour S, Chafets DM, Wen L, van der Meer PF, Mundt JM, Marschner S, et al. Development of a mitochondrial DNA real-time polymerase chain reaction assay for quality control of pathogen reduction with riboflavin and ultraviolet light. Vox Sang (2014) 107:351–9. doi:10.1111/vox.12173
130. Bruchmüller I, Lösel R, Bugert P, Corash L, Lin L, Klüter H, et al. Effect of the psoralen-based photochemical pathogen inactivation on mitochondrial DNA in platelets. Platelets (2005) 16:441–5. doi:10.1080/09537100500129300
131. Bakkour S, Chafets DM, Wen L, Dupuis K, Castro G, Green JM, et al. Assessment of nucleic acid modification induced by amotosalen and ultraviolet A light treatment of platelets and plasma using real-time polymerase chain reaction amplification of variable length fragments of mitochondrial DNA. Transfusion (2016) 56:410–20. doi:10.1111/trf.13360
132. Simmons JD, Lee YL, Pastukh VM, Capley G, Muscat CA, Muscat DC, et al. Potential contribution of mitochondrial DNA damage associated molecular patterns in transfusion products to the development of acute respiratory distress syndrome after multiple transfusions. J Trauma Acute Care Surg (2017) 82:1023–9. doi:10.1097/TA.0000000000001421
133. Boilard E, Belleannee C. (Dicer)phering roles of microRNA in platelets. Blood (2016) 127:1733–4. doi:10.1182/blood-2016-01-694893
134. Plé H, Landry P, Benham A, Coarfa C, Gunaratne PH, Provost P. The repertoire and features of human platelet microRNAs. PLoS One (2012) 7:e50746. doi:10.1371/journal.pone.0050746
135. Laffont B, Corduan A, Plé H, Duchez AC, Cloutier N, Boilard E, et al. Activated platelets can deliver mRNA regulatory Ago2*microRNA complexes to endothelial cells via microparticles. Blood (2013) 122:253–61. doi:10.1182/blood-2013-03-492801
136. Osman A, Hitzler WE, Meyer CU, Landry P, Corduan A, Laffont B, et al. Effects of pathogen reduction systems on platelet microRNAs, mRNAs, activation, and function. Platelets (2015) 26:154–63. doi:10.3109/09537104.2014.898178
137. Osman A, Hitzler WE, Ameur A, Provost P. Differential expression analysis by RNA-seq reveals perturbations in the platelet mRNA transcriptome triggered by pathogen reduction systems. PLoS One (2015) 10:e0133070. doi:10.1371/journal.pone.0133070
138. Arnason NA, Landro R, Harðarson B, Guðmundsson S, Sigurjónsson ÓE. Effect of Pathogen Inactivation on MicroRNA Profile of Platelet Concentrates During Storage Under Standard Blood Banking Condition in: ISBT 2017. Copenhagen: Wiley (2017).
139. Weyrich AS, Lindemann S, Tolley ND, Kraiss LW, Dixon DA, Mahoney TM, et al. Change in protein phenotype without a nucleus: translational control in platelets. Semin Thromb Hemost (2004) 30:491–8. doi:10.1055/s-2004-833484
140. Zimmerman GA, Weyrich AS. Signal-dependent protein synthesis by activated platelets: new pathways to altered phenotype and function. Arterioscler Thromb Vasc Biol (2008) 28:s17–24. doi:10.1161/ATVBAHA.107.160218
141. Weyrich AS, Schwertz H, Kraiss LW, Zimmerman GA. Protein synthesis by platelets: historical and new perspectives. J Thromb Haemost (2009) 7:241–6. doi:10.1111/j.1538-7836.2008.03211.x
142. Klein-Bosgoed C, Schubert P, Devine DV. Riboflavin and ultraviolet illumination affects selected platelet mRNA transcript amounts differently. Transfusion (2016) 56:2286–95. doi:10.1111/trf.13715
143. Schubert P, Culibrk B, Karwal S, Goodrich RP, Devine DV. Protein translation occurs in platelet concentrates despite riboflavin/UV light pathogen inactivation treatment. Proteomics Clin Appl (2016) 10:839–50. doi:10.1002/prca.201500139
144. O’Donnell VB, Murphy RC, Watson SP. Platelet lipidomics: modern day perspective on lipid discovery and characterization in platelets. Circ Res (2014) 114:1185–203. doi:10.1161/CIRCRESAHA.114.301597
145. Slatter DA, Aldrovandi M, O’Connor A, Allen SM, Brasher CJ, Murphy RC, et al. Mapping the human platelet lipidome reveals cytosolic phospholipase A2 as a regulator of mitochondrial bioenergetics during activation. Cell Metab (2016) 23:930–44. doi:10.1016/j.cmet.2016.04.001
146. Prudent M, Crettaz D, Delobel J, Tissot JD, Lion N. Proteomic analysis of intercept-treated platelets. J Proteomics (2012) 76 Spec No:316–28. doi:10.1016/j.jprot.2012.07.008
147. Thiele T, Sablewski A, Iuga C, Bakchoul T, Bente A, Görg S, et al. Profiling alterations in platelets induced by amotosalen/UVA pathogen reduction and gamma irradiation – a LC-ESI-MS/MS-based proteomics approach. Blood Transfus (2012) 10(Suppl 2):s63–70. doi:10.2450/2012.010S
148. Marrocco C, D’Alessandro A, Girelli G, Zolla L. Proteomic analysis of platelets treated with gamma irradiation versus a commercial photochemical pathogen reduction technology. Transfusion (2013) 53:1808–20. doi:10.1111/trf.12060
149. Prudent M, D’Alessandro A, Cazenave JP, Devine DV, Gachet C, Greinacher A, et al. Proteome changes in platelets after pathogen inactivation – an interlaboratory consensus. Transfus Med Rev (2014) 28:72–83. doi:10.1016/j.tmrv.2014.02.002
150. Schubert P, Coupland D, Culibrk B, Goodrich RP, Devine DV. Riboflavin and ultraviolet light treatment of platelets triggers p38MAPK signaling: inhibition significantly improves in vitro platelet quality after pathogen reduction treatment. Transfusion (2015) 53:3164–73. doi:10.1111/trf.12173
151. Skripchenko A, Awatefe H, Thompson-Montgomery D, Myrup A, Turgeon A, Wagner SJ. An inhibition of p38 mitogen activated protein kinase delays the platelet storage lesion. PLoS One (2013) 8:e70732. doi:10.1371/journal.pone.0070732
152. Canault M, Duerschmied D, Brill A, Stefanini L, Schatzberg D, Cifuni SM, et al. p38 mitogen-activated protein kinase activation during platelet storage: consequences for platelet recovery and hemostatic function in vivo. Blood (2010) 115:1835–42. doi:10.1182/blood-2009-03-211706
153. Josefsson EC, White MJ, Dowling MR, Kile BT. Platelet life span and apoptosis. Methods Mol Biol (2012) 788:59–71. doi:10.1007/978-1-61779-307-3_5
Keywords: platelets, pathogen inactivation, transfusion, mechanisms, signaling
Citation: Schubert P, Johnson L, Marks DC and Devine DV (2018) Ultraviolet-Based Pathogen Inactivation Systems: Untangling the Molecular Targets Activated in Platelets. Front. Med. 5:129. doi: 10.3389/fmed.2018.00129
Received: 03 January 2018; Accepted: 19 April 2018;
Published: 07 May 2018
Edited by:
Michel Prudent, Transfusion Interrégionale CRS SA, SwitzerlandReviewed by:
Fabrice Cognasse, Groupe Sur L’immunité Des Muqueuses Et Agents Pathogènes (GIMAP), FranceBrian R. Branchford, University of Colorado Denver School of Medicine, United States
Christian Gachet, Établissement Français du Sang, France
Copyright: © 2018 Schubert, Johnson, Marks and Devine. This is an open-access article distributed under the terms of the Creative Commons Attribution License (CC BY). The use, distribution or reproduction in other forums is permitted, provided the original author(s) and the copyright owner are credited and that the original publication in this journal is cited, in accordance with accepted academic practice. No use, distribution or reproduction is permitted which does not comply with these terms.
*Correspondence: Dana V. Devine, dana.devine@blood.ca