- 1The Irish Centre for Fetal and Neonatal Translational Research (INFANT), University College Cork, Cork, Ireland
- 2Department of Obstetrics and Gynecology, University College Cork, Cork, Ireland
- 3Faculty of Health and Life Sciences, University of Liverpool, Liverpool, United Kingdom
- 4School of Chemistry, The University of Manchester, Manchester, United Kingdom
- 5The Manchester Institute of Biotechnology, The University of Manchester, Manchester, United Kingdom
Although it is widely considered, in many cases, to involve two separable stages (poor placentation followed by oxidative stress/inflammation), the precise originating causes of preeclampsia (PE) remain elusive. We have previously brought together some of the considerable evidence that a (dormant) microbial component is commonly a significant part of its etiology. However, apart from recognizing, consistent with this view, that the many inflammatory markers of PE are also increased in infection, we had little to say about immunity, whether innate or adaptive. In addition, we focused on the gut, oral and female urinary tract microbiomes as the main sources of the infection. We here marshall further evidence for an infectious component in PE, focusing on the immunological tolerance characteristic of pregnancy, and the well-established fact that increased exposure to the father’s semen assists this immunological tolerance. As well as these benefits, however, semen is not sterile, microbial tolerance mechanisms may exist, and we also review the evidence that semen may be responsible for inoculating the developing conceptus (and maybe the placenta) with microbes, not all of which are benign. It is suggested that when they are not, this may be a significant cause of PE. A variety of epidemiological and other evidence is entirely consistent with this, not least correlations between semen infection, infertility and PE. Our view also leads to a series of other, testable predictions. Overall, we argue for a significant paternal role in the development of PE through microbial infection of the mother via insemination.
“In one of the last articles which he wrote, the late Professor F.J. Browne (1958) expressed the opinion that all the essential facts about pregnancy toxemia are now available and that all that is required to solve the problem is to fit them together in the right order, like the pieces of a jigsaw puzzle. (1)”
“It appears astonishing how little attention has been given in reproductive medicine to the maternal immune system over the last few decades. (2)”
Introduction
Preeclampsia (PE) is a multifactorial disease of pregnancy, in which the chief manifestations are hypertension and proteinuria (3–11). It is commonest in primigravidae, where it affects some 3–5% of such pregnancies worldwide (10, 12, 13), and is associated (if untreated) with high morbidity and mortality (14–18). The incidence can be even greater in some geographical locations (19, 20). There is much literature on accompanying features, and, notwithstanding possible disease subdivisions (21, 22), the development of PE is typically seen as a “two-stage” process [e.g., Ref. (23–29)], in which in a first stage incomplete remodeling of spiral arteries leads to poor placentation. In a second stage, the resulting stress, especially hypoxia-induced oxidative stress (30–36) (and possibly hypoxia-reperfusion injury), then leads to the symptoms typical of later-pregnancy PE. However, the various actual originating causes of either of these two stages remain obscure. Many theories have been proposed [albeit a unitary explanation is unlikely (21)], and indeed, PE has been referred to as a “disease of theories” (1, 37–39). The only effective “cure” is delivery (40, 41), which often occurs significantly preterm, with its attendant complications for both the neonate and in later life (42, 43). Consequently, it would be highly desirable to improve our understanding of the ultimate causes of PE, so that better prevention or treatments might be possible.
The “two-stage” theory is well established, and nothing we have to say changes it. However, none of this serves to explain what “initiating” or “external” factors are typically responsible for the poor placentation, inflammation, and other observable features of PE (44).
Microbes are ubiquitous in the environment, and one potential external or initiating factor is low-level microbial infection. In a recent review (44), we developed the idea (and summarized extensive evidence for it) that a significant contributor to PE might be a [largely dormant (45–48) and non-replicating] microbiome within the placenta and related tissues, also detectable in blood and urine. Others [e.g., Ref. (49–56)] have drawn similar conclusions. Interestingly, recent analyses (21, 57) of placental gene expression in PE implicate changes in the expression of triggering receptor on myeloid cells-1 and the metalloprotease INHA, and in one case (21) also lactotransferrin, that also occur during infection (58–61). Although we highlighted the role of antibiotics as potentially preventative of PE (44), and summarized the significant evidence for that, we had relatively little to say about immunology, and ignored another well-known antidote to infectious organisms in the form of vaccines. There is certainly also an immune component to PE [e.g., Ref. (26, 62–70) and below]. One of the main theories of (at least part of the explanation of) PE is that of “immune maladaptation” (62, 64, 66, 71). Thus, the main focus of the present analysis is to assess the extent to which there is any immunological evidence for a role of infectious agents (and the utility of immunotolerance to or immunosuppression of them) in PE. Figure 1 summarizes our review in the form of a “mind map” (72). We begin with the broad question of immunotolerance, before turning to an epidemiological analysis. A preprint has been lodged in bioRxiv (73).
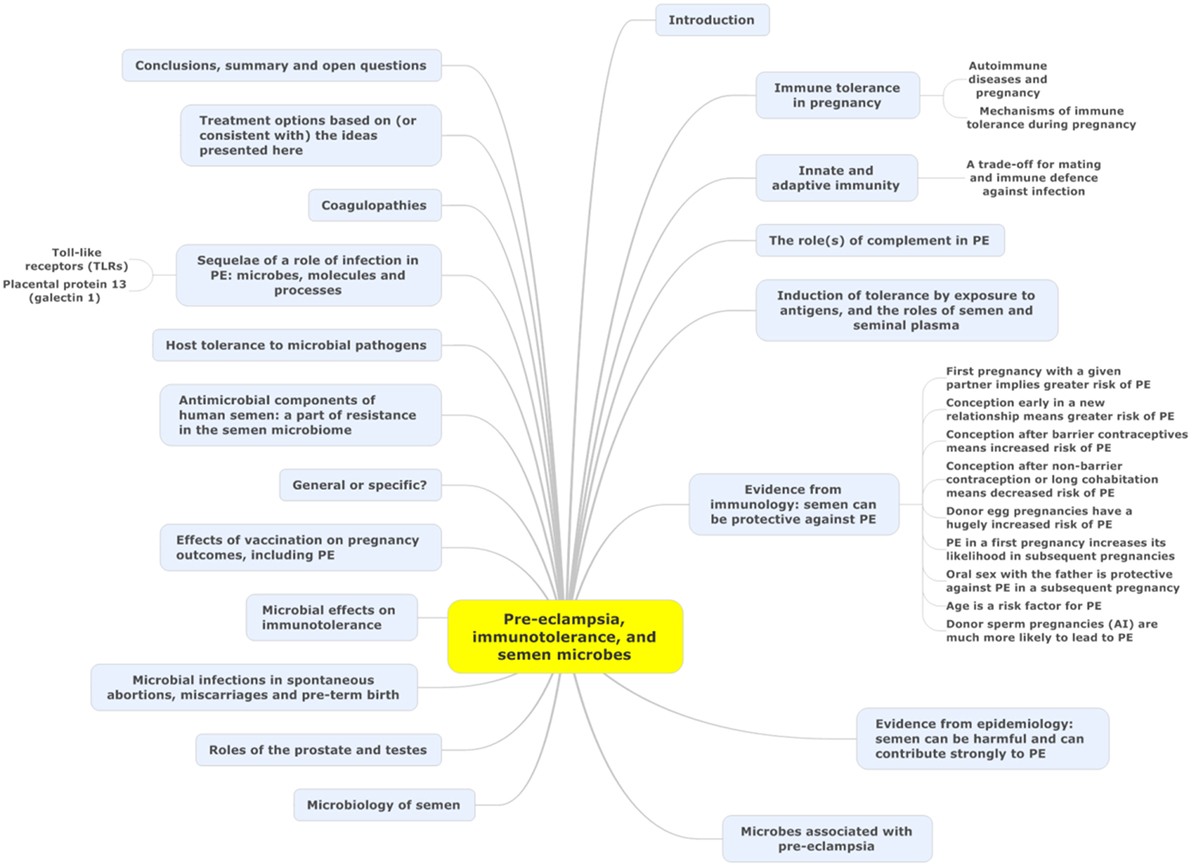
Figure 1. A “mind map” (72) of the review. Start at “midnight” and read clockwise.
Immune Tolerance in Pregnancy
Much of the original thinking on this dates back to Sir Peter Medawar (74–79), who recognized that the paternal origin of potentially half the antigens of the fetus (80) created an immunological conundrum: it should normally be expected that the fetus’s alloantigens would cause it to be attacked by the maternal immune system as “foreign.” There would therefore have to be an “immune tolerance” (79, 81–83). Historically it was believed that the fetus is largely “walled off” from the mother (84); however, we now appreciate (85–88) that significant trafficking of fetal material across the placenta into the maternal circulation and vice versa occurs throughout pregnancy. Indeed, this is the basis for the development of non-invasive prenatal testing. In line with this, grams of trophoblast alloantigens are secreted daily into the maternal circulation during the third trimester (Figure 2), and this is related to the prevalence of PE (89–95). Consequently, both the concept and the issue of immune tolerance are certainly both real and important. At all events, the immunobiology of the fetus has been treated in theory largely in the way that a transplanted graft is treated, and uteroplacental dysfunction [leading to PET and intrauterine growth restriction (IUGR)] has in some cases been regarded as a graft rejection [e.g., Ref. (70, 96–102)]. Clearly there are relationships between the immunogenicity of the foreign agent and the responsiveness of the host; to this end, Zelante et al. (103) recognize the interesting similarities between tolerance to paternal alloantigens (as in pregnancy) and the tolerance observed in chronic fungal infections. This said, the host–graft analogy is increasingly seen as somewhat naive (104–106).
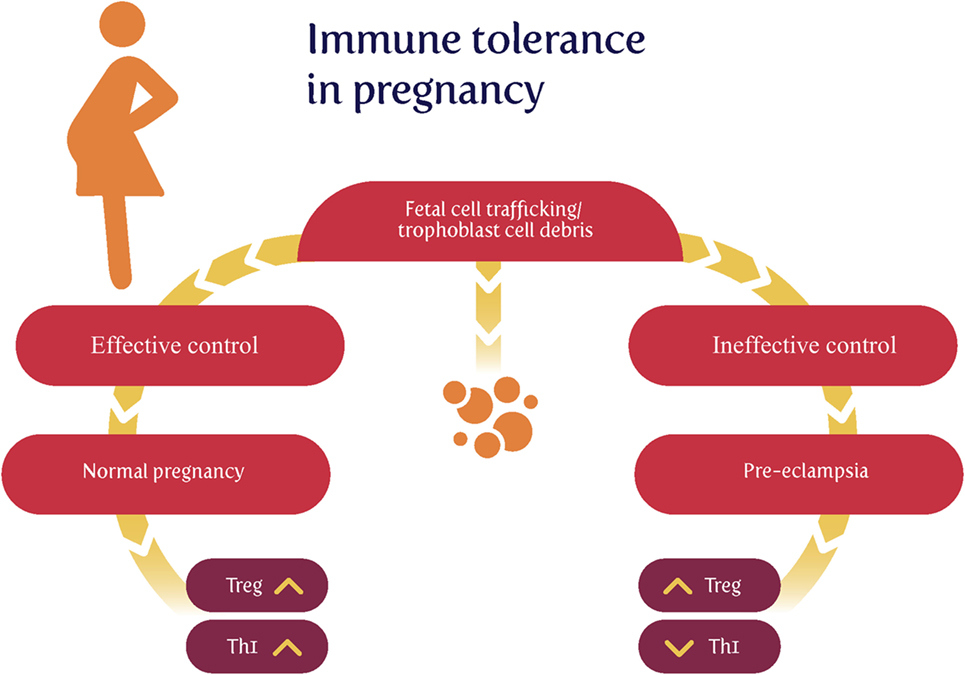
Figure 2. Effective lowering of the normal immunological response to fetal cell trafficking [sometimes referred to as “suppressed” but in fact a highly dynamic state (104, 106)] leads to a normal pregnancy, while its failure can lead to preeclampsia. We note too that other Thelper populations may play roles in the physiologic and pathologic immune interactions between mother and offspring.
The Clinical Course of Automimmune Disease during Pregnancy: An Inconsistent Effect
The seminal observation by Philip Hench that the symptoms of the rheumatoid arthritis (RA) were frequently and dramatically ameliorated by several conditions, including pregnancy (107), led to the discovery of cortisone (108) and gave unique insights into the complex interaction between the maternal immune system and the developing fetal/placental unit. Contemporary data suggests that the improvement in RA is not ubiquitous as first thought. Amongst all pregnant women about 25% of women have no improvement in their symptoms at any stage in pregnancy and in a small number of cases the disease may actually worsen (109). The process by which pregnancy affects disease activity in RA is not completely understood and several putative mechanisms have been proposed. Of interest, although plasma cortisol rises during pregnancy and was initially thought to be key in the amelioration of symptoms, there is actually no correlation between cortisol concentrations and disease state (110). It has also been reported that the degree of maternal and paternal MHC mismatch has been shown to correlate with the effect of the RA remission during pregnancy (111), leading to the hypothesis that the early immunological events in pregnancy that establish tolerance to the fetal allograft contribute to RA remission. Clearly, this may also account for the disparity in response to pregnancy. RA is not unique in being the only autoimmune disease to be profoundly altered by pregnancy. Although less well studied, non-infectious uveitis tends to improve during pregnancy from the second trimester onward, with the third trimester being associated with the lowest disease activity (112). Again, the mechanism underlying this phenomenon is not completely elucidated.
It is now generally accepted (113) that, notwithstanding the sweeping generalization, autoimmune diseases with a strong cellular (innate) pathophysiology (RA, multiple sclerosis) improve, whereas diseases characterized by autoantibody production such as systemic lupus erythematous and Grave’s disease tend toward increased severity in pregnancy.
We have previously reported an association between pregnancy and the risk of subsequent maternal autoimmune disease which was also related to the mode and gestation of delivery. There was an increased risk of autoimmune disease after cesarean section may be explained by amplified fetal cell traffic at delivery, while decreased risks after abortion may be due to the transfer of more primitive fetal stem cells (114).
Mechanisms of Immune Tolerance during Pregnancy
Following the recognition of maternal immunotolerance, a chief discovery was the choice of HLA-G, a gene with few alleles, for the antigens used at the placental interface. Thus, the idea that placental HLA-G proteins facilitate semiallogeneic pregnancy by inhibiting maternal immune responses to foreign (paternal) antigens via these actions on immune cells is now well established (115–120).
It is also well established that regulatory T cells (Tregs) play an indispensable role in maintaining immunological unresponsiveness to self-antigens and in suppressing excessive immune responses deleterious to the host (121). Consequently, much of present thinking seems to involve a crucial role for Tregs in maintaining immunological tolerance during pregnancy (70, 77, 122–132), with the result that effector T cells cannot accumulate within the decidua (the specialized stromal tissue encapsulating the fetus and placenta) (133).
In an excellent review, Williams et al. (134) remark “Regulatory T cells (Tregs) are a subset of inhibitory CD4+ helper T cells that function to curb the immune response to infection, inflammation, and autoimmunity.” “There are two developmental pathways of Tregs: thymic (tTreg) and extrathymic or peripheral (pTreg). tTregs appear to suppress autoimmunity, whereas pTregs may restrain immune responses to foreign antigens, such as those from diet, commensal bacteria, and allergens.” Their differential production is controlled by a transcription factor called Foxp3.
Further, “a Foxp3 enhancer, conserved noncoding sequence 1 (CNS1), essential for pTreg but dispensable for tTreg cell generation, is present only in placental mammals. It is suggested that during evolution, a CNS1-dependent mechanism of extrathymic differentiation of Treg cells emerged in placental animals to enforce maternal–fetal tolerance” (135).
Williams et al. conclude that “These findings indicate that maternal–fetal tolerance to paternal alloantigens is an active process in which pTregs specifically respond to paternal antigens to induce tolerance. Thus, therapies should aim not to suppress the maternal immune system but rather to enhance tolerance. These findings are consistent with an increase in the percentage of Tregs during pregnancy and with no such increase in women with recurrent pregnancy loss (136)” (134). Thus maternal tolerance is based on exposure to the paternal alloantigens, although mechanisms such as the haem oxygenase detoxification of haem from degrading erythrocytes (137) are also important. Note too that pregnancy loss is often caused by automimmune activity (138) (and see later).
Additionally, Treg cells have several important roles in the control of infection [e.g., Ref. (139–144)]. These include moderating the otherwise potentially dangerous response to infection and being exploited by certain parasites to induce immunotolerance.
Finally, here, it is also recognized that the placenta does allow maternal IgG antibodies to pass to the fetus to protect it against infections. Also, foreign fetal cells persist in the maternal circulation (145) [as does fetal DNA (146, 147), nowadays used in prenatal diagnosis]. One cause of PE is clearly an abnormal immune response toward the placenta. There is substantial evidence for exposure to partner’s semen as prevention for PE, largely due to the absorption of several immune modulating factors present in seminal fluid (148). We discuss this in detail below.
Innate and Adaptive Immunity
Although they are not entirely independent (149, 150), and both respond to infection, it is usual to discriminate (the faster) innate and (the more leisurely) adaptive immune responses [e.g., Ref. (151–155)]. As is well known [reviewed recently (156)], the innate immune system is responsible for the recognition of foreign organisms such as microbes. It would be particularly convenient if something in the immune response did actually indicate an infection rather than simply any alloantigen, but unfortunately—especially because of the lengthy timescale over which PE develops—innate responses tend to morph into adaptive ones. This means (i) that there may be specific signals from early innate events that may be more or less specific to innate responses and (ii) that it also does not exclude the use of particular patterns of immune responsive elements (157–159) to characterize disease states.
An alteration of the immune system is widely recognized as an accompaniment to normal pregnancy (77, 104–106, 127, 160–162), and especially in PE (63–65, 67, 69–71, 163–170), and it is worth looking at it a little more closely.
The innate immune system responds to microbial components such as lipopolysaccharide (LPS) via cell membrane receptors. Innate immune cells express a series of evolutionarily conserved receptors known as pattern-recognition receptors (PRRs). PRRs recognize and bind conserved sequences known as pathogen-associated molecular patterns (PAMPs). Bacterial LPS and peptidoglycan, and double stranded viral RNA are unique to microbes and act as canonical PAMPs, while the main family of PRRs is represented by the Toll-like receptors (TLRs) (171, 172). Downstream events, as with many others (173, 174) converge on the NF-κB system and/or interferon, leading to the release of a series of inflammatory cytokines such as IL-2, IL-6, IL-8, TNF-α, and especially IL-1β.
Matzinger’s “danger model” (175–180) [and see Ref. (79) and Figure 3] suggested that activation of the immune system could be evoked by danger signals from endogenous molecules expelled from injured/damaged tissues, rather than simply from the recognition of non-self (although of course in the case of pregnancy some of these antigens are paternal alloantigens). Such endogenous molecules are referred to as damage-associated molecular patterns (DAMPs), but are not our focus here, albeit they likely have a role in at least some elements of PE (181). We shall see later, however, that Matzinger’s theory is entirely consistent with the kinds of microbial (and disease) tolerance that do seem to be an important part of pregnancy and PE [and see Ref. (182)].
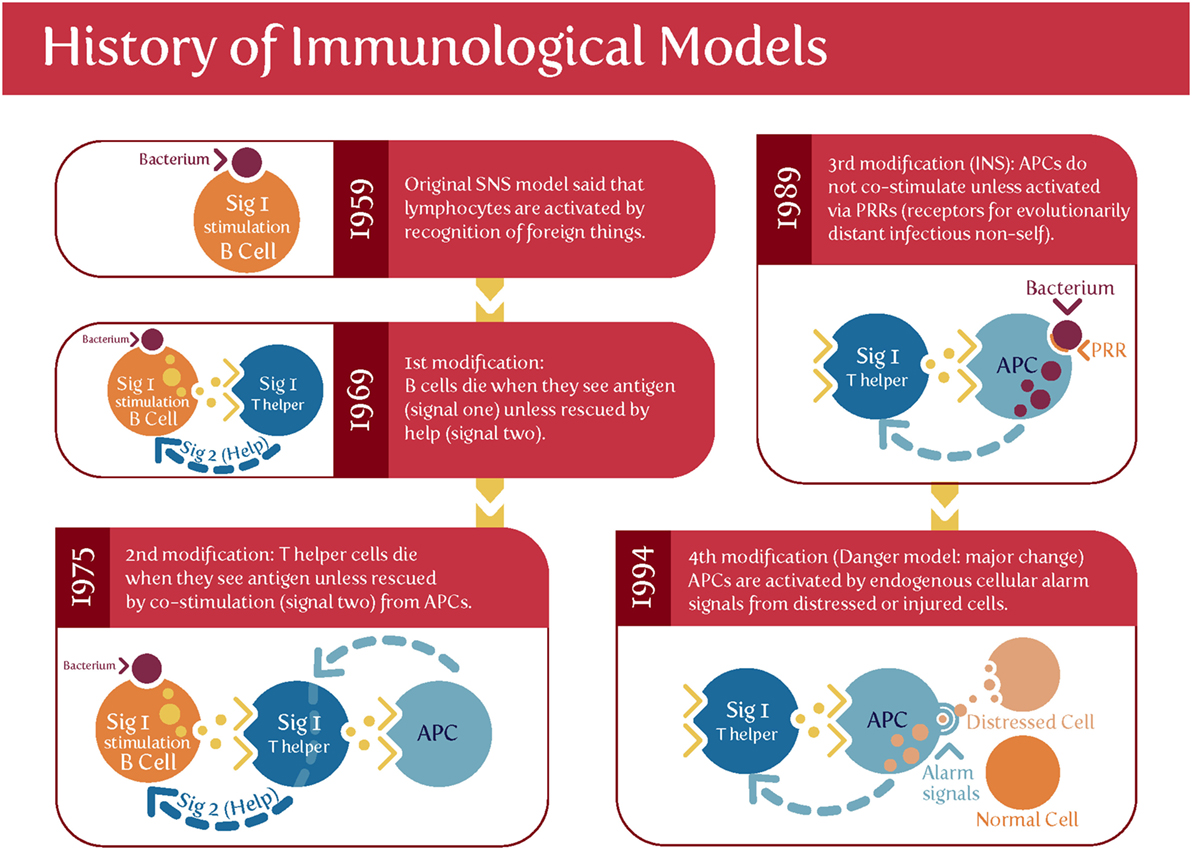
Figure 3. Matzinger’s “danger model” vs. the classical theory of self vs. self-nonself. Based on and redrawn from Ref. (178).
The maternal innate immune system plays an important role both in normal pregnancy and in particular in hypertensive disorders of pregnancy including preeclampsia (PE) (167, 183–189). One persuasive and widely accepted view is that normal pregnancy is characterized by a low-grade systemic inflammatory response and specific metabolic changes, and that virtually all of the features of normal pregnancy are simply exaggerated in PE (44, 183, 190, 191). Certainly it is long established that “Normal pregnancy and PE both produce inflammatory changes in peripheral blood leukocytes akin to those of sepsis” (183), and there are innate immune defenses in the uterus during pregnancy (160). Normal pregnancy has been considered to be a Th2 type immunological state that favors immune tolerance in order to prevent fetal rejection (137). However, normal pregnancy actually fluctuates between pro- (implantation and placentation; parturition) and anti-inflammatory (fetal growth) phases (105, 106). By contrast, PE has been classically described as a Th1/Th2 imbalance (125, 164, 192–194), but as mentioned above [and before (44)], recent studies have highlighted the role of Tregs as part of a Th1/Th2/Th17 paradigm (167, 168). This leads to the question of whether there is some kind of trade-off between the responses to paternal alloantigens and those of microbes.
A Trade-Off for Mating and Immune Defense against Infection
Certainly there is some evidence for a trade-off between mating and immune defense against infection (195–197). Consistent with this (albeit with much else) is the fact (198–200) that pregnancy is associated with an increased severity of at least some infectious diseases. There is evidence (201, 202) that “adaptive immune responses are weakened, potentially explaining reduced viral clearance. Evidence also suggests a boosted innate response, which may represent a compensatory immune mechanism to protect the pregnant mother and the fetus and which may imply decreased susceptibility to initial infection” (199).
The Role(S) of Complement in PE
Complement, or more accurately the complement cascade, is an important part of the innate immune system that responds to infection. Later (downstream) elements also respond to the adaptive immune system. Our previous review (44) listed many proteins whose concentrations are changed in both infection and PE. Since we regard low-level infection as a major cause of the inflammation observed in PE, one would predict that the complement system is activated in PE, and this observation is amply borne out (203–217). Some of the details are mentioned in Table 1.
The complement cascade may be activated in three main ways (Figure 4), known as classical, alternative or lectin pathways (150, 206, 208, 228, 229). Complement activation by the classical, alternative or lectin pathway results in the generation of split products C3a, C4a, and C5a with proinflammatory properties.
Because both innate and adaptive immunity can activate elements of the downstream complement system, it is hard to be definitive, but there is some evidence that elements such as Ba and Bb [the latter of known structure (230)] are selectively released during infection, very much upstream and in the alternative pathway (208, 228, 229, 231–233). Most importantly (Table 1), while probably not a specific serum marker, there is considerable evidence that Bb levels are increased in PE, arguably providing further evidence for a role of infectious agents in the etiology of PE.
We might also note that C1q−/− mice shows features of PE (234), consistent with the view that lowering levels of anti-infection response elements of the complement system leads to PE, consistent again with an infectious component to PE.
Induction of Tolerance by Exposure to Antigens and Our Main Hypothesis: Roles of Semen and Seminal Plasma
A number of groups [e.g., Ref. (118, 148, 235–240)] have argued for a crucial role of semen in inducing maternal immunological protection, and this is an important part of our own hypothesis here. The second component, however, is a corollary of it. If it is accepted that semen can have beneficial effects, it may also be that in certain cases it can also have harmful effects. Specifically, we rehearse the fact that semen is not sterile, and that it can be a crucial source of the microbes that may, over time, be responsible for the development of PE (and indeed other disorders of pregnancy, some of which we rehearse).
Semen consists essentially of the sperm cells suspended in a fluid known as seminal plasma (241). Seminal plasma contains many components (242, 243), such as transforming growth factor β (TGF-β) (236, 244–248), and there is much evidence that a number of them are both protective and responsible for inducing the immune tolerance observed in pregnancy. Thus, in a key article on the issue, Robertson et al. state, “TGF-β has potent immune-deviating effects and is likely to be the key agent in skewing the immune response against a Type-1 bias. Prior exposure to semen in the context of TGF-β can be shown to be associated with enhanced fetal/placental development late in gestation. In this article, we review the experimental basis for these claims and propose the hypothesis that, in women, the partner-specific protective effect of insemination in PE might be explained by induction of immunological hyporesponsiveness conferring tolerance to histocompatibility antigens present in the ejaculate and shared by the conceptus” (148).
Transforming growth factor-β and prostaglandin E [also prevalent in seminal fluid (249)] are potent Treg cell-inducing agents, and coitus is one key factor involved in expanding the pool of inducible Treg cells that react with paternal alloantigens shared by conceptus tissues (250–253).
Both in humans and in agricultural practice, semen may be stored with or without the seminal fluid (in the latter cases, the sperm have been removed from it and they alone are used in the insemination). However, a number of articles have shown very clearly that it is the seminal fluid itself that contains many protective factors, not least in improving the likelihood of avoiding adverse pregnancy outcomes (148, 197, 254, 255). Thus semen is the preferred substrate for inducing immunotolerance and hence protection against PE.
Evidence from Epidemiology—Semen Can be Protective Against PE
As well as those [such as preexisting diseases such as hypertension and diabetes (256, 257), that we covered previously (44)], there are several large-scale risk (or antirisk) factors that correlate with the incidence of PE. They are consistent with the idea that a woman’s immune system adapts slowly to (semen) proteins from a specific male partner (148, 235, 236), and that the content of semen thus has major phenotypic effects well beyond its donation of (epi)genetic material. We believe that our hypothesis about the importance of semen in PE has the merit of being able to explain each of them in a simple and natural way:
1. The first pregnancy with any given partner means an increased susceptibility to PE (5, 258, 259).
2. Conception early in a new relationship means an increased susceptibility to PE (260–262).
3. Conception after using barrier contraceptives means an increased susceptibility to PE (261, 263, 264).
4. Conception after using non-barrier methods or after a long period of cohabitation means a decreased susceptibility to PE (235, 261).
5. Donor egg pregnancies have a hugely inflated chance of PE (259, 265–267).
6. PE in a first pregnancy increases its likelihood in subsequent pregnancies (268).
7. Oral sex with the father is protective against PE in a subsequent pregnancy (269, 270).
8. Age is a risk factor for PE (271–275).
9. Donor sperm pregnancies (artificial insemination) are much more likely to lead to PE (270, 276–279).
We consider each in turn (Figure 5).
The First Pregnancy with Any Given Partner Means an Increased Susceptibility to PE
This is extremely well established [e.g., Ref. (5, 67, 163, 256, 258, 259, 280–288)]. Thus, Duckitt and Harrington (256) showed nulliparity to have a risk ratio (over pregnant women with previous pregnancies) of 2.91 (95% CI 1.28–6.61). Luo et al. (283) find an odds ratio (OR) of 2.42 (95% CI 2.16–2.71) for PE in primiparous vs. multiparous women, while Deis et al. found the OR to be 2.06 (CI 1.63–2.60), P = 0.0021. Dildy et al. (289) summarize several studies, including a very large one by Conde-Agudelo and Belizán (290) (RR 2.38; 95% CI 2.28–2.49), while the meta-analysis of English et al. (287) gives a risk ratio for nulliparity of 2.91 (CI 1.28–6.61). The consistency of each of these studies allows one to state with considerable confidence that there is a two- to threefold greater chance of PE with a first baby.
However, an additional and key clue here is not simply (and maybe even not mainly) that it is just being nulliparous (i.e., one’s first pregnancy) but that it is primipaternity—one’s first pregnancy with a given father—that leads to an increased susceptibility to PE (19, 204, 291–303) [cf. (304)]. Changing partners effectively “resets the clock” such that the risk with a new father is essentially as for first pregnancies. Thus, Lie et al. (305) noted that if a woman becomes pregnant by a man who has already fathered a pre-eclamptic pregnancy in a different woman her increased risk of developing pre-eclampsia is 1.8-fold (CI 1.2–2.6). This is far greater than the typical incidence of PE, even for nulliparous women. The equivalent figure in the study of Lynch et al. (204) was RR = 5.1, 95% CI 1.6–15. The strong implication of all of this is that the father can have bad effects but that some kind of “familiarity” with the partner is protective (301), the obvious version—and that more or less universally accepted—being an immunological familiarity (i.e., tolerance). Note, however, that this is when the pregnancy goes to term: a prior birth confers a strong protective effect against PE, whereas a prior abortion confers only a weaker protective effect (259).
Conception Early in a New Relationship Means an Increased Susceptibility to PE
The idea that conception early in a new relationship means an increased susceptibility to PE follows immediately from the above. The landmark studies here are those of Robillard et al. (19, 260, 296), of Einarsson et al. (261), and of Saftlas et al. (262).
Robillard et al. (260) studied 1,011 consecutive mothers in an obstetrics unit. The incidence of pregnancy-induced hypertension (PIH) was 11.9% among primigravidae, 4.7% among same-paternity multigravidae, and 24.0% among new-paternity multigravidae. For both primigravidae and multigravidae, the length of (sexual) cohabitation before conception was inversely related to the incidence of PIH (P < 0.0001).
Einarsson et al. (261) studied both the use of barrier methods and the extent of cohabitation prior to pregnancy. For those (allegedly, etc.) using barrier methods before insemination, the OR for PE when prior cohabitation was only 0–4 months versus the OR for PE: normotensive was 17.1 (CI 2.9–150.6) versus 1.2 (CI 0.1–11.5) when the period of cohabitation was 8–12 months, and 1.0 for periods of cohabitation exceeding 1 year.
Saftlas et al. (262) recognized that parous women who change partners before a subsequent pregnancy appear to lose the protective effect of a prior birth. In a large study (mainly based around calcium supplementation), they noted that women with a history of abortion who conceived again with the same partner had nearly half the risk of PE [adjusted odds ratio (aOR) = 0.54, 95% confidence interval: 0.31–0.97]. In contrast, women with an abortion history who conceived with a new partner had the same risk of PE as women without a history of abortion (aOR = 1.03, 95% confidence interval: 0.72–1.47). Thus, the protective effect of a prior abortion operated only among women who conceived again with the same partner.
Conception after Using Barrier Contraceptives Means an Increased Susceptibility to PE
A prediction that follows immediately from the idea that paternal antigens in semen (or seminal fluid) are protective is that the regular use of barrier methods will lower maternal exposure to them, and hence increase the likelihood of PE. This too is borne out (261, 263, 264). Thus Klonoff-Cohen et al. found a 2.37-fold (CI 1.01–5.58) increased risk of PE for users of contraceptives that prevent exposure to sperm. A dose-response gradient was observed, with increasing risk of PE for those with fewer episodes of sperm exposure. Similarly, Hernández-Valencia et al. (264) found that the OR for PE indicated a 2.52-fold (CI 1.17–5.44, P < 0.05), increased risk of PE for users of barrier contraceptives compared with women using nonbarrier contraceptive methods.
Conception after Using Non-Barrier Methods or after a Long Period of Cohabitation Means a Decreased Susceptibility to PE
This is the flip side of the studies given above [e.g., Ref. (260–262)]. It is clear that maternal–fetal HLA sharing is associated with the risk of PE, and the benefits of long-term exposure to the father’s semen, while complex (306), seem to be cumulative (307). Thus, short duration of sexual relationship was more common in women with PE compared with uncomplicated pregnancies [≤6 months 14.5 versus 6.9%, aOR 1.88, 95% CI 1.05–3.36; ≤ 3 months 6.9% versus 2.5%, aOR 2.32, 95% CI 1.03–5.25 (308)]. Oral contraceptives are somewhat confounding here, in that they may either be protective or a risk factor depending on the duration of their use and the mother’s physiological reaction to them (309).
Donor Egg Pregnancies Have a Hugely Inflated Chance of PE
If an immunological component is important to PE (as it evidently is), it is to be predicted that donor egg pregnancies are likely to be at much great risk of PE, and they are [e.g., Ref. (259, 265–267, 310–314)] [and also of preterm birth (PTB) (315)]. Thus, Letur et al. (265, 266) found that PE was some fourfold more prevalent using donated eggs (11.2 vs. 2.8%, P < 0.001), while Tandberg et al. (259) found that various “assisted reproductive technologies” had risk ratios of 1.3 (1.1–1.6) and 1.8 (1.2–2.8) in second and third pregnancies, respectively. Pecks et al. studied PIH (not just PE) and found that the calculated OR for PIH after oocyte donation, compared to conventional reproductive therapy, was 2.57 (CI 1.91–3.47), while the calculated OR for PIH after oocyte donation, compared to other women in the control group, was 6.60 (CI 4.55–9.57). Stoop et al. (316) found a Risk Ratio of 1.502 (CI 1.024–2.204) for PIH. In a study by Levron et al. (317), adjustment for maternal age, gravidity, parity, and chronic hypertension revealed that oocyte donation was independently associated with a higher rate of hypertensive diseases of pregnancy (P < 0.01). In a twins study, Fox et al. (318) found, on adjusted analysis, that the egg donation independently associated with PE (aOR 2.409, CI 1.051–5.524). The meta-anaysis of Thomopoulos et al. (319) gave a risk ratio for egg donation of 3.60 (CI 2.56–5.05) over controls, a value similar to that of Blázquez et al. (320). Finally, a recent meta-analysis by Masoudian et al. (313) found that that the risk of PE is considerably higher in oocyte-donation pregnancies compared to other methods of assisted reproductive technology (OR, 2.54; CI 1.98–3.24; P < 0.0001) or to natural conception (OR, 4.34; CI 3.10–6.06; P < 0.0001). The incidence of gestational hypertension and PE was significantly higher in ovum donor recipients compared with women undergoing autologous IVF [24.7% compared with 7.4%, P < 0.01, and 16.9% compared with 4.9%, P < 0.02 (321)]. All of these are entirely consistent with an immune component being a significant contributor to PE. Given our suggestion that many of these disorders of pregnancy have a microbial component, one obvious question pertains to whether the use of antibiotics assists the successful progression of IVF. Unfortunately this question has been little researched in humans (322).
PE in a First Pregnancy Increases Its Likelihood in Subsequent Pregnancies
This too is well established: a woman who has had PE has an increased risk of PE in subsequent pregnancies (288, 323), especially if suffering from hypertension (324). This may be seen as relatively unsurprising, and of course bears many explanations, and the increased risks can be very substantial (268). In the overall analysis of English et al. (287), the risk ratio was 7.19 (CI 5.85–8.83). Other examples give the recurrence risk, overall, as some 15–18% (288). The risk of recurrent PE is inversely related to gestational age at the first delivery, and in the study of Mostello et al. (325) was 38.6% for 28 weeks’ gestation or earlier, 29.1% for 29–32 weeks, 21.9% for 33–36 weeks, and 12.9% for 37 weeks or more. Low birthweight in the first pregnancy is an independent predictor of PE in the second: birth weight below the tenth percentile in the first delivery accounted for 10% of the total cases of PE in the second pregnancy and 30% of recurrent cases (326). From the perspective developed here, the suggestion is that whatever is responsible for PE in one pregnancy can “live on” in the mother and afflict subsequent ones. One thing that can “live on” is a dormant microbial community. We discussed at length in the previous review (44), and develop in more detail later (in the section “host tolerance to microbial pathogens”) the evidence that dormant microbes (such as Helicobacter pylori and Mycobacterium tuberculosis) can live within their host for decades.
Oral Sex with the Father Is Protective against PE in a Subsequent Pregnancy
Oral sex (with the father of one’s baby) protects against PE (269, 270) (P = 0.0003), arguably because exposure to the paternal antigens in the seminal fluid have a greater exposure to the blood stream via the buccal mucosa than they would via the vagina. This is a particularly interesting (and probably unexpected) finding, that is relatively easily understood from an immunological point of view, and it is hard to conceive of alternative explanations. [Note, however, that in the index study (269), the correlation or otherwise of oral and vaginal sex was not reported, so it is not entirely excluded that more oral sex also meant more vaginal sex.]
Age Is a Risk Factor for PE
Age is a well known risk factor for PE (271–275), and of course age is a risk factor for many other diseases, so we do not regard this as particularly strong evidence for our ideas. However, we have included it in order to note that age-associated microbial dysbiosis promotes intestinal permeability, systemic inflammation, and macrophage dysfunction (327).
Donor Sperm Pregnancies (Artificial Insemination) Are Much More Likely to Lead to PE
Finally, here, turning again to the father, it has been recognized that certain fathers can simply be “dangerous” in terms of their ability to induce PE in those who they inseminate (302, 328). By contrast, if immunotolerance to a father builds up slowly as a result of cohabitation and unprotected sex, a crucial prediction is that donor sperm pregnancies will not have this property, and should lead to a much greater incidence of PE. This is precisely what is observed (270, 276–279, 310).
In an early study (276), Need et al. observed that the overall incidence of PE was high (9.3%) in pregnancies involving artificial insemination by donor (AID) compared with the expected incidence of 0.5–5.0%. The expected protective effect of a previous pregnancy was not seen, with a 47-fold increase in PE (observed versus expected) in AID pregnancies after a previous full-term pregnancy. That is a truly massive risk ratio.
Smith et al. (277) compared the frequency of PE when AI was via washed sperm from a partner or a donor, finding a relative risk for PE of 1.85 (95% CI 1.20–2.85) for the latter, and implying that the relevant factor was attached to (in or on) the sperm themselves.
In a similar kind of study, Hoy et al. found (278), after adjusting for maternal age, multiple birth, parity and presentation, that “donor sperm” pregnancies were more likely to develop PE (OR 1.4, 95% CI 1.2–1.8).
Salha et al. (310) found that the incidence of PE in pregnancies resulting from donated spermatozoa was 18.2% (6/33) compared with 0% in the age- and parity-matched partner insemination group (P < 0.05).
Wang et al. (329) found that the risk of PE tripled in those never exposed to their partner’s sperm, i.e., those treated with intracytoplasmatic sperm injection done with surgically obtained sperm.
In a study of older women, Le Ray et al. (330) noted that the PE rate differed significantly between various groups using assisted reproductive technology (3.8% after no IVF, 10.0% after IVF only, and 19.2% after IVF with oocyte donation, P < 0.001).
Davis and Gallup reviewed what was known in 2006 (279), particularly from an evolutionary point of view, concluding that one interpretation of PE was that it was the mother’s way of removing “unsuitable” fetuses. This does not sit easily with the considerable mortality and morbidity associated with PE predelivery, especially in the absence of treatment. However, Davis and Gallup (279) did recognize that “pregnancies and children that result from unfamiliar semen have a lower probability of receiving sufficient paternal investment than do pregnancies and children that result from familiar semen,” and that is fully consistent with our general thinking here. Bonney draws a similar view (182), based on the “danger” model (176, 178), that takes a different view from that of the “allograft” or “self-nonself discrimination” model. In the “danger model,” the decision to initiate an immune response is based not on discrimination between self and non-self, but instead is based on the recognition of “danger” (abnormal cell death, injury, or stress). One such recognition is the well-established recognition of microbes as something likely to be causative of undesirable outcomes.
In the study of González-Comadran et al. (331), conception using donor sperm was again associated with an increased risk of PE (OR 1.63, 95% CI 1.36–1.95).
Thomopoulos et al. carried out two detailed and systematic reviews (319, 332); the latter (319) covered 7,038,029 pregnancies (203,375 following any invasive ART) and determined that the risk of PE was increased by 75% (95% CI 50–103%).
Overall, these studies highlight very strongly indeed that the use of unfamiliar male sperm is highly conducive to PE relative to that of partner’s sperm, especially when exposure is over a long period. We next turn to the question of why, in spite of this, we also see PE even in partner-inseminated semen, as well as more generally.
Evidence from Epidemiology—Semen Can be Harmful and Can Contribute Strongly to PE
In our previous review (44), we rehearsed the evidence for a considerable placental and vaginal microbiome [see also (333–335)], but did not discuss the semen microbiome at all. To repeat, therefore, the particular, and essentially novel, part of our hypothesis here is that if it is accepted that semen (and seminal plasma) can have beneficial effects, it should also be recognized that in certain cases it can also have harmful effects. In particular, we shall be focusing on its microbial content [we ignore any epigenetic effects (336)]. We note that this idea would fit easily with the recognition that as well as inducing tolerance to paternal antigens, exposures to the father’s semen can build tolerance (immunity) to its microbes, thereby decreasing the risk of PE. However, microbes and their associated PAMPs are well known to be highly inflammatory, whether or not they are reproducing, and we consider that it is this that is likely the particular driver of the sequelae observable in PE.
Microbes Associated with PE
The female’s urogenital microbiome is important in a number of pregnancy disorders (56, 337–339). Specifically, we previously found many examples in which microbes are associated with PE, and we here update the CC-BY-licensed Table 2 thereof (44).
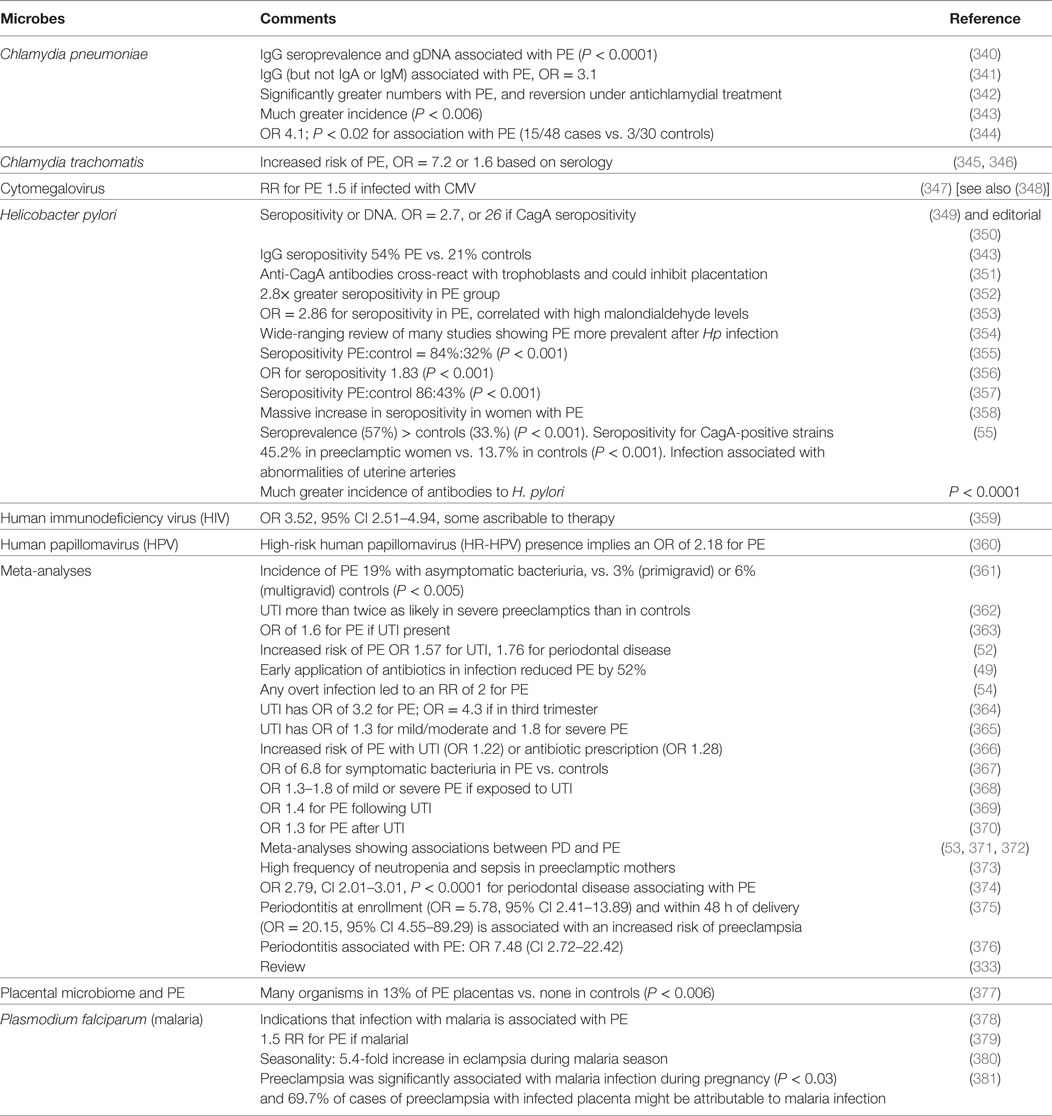
Table 2. Many studies have identified a much greater prevalence of infectious agents in the blood or urine or gums of those exhibiting PE than in matched controls.
In addition, we recognize the considerable evidence for a role of viruses in various disorders of pregnancy (106, 382, 383).
Microbiology of Semen
Semen itself is very far from being sterile, even in normal individuals, with infection usually being defined as 103 organisms/mL semen (384). Of course the mere existence of sexually transmitted diseases implies strongly that there is a seminal fluid (or semen) microbiome that can vary substantially between individuals, and that can contribute to infection [e.g., Ref. (385–387)], fertility (385) (and see below), and any other aspect of pregnancy (388), or even health in later life (389).
It is logical to start here with the observation that semen is a source of microbes from the fact that there are a great many sexually transmitted infectious diseases for which it is the vehicle. Table 3 summarizes some of these.
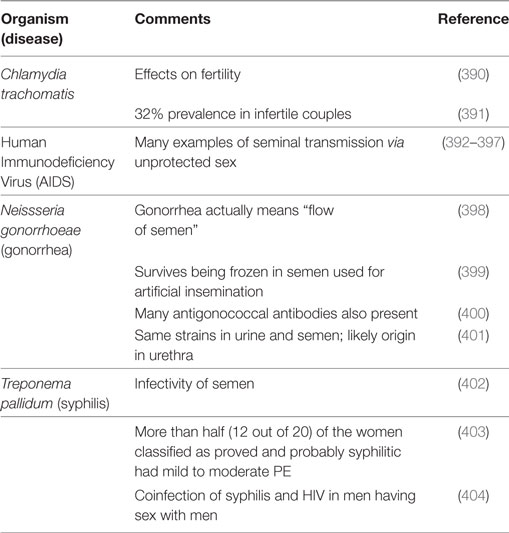
Table 3. Organisms of well-known sexually transmitted diseases that have been associated with semen.
Notwithstanding the difficulties of measurement (405), there is, in particular, a considerable literature on fertility (406), since infertile males tend to donate sperm for assay in fertility clinics, and infection is a common cause of infertility [e.g., Ref. (384) and Table 4]. Note that “infertility” is not always an absolute term: pregnancies result in 27% of cases of treated “infertile” couples followed up after trying to conceive for 2 years, and with oligozoospermia as the primary cause of infertility (407). Most studies involve bacteria (bacteriospermia). Articles on this and other microbial properties of semen beyond STDs include those in Table 4.
We deliberately avoid discussing mechanisms in any real detail here, since our purpose is merely to show that semen is commonly infected with microbes, whose presence might well lead to PE. However, we were very struck by the ability of Escherichia coli and other organisms (440, 448, 471) actually to immobilize sperm [e.g., Ref. (472–475)]. As with amyloidogenic blood clotting (476, 477), bacterial LPS (156) may be a chief culprit (459). The Gram-positive equivalent, lipoteichoic acid (LTA), is just as potent in the fibrinogen-clotting amyloidogen assay (478), but while Gram-positives can also immobilize sperm (479, 480), the influence of purified LTA on sperm seems not to have been tested.
Another prediction from this analysis is that since infection is a significant cause of both infertility and PE [and it may account for 15% of infertile cases (384, 473)], we might expect to see some correlations between them. Although one might argue that anything seen as imperfect “background” health or subfecundity might impinge on the incidence of PE [such as endocrine disruption (481) or DNA damage of whatever cause (482)], the risk ratio for PE in couples whose infertility had an unknown basis was 5.61 (CI 3.3–9.3) in one study in Aberdeen (483) and 1.29 (CI 1.05–1.60) in another in Norway (484). Time to pregnancy in couples may be used (in part) as a surrogate for (in)fertility and is associated with a variety of poor pregnancy outcomes (485); in this case, the risk ratio for PE for TTP exceeding 6 months was 2.47 (CI 1.3–4.69) (486). Given the prevalence of infection in infertile sperm (Table 4), and the frequency of infertility [10% in the Danish study (485), which defined it as couples taking a year or more to conceive], it seems reasonable to suggest that microbiological testing of semen should be done on a more routine basis. It would also help to light up any relationships between the microbiological properties of sperm and the potentially causal consequence of increased PE risk.
We also note, as thoughtfully and importantly suggested by referee 1, that the microbes in the semen may already induce inflammation in the endometrium a few days before the conceptus implants. This may itself constitute a hostile “environment” that can contribute to the process of defective implantation, rather than working via the fetus itself.
More quantitatively, and importantly intellectually, if infection is seen as a major cause of PE, as we argue here, and it is known that infection is a cause of infertility, then one should suppose that infertility, and infertility caused by infection, should be at least as common, and probably more common than is PE, and this is the case, adding some considerable weight to the argument. Indeed, if PE was much more common than infertility or even infection, it would be much harder to argue that the latter was a major cause of the former. In European countries ~10–15% of couples are afflicted by infertility (384, 485), and in some 60% of cases infection or a male factor is implicated (384). In some countries, the frequency of male infertility is 13–15% http://bionumbers.hms.harvard.edu/bionumber.aspx?id=113483&ver=0 or higher (487), and the percentage of females with impaired fecundity has been given as 12.3% https://www.cdc.gov/nchs/fastats/infertility.htm. These kinds of numbers would imply that 6–9% of couples experience infection- or male-based infertility, and this exceeds the 3–5% incidence of PE.
In a similar vein, antibiotics, provided they can get through the relevant membranes (488–490), should also have benefits on sperm parameters or fertility if a lack of it is caused by infection, and this has indeed been observed [e.g., Ref. (436, 452, 491)].
Roles of the Prostate and Testes
In the previous review, we focused on the gut, periodontitis, and the urinary tract of the mother as the main source of organisms that might lead to PE. Here we focus on the male, specifically the prostate and the testes, given the evidence for how common infection is in semen. The main function of the prostate gland is to secrete prostate fluid, one of the components of semen. Thus, although it is unlikely that measurements have regularly been done to assess any relationship between this and any adverse effects of pregnancy, it was of interest to establish whether it too is likely to harbor microbes. Indeed, such “male accessory gland infection” is common (492–496). In some cases, the origin is probably periodontal (497). Recent studies have implicated microbial PRRs, especially TLRs, as well as inflammatory cytokines and their signaling pathways, in testicular function, implying an important link between infection/inflammation and testicular dysfunction (498). The testes are a common and important site of infection in the male (499, 500), and even bacterial LPS can cause testitis (501). Similarly, infection (especially urinary tract infection) is a common cause of prostatitis (502–512). Finally, prostatitis is also a major cause of infertility (492, 493, 495). Such data contribute strongly to the recognition that semen is not normally going to be sterile, consistent with the view that it is likely to be a major originating cause of the infections characteristic of PE.
Microbial Infections in Spontaneous Abortions, Miscarriages, and PTB
Our logic would also imply a role for (potentially male-derived) microbes in miscarriages and spontaneous abortions. A microbial component to these seems well established for both miscarriages (513–515) and spontaneous abortions (516–521). Of course the ability of Brucella abortus to induce abortions in domesticated livestock, especially cattle (and occasionally in humans), is well known (522–524); indeed, bacteriospermia is inimical to fertilization success (525), and nowadays it is well controlled in livestock by the use of vaccines (526) or antimicrobials (525). Indeed, stored semen is so widely used for the artificial insemination of livestock in modern agriculture that the recognition that semen is not sterile has led to the routine use of antibiotics in semen “extenders” [e.g., Ref. (527–530)].
The same general logic is true for infection as a common precursor to PTB in the absence of PE, where it is much better established [e.g., Ref. (531–565)]. It arguably has the same basic origins in semen.
Although recurrent pregnancy loss is usually treated separately from infertility (where the role of infection is reasonably well established) it is possible that in many cases it is, like PE, partly just a worsened form of an immune reaction, with both sharing similar causes (including the microbial infection of semen). There is in fact considerable evidence for this [e.g., Ref. (138, 443, 566–580)]. Of course it is not unreasonable that poor sperm quality, that may be just sufficient to initiate a pregnancy, may ultimately contribute to its premature termination or other disorders of pregnancy, so this association might really be expected. It does, however, add considerable weight to the view that a more common screening of the male than presently done might be of value (581) in assessing a range of pregnancy disorders besides PE. In particular, it seems that infection affects motility (see above), and that this in turn is well correlated (573) with sperm DNA fragmentation and ultimate loss of reproductive quality.
Amyloids in semen are known to enhance human immunodeficiency virus infectivity (582). According to our own recent experimental analyzes, they may be caused by bacterial LPS (476, 477) or LTA (478). We note too that the sperm metabolome also influences offspring, e.g., from obese parents (583), and that many other variables are related to sperm quality, including oxidative stress (584–591). Thus it is entirely reasonable to see semen as a cause of problems as well as benefits to an ensuing pregnancy.
Microbial Effects on Immunotolerance
If our thesis is sound, one may expect to find evidence for the effects of microbes on the loss of immunotolerance in other settings. One such is tolerance to dietary antigens, of which gluten, a cause of celiac disease, is preeminent. Recently, evidence has come forward that shows a substantial effect of a reovirus in lowering the immunotolerance to gluten in a mouse model of celiac disease, and thereby causing inflammation (592, 593). Interestingly, pregnancies in women with celiac disease were considerably more susceptible to PTB and other complications than were controls (594–601), especially when mothers were not on a gluten-free diet. Similarly, preeclamptic pregnancies led to a much (4-fold) higher likelihood of allergic sensitization in the offspring (602) The roles of hygiene, the microbiome and disease are a matter of considerable current interest [e.g., Ref. (603)].
It was consequently logical to see if intolerance to peanut antigen was also predictive of PE, but we could find no evidence for this. Again, however, in a study (604) in which PE had roughly its normal prevalence, mothers experiencing it were significantly more likely to give birth to children with increased risk of asthma, eczema, and aeroallergen and food allergy.
Effects of Vaccination on Pregnancy Outcomes, Including PE
We noted above (and again below) that the evidence for a role of microbes in PTB is overwhelming [also reviewed in Ref. (44)]. From an immunological point of view, there seems to be a hugely beneficial outcome of vaccination against influenza in terms of lowering PTB (605–610) [cf. (611)] or stillbirth (612). PE was not studied, save in Ref. (613) where the risk ratio of vaccination (0.484, CI 0.18–1.34) implied a marginal benefit. There do not seem to be any safety issues, either for influenza vaccine (612–633) or for other vaccines (625) such as those against pertussis (634–636) or human papillomavirus (637).
As well as miscarriage and PTB, other adverse pregnancy outcomes studied in relation to vaccine exposure (638) include IUGR. IUGR frequently presents as the fetal phenotype of PE, sharing a common etiology in terms of poor placentation in early pregnancy (639). These other adverse events have been scored more frequently than has been PE, and Table 5 summarizes the evidence for a protective effect of vaccines, though it is recognized that there is the potential for considerable confounding effects [e.g., Ref. (632, 640)]. While Table 5 does not have examples from PE this is because the tests have seemingly not been done; because the effects on related disorders of pregnancy are clear, we think these should be sufficient to encourage people to look at the effects on PE (indeed readers may already have unpublished data).
There are no apparent benefits of vaccine-based immunization vs. recurrent miscarriage (642, 643).
Unrelated to the present question, but very interesting, is the fact that the risk of RA for men was higher among men who fathered their first child at a young age (P for trend <0.001) (644). This is consistent with the fact that its prevalence in females is 3.5 times higher, and that it has a microbial origin (645–648).
General or Specific?
The fact that vaccination against organisms not usually associated with adverse pregnancy outcomes is protective can be interpreted in one (or both) of two ways, i.e., that the vaccine is unselective in terms of inhibiting the effects of its target organism, or the generally raised level of <some kind of> immune response is itself protective. Data to discriminate these are not yet to hand.
In a similar vein, the survival of the host in any “battle” between host and parasite (e.g., microbe) can be effected in one or both of two main ways: (i) the host invokes antimicrobial processes such as the immune systems described above, or produces antimicrobial compounds or (ii) the host modifies itself in ways that allow it to become tolerant to the presence of a certain standing crop of microbes. We consider each in turn.
Antimicrobial Components of Human Semen, a Part of Resistance in the Semen Microbiome
Antimicrobial peptides (AMPs) [http://aps.unmc.edu/AP/main.php (649)] are a well-known part of the defense systems of many animals [e.g., Ref. (650–659)] [and indeed plants (650, 660)], and are widely touted as potential anti-infectives [e.g., Ref. (661–663)]. Their presence in the cells and tissues of the uterus, fetus and the neonate indicates an important role in immunity during pregnancy and in early life (657, 664–668). Unsurprisingly, they have been proposed as agents for use in preventing the transmission of STDs (669, 670), and as antimicrobials for addition to stored semen for use in agriculture (671–675). Our interest here, however, is around whether there are natural AMPs in human (or animal) semen, and the answer is in the affirmative. They include secretory leukocyte protease inhibitor (659), semen-derived enhancer of viral infection (676), and in particular the semenogelins (677, 678). HE2 is another AMP that resides in the epididymis (679, 680), while the human cathelicidin hCAP-18 [cathelicidin AMP, 18 kDa)] is inactive in seminal plasma but is processed to the AMP LL-37 by the prostate-derived protease gastricsin (668, 681). Thus it is clear that at least some of the reason that the semen microbiome is not completely unchecked is down to AMPs. Stimulating their production, provided they are not also spermicidal, would seem like an excellent therapeutic option.
Host Tolerance to Microbial Pathogens
It is a commonplace that—for any number of systems biology reasons based on biochemical individuality (682)—even highly virulent diseases do not kill everyone who is exposed to them at the same level. As indicated above, this could be because the host is resistant and simply clears the infections; this is certainly the more traditional view. However, an additional or alternative contribution is because while hosts do not clear all of them they can develop “tolerance” to them. This latter view is gaining considerable ground, not least since the work of Schneider, Ayres et al. (683) showing that a variety of Drosophila mutants with known genetic defects could differentially tolerate infection by Listeria monocytogenes. This concept of tolerance (684–691) is very important to our considerations here, since it means that we do indeed have well-established methods of putting up with microbes more generally, without killing them. It is consistent with clearly established evolutionary theory (692–694), and the relative importance of resistance and tolerance within a population affects host–microbe coevolution (695). The concept of tolerance sits easily with the Matzinger model of danger/damage [e.g., Ref. (175, 177, 178, 180)], as well as the concept of a resident population of dormant microbes (45, 47, 48), and may indeed be seen in terms of a coevolution or mutualistic association (696, 697). Some specific mechanisms are becoming established, e.g., the variation by microbes of their danger signal to promote host defense (698). Others, such as the difference in the host metabolomes [that we reviewed (44)] as induced by resistance vs. tolerance responses (690) may allow one to infer the relative importance of each. At all events, it is clear from the concept of dormancy that we do not kill all the intracellular microbes that our bodies harbor, and that almost by definition we must then tolerate them. As well as the established maternal immunotolerance of pregnancy, tolerance of microbes seems to be another hallmark of pregnancy.
Sequelae of a Role of Infection in PE: Microbes, Molecules and Processes
The chief line taken in our previous review (44) and herein is that this should be detectable by various means. Those three chief means involve detecting the microbes themselves, detecting molecules whose concentration changes as a result of the microbes (and their inflammatory components) being present, and detecting host processes whose activities have been changed by the presence of the microbes.
Previously (44), updated here (Table 2), we provided considerable evidence for the presence of microbes within the mother as part of PE. Here we have adduced the equally considerable evidence that in many cases semen is very far from being sterile, and that the source of the originating infection may actually be the father. Equally, we showed (44) that a long list of proteins that were raised (or less commonly lowered) in PE were equally changed by known infections, consistent with the view that PE also involved such infections, albeit at a lower level at which their overt presence could be kept in check. One protein we did not discuss was Placental Protein 13 (PP13) or galectin 1, so we now discuss this briefly.
PP13 (Galectin 13)
Galectins are glycan-binding proteins that regulate innate and adaptive immune responses. Three of the five human cluster galectins are solely expressed in the placenta (699). One of these, encoded by the LGALS13 gene (700, 701), is known as galectin-13 or PP13 (702). Its β-sheet-rich “jelly-roll” structure places it strongly as a galectin homolog (701). It has a MW of ~16 kDa [32 kDa dimer (703)] and is expressed solely in the placenta (700, 704) (and see http://www.proteinatlas.org/ENSG00000105198-LGALS13/tissue). A decreased placental expression of PP13 and its low concentrations in first trimester maternal sera are associated with elevated risk of PE (699, 705–707), plausibly reflecting poor placentation. By contrast, and consistent with the usual oxidative stress, there is increased trophoblastic shedding of PP13-immunopositive microvesicles in PE, starting in the second trimester, which leads to high maternal blood PP13 concentrations (699, 708). Certain alleles such as promoter variant 98A-C predispose strongly to PE (709).
Galectin-1 is also highly overexpressed in PE (710). However, as with all the other proteomic biomarkers surveyed previously (44), galectins (including galectin-13 #http://amp.pharm.mssm.edu/Harmonizome/gene/LGALS13) are clear biomarkers of infection (711).
Toll-Like Receptors
Toll-like receptors are among the best known receptors for “DAMPs” such as LPS from Gram-negatives [TLR4 (156, 712–714)], LTAs from Gram-positives [TLR2 (715–726)] and viral DNA and its mimics (TLR3) (727). Note, however, that TLRs are not expressed solely at the cell surface, and that pathogens (and their DNA) may also be recognized intracellularly (728–733), often via a pathway involving an AIM2 (“absent in melanoma 2”) inflammasome and or STING (“stimulator of interferon genes”).
As expected, they are intimately involved in disorders of pregnancy such as PE (185, 727, 734–745). Indeed the animal model for PE developed by Faas et al. (746) actually involves injecting an ultralow dose of LPS into pregnant rat on day 14 of gestation. Overall, such data are fully consistent with the view that infection is a significant part of PE. In view of our suggestions surrounding the role of semen infection in PE it would be of interest to know if these markers were also raised in the semen of partners of women who later manifest PE. Sperm cells are well endowed with TLRs (498, 747–749). However, we can find only one study showing that increased semen expression of TLRs is indeed observed during inflammation and oxidative stress such as occurs during infection and infertility (750). A more wide-ranging assessment of TLR expression in sperm cells as a function of fertility seems warranted.
LPS Mimics
An interesting and striking feature of PE is the common appearance (2–7 weeks before the onset of clinical disease) of inositolphosphoglycan-P type (IPG-P) in the urine of patients destined to manifest PE (20, 751–762). These molecules are second messegers of insulin, and hence related to gestational diabetes. Robillard et al. (20) comment “These carbohydrate–lipid long-chain molecules mimic exactly endotoxins (such as E. coli or Plasmodium falciparum membranes). In theory, these compounds could circulate as endotoxins floating around in the bloodstream for weeks (before and during the appearance of clinical signs of PE). Would these greatly augment the systemic and more specific endothelial inflammation in the mother? This area needs urgent further research as anti-IPG-Pdrugs (or others, monoclonal antibodies, etc.) are intellectually conceivable.” In view of the arguments raised here about the role of other endotoxins such as LPS, we consider these observations as providing potentially significant clues. Surprisingly, little is known of changes in their levels that might accompany genuine infection.
Coagulopathies
Although we discussed this in the previous review (44), some further brief rehearsal is warranted, since coagulopathies are such a common feature of PE (44). Specifically, our finding that very low concentrations of cell wall products can induce amyloid formation during blood clotting (476, 478) has been further extended to recognize the ubiquity of the phenomenon in chronic, inflammatory diseases (477, 478, 648, 763–766). Often, an extreme example gives strong pointers, and the syndrome with the highest likelihood of developing PE is antiphospholipid syndrome (APS) (767–771), which is also caused by infection (772–777) and where the coagulopathies are also especially noteworthy (778–782). Consequently, the recognition of PE as an amyloidogenic coagulopathy (44, 783–785) is significant.
APS and Cardiolipin
Antiphospholipid syndrome is an autoimmune disorder defined in particular by the presence high circulating titers of what are referred to as antiphospholipid antibodies (aPL) [e.g., Ref. (786)]. Given that every human cell’s plasma membrane contains phospholipids, one might wonder how “antiphospholipid antibodies” do not simply attack every cell. The answer, most interestingly, is that, despite the name, anticardiolipin antibodies, anti-β2-glycoprotein-I, and lupus anticoagulant are the main autoantibodies found in APS (787).
In contrast to common phospholipids such as phosphatidylcholine, phosphatidylserine, and phosphatidylethanolamine, cardiolipins [1,3-bis(sn-3′-phosphatidyl)-sn-glycerol derivatives] (see Figure 6 for some structures) are synthesized in (Ref. (788–790)) and essentially confined to mitochondria, and in particular the inner mitochondrial membrane. While heart failure is a separate clinical condition, we note that such phospholipids can serve important functions in oxidative phosphorylation, apoptosis, and heart failure development (790–797).
Overall, there seems to be little doubt that APS and aPL are the result of infection (773–777, 798–800), and that, as with RA (645–648, 801), the autoimmune responses are essentially due to molecular mimicry.
Now, of course, from an evolutionary point of view, mitochondria are considered to have evolved from (α-proteo)bacteria (802–808) that were engulfed by a protoeukaryote (809), and bacteria might consequently be expected to possess cardiolipin. This is very much the case for both Gram-negative and Gram-positive strains (810–814), with Gram-positive organisms typically having the greater content. Particularly significant, from our point of view, is that the relative content of cardiolipin among phospholipids increases enormously as (at least Gram-positive) bacterial cells become dormant (815).
Thus, the cardiolipin can come from two main sources: (i) host cell death that liberates mitochondrial products or (ii) invading bacteria (especially those that lay dormant and awaken). Serum ferritin is a cell death marker (816), and some evidence for the former source (817) [and see Ref. (818)] is that hyperferritinemia was present in 9% vs. 0% of APS patients and controls, respectively (P < 0.001), and that hyperferritinemia was present in 71% of catastrophic APS (cAPS) patients, and ferritin levels among this subgroup were significantly higher compared with APS-non-cAPS patients (816–847 vs. 120–230 ng/ml, P < 0.001). One easy hypothesis is that both are due to invading bacteria, but cAPS patients also exhibit comparatively large amounts of host cell death (Figure 7).
Treatment Options Based on (Or Consistent with) the Ideas Presented Here
Although often unwritten or implicit, the purposes of much of fundamental biomedical science are to find better diagnostics and treatments for diseases (a combination sometimes referred to as theranostics). Consequently, our purposes here are to rehearse some of those areas where appropriate tests (in the form, ultimately, of randomized clinical trials) may be performed. Clearly, as before (44), and recognizing the issues of antimicrobial resistance, one avenue would exploit antibiotics much more commonly than now. We note that pharmaceutical drugs are prescribed or taken during 50% or more of pregnancies (819–828). Anti-infectives are the most common such drugs, and some 20–25% of women or more are prescribed one or more antibiotics during their pregnancies (820, 821, 824, 826, 828–832).
Given the role of male semen infection, we suggest that more common testing of semen for infection is warranted, especially using molecular tests. Our analyses suggest that antibiotics might also be of benefit to those males presenting with high microbial semen loads or poor fertility (833). Another strategy might involve stimulating the production of AMPs in semen.
Of the list of bacteria given in Table 2 as being associated with PE, H. pylori stands out as the most frequent. One may wonder why a vaccine against it has not been developed, but it seems to be less straightforward than for other infections (834, 835), probably because—consistent with its ability to persist within its hosts—it elicits only a poor immune response (836, 837). Our own experience (838) is that many small molecules can improve the ability of other agents to increase the primary mechanisms that are the target assay, while having no direct effects on them themselves. Although “combinatorial” strategies often lead to quite unexpected beneficial effects [e.g., Ref. (839, 840)], this “binary weapon” strategy is both novel and untried.
As also rehearsed in more detail previously [e.g., Ref. (841, 842)] many polyphenolic antioxidants act through their ability to chelate unliganded iron, and thereby keep it from doing damage or acting as a source of iron for microbial proliferation. Such molecules may also be expected to be beneficial. Other strategies may be useful for inhibiting the downstream sequelae of latent infections, such as targeting inflammation or coagulopathies.
Conclusion, Summary, and Open Questions
We consider that our previous review (44) made a very convincing case for the role of (mostly dormant) microbes in the etiology of PE. However, we there paid relatively scant attention to two elements, viz (i) the importance of the immune system (164), especially in maternal immunotolerance and (ii) the idea that possibly the commonest cause of the microbes providing the initial infection was actually infected semen from the father. We also recognize that epigenetic information (389, 843–845) can be provided by the father and this can be hard to discriminate from infection (if not measured), at least in the F1 generation. This said, microbiological testing of semen seems to be a key discriminator if applied. The “danger model” (175, 177–180), in which it is recognized that immune activation owes more to the detection of specific damage signals than to “non-self,” thus seems to be highly relevant to PE (182).
Overall, we think the most important ideas and facts that we have rehearsed here include the following:
• Following Medawar’s recognition of the potential conundrum of paternal alloantigens in pregnancy, most thinking has focused on the role of maternal immunotolerance, and the role of Tregs therein.
• Many examples show that sexual familiarity with the father helps protect against PE; however, this does not explain why in many cases exposure to paternal antigens is actually protective (and not even merely neutral).
• Semen contains many protective and immune-tolerance-inducing substances such as TGF-β.
• However, semen is rarely sterile, and contains many microbes, some of which are not at all benign, and can be transferred to the mother during copulation.
• If one accepts that there is often a microbial component to the development of PE, and we and others have rehearsed the considerable evidence that it is so, then semen seems to a substantial, and previous largely unconsidered source of microbes.
• Some determinands, such as complement factor Bb, seem to reflect microbial infection and not just general inflammation that can have many other causes, and may therefore be of value in untangling the mechanisms involved.
• An improved understanding of the microbiology of semen, and the role of antibiotics and vaccination in the father, seems particularly worthwhile; novel antioxidants may also hold promise (846–848).
• Coagulopathies are a somewhat underappreciated accompaniment to PE and may contribute to its etiology.
• The “danger model” of immune response seems much better suited to describing events in pregnancy and PE than is the classical self/non-self analysis.
• The features of PE are not at all well recapitulated in animal models (26), and certainly not in rodents. However, it seems likely that they still have much to contribute (849–851).
Open questions and further research agenda items include the following:
• There is a need for improved molecular and culture-based methods of detecting microbes in blood and tissues in which they are normally considered to be absent, both in the mother and the father.
• Notwithstanding the promise of metabolomics [see e.g., Ref. (852, 853)], there remains a need for better diagnostics, especially early in pregnancy.
• Issues of antimicrobial resistance are well known [e.g., Ref. (854–856)], and most antibiotics work only on growing cells, so there is a significant role for those that work on persisters and other non-replicating forms (857–859).
• As increasing numbers of infectious diseases are seen to be associated with diseases previously considered noncommunicable [e.g., tuberculosis and Parkinson’s disease (860–862)], we may anticipate more careful study of such an association between overt infection and PE.
• In these discussions, we have largely avoided discriminating between early-onset (<34 weeks) and late-onset (>34 weeks) PE, but recognize both the distinctions and their varying prevalences (20, 863–867).
• The increasing online availability of patient information will permit greater exploitation to assess these ideas from an epidemiological point of view; in this sense, an improved understanding of the basis for the widely varying geographical incidence of PE (20) is also likely to offer important clues.
Author Contributions
In discussion, DK and LK jointly came up with the original idea for the role of semen in preeclampsia, and the many sequelae it entails, and during many subsequent discussions wrote the review.
Conflict of Interest Statement
The authors declare that the research was conducted in the absence of any commercial or financial relationships that could be construed as a potential conflict of interest.
Funding
DK thanks the Biotechnology and Biological Sciences Research Council (grant BB/L025752/1) for financial support. LK is a Science Foundation Ireland Principal Investigator (grant number 08/IN.1/B2083). LK is also the Director of the Science Foundation Ireland-funded INFANT Research Centre (grant no. 12/RC/2272). The funders had no other role in the conception or submission of the manuscript, and have no conflicts of interest to declare.
References
1. Jeffcoate TNA. Pre-eclampsia and eclampsia: the disease of theories. Proc R Soc Med (1966) 59:397–404.
2. Barad DH, Kushnir VA, Gleicher N. Focus on recurrent miscarriage phenotypes. Fertil Steril (2017) 107:64–5. doi:10.1016/j.fertnstert.2016.10.034
3. Grill S, Rusterholz C, Zanetti-Dällenbach R, Tercanli S, Holzgreve W, Hahn S, et al. Potential markers of preeclampsia – a review. Reprod Biol Endocrinol (2009) 7:70. doi:10.1186/1477-7827-7-70
4. Steegers EAP, Von Dadelszen P, Duvekot JJ, Pijnenborg R. Pre-eclampsia. Lancet (2010) 376:631–44. doi:10.1016/S0140-6736(10)60279-6
5. North RA, Mccowan LM, Dekker GA, Poston L, Chan EH, Stewart AW, et al. Clinical risk prediction for pre-eclampsia in nulliparous women: development of model in international prospective cohort. BMJ (2011) 342:d1875. doi:10.1136/bmj.d1875
6. Uzan J, Carbonnel M, Piconne O, Asmar R, Ayoubi JM. Pre-eclampsia: pathophysiology, diagnosis, and management. Vasc Health Risk Manag (2011) 7:467–74. doi:10.2147/VHRM.S20181
7. Kenny L. Improving diagnosis and clinical management of pre-eclampsia. MLO Med Lab Obs (2012) 44(12):14.
9. Chaiworapongsa T, Chaemsaithong P, Yeo L, Romero R. Pre-eclampsia part 1: current understanding of its pathophysiology. Nat Rev Nephrol (2014) 10:466–80. doi:10.1038/nrneph.2014.102
10. Kenny LC, Black MA, Poston L, Taylor R, Myers JE, Baker PN, et al. Early pregnancy prediction of preeclampsia in nulliparous women, combining clinical risk and biomarkers: the Screening for Pregnancy Endpoints (SCOPE) international cohort study. Hypertension (2014) 64:644–52. doi:10.1161/HYPERTENSIONAHA.114.03578
11. Sircar M, Thadhani R, Karumanchi SA. Pathogenesis of preeclampsia. Curr Opin Nephrol Hypertens (2015) 24:131–8. doi:10.1097/MNH.0000000000000105
12. Abalos E, Cuesta C, Grosso AL, Chou D, Say L. Global and regional estimates of preeclampsia and eclampsia: a systematic review. Eur J Obstet Gynecol Reprod Biol (2013) 170:1–7. doi:10.1016/j.ejogrb.2013.05.005
13. Vest AR, Cho LS. Hypertension in pregnancy. Curr Atheroscler Rep (2014) 16:395. doi:10.1007/s11883-013-0395-8
14. Khan KS, Wojdyla D, Say L, Gulmezoglu AM, Van Look PF. WHO analysis of causes of maternal death: a systematic review. Lancet (2006) 367:1066–74. doi:10.1016/S0140-6736(06)68397-9
15. Duley L. The global impact of pre-eclampsia and eclampsia. Semin Perinatol (2009) 33:130–7. doi:10.1053/j.semperi.2009.02.010
16. Cantwell R, Clutton-Brock T, Cooper G, Dawson A, Drife J, Garrod D, et al. Saving mothers’ lives: reviewing maternal deaths to make motherhood safer: 2006–2008. The eighth report of the confidential enquiries into maternal deaths in the United Kingdom. BJOG (2011) 118(Suppl 1):1–203. doi:10.1111/j.1471-0528.2010.02847.x
17. Ghulmiyyah L, Sibai B. Maternal mortality from preeclampsia/eclampsia. Semin Perinatol (2012) 36:56–9. doi:10.1053/j.semperi.2011.09.011
18. Abalos E, Cuesta C, Carroli G, Qureshi Z, Widmer M, Vogel JP, et al. Pre-eclampsia, eclampsia and adverse maternal and perinatal outcomes: a secondary analysis of the World Health Organization multicountry survey on maternal and newborn health. BJOG (2014) 121(Suppl 1):14–24. doi:10.1111/1471-0528.12629
19. Robillard PY, Dekker G, Chaouat G, Hulsey TC, Saftlas A. Epidemiological studies on primipaternity and immunology in preeclampsia – a statement after twelve years of workshops. J Reprod Immunol (2011) 89:104–17. doi:10.1016/j.jri.2011.02.003
20. Robillard PY, Dekker G, Iacobelli S, Chaouat G. An essay of reflection: why does preeclampsia exist in humans, and why are there such huge geographical differences in epidemiology? J Reprod Immunol (2016) 114:44–7. doi:10.1016/j.jri.2015.07.001
21. Brew O, Sullivan MH, Woodman A. Comparison of normal and pre-eclamptic placental gene expression: a systematic review with meta-analysis. PLoS One (2016) 11:e0161504. doi:10.1371/journal.pone.0161504
22. Leavey K, Benton SJ, Grynspan D, Kingdom JC, Bainbridge SA, Cox BJ. Unsupervised placental gene expression profiling identifies clinically relevant subclasses of human preeclampsia. Hypertension (2016) 68:137–47. doi:10.1161/HYPERTENSIONAHA.116.07293
23. Redman CWG. Current topic: pre-eclampsia and the placenta. Placenta (1991) 12:301–8. doi:10.1016/0143-4004(91)90339-H
24. Roberts JM, Hubel CA. The two stage model of preeclampsia: variations on the theme. Placenta (2009) 30(Suppl A):S32–7. doi:10.1016/j.placenta.2008.11.009
26. Pennington KA, Schlitt JM, Jackson DL, Schulz LC, Schust DJ. Preeclampsia: multiple approaches for a multifactorial disease. Dis Model Mech (2012) 5:9–18. doi:10.1242/dmm.008516
27. Redman CW, Sargent IL, Staff AC. IFPA senior award lecture: making sense of pre-eclampsia – two placental causes of preeclampsia? Placenta (2014) 35(Suppl):S20–5. doi:10.1016/j.placenta.2013.12.008
28. Redman CWG. The six stages of pre-eclampsia. Pregnancy Hypertens (2014) 4:246. doi:10.1016/j.preghy.2014.04.020
29. Perucci LO, Corrêa MD, Dusse LM, Gomes KB, Sousa LP. Resolution of inflammation pathways in preeclampsia-a narrative review. Immunol Res (2017) 65:774–89. doi:10.1007/s12026-017-8921-3
30. Hung TH, Skepper JN, Burton GJ. In vitro ischemia-reperfusion injury in term human placenta as a model for oxidative stress in pathological pregnancies. Am J Pathol (2001) 159:1031–43. doi:10.1016/S0002-9440(10)61778-6
31. Burton GJ, Jauniaux E. Placental oxidative stress: from miscarriage to preeclampsia. J Soc Gynecol Investig (2004) 11:342–52. doi:10.1016/j.jsgi.2004.03.003
32. Burdon C, Mann C, Cindrova-Davies T, Ferguson-Smith AC, Burton GJ. Oxidative stress and the induction of cyclooxygenase enzymes and apoptosis in the murine placenta. Placenta (2007) 28:724–33. doi:10.1016/j.placenta.2006.12.001
33. Cindrova-Davies T, Spasic-Boskovic O, Jauniaux E, Charnock-Jones DS, Burton GJ. Nuclear factor-kappa B, p38, and stress-activated protein kinase mitogen-activated protein kinase signaling pathways regulate proinflammatory cytokines and apoptosis in human placental explants in response to oxidative stress: effects of antioxidant vitamins. Am J Pathol (2007) 170:1511–20. doi:10.2353/ajpath.2007.061035
34. Burton GJ, Yung HW, Cindrova-Davies T, Charnock-Jones DS. Placental endoplasmic reticulum stress and oxidative stress in the pathophysiology of unexplained intrauterine growth restriction and early onset preeclampsia. Placenta (2009) 30(Suppl A):S43–8. doi:10.1016/j.placenta.2008.11.003
35. Burton GJ, Jauniaux E. Oxidative stress. Best Pract Res Clin Endocrinol Metab (2011) 25:287–99. doi:10.1016/j.bpobgyn.2010.10.016
36. Sánchez-Aranguren LC, Prada CE, Riaño-Medina CE, Lopez M. Endothelial dysfunction and preeclampsia: role of oxidative stress. Front Physiol (2014) 5:1. doi:10.3389/fphys.2014.00372
37. Broughton Pipkin F, Rubin PC. Pre-eclampsia – the ‘disease of theories’. Br Med Bull (1994) 50:381–96. doi:10.1093/oxfordjournals.bmb.a072898
38. Schlembach D. Pre-eclampsia – still a disease of theories. Fukushima J Med Sci (2003) 49:69–115. doi:10.5387/fms.49.69
39. George EM. The disease of theories: unravelling the mechanisms of pre-eclampsia. Biochemist (2017) 39:22–5.
40. Hubel CA. Oxidative stress in the pathogenesis of preeclampsia. Proc Soc Exp Biol Med (1999) 222:222–35. doi:10.1046/j.1525-1373.1999.d01-139.x
41. George EM. New approaches for managing preeclampsia: clues from clinical and basic research. Clin Ther (2014) 36:1873–81. doi:10.1016/j.clinthera.2014.09.023
42. Wu P, Kwok CS, Haththotuwa R, Kotronias RA, Babu A, Fryer AA, et al. Pre-eclampsia is associated with a twofold increase in diabetes: a systematic review and meta-analysis. Diabetologia (2016) 59:2518–26. doi:10.1007/s00125-016-4098-x
43. Wu P, Haththotuwa R, Kwok CS, Babu A, Kotronias RA, Rushton C, et al. Preeclampsia and future cardiovascular health: a systematic review and meta-analysis. Circ Cardiovasc Qual Outcomes (2017) 10:e003497. doi:10.1161/CIRCOUTCOMES.116.003497
44. Kell DB, Kenny LC. A dormant microbial component in the development of pre-eclampsia. Front Med Obs Gynecol (2016) 3:60. doi:10.3389/fmed.2016.00060
45. Kaprelyants AS, Gottschal JC, Kell DB. Dormancy in non-sporulating bacteria. FEMS Microbiol Rev (1993) 10:271–86. doi:10.1111/j.1574-6968.1993.tb05871.x
46. Kell DB, Kaprelyants AS, Weichart DH, Harwood CL, Barer MR. Viability and activity in readily culturable bacteria: a review and discussion of the practical issues. Antonie Van Leeuwenhoek (1998) 73:169–87. doi:10.1023/A:1000664013047
47. Kell DB, Potgieter M, Pretorius E. Individuality, phenotypic differentiation, dormancy and ‘persistence’ in culturable bacterial systems: commonalities shared by environmental, laboratory, and clinical microbiology. F1000Research (2015) 4:179. doi:10.12688/f1000research.6709.1
48. Potgieter M, Bester J, Kell DB, Pretorius E. The dormant blood microbiome in chronic, inflammatory diseases. FEMS Microbiol Rev (2015) 39:567–91. doi:10.1093/femsre/fuv013
49. Herrera JA, Chaudhuri G, López-Jaramillo P. Is infection a major risk factor for preeclampsia? Med Hypotheses (2001) 57:393–7. doi:10.1054/mehy.2001.1378
50. Trogstad LIS, Eskild A, Bruu AL, Jeansson S, Jenum PA. Is preeclampsia an infectious disease? Acta Obstet Gynecol Scand (2001) 80:1036–8. doi:10.1034/j.1600-0412.2001.801112.x
51. Todros T, Vasario E, Cardaropoli S. Preeclampsia as an infectious disease. Exp Rev Obs Gynecol (2007) 2:735–41. doi:10.1586/17474108.2.6.735
52. Conde-Agudelo A, Villar J, Lindheimer M. Maternal infection and risk of preeclampsia: systematic review and metaanalysis. Am J Obstet Gynecol (2008) 198:7–22. doi:10.1016/j.ajog.2007.07.040
53. López-Jaramillo P, Herrera JA, Arenas-Mantilla M, Jauregui IE, Mendoza MA. Subclinical infection as a cause of inflammation in preeclampsia. Am J Ther (2008) 15:373–6. doi:10.1097/MJT.0b013e318164c149
54. Rustveld LO, Kelsey SF, Sharma R. Association between maternal infections and preeclampsia: a systematic review of epidemiologic studies. Matern Child Health J (2008) 12:223–42. doi:10.1007/s10995-007-0224-1
55. Di Simone N, Tersigni C, Cardaropoli S, Franceschi F, Di Nicuolo F, Castellani R, et al. Helicobacter pylori infection contributes to placental impairment in preeclampsia: basic and clinical evidences. Helicobacter (2017) 22:e12347. doi:10.1111/hel.12347
56. Nourollahpour Shiadeh M, Behboodi Moghadam Z, Adam I, Saber V, Bagheri M, Rostami A. Human infectious diseases and risk of preeclampsia: an updated review of the literature. Infection (2017) 45:589–600. doi:10.1007/s15010-017-1031-2
57. Kleinrouweler CE, Van Uitert M, Moerland PD, Ris-Stalpers C, Van Der Post JA, Afink GB. Differentially expressed genes in the pre-eclamptic placenta: a systematic review and meta-analysis. PLoS One (2013) 8:e68991. doi:10.1371/journal.pone.0068991
58. Chung MC, Jorgensen SC, Popova TG, Tonry JH, Bailey CL, Popov SG. Activation of plasminogen activator inhibitor implicates protease InhA in the acute-phase response to Bacillus anthracis infection. J Med Microbiol (2009) 58:737–44. doi:10.1099/jmm.0.007427-0
59. Ubagai T, Tansho S, Ieki R, Ono Y. Evaluation of TREM1 gene expression in circulating polymorphonuclear leukocytes and its inverse correlation with the severity of pathophysiological conditions in patients with acute bacterial infections. Jpn J Infect Dis (2012) 65:376–82. doi:10.7883/yoken.65.376
60. Ubagai T, Nakano R, Kikuchi H, Ono Y. Gene expression analysis of TREM1 and GRK2 in polymorphonuclear leukocytes as the surrogate biomarkers of acute bacterial infections. Int J Med Sci (2014) 11:215–21. doi:10.7150/ijms.7231
61. Fillerova R, Gallo J, Radvansky M, Kraiczova V, Kudelka M, Kriegova E. Excellent diagnostic characteristics for ultrafast gene profiling of DEFA1-IL1B-LTF in detection of prosthetic joint infections. J Clin Microbiol (2017) 55:2686–97. doi:10.1128/JCM.00558-17
62. Dekker GA, Sibai BM. Etiology and pathogenesis of preeclampsia: current concepts. Am J Obstet Gynecol (1998) 179:1359–75. doi:10.1016/S0002-9378(98)70160-7
63. Sargent IL, Borzychowski AM, Redman CW. Immunoregulation in normal pregnancy and pre-eclampsia: an overview. Reprod Biomed Online (2006) 13:680–6. doi:10.1016/S1472-6483(10)60659-1
64. Dekker G, Robillard PY. Pre-eclampsia: is the immune maladaptation hypothesis still standing? An epidemiological update. J Reprod Immunol (2007) 76:8–16. doi:10.1016/j.jri.2007.03.015
65. Moffett A, Hiby SE. How does the maternal immune system contribute to the development of pre-eclampsia? Placenta (2007) 28(Suppl A):S51–6. doi:10.1016/j.placenta.2006.11.008
66. Cudihy D, Lee RV. The pathophysiology of pre-eclampsia: current clinical concepts. J Obstet Gynaecol (2009) 29:576–82. doi:10.1080/01443610903061751
67. James JL, Whitley GS, Cartwright JE. Pre-eclampsia: fitting together the placental, immune and cardiovascular pieces. J Pathol (2010) 221:363–78. doi:10.1002/path.2719
68. Jianjun Z, Yali H, Zhiqun W, Mingming Z, Xia Z. Imbalance of T-cell transcription factors contributes to the Th1 type immunity predominant in pre-eclampsia. Am J Reprod Immunol (2010) 63:38–45. doi:10.1111/j.1600-0897.2009.00763.x
69. Redman CWG, Sargent IL. Immunology of pre-eclampsia. Am J Reprod Immunol (2010) 63:534–43. doi:10.1111/j.1600-0897.2010.00831.x
70. Hsu P, Nanan RK. Innate and adaptive immune interactions at the fetal-maternal interface in healthy human pregnancy and pre-eclampsia. Front Immunol (2014) 5:125. doi:10.3389/fimmu.2014.00125
71. Dekker GA, Robillard PY, Hulsey TC. Immune maladaptation in the etiology of preeclampsia: a review of corroborative epidemiologic studies. Obstet Gynecol Surv (1998) 53:377–82. doi:10.1097/00006254-199806000-00023
73. Kenny LC, Kell DB. Immunological Tolerance, Pregnancy and Pre-Eclampsia: The Roles of Semen Microbes and the Father. bioRxiv Preprint. bioRxiv, 198796 (2017). doi:10.1101/198796
74. Medawar PB. Some immunological and endocrinological problems raised by the evolution of viviparity in vertebrates. Symp Soc Exp Biol (1953) 7:320–38.
75. Billington WD. The immunological problem of pregnancy: 50 years with the hope of progress. A tribute to Peter Medawar. J Reprod Immunol (2003) 60:1–11. doi:10.1016/S0165-0378(03)00083-4
76. Trowsdale J, Betz AG. Mother’s little helpers: mechanisms of maternal-fetal tolerance. Nat Immunol (2006) 7:241–6. doi:10.1038/ni1317
77. Munoz-Suano A, Hamilton AB, Betz AG. Gimme shelter: the immune system during pregnancy. Immunol Rev (2011) 241:20–38. doi:10.1111/j.1600-065X.2011.01002.x
78. Colucci F, Moffett A, Trowsdale J. Medawar and the immunological paradox of pregnancy: 60 years on. Eur J Immunol (2014) 44:1883–5. doi:10.1002/eji.201470065
79. Bonney EA. Immune regulation in pregnancy: a matter of perspective? Obstet Gynecol Clin North Am (2016) 43:679–98. doi:10.1016/j.ogc.2016.07.004
81. Robertson SA, Sharkey DJ. The role of semen in induction of maternal immune tolerance to pregnancy. Semin Immunol (2001) 13:243–54. doi:10.1006/smim.2000.0320
82. Clark GF, Schust DJ. Manifestations of immune tolerance in the human female reproductive tract. Front Immunol (2013) 4:26. doi:10.3389/fimmu.2013.00026
83. Gleicher N, Kushnir VA, Barad DH. Redirecting reproductive immunology research toward pregnancy as a period of temporary immune tolerance. J Assist Reprod Genet (2017) 34:425–30. doi:10.1007/s10815-017-0874-x
84. Moffett A, Loke C. Immunology of placentation in eutherian mammals. Nat Rev Immunol (2006) 6:584–94. doi:10.1038/nri1897
85. Rusterholz C, Hahn S, Holzgreve W. Role of placentally produced inflammatory and regulatory cytokines in pregnancy and the etiology of preeclampsia. Semin Immunopathol (2007) 29:151–62. doi:10.1007/s00281-007-0071-6
86. Dutta P, Burlingham WJ. Microchimerism: tolerance vs. sensitization. Curr Opin Organ Transplant (2011) 16:359–65. doi:10.1097/MOT.0b013e3283484b57
87. Rusterholz C, Messerli M, Hoesli I, Hahn S. Placental microparticles, DNA, and RNA in preeclampsia. Hypertens Pregnancy (2011) 30:364–75. doi:10.3109/10641951003599571
88. Kinder JM, Stelzer IA, Arck PC, Way SS. Immunological implications of pregnancy-induced microchimerism. Nat Rev Immunol (2017) 17:483–94. doi:10.1038/nri.2017.38
89. Knight M, Redman CWG, Linton EA, Sargent IL. Shedding of syncytiotrophoblast microvilli into the maternal circulation in pre-eclamptic pregnancies. Br J Obstet Gynaecol (1998) 105:632–40. doi:10.1111/j.1471-0528.1998.tb10178.x
90. Huppertz B, Kingdom J, Caniggia I, Desoye G, Black S, Korr H, et al. Hypoxia favours necrotic versus apoptotic shedding of placental syncytiotrophoblast into the maternal circulation. Placenta (2003) 24:181–90. doi:10.1053/plac.2002.0903
91. Sargent IL, Germain SJ, Sacks GP, Kumar S, Redman CWG. Trophoblast deportation and the maternal inflammatory response in pre-eclampsia. J Reprod Immunol (2003) 59:153–60. doi:10.1016/S0165-0378(03)00044-5
92. Gupta AK, Hasler P, Holzgreve W, Gebhardt S, Hahn S. Induction of neutrophil extracellular DNA lattices by placental microparticles and IL-8 and their presence in preeclampsia. Hum Immunol (2005) 66:1146–54. doi:10.1016/j.humimm.2005.11.003
93. Goswami D, Tannetta DS, Magee LA, Fuchisawa A, Redman CWG, Sargent IL, et al. Excess syncytiotrophoblast microparticle shedding is a feature of early-onset pre-eclampsia, but not normotensive intrauterine growth restriction. Placenta (2006) 27:56–61. doi:10.1016/j.placenta.2004.11.007
94. Reddy A, Zhong XY, Rusterholz C, Hahn S, Holzgreve W, Redman CWG, et al. The effect of labour and placental separation on the shedding of syncytiotrophoblast microparticles, cell-free DNA and mRNA in normal pregnancy and pre-eclampsia. Placenta (2008) 29:942–9. doi:10.1016/j.placenta.2008.08.018
95. Hartley JDR, Ferguson BJ, Moffett A. The role of shed placental DNA in the systemic inflammatory syndrome of preeclampsia. Am J Obstet Gynecol (2015) 213:268–77. doi:10.1016/j.ajog.2015.03.026
96. Clark DA, Chaput A, Tutton D. Active suppression of host-vs-graft reaction in pregnant mice. VII. Spontaneous abortion of allogeneic CBA/J x DBA/2 fetuses in the uterus of CBA/J mice correlates with deficient non-T suppressor cell activity. J Immunol (1986) 136:1668–75.
97. Mellor AL, Sivakumar J, Chandler P, Smith K, Molina H, Mao D, et al. Prevention of T cell-driven complement activation and inflammation by tryptophan catabolism during pregnancy. Nat Immunol (2001) 2:64–8. doi:10.1038/83183
98. Wilczyński JR. Immunological analogy between allograft rejection, recurrent abortion and pre-eclampsia – the same basic mechanism? Hum Immunol (2006) 67:492–511. doi:10.1016/j.humimm.2006.04.007
99. Warning JC, Mccracken SA, Morris JM. A balancing act: mechanisms by which the fetus avoids rejection by the maternal immune system. Reproduction (2011) 141:715–24. doi:10.1530/REP-10-0360
100. Kim CJ, Romero R, Chaemsaithong P, Kim JS. Chronic inflammation of the placenta: definition, classification, pathogenesis, and clinical significance. Am J Obstet Gynecol (2015) 213:S53–69. doi:10.1016/j.ajog.2015.08.041
101. Raman K, Wang H, Troncone MJ, Khan WI, Pare G, Terry J. Overlap chronic placental inflammation is associated with a unique gene expression pattern. PLoS One (2015) 10:e0133738. doi:10.1371/journal.pone.0133738
102. Hyde KJ, Schust DJ. Immunologic challenges of human reproduction: an evolving story. Fertil Steril (2016) 106:499–510. doi:10.1016/j.fertnstert.2016.07.1073
103. Zelante T, Pieraccini G, Scaringi L, Aversa F, Romani L. Learning from other diseases: protection and pathology in chronic fungal infections. Semin Immunopathol (2016) 38:239–48. doi:10.1007/s00281-015-0523-3
104. Mor G, Cardenas I, Abrahams V, Guller S. Inflammation and pregnancy: the role of the immune system at the implantation site. Ann N Y Acad Sci (2011) 1221:80–7. doi:10.1111/j.1749-6632.2010.05938.x
105. Racicot K, Kwon JY, Aldo P, Silasi M, Mor G. Understanding the complexity of the immune system during pregnancy. Am J Reprod Immunol (2014) 72:107–16. doi:10.1111/aji.12289
106. Mor G, Aldo P, Alvero AB. The unique immunological and microbial aspects of pregnancy. Nat Rev Immunol (2017) 17:469–82. doi:10.1038/nri.2017.64
107. Hench PS. The ameliorating effect of pregnancy on chronic atrophic (infectious rheumatoid) arthritis, fibrositis and intermittent hydrarthritis. Mayo Clin Proc (1938) 13:161–7.
108. Glyn J. The discovery and early use of cortisone. J R Soc Med (1998) 91:513–7. doi:10.1177/014107689809101004
109. de Man YA, Dolhain RJ, Van De Geijn FE, Willemsen SP, Hazes JM. Disease activity of rheumatoid arthritis during pregnancy: results from a nationwide prospective study. Arthritis Rheum (2008) 59:1241–8. doi:10.1002/art.24003
110. Muñoz-Valle JF, Vazquez-Del Mercado M, García-Iglesias T, Orozco-Barocio G, Bernard-Medina G, Martínez-Bonilla G, et al. T(H)1/T(H)2 cytokine profile, metalloprotease-9 activity and hormonal status in pregnant rheumatoid arthritis and systemic lupus erythematosus patients. Clin Exp Immunol (2003) 131:377–84. doi:10.1046/j.1365-2249.2003.02059.x
111. Nelson JL, Hughes KA, Smith AG, Nisperos BB, Branchaud AM, Hansen JA. Maternal-fetal disparity in HLA class II alloantigens and the pregnancy-induced amelioration of rheumatoid arthritis. N Engl J Med (1993) 329:466–71. doi:10.1056/NEJM199308123290704
112. Chiam NP, Lim LL. Uveitis and gender: the course of uveitis in pregnancy. J Ophthalmol (2014) 2014:401915. doi:10.1155/2014/401915
113. James D, Steer PL, Weiner C, Gonik B, Crowther C, Robson S, editors. High Risk Pregnancy. Amsterdam: Elsevier/Saunders (2010).
114. Khashan AS, Kenny LC, Laursen TM, Mahmood U, Mortensen PB, Henriksen TB, et al. Pregnancy and the risk of autoimmune disease. PLoS One (2011) 6:e19658. doi:10.1371/journal.pone.0019658
115. Hunt JS, Petroff MG, Mcintire RH, Ober C. HLA-G and immune tolerance in pregnancy. FASEB J (2005) 19:681–93. doi:10.1096/fj.04-2078rev
116. Hviid TV. HLA-G in human reproduction: aspects of genetics, function and pregnancy complications. Hum Reprod Update (2006) 12:209–32. doi:10.1093/humupd/dmi048
117. Guleria I, Sayegh MH. Maternal acceptance of the fetus: true human tolerance. J Immunol (2007) 178:3345–51. doi:10.4049/jimmunol.178.6.3345
118. Dahl M, Perin TL, Djurisic S, Rasmussen M, Ohlsson J, Buus S, et al. Soluble human leukocyte antigen-G in seminal plasma is associated with HLA-G genotype: possible implications for fertility success. Am J Reprod Immunol (2014) 72:89–105. doi:10.1111/aji.12251
119. Lynge Nilsson L, Djurisic S, Hviid TVF. Controlling the immunological crosstalk during conception and pregnancy: HLA-G in reproduction. Front Immunol (2014) 5:198. doi:10.3389/fimmu.2014.00198
120. Martínez-Varea A, Pellicer B, Perales-Marin A, Pellicer A. Relationship between maternal immunological response during pregnancy and onset of preeclampsia. J Immunol Res (2014) 2014:210241. doi:10.1155/2014/210241
121. Sakaguchi S, Yamaguchi T, Nomura T, Ono M. Regulatory T cells and immune tolerance. Cell (2008) 133:775–87. doi:10.1016/j.cell.2008.05.009
122. Aluvihare VR, Kallikourdis M, Betz AG. Regulatory T cells mediate maternal tolerance to the fetus. Nat Immunol (2004) 5:266–71. doi:10.1038/ni1037
123. Guerin LR, Prins JR, Robertson SA. Regulatory T-cells and immune tolerance in pregnancy: a new target for infertility treatment? Hum Reprod Update (2009) 15:517–35. doi:10.1093/humupd/dmp004
124. Saito S. Th17 cells and regulatory T cells: new light on pathophysiology of preeclampsia. Immunol Cell Biol (2010) 88:615–7. doi:10.1038/icb.2010.68
125. Saito S, Nakashima A, Shima T, Ito M. Th1/Th2/Th17 and regulatory T-cell paradigm in pregnancy. Am J Reprod Immunol (2010) 63:601–10. doi:10.1111/j.1600-0897.2010.00852.x
126. Clark DA, Chaouat G. Regulatory T cells and reproduction: how do they do it? J Reprod Immunol (2012) 96:1–7. doi:10.1016/j.jri.2012.07.007
127. Alijotas-Reig J, Llurba E, Gris JM. Potentiating maternal immune tolerance in pregnancy: a new challenging role for regulatory T cells. Placenta (2014) 35:241–8. doi:10.1016/j.placenta.2014.02.004
128. Jiang TT, Chaturvedi V, Ertelt JM, Kinder JM, Clark DR, Valent AM, et al. Regulatory T cells: new keys for further unlocking the enigma of fetal tolerance and pregnancy complications. J Immunol (2014) 192:4949–56. doi:10.4049/jimmunol.1400498
129. La Rocca C, Carbone F, Longobardi S, Matarese G. The immunology of pregnancy: regulatory T cells control maternal immune tolerance toward the fetus. Immunol Lett (2014) 162:41–8. doi:10.1016/j.imlet.2014.06.013
130. Clark DA. The importance of being a regulatory T cell in pregnancy. J Reprod Immunol (2016) 116:60–9. doi:10.1016/j.jri.2016.04.288
131. Harmon AC, Cornelius DC, Amaral LM, Faulkner JL, Cunningham MW Jr, Wallace K, et al. The role of inflammation in the pathology of preeclampsia. Clin Sci (Lond) (2016) 130:409–19. doi:10.1042/CS20150702
132. LaMarca B, Cornelius DC, Harmon AC, Amaral LM, Cunningham MW, Faulkner JL, et al. Identifying immune mechanisms mediating the hypertension during preeclampsia. Am J Physiol Regul Integr Comp Physiol (2016) 311:R1–9. doi:10.1152/ajpregu.00052.2016
133. Nancy P, Tagliani E, Tay CS, Asp P, Levy DE, Erlebacher A. Chemokine gene silencing in decidual stromal cells limits T cell access to the maternal-fetal interface. Science (2012) 336:1317–21. doi:10.1126/science.1220030
134. Williams Z. Inducing tolerance to pregnancy. N Engl J Med (2012) 367:1159–61. doi:10.1056/NEJMcibr1207279
135. Samstein RM, Josefowicz SZ, Arvey A, Treuting PM, Rudensky AY. Extrathymic generation of regulatory T cells in placental mammals mitigates maternal-fetal conflict. Cell (2012) 150:29–38. doi:10.1016/j.cell.2012.05.031
136. Somerset DA, Zheng Y, Kilby MD, Sansom DM, Drayson MT. Normal human pregnancy is associated with an elevation in the immune suppressive CD25+ CD4+ regulatory T-cell subset. Immunology (2004) 112:38–43. doi:10.1111/j.1365-2567.2004.01869.x
137. Schumacher A, Zenclussen AC. Effects of heme oxygenase-1 on innate and adaptive immune responses promoting pregnancy success and allograft tolerance. Front Pharmacol (2014) 5:288. doi:10.3389/fphar.2014.00288
138. Carp HJA, Selmi C, Shoenfeld Y. The autoimmune bases of infertility and pregnancy loss. J Autoimmun (2012) 38:J266–74. doi:10.1016/j.jaut.2011.11.016
139. Mittrücker H-W, Kaufmann SHE. Mini-review: regulatory T cells and infection: suppression revisited. Eur J Immunol (2004) 34:306–12. doi:10.1002/eji.200324578
140. Belkaid Y. Regulatory T cells and infection: a dangerous necessity. Nat Rev Immunol (2007) 7:875–88. doi:10.1038/nri2189
141. Belkaid Y, Tarbell K. Regulatory T cells in the control of host-microorganism interactions. Annu Rev Immunol (2009) 27:551–89. doi:10.1146/annurev.immunol.021908.132723
142. Maizels RM, Smith KA. Regulatory T cells in infection. Adv Immunol (2011) 112:73–136. doi:10.1016/B978-0-12-387827-4.00003-6
143. Sanchez AM, Yang Y. The role of natural regulatory T cells in infection. Immunol Res (2011) 49:124–34. doi:10.1007/s12026-010-8176-8
144. Berod L, Puttur F, Huehn J, Sparwasser T. Tregs in infection and vaccinology: heroes or traitors? Microb Biotechnol (2012) 5:260–9. doi:10.1111/j.1751-7915.2011.00299.x
145. Williams Z, Zepf D, Longtine J, Anchan R, Broadman B, Missmer SA, et al. Foreign fetal cells persist in the maternal circulation. Fertil Steril (2009) 91:2593–5. doi:10.1016/j.fertnstert.2008.02.008
146. Hahn S, Rusterholz C, Hösli I, Lapaire O. Cell-free nucleic acids as potential markers for preeclampsia. Placenta (2011) 32(Suppl):S17–20. doi:10.1016/j.placenta.2010.06.018
147. Visca E, Lapaire O, Hosli I, Hahn S. Cell-free fetal nucleic acids as prenatal biomarkers. Expert Opin Med Diagn (2011) 5:151–60. doi:10.1517/17530059.2011.554821
148. Robertson SA, Bromfield JJ, Tremellen KP. Seminal ‘priming’ for protection from pre-eclampsia-a unifying hypothesis. J Reprod Immunol (2003) 59:253–65. doi:10.1016/S0165-0378(03)00052-4
149. Getz GS. Thematic review series: the immune system and atherogenesis. Bridging the innate and adaptive immune systems. J Lipid Res (2005) 46:619–22. doi:10.1194/jlr.E500002-JLR200
150. Sarma JV, Ward PA. The complement system. Cell Tissue Res (2011) 343:227–35. doi:10.1007/s00441-010-1034-0
151. Kennedy MA. A brief review of the basics of immunology: the innate and adaptive response. Vet Clin North Am Small Anim Pract (2010) 40:369–79. doi:10.1016/j.cvsm.2010.01.003
152. Warrington R, Watson W, Kim HL, Antonetti FR. An introduction to immunology and immunopathology. Allergy Asthma Clin Immunol (2011) 7(Suppl 1):S1. doi:10.1186/1710-1492-7-S1-S1
153. Abbas AK, Lichtman AH, Pillai S. Basic Immunology: Functions and Disorders of the Immune System. 8th ed. St Louis, MO: Elsevier (2012).
154. Abbas AK, Lichtman AH, Pillai S. Basic Immunology: Functions and Disorders of the Immune System. 5th ed. St Louis, MO: Elsevier (2016).
156. Kell DB, Pretorius E. On the translocation of bacteria and their lipopolysaccharides between blood and peripheral locations in chronic, inflammatory diseases: the central roles of LPS and LPS-induced cell death. Integr Biol (2015) 7:1339–77. doi:10.1039/C5IB00158G
157. Legutki JB, Magee DM, Stafford P, Johnston SA. A general method for characterization of humoral immunity induced by a vaccine or infection. Vaccine (2010) 28:4529–37. doi:10.1016/j.vaccine.2010.04.061
158. Stafford P, Halperin R, Legutki JB, Magee DM, Galgiani J, Johnston SA. Physical characterization of the “immunosignaturing effect”. Mol Cell Proteomics (2012) 11:M111011593. doi:10.1074/mcp.M111.011593
159. Sykes KF, Legutki JB, Stafford P. Immunosignaturing: a critical review. Trends Biotechnol (2013) 31:45–51. doi:10.1016/j.tibtech.2012.10.012
160. King AE, Kelly RW, Sallenave J-M, Bocking AD, Challis JRG. Innate immune defences in the human uterus during pregnancy. Placenta (2007) 28:1099–106. doi:10.1016/j.placenta.2007.06.002
161. Schminkey DL, Groer M. Imitating a stress response: a new hypothesis about the innate immune system’s role in pregnancy. Med Hypotheses (2014) 82:721–9. doi:10.1016/j.mehy.2014.03.013
162. Zhang J, Dunk C, Croy AB, Lye SJ. To serve and to protect: the role of decidual innate immune cells on human pregnancy. Cell Tissue Res (2016) 363:249–65. doi:10.1007/s00441-015-2315-4
163. Redman CW. Immunological aspects of pre-eclampsia. Baillieres Clin Obstet Gynaecol (1992) 6:601–15. doi:10.1016/S0950-3552(05)80012-4
164. Saito S, Shiozaki A, Nakashima A, Sakai M, Sasaki Y. The role of the immune system in preeclampsia. Mol Aspects Med (2007) 28:192–209. doi:10.1016/j.mam.2007.02.006
165. Visser N, Van Rijn BB, Rijkers GT, Franx A, Bruinse HW. Inflammatory changes in preeclampsia: current understanding of the maternal innate and adaptive immune response. Obstet Gynecol Surv (2007) 62:191–201. doi:10.1097/01.ogx.0000256779.06275.c4
166. Brewster JA, Orsi NM, Gopichandran N, Mcshane P, Ekbote UV, Walker JJ. Gestational effects on host inflammatory response in normal and pre-eclamptic pregnancies. Eur J Obstet Gynecol Reprod Biol (2008) 140:21–6. doi:10.1016/j.ejogrb.2007.12.020
167. Laresgoiti-Servitje E. A leading role for the immune system in the pathophysiology of preeclampsia. J Leukoc Biol (2013) 94:247–57. doi:10.1189/jlb.1112603
168. Perez-Sepulveda A, Torres MJ, Khoury M, Illanes SE. Innate immune system and preeclampsia. Front Immunol (2014) 5:244. doi:10.3389/fimmu.2014.00244
169. Staff AC, Johnsen GM, Dechend R, Redman CWG. Preeclampsia and uteroplacental acute atherosis: immune and inflammatory factors. J Reprod Immunol (2014) 10(1–102):120–6. doi:10.1016/j.jri.2013.09.001
170. Redman CWG, Sargent IL, Taylor RN. Immunology of normal pregnancy and preeclampsia. 4th ed. Chesley’s Hypertensive Disorders in Pregnancy. (2015). p. 161–79. doi:10.1016/B978-0-12-407866-6.00008-0
171. Parker LC, Prince LR, Sabroe I. Translational mini-review series on toll-like receptors: networks regulated by toll-like receptors mediate innate and adaptive immunity. Clin Exp Immunol (2007) 147:199–207. doi:10.1111/j.1365-2249.2006.03203.x
172. Trinchieri G, Sher A. Cooperation of toll-like receptor signals in innate immune defence. Nat Rev Immunol (2007) 7:179–90. doi:10.1038/nri2038
173. Nelson DE, Ihekwaba AEC, Elliott M, Gibney CA, Foreman BE, Nelson G, et al. Oscillations in NF-κB signalling control the dynamics of gene expression. Science (2004) 306:704–8. doi:10.1126/science.1099962
174. Ashall L, Horton CA, Nelson DE, Paszek P, Ryan S, Sillitoe K, et al. Pulsatile stimulation determines timing and specificity of NFkappa-B-dependent transcription. Science (2009) 324:242–6. doi:10.1126/science.1164860
175. Matzinger P. Tolerance, danger, and the extended family. Annu Rev Immunol (1994) 12:991–1045. doi:10.1146/annurev.iy.12.040194.005015
176. Anderson CC, Matzinger P. Danger: the view from the bottom of the cliff. Semin Immunol (2000) 12:231–8; discussion 257–344. doi:10.1006/smim.2000.0236
177. Matzinger P. Essay 1: the danger model in its historical context. Scand J Immunol (2001) 54:4–9. doi:10.1046/j.1365-3083.2001.00974.x
178. Matzinger P. The danger model: a renewed sense of self. Science (2002) 296:301–5. doi:10.1126/science.1071059
179. Matzinger P, Kamala T. Tissue-based class control: the other side of tolerance. Nat Rev Immunol (2011) 11:221–30. doi:10.1038/nri2940
180. Matzinger P. The evolution of the danger theory. Expert Rev Clin Immunol (2012) 8:311–7. doi:10.1586/eci.12.21
181. McCarthy CM, Kenny LC. Immunostimulatory role of mitochondrial DAMPs: alarming for pre-eclampsia? Am J Reprod Immunol (2016) 76:341–7. doi:10.1111/aji.12526
182. Bonney EA. Preeclampsia: a view through the danger model. J Reprod Immunol (2007) 76:68–74. doi:10.1016/j.jri.2007.03.006
183. Sacks GP, Studena K, Sargent K, Redman CWG. Normal pregnancy and preeclampsia both produce inflammatory changes in peripheral blood leukocytes akin to those of sepsis. Am J Obstet Gynecol (1998) 179:80–6. doi:10.1016/S0002-9378(98)70254-6
184. Redman CWG, Sacks GP, Sargent IL. Preeclampsia: an excessive maternal inflammatory response to pregnancy. Am J Obstet Gynecol (1999) 180:499–506. doi:10.1016/S0002-9378(99)70239-5
185. Kim YM, Romero R, Oh SY, Kim CJ, Kilburn BA, Armant DR, et al. Toll-like receptor 4: a potential link between “danger signals,” the innate immune system and preeclampsia? Am J Obstet Gynecol (2005) 193:921–7. doi:10.1016/j.ajog.2005.06.053
186. Wang CC, Yim KW, Poon TCW, Choy KW, Chu CY, Lui WT, et al. Innate immune response by ficolin binding in apoptotic placenta is associated with the clinical syndrome of preeclampsia. Clin Chem (2007) 53:42–52. doi:10.1373/clinchem.2007.074401
187. Yeh CC, Chao KC, Huang SJ. Innate immunity, decidual cells, and preeclampsia. Reprod Sci (2013) 20:339–53. doi:10.1177/1933719112450330
188. Bounds KR, Newell-Rogers MK, Mitchell BM. Four pathways involving innate immunity in the pathogenesis of preeclampsia. Front Cardiovasc Med (2015) 2:20. doi:10.3389/fcvm.2015.00020
189. Triggianese P, Perricone C, Chimenti MS, De Carolis C, Perricone R. Innate immune system at the maternal-fetal interface: mechanisms of disease and targets of therapy in pregnancy syndromes. Am J Reprod Immunol (2016) 76:245–57. doi:10.1111/aji.12509
190. Redman CWG, Sargent IL. Pre-eclampsia, the placenta and the maternal systemic inflammatory response – a review. Placenta (2003) 24(Suppl A):S21–7. doi:10.1053/plac.2002.0930
191. Hubel CA. Dyslipidemia and pre-eclampsia. In: Belfort MA, Lydall F, editors. Pre-Eclampsia-Aetiology and Clinical Practice. Cambridge: Cambridge University Press (2006). p. 164–82.
192. Shurin MR, Lu L, Kalinski P, Stewart-Akers AM, Lotze MT. Th1/Th2 balance in cancer, transplantation and pregnancy. Springer Semin Immunopathol (1999) 21:339–59. doi:10.1007/BF00812261
193. Saito S, Sakai M. Th1/Th2 balance in preeclampsia. J Reprod Immunol (2003) 59:161–73. doi:10.1016/S0165-0378(03)00045-7
194. Chaouat G. The Th1/Th2 paradigm: still important in pregnancy? Semin Immunopathol (2007) 29:95–113. doi:10.1007/s00281-007-0069-0
195. Short SM, Wolfner MF, Lazzaro BP. Female Drosophila melanogaster suffer reduced defense against infection due to seminal fluid components. J Insect Physiol (2012) 58:1192–201. doi:10.1016/j.jinsphys.2012.06.002
196. Short SM, Lazzaro BP. Reproductive status alters transcriptomic response to infection in female Drosophila melanogaster. G3 (Bethesda) (2013) 3:827–40. doi:10.1534/g3.112.005306
197. Schjenken JE, Robertson SA. Seminal fluid signalling in the female reproductive tract: implications for reproductive success and offspring health. Adv Exp Med Biol (2015) 868:127–58. doi:10.1007/978-3-319-18881-2_6
198. Pejcic-Karapetrovic B, Gurnani K, Russell MS, Finlay BB, Sad S, Krishnan L. Pregnancy impairs the innate immune resistance to Salmonella typhimurium leading to rapid fatal infection. J Immunol (2007) 179:6088–96. doi:10.4049/jimmunol.179.9.6088
199. Sappenfield E, Jamieson DJ, Kourtis AP. Pregnancy and susceptibility to infectious diseases. Infect Dis Obstet Gynecol (2013) 2013:752852. doi:10.1155/2013/752852
200. Kourtis AP, Read JS, Jamieson DJ. Pregnancy and infection. N Engl J Med (2014) 370:2211–8. doi:10.1056/NEJMra1213566
201. Kraus TA, Engel SM, Sperling RS, Kellerman L, Lo Y, Wallenstein S, et al. Characterizing the pregnancy immune phenotype: results of the viral immunity and pregnancy (VIP) study. J Clin Immunol (2012) 32:300–11. doi:10.1007/s10875-011-9627-2
202. Pazos M, Sperling RS, Moran TM, Kraus TA. The influence of pregnancy on systemic immunity. Immunol Res (2012) 54:254–61. doi:10.1007/s12026-012-8303-9
203. Lynch AM, Gibbs RS, Murphy JR, Byers T, Neville MC, Giclas PC, et al. Complement activation fragment Bb in early pregnancy and spontaneous preterm birth. Am J Obstet Gynecol (2008) 199(354):e351–8. doi:10.1016/j.ajog.2008.07.044
204. Lynch AM, Murphy JR, Byers T, Gibbs RS, Neville MC, Giclas PC, et al. Alternative complement pathway activation fragment Bb in early pregnancy as a predictor of preeclampsia. Am J Obstet Gynecol (2008) 198:385.e381–9. doi:10.1016/j.ajog.2007.10.793
205. Lynch AM, Murphy JR, Gibbs RS, Levine RJ, Giclas PC, Salmon JE, et al. The interrelationship of complement-activation fragments and angiogenesis-related factors in early pregnancy and their association with pre-eclampsia. BJOG (2010) 117:456–62. doi:10.1111/j.1471-0528.2009.02473.x
206. Soto E, Romero R, Richani K, Espinoza J, Chaiworapongsa T, Nien JK, et al. Preeclampsia and pregnancies with small-for-gestational age neonates have different profiles of complement split products. J Matern Fetal Neonatal Med (2010) 23:646–57. doi:10.3109/14767050903301009
207. Girardi G, Prohaszka Z, Bulla R, Tedesco F, Scherjon S. Complement activation in animal and human pregnancies as a model for immunological recognition. Mol Immunol (2011) 48:1621–30. doi:10.1016/j.molimm.2011.04.011
208. Lynch AM, Gibbs RS, Murphy JR, Giclas PC, Salmon JE, Holers VM. Early elevations of the complement activation fragment C3a and adverse pregnancy outcomes. Obs Gynecol (2011) 117:75–83. doi:10.1097/AOG.0b013e3181fc3afa
209. Qing X, Redecha PB, Burmeister MA, Tomlinson S, D’agati VD, Davisson RL, et al. Targeted inhibition of complement activation prevents features of preeclampsia in mice. Kidney Int (2011) 79:331–9. doi:10.1038/ki.2010.393
210. Buurma A, Cohen D, Veraar K, Schonkeren D, Claas FH, Bruijn JA, et al. Preeclampsia is characterized by placental complement dysregulation. Hypertension (2012) 60:1332–7. doi:10.1161/HYPERTENSIONAHA.112.194324
211. Wang W, Irani RA, Zhang Y, Ramin SM, Blackwell SC, Tao L, et al. Autoantibody-mediated complement C3a receptor activation contributes to the pathogenesis of preeclampsia. Hypertension (2012) 60:712–21. doi:10.1161/HYPERTENSIONAHA.112.191817
212. Denny KJ, Coulthard LG, Finnell RH, Callaway LK, Taylor SM, Woodruff TM. Elevated complement factor C5a in maternal and umbilical cord plasma in preeclampsia. J Reprod Immunol (2013) 97:211–6. doi:10.1016/j.jri.2012.11.006
213. Denny KJ, Woodruff TM, Taylor SM, Callaway LK. Complement in pregnancy: a delicate balance. Am J Reprod Immunol (2013) 69:3–11. doi:10.1111/aji.12000
214. Hoffman MC, Rumer KK, Kramer A, Lynch AM, Winn VD. Maternal and fetal alternative complement pathway activation in early severe preeclampsia. Am J Reprod Immunol (2014) 71:55–60. doi:10.1111/aji.12162
215. Banadakoppa M, Vidaeff AC, Yallampalli U, Ramin SM, Belfort MA, Yallampalli C. Complement split products in amniotic fluid in pregnancies subsequently developing early-onset preeclampsia. Dis Markers (2015) 2015:263109. doi:10.1155/2015/263109
216. He Y, Xu B, Song D, Yu F, Chen Q, Zhao M. Expression of the complement system’s activation factors in plasma of patients with early/late-onset severe pre-eclampsia. Am J Reprod Immunol (2016) 76:205–11. doi:10.1111/aji.12541
217. Wu W, Yang H, Feng Y, Zhang P, Li S, Wang X, et al. Polymorphisms in complement genes and risk of preeclampsia in Taiyuan, China. Inflamm Res (2016) 65:837–45. doi:10.1007/s00011-016-0968-4
218. Velickovic I, Dalloul M, Wong KA, Bakare O, Schweis F, Garala M, et al. Complement factor B activation in patients with preeclampsia. J Reprod Immunol (2015) 109:94–100. doi:10.1016/j.jri.2014.12.002
219. Soto E, Romero R, Vaisbuch E, Erez O, Mazaki-Tovi S, Kusanovic JP, et al. Fragment Bb: evidence for activation of the alternative pathway of the complement system in pregnant women with acute pyelonephritis. J Matern Fetal Neonatal Med (2010) 23:1085–90. doi:10.3109/14767051003649870
220. He Y, Xu B, Song D, Yu F, Chen Q, Zhao M. Correlations between complement system’s activation factors and anti-angiogenesis factors in plasma of patients with early/late-onset severe preeclampsia. Hypertens Pregnancy (2016) 35:499–509. doi:10.1080/10641955.2016.1190845
221. Lynch AM, Eckel RH, Murphy JR, Gibbs RS, West NA, Giclas PC, et al. Prepregnancy obesity and complement system activation in early pregnancy and the subsequent development of preeclampsia. Am J Obstet Gynecol (2012) 206:428.e421–28. doi:10.1016/j.ajog.2012.02.035
222. Halmos A, Rigo J Jr, Szijarto J, Fust G, Prohaszka Z, Molvarec A. Circulating ficolin-2 and ficolin-3 in normal pregnancy and pre-eclampsia. Clin Exp Immunol (2012) 169:49–56. doi:10.1111/j.1365-2249.2012.04590.x
223. Haeger M, Bengtson A, Karlsson K, Heideman M. Complement activation and anaphylatoxin (C3a and C5a) formation in preeclampsia and by amniotic fluid. Obstet Gynecol (1989) 73:551–6.
224. Haeger M, Unander M, Bengtsson A. Complement activation in relation to development of preeclampsia. Obstet Gynecol (1991) 78:46–9.
225. Ye Y, Kong Y, Zhang Y. Complement split products C3a/C5a and receptors: are they regulated by circulating angiotensin II type 1 receptor autoantibody in severe preeclampsia? Gynecol Obstet Invest (2016) 81:28–33. doi:10.1159/000440651
226. Kestlerová A, Feyereisl J, Frisová V, Měchurová A, Šůla K, Zima T, et al. Immunological and biochemical markers in preeclampsia. J Reprod Immunol (2012) 96:90–4. doi:10.1016/j.jri.2012.10.002
227. Burwick RM, Fichorova RN, Dawood HY, Yamamoto HS, Feinberg BB. Urinary excretion of C5b-9 in severe preeclampsia: tipping the balance of complement activation in pregnancy. Hypertension (2013) 62:1040–5. doi:10.1161/HYPERTENSIONAHA.113.01420
228. Merle NS, Church SE, Fremeaux-Bacchi V, Roumenina LT. Complement system part I – molecular mechanisms of activation and regulation. Front Immunol (2015) 6:262. doi:10.3389/fimmu.2015.00262
229. Merle NS, Noe R, Halbwachs-Mecarelli L, Fremeaux-Bacchi V, Roumenina LT. Complement system part II: role in immunity. Front Immunol (2015) 6:257. doi:10.3389/fimmu.2015.00257
230. Ponnuraj K, Xu Y, Macon K, Moore D, Volanakis JE, Narayana SVL. Structural analysis of engineered Bb fragment of complement factor B: insights into the activation mechanism of the alternative pathway C3-convertase. Mol Cell (2004) 14:17–28. doi:10.1016/S1097-2765(04)00160-1
231. Rooijakkers SHM, Wu J, Ruyken M, Van Domselaar R, Planken KL, Tzekou A, et al. Structural and functional implications of the alternative complement pathway C3 convertase stabilized by a staphylococcal inhibitor. Nat Immunol (2009) 10:721–7. doi:10.1038/ni.1756
232. Vaisbuch E, Romero R, Erez O, Mazaki-Tovi S, Kusanovic JP, Soto E, et al. Fragment Bb in amniotic fluid: evidence for complement activation by the alternative pathway in women with intra-amniotic infection/inflammation. J Matern Fetal Neonatal Med (2009) 22:905–16. doi:10.1080/14767050902994663
233. Li Q, Li YX, Stahl GL, Thurman JM, He Y, Tong HH. Essential role of factor B of the alternative complement pathway in complement activation and opsonophagocytosis during acute pneumococcal otitis media in mice. Infect Immun (2011) 79:2578–85. doi:10.1128/IAI.00168-11
234. Singh J, Ahmed A, Girardi G. Role of complement component C1q in the onset of preeclampsia in mice. Hypertension (2011) 58:716–24. doi:10.1161/HYPERTENSIONAHA.111.175919
235. Marti JJ, Herrmann U. Immunogestosis: a new etiologic concept of “essential” EPH gestosis, with special consideration of the primigravid patient; preliminary report of a clinical study. Am J Obstet Gynecol (1977) 128:489–93. doi:10.1016/0002-9378(77)90030-8
236. Dekker G, Sukcharoen N. Etiology of preeclampsia: an update. J Med Assoc Thai (2004) 87(Suppl 3):S96–103.
237. Johansson M, Bromfield JJ, Jasper MJ, Robertson SA. Semen activates the female immune response during early pregnancy in mice. Immunology (2004) 112:290–300. doi:10.1111/j.1365-2567.2004.01876.x
238. Robertson SA, Guerin LR, Bromfield JJ, Branson KM, Ahlström AC, Care AS. Seminal fluid drives expansion of the CD4+CD25+ T regulatory cell pool and induces tolerance to paternal alloantigens in mice. Biol Reprod (2009) 80:1036–45. doi:10.1095/biolreprod.108.074658
239. Larsen MH, Bzorek M, Pass MB, Larsen LG, Nielsen MW, Svendsen SG, et al. Human leukocyte antigen-G in the male reproductive system and in seminal plasma. Mol Hum Reprod (2011) 17:727–38. doi:10.1093/molehr/gar052
240. Hviid TVF. Human leukocyte antigen-G within the male reproductive system: implications for reproduction. Adv Exp Med Biol (2015) 868:171–90. doi:10.1007/978-3-319-18881-2_8
241. Anderson DJ, Politch JA. Role of seminal plasma in human female reproductive failure: immunomodulation, inflammation, and infections. Adv Exp Med Biol (2015) 868:159–69. doi:10.1007/978-3-319-18881-2_7
242. Milardi D, Grande G, Vincenzoni F, Castagnola M, Marana R. Proteomics of human seminal plasma: identification of biomarker candidates for fertility and infertility and the evolution of technology. Mol Reprod Dev (2013) 80:350–7. doi:10.1002/mrd.22178
243. Drabovich AP, Saraon P, Jarvi K, Diamandis EP. Seminal plasma as a diagnostic fluid for male reproductive system disorders. Nat Rev Urol (2014) 11:278–88. doi:10.1038/nrurol.2014.74
244. Robertson SA. Seminal plasma and male factor signalling in the female reproductive tract. Cell Tissue Res (2005) 322:43–52. doi:10.1007/s00441-005-1127-3
245. Robertson SA. Seminal fluid signaling in the female reproductive tract: lessons from rodents and pigs. J Anim Sci (2007) 85:E36–44. doi:10.2527/jas.2006-578
246. Bromfield JJ. Seminal fluid and reproduction: much more than previously thought. J Assist Reprod Genet (2014) 31:627–36. doi:10.1007/s10815-014-0243-y
247. Bromfield JJ, Schjenken JE, Chin PY, Care AS, Jasper MJ, Robertson SA. Maternal tract factors contribute to paternal seminal fluid impact on metabolic phenotype in offspring. Proc Natl Acad Sci U S A (2014) 111:2200–5. doi:10.1073/pnas.1305609111
248. Robertson SA, Sharkey DJ. Seminal fluid and fertility in women. Fertil Steril (2016) 106:511–9. doi:10.1016/j.fertnstert.2016.07.1101
249. García-Montalvo IA, Mayoral Andrade G, Perez-Campos Mayoral L, Pina Canseco S, Martinez Cruz R, Martinez-Cruz M, et al. Molecules in seminal plasma related to platelets in preeclampsia. Med Hypotheses (2016) 93:27–9. doi:10.1016/j.mehy.2016.05.009
250. Robertson SA, Guerin LR, Moldenhauer LM, Hayball JD. Activating T regulatory cells for tolerance in early pregnancy – the contribution of seminal fluid. J Reprod Immunol (2009) 83:109–16. doi:10.1016/j.jri.2009.08.003
251. Robertson SA, Prins JR, Sharkey DJ, Moldenhauer LM. Seminal fluid and the generation of regulatory T cells for embryo implantation. Am J Reprod Immunol (2013) 69:315–30. doi:10.1111/aji.12107
252. Shima T, Inada K, Nakashima A, Ushijima A, Ito M, Yoshino O, et al. Paternal antigen-specific proliferating regulatory T cells are increased in uterine-draining lymph nodes just before implantation and in pregnant uterus just after implantation by seminal plasma-priming in allogeneic mouse pregnancy. J Reprod Immunol (2015) 108:72–82. doi:10.1016/j.jri.2015.02.005
253. Saito S, Shima T, Nakashima A, Inada K, Yoshino O. Role of paternal antigen-specific treg cells in successful implantation. Am J Reprod Immunol (2016) 75:310–6. doi:10.1111/aji.12469
254. Okazaki T, Akiyoshi T, Kan M, Mori M, Teshima H, Shimada M. Artificial insemination with seminal plasma improves the reproductive performance of frozen-thawed boar epididymal spermatozoa. J Androl (2012) 33:990–8. doi:10.2164/jandrol.111.015115
255. Bromfield JJ. A role for seminal plasma in modulating pregnancy outcomes in domestic species. Reproduction (2016) 152:R223–32. doi:10.1530/REP-16-0313
256. Duckitt K, Harrington D. Risk factors for pre-eclampsia at antenatal booking: systematic review of controlled studies. Br Med J (2005) 330:565–7. doi:10.1136/bmj.38380.674340.E0
257. Bartsch E, Medcalf KE, Park AL, Ray JG; High Risk of Pre-eclampsia Identification Group. Clinical risk factors for pre-eclampsia determined in early pregnancy: systematic review and meta-analysis of large cohort studies. BMJ (2016) 353:i1753. doi:10.1136/bmj.i1753
258. Bdolah Y, Elchalal U, Natanson-Yaron S, Yechiam H, Bdolah-Abram T, Greenfield C, et al. Relationship between nulliparity and preeclampsia may be explained by altered circulating soluble fms-like tyrosine kinase 1. Hypertens Pregnancy (2014) 33:250–9. doi:10.3109/10641955.2013.858745
259. Tandberg A, Klungsoyr K, Romundstad LB, Skjaerven R. Pre-eclampsia and assisted reproductive technologies: consequences of advanced maternal age, interbirth intervals, new partner and smoking habits. BJOG (2015) 122:915–22. doi:10.1111/1471-0528.13051
260. Robillard PY, Hulsey TC, Perianin J, Janky E, Miri EH, Papiernik E. Association of pregnancy-induced hypertension with duration of sexual cohabitation before conception. Lancet (1994) 344:973–5. doi:10.1016/S0140-6736(94)91638-1
261. Einarsson JI, Sangi-Haghpeykar H, Gardner MO. Sperm exposure and development of preeclampsia. Am J Obstet Gynecol (2003) 188:1241–3. doi:10.1067/mob.2003.401
262. Saftlas AF, Levine RJ, Klebanoff MA, Martz KL, Ewell MG, Morris CD, et al. Abortion, changed paternity, and risk of preeclampsia in nulliparous women. Am J Epidemiol (2003) 157:1108–14. doi:10.1093/aje/kwg101
263. Klonoff-Cohen HS, Savitz DA, Cefalo RC, Mccann MF. An epidemiologic study of contraception and preeclampsia. JAMA (1989) 262:3143–7. doi:10.1001/jama.262.22.3143
264. Hernández-Valencia M, Saldaña Quezada L, Alvarez Muñoz M, Valdez Martínez E. Barrier family planning methods as risk factor which predisposes to preeclampsia. Ginecol Obstet Mex (2000) 68:333–8.
265. Letur-Köenirsch H, Peigné M, Ohl J, Cédrin I, D’argent EM, Scheffler F, et al. Pregnancies issued from egg donation are associated to a higher risk of hypertensive pathologies then control ART pregnancies. Results of a large comparative cohort study. Hum Reprod (2014) 29:68–9.
266. Letur H, Peigné M, Ohl J, Cédrin-Durnerin I, Mathieu-D’argent E, Scheffler F, et al. Hypertensive pathologies and egg donation pregnancies: results of a large comparative cohort study. Fertil Steril (2016) 106:284–90. doi:10.1016/j.fertnstert.2016.03.031
267. Tarlatzi TB, Imbert R, Alvaro Mercadal B, Demeestere I, Venetis CA, Englert Y, et al. Does oocyte donation compared with autologous oocyte IVF pregnancies have a higher risk of preeclampsia? Reprod Biomed Online (2017) 34:11–8. doi:10.1016/j.rbmo.2016.10.002
268. Giannubilo SR, Landi B, Ciavattini A. Preeclampsia: what could happen in a subsequent pregnancy? Obstet Gynecol Surv (2014) 69:747–62. doi:10.1097/OGX.0000000000000126
269. Koelman CA, Coumans ABC, Nijman HW, Doxiadis IIN, Dekker GA, Claas FHJ. Correlation between oral sex and a low incidence of preeclampsia: a role for soluble HLA in seminal fluid? J Reprod Immunol (2000) 46:155–66. doi:10.1016/S0165-0378(99)00062-5
270. Martin RD. A Biological Function for Oral Sex? Psychology Today (2016). Available from: https://www.psychologytoday.com/blog/how-we-do-it/201602/biological-function-oral-sex
271. Saftlas AF, Olson DR, Franks AL, Atrash HK, Pokras R. Epidemiology of preeclampsia and eclampsia in the United-States, 1979–1986. Am J Obstet Gynecol (1990) 163:460–5. doi:10.1016/0002-9378(90)91176-D
272. Zhang J, Zeisler J, Hatch MC, Berkowitz G. Epidemiology of pregnancy-induced hypertension. Epidemiol Rev (1997) 19:218–32. doi:10.1093/oxfordjournals.epirev.a017954
273. Lamminpää R, Vehvilainen-Julkunen K, Gissler M, Heinonen S. Preeclampsia complicated by advanced maternal age: a registry-based study on primiparous women in Finland 1997–2008. BMC Pregnancy Childbirth (2012) 12:47. doi:10.1186/1471-2393-12-47
274. Ananth CV, Keyes KM, Wapner RJ. Pre-eclampsia rates in the United States, 1980–2010: age-period-cohort analysis. BMJ (2013) 347:f6564. doi:10.1136/bmj.f6564
275. Carolan M. Maternal age >= 45 years and maternal and perinatal outcomes: a review of the evidence. Midwifery (2013) 29:479–89. doi:10.1016/j.midw.2012.04.001
276. Need JA, Bell B, Meffin E, Jones WR. Pre-eclampsia in pregnancies from donor inseminations. J Reprod Immunol (1983) 5:329–38. doi:10.1016/0165-0378(83)90242-5
277. Smith GN, Walker M, Tessier JL, Millar KG. Increased incidence of preeclampsia in women conceiving by intrauterine insemination with donor versus partner sperm for treatment of primary infertility. Am J Obs Gynecol (1997) 177:455–8. doi:10.1016/S0002-9378(97)70215-1
278. Hoy J, Venn A, Halliday J, Kovacs G, Waalwyk K. Perinatal and obstetric outcomes of donor insemination using cryopreserved semen in Victoria, Australia. Hum Reprod (1999) 14:1760–4. doi:10.1093/humrep/14.7.1760
279. Davis JA, Gallup GG. Preeclampsia and other pregnancy complications as an adaptive response to unfamiliar semen. In: Platek SM, Shackelford TK, editors. Female Infidelity and Paternal Uncertainty: Evolutionary Perspectives on Male Anti-Cuckoldry Tactics. Cambridge: CUPpip (2006). p. 191–204.
280. Gleicher N, Boler LR Jr, Norusis M, Del Granado A. Hypertensive diseases of pregnancy and parity. Am J Obstet Gynecol (1986) 154:1044–9. doi:10.1016/0002-9378(86)90747-7
281. Roberts JM, Redman CW. Pre-eclampsia: more than pregnancy-induced hypertension. Lancet (1993) 341:1447–51. doi:10.1016/0140-6736(93)90889-O
282. Sibai B, Dekker G, Kupferminc M. Pre-eclampsia. Lancet (2005) 365:785–99. doi:10.1016/S0140-6736(05)71003-5
283. Luo ZC, An N, Xu HR, Larante A, Audibert F, Fraser WD. The effects and mechanisms of primiparity on the risk of pre-eclampsia: a systematic review. Paediatr Perinat Epidemiol (2007) 21(Suppl 1):36–45. doi:10.1111/j.1365-3016.2007.00836.x
284. Hernández-Díaz S, Toh S, Cnattingius S. Risk of pre-eclampsia in first and subsequent pregnancies: prospective cohort study. BMJ (2009) 338:b2255. doi:10.1136/bmj.b2255
285. Wu CS, Nohr EA, Bech BH, Vestergaard M, Catov JM, Olsen J. Health of children born to mothers who had preeclampsia: a population-based cohort study. Am J Obstet Gynecol (2009) 201:269.e261–269.e210. doi:10.1016/j.ajog.2009.06.060
286. Wikström AK, Gunnarsdóttir J, Cnattingius S. The paternal role in pre-eclampsia and giving birth to a small for gestational age infant; a population-based cohort study. BMJ Open (2012) 2:e001178. doi:10.1136/bmjopen-2012-001178
287. English FA, Kenny LC, Mccarthy FP. Risk factors and effective management of preeclampsia. Integr Blood Press Control (2015) 8:7–12. doi:10.2147/IBPC.S50641
288. Rich-Edwards JW, Ness RB, Roberts JM. Epidemiology of pregnancy-related hypertension. 4th ed. Chesley’s Hypertensive Disorders in Pregnancy. (2015). p. 37–55. doi:10.1016/B978-0-12-407866-6.00003-1
289. Dildy GA III, Belfort MA, Smulian JC. Preeclampsia recurrence and prevention. Semin Perinatol (2007) 31:135–41. doi:10.1053/j.semperi.2007.03.005
290. Conde-Agudelo A, Belizán JM. Risk factors for pre-eclampsia in a large cohort of Latin American and Caribbean women. BJOG (2000) 107:75–83. doi:10.1111/j.1471-0528.2000.tb11582.x
291. Feeney JG, Scott JS. Pre-eclampsia and changed paternity. Eur J Obstet Gynecol Reprod Biol (1980) 11:35–8. doi:10.1016/0028-2243(80)90051-9
293. Chng PK. Occurrence of pre-eclampsia in pregnancies to three husbands. Case report. Br J Obstet Gynaecol (1982) 89:862–3. doi:10.1111/j.1471-0528.1982.tb05042.x
294. Robillard PY, Hulsey TC, Alexander GR, Keenan A, De Caunes F, Papiernik E. Paternity patterns and risk of preeclampsia in the last pregnancy in multiparae. J Reprod Immunol (1993) 24:1–12. doi:10.1016/0165-0378(93)90032-D
295. Trupin LS, Simon LP, Eskenazi B. Change in paternity: a risk factor for preeclampsia in multiparas. Epidemiology (1996) 7:240–4. doi:10.1097/00001648-199605000-00004
296. Robillard PY, Dekker GA, Hulsey TC. Revisiting the epidemiological standard of preeclampsia: primigravidity or primipaternity? Eur J Obstet Gynecol Reprod Biol (1999) 84:37–41. doi:10.1016/S0301-2115(98)00250-4
297. Tubbergen P, Lachmeijer AMA, Althuisius SM, Vlak MEJ, Van Geijn HP, Dekker GA. Change in paternity: a risk factor for preeclampsia in multiparous women? J Reprod Immunol (1999) 45:81–8. doi:10.1016/S0165-0378(99)00040-6
298. Li DK, Wi S. Changing paternity and the risk of preeclampsia/eclampsia in the subsequent pregnancy. Am J Epidemiol (2000) 151:57–62. doi:10.1093/oxfordjournals.aje.a010122
299. Dekker GA, Robillard PY. Preeclampsia: a couple’s disease with maternal and fetal manifestations. Curr Pharm Des (2005) 11:699–710. doi:10.2174/1381612053381828
300. Deen ME, Ruurda LG, Wang J, Dekker GA. Risk factors for preeclampsia in multiparous women: primipaternity versus the birth interval hypothesis. J Matern Fetal Neonatal Med (2006) 19:79–84. doi:10.1080/14767050500361653
301. Dekker G, Robillard PY, Roberts C. The etiology of preeclampsia: the role of the father. J Reprod Immunol (2011) 89:126–32. doi:10.1016/j.jri.2010.12.010
302. Nagayama S, Ohkuchi A, Usui R, Matsubara S, Suzuki M. The role of the father in the occurrence of preeclampsia. Med J Obs Gynecol (2014) 2:1029–32.
303. Robillard PY, Dekker G, Chaouat G, Scioscia M, Iacobelli S, Hulsey TC. Historical evolution of ideas on eclampsia/preeclampsia: a proposed optimistic view of preeclampsia. J Reprod Immunol (2017) 123:72–7. doi:10.1016/j.jri.2017.09.006
304. Trogstad LIS, Eskild A, Magnus P, Samuelsen SO, Nesheim BI. Changing paternity and time since last pregnancy; the impact on pre-eclampsia risk. A study of 547 238 women with and without previous pre-eclampsia. Int J Epidemiol (2001) 30:1317–22. doi:10.1093/ije/30.6.1317
305. Lie RT, Rasmussen S, Brunborg H, Gjessing HK, Lie-Nielsen E, Irgens LM. Fetal and maternal contributions to risk of pre-eclampsia: population based study. BMJ (1998) 316:1343–7. doi:10.1136/bmj.316.7141.1343
306. Triche EW, Harland KK, Field EH, Rubenstein LM, Saftlas AF. Maternal-fetal HLA sharing and preeclampsia: variation in effects by seminal fluid exposure in a case-control study of nulliparous women in Iowa. J Reprod Immunol (2014) 101-102:111–9. doi:10.1016/j.jri.2013.06.004
307. Saftlas AF, Rubenstein L, Prater K, Harland KK, Field E, Triche EW. Cumulative exposure to paternal seminal fluid prior to conception and subsequent risk of preeclampsia. J Reprod Immunol (2014) 101-102:104–10. doi:10.1016/j.jri.2013.07.006
308. Kho EM, Mccowan LM, North RA, Roberts CT, Chan E, Black MA, et al. Duration of sexual relationship and its effect on preeclampsia and small for gestational age perinatal outcome. J Reprod Immunol (2009) 82:66–73. doi:10.1016/j.jri.2009.04.011
309. Bellamy L, Casas JP, Hingorani AD, Williams DJ. Pre-eclampsia and risk of cardiovascular disease and cancer in later life: systematic review and meta-analysis. BMJ (2007) 335:974. doi:10.1136/bmj.39335.385301.BE
310. Salha O, Sharma V, Dada T, Nugent D, Rutherford AJ, Tomlinson AJ, et al. The influence of donated gametes on the incidence of hypertensive disorders of pregnancy. Hum Reprod (1999) 14:2268–73. doi:10.1093/humrep/14.9.2268
311. Gelbaya TA. Short and long-term risks to women who conceive through in vitro fertilization. Hum Fertil (Camb) (2010) 13:19–27. doi:10.3109/14647270903437923
312. van der Hoorn ML, Lashley EE, Bianchi DW, Claas FH, Schonkeren CM, Scherjon SA. Clinical and immunologic aspects of egg donation pregnancies: a systematic review. Hum Reprod Update (2010) 16:704–12. doi:10.1093/humupd/dmq017
313. Masoudian P, Nasr A, De Nanassy J, Fung-Kee-Fung K, Bainbridge SA, El Demellawy D. Oocyte donation pregnancies and the risk of preeclampsia or gestational hypertension: a systematic review and metaanalysis. Am J Obstet Gynecol (2016) 214:328–39. doi:10.1016/j.ajog.2015.11.020
314. Porreco RP, Heyborne KD. Immunogenesis of preeclampsia: lessons from donor gametes. J Matern Fetal Neonatal Med (2017):1–7. doi:10.1080/14767058.2017.1309385
315. Dude AM, Yeh JS, Muasher SJ. Donor oocytes are associated with preterm birth when compared to fresh autologous in vitro fertilization cycles in singleton pregnancies. Fertil Steril (2016) 106:660–5. doi:10.1016/j.fertnstert.2016.05.029
316. Stoop D, Baumgarten M, Haentjens P, Polyzos NP, De Vos M, Verheyen G, et al. Obstetric outcome in donor oocyte pregnancies: a matched-pair analysis. Reprod Biol Endocrinol (2012) 10:42. doi:10.1186/1477-7827-10-42
317. Levron Y, Dviri M, Segol I, Yerushalmi GM, Hourvitz A, Orvieto R, et al. The ’immunologic theory’ of preeclampsia revisited: a lesson from donor oocyte gestations. Am J Obstet Gynecol (2014) 211(383):e381–5. doi:10.1016/j.ajog.2014.03.044
318. Fox NS, Roman AS, Saltzman DH, Hourizadeh T, Hastings J, Rebarber A. Risk factors for preeclampsia in twin pregnancies. Am J Perinatol (2014) 31:163–6. doi:10.1055/s-0033-1343775
319. Thomopoulos C, Salamalekis G, Kintis K, Andrianopoulou I, Michalopoulou H, Skalis G, et al. Risk of hypertensive disorders in pregnancy following assisted reproductive technology: overview and meta-analysis. J Clin Hypertens (Greenwich) (2017) 19:173–83. doi:10.1111/jch.12945
320. Blázquez A, García D, Rodríguez A, Vassena R, Figueras F, Vernaeve V. Is oocyte donation a risk factor for preeclampsia? A systematic review and meta-analysis. J Assist Reprod Genet (2016) 33:855–63. doi:10.1007/s10815-016-0701-9
321. Klatsky PC, Delaney SS, Caughey AB, Tran ND, Schattman GL, Rosenwaks Z. The role of embryonic origin in preeclampsia: a comparison of autologous in vitro fertilization and ovum donor pregnancies. Obstet Gynecol (2010) 116:1387–92. doi:10.1097/AOG.0b013e3181fb8e59
322. Kroon B, Hart RJ, Wong BM, Ford E, Yazdani A. Antibiotics prior to embryo transfer in ART. Cochrane Database Syst Rev (2012) (3):CD008995. doi:10.1002/14651858.CD008995.pub2
323. Sibai BM, Mercer B, Sarinoglu C. Severe preeclampsia in the second trimester: recurrence risk and long-term prognosis. Am J Obstet Gynecol (1991) 165:1408–12. doi:10.1016/S0002-9378(12)90773-5
324. van Rijn BB, Hoeks LB, Bots ML, Franx A, Bruinse HW. Outcomes of subsequent pregnancy after first pregnancy with early-onset preeclampsia. Am J Obstet Gynecol (2006) 195:723–8. doi:10.1016/j.ajog.2006.06.044
325. Mostello D, Kallogjeri D, Tungsiripat R, Leet T. Recurrence of preeclampsia: effects of gestational age at delivery of the first pregnancy, body mass index, paternity, and interval between births. Am J Obstet Gynecol (2008) 199(55):e51–7. doi:10.1016/j.ajog.2007.11.058
326. Rasmussen S, Irgens LM, Albrechtsen S, Dalaker K. Predicting preeclampsia in the second pregnancy from low birth weight in the first pregnancy. Obstet Gynecol (2000) 96:696–700. doi:10.1097/00006250-200011000-00010
327. Thevaranjan N, Puchta A, Schulz C, Naidoo A, Szamosi JC, Verschoor CP, et al. Age-associated microbial dysbiosis promotes intestinal permeability, systemic inflammation, and macrophage dysfunction. Cell Host Microbe (2017) 21(455–466):e454. doi:10.1016/j.chom.2017.03.002
328. Astin M, Scott JR, Worley RJ. Pre-eclampsia/eclampsia: a fatal father factor. Lancet (1981) 2:533. doi:10.1016/S0140-6736(81)90925-9
329. Wang JX, Knottnerus AM, Schuit G, Norman RJ, Chan A, Dekker GA. Surgically obtained sperm, and risk of gestational hypertension and pre-eclampsia. Lancet (2002) 359:673–4. doi:10.1016/S0140-6736(02)07804-2
330. Le Ray C, Scherier S, Anselem O, Marszalek A, Tsatsaris V, Cabrol D, et al. Association between oocyte donation and maternal and perinatal outcomes in women aged 43 years or older. Hum Reprod (2012) 27:896–901. doi:10.1093/humrep/der469
331. González-Comadran M, Avila JU, Tascón AS, Jimenéz R, Solà I, Brassesco M, et al. The impact of donor insemination on the risk of preeclampsia: a systematic review and meta-analysis. Eur J Obstet Gynecol Reprod Biol (2014) 182:160–6. doi:10.1016/j.ejogrb.2014.09.022
332. Thomopoulos C, Tsioufis C, Michalopoulou H, Makris T, Papademetriou V, Stefanadis C. Assisted reproductive technology and pregnancy-related hypertensive complications: a systematic review. J Hum Hypertens (2013) 27:148–57. doi:10.1038/jhh.2012.13
333. Baud D, Greub G. Intracellular bacteria and adverse pregnancy outcomes. Clin Microbiol Infect (2011) 17:1312–22. doi:10.1111/j.1469-0691.2011.03604.x
334. Vigliani MB, Bakardjiev AI. Intracellular organisms as placental invaders. Fetal Matern Med Rev (2014) 25:332–8. doi:10.1017/S0965539515000066
335. Parnell LA, Briggs CM, Cao B, Delannoy-Bruno O, Schrieffer AE, Mysorekar IU. Microbial communities in placentas from term normal pregnancy exhibit spatially variable profiles. Sci Rep (2017) 7:11200. doi:10.1038/s41598-017-11514-4
336. Wu H, Estill MS, Shershebnev A, Suvorov A, Krawetz SA, Whitcomb BW, et al. Preconception urinary phthalate concentrations and sperm DNA methylation profiles among men undergoing IVF treatment: a cross-sectional study. Hum Reprod (2017) 32(11):2159–69. doi:10.1093/humrep/dex283
337. Verstraelen H, Senok AC. Vaginal lactobacilli, probiotics, and IVF. Reprod Biomed Online (2005) 11:674–5. doi:10.1016/S1472-6483(10)61683-5
338. Sirota I, Zarek SM, Segars JH. Potential influence of the microbiome on infertility and assisted reproductive technology. Semin Reprod Med (2014) 32:35–42. doi:10.1055/s-0033-1361821
339. Reid G, Brigidi P, Burton JP, Contractor N, Duncan S, Fargier E, et al. Microbes central to human reproduction. Am J Reprod Immunol (2015) 73:1–11. doi:10.1111/aji.12319
340. Xie F, Hu Y, Magee LA, Money DM, Patrick DM, Brunham RM, et al. Chlamydia pneumoniae infection in preeclampsia. Hypertens Pregnancy (2010) 29:468–77. doi:10.3109/10641950903242642
341. Heine RP, Ness RB, Roberts JM. Seroprevalence of antibodies to Chlamydia pneumoniae in women with preeclampsia. Obstet Gynecol (2003) 101:221–6. doi:10.1016/S0029-7844(02)02591-7
342. El-Shourbagy MAA, El-Refaie TA, Sayed KKA, Wahba KAH, El-Din ASS, Fathy MM. Impact of seroconversion and antichlamydial treatment on the rate of pre-eclampsia among Egyptian primigravidae. Int J Gynaecol Obstet (2011) 113:137–40. doi:10.1016/j.ijgo.2010.11.014
343. Mosbah A, Nabiel Y. Helicobacter pylori, Chlamydiae pneumoniae and trachomatis as probable etiological agents of preeclampsia. J Matern Fetal Neonat Med (2016) 29:1607–12. doi:10.3109/14767058.2015.1056146
344. Gomez LM, Parry S. Trophoblast infection with Chlamydia pneumoniae and adverse pregnancy outcomes associated with placental dysfunction. Am J Obstet Gynecol (2009) 200(526):e521–7. doi:10.1016/j.ajog.2009.03.001
345. Haggerty CL, Klebanoff MA, Panum I, Uldum SA, Bass DC, Olsen J, et al. Prenatal Chlamydia trachomatis infection increases the risk of preeclampsia. Pregnancy Hypertens (2013) 3:151–4. doi:10.1016/j.preghy.2013.03.002
346. Haggerty CL, Panum I, Uldum SA, Bass DC, Olsen J, Darville T, et al. Chlamydia trachomatis infection may increase the risk of preeclampsia. Pregnancy Hypertens (2013) 3:28–33. doi:10.1016/j.preghy.2012.09.002
347. Xie F, Hu Y, Magee LA, Money DM, Patrick DM, Krajden M, et al. An association between cytomegalovirus infection and pre-eclampsia: a case-control study and data synthesis. Acta Obstet Gynecol Scand (2010) 89:1162–7. doi:10.3109/00016349.2010.499449
348. Xie F, Von Dadelszen P, Nadeau J. CMV infection, TLR-2 and -4 expression, and cytokine profiles in early-onset preeclampsia with HELLP syndrome. Am J Reprod Immunol (2014) 71:379–86. doi:10.1111/aji.12199
349. Ponzetto A, Cardaropoli S, Piccoli E, Rolfo A, Gennero L, Kanduc D, et al. Pre-eclampsia is associated with Helicobacter pylori seropositivity in Italy. J Hypertens (2006) 24:2445–9. doi:10.1097/HJH.0b013e3280109e8c
350. Panarelli M, Sattar N. Pre-eclampsia associated with Helicobacter pylori seropositivity. J Hypertens (2006) 24:2353–4. doi:10.1097/HJH.0b013e3280113638
351. Tersigni C, Franceschi F, Todros T, Cardaropoli S, Scambia G, Di Simone N. Insights into the role of Helicobacter pylori infection in preeclampsia: from the bench to the bedside. Front Immunol (2014) 5:484. doi:10.3389/fimmu.2014.00484
352. Üstün Y, Engin-Üstün Y, Ozkaplan E, Otlu B, Sait Tekerekoğlu M. Association of Helicobacter pylori infection with systemic inflammation in preeclampsia. J Matern Fetal Neonatal Med (2010) 23:311–4. doi:10.3109/14767050903121456
353. Aksoy H, Ozkan A, Aktas F, Borekci B. Helicobacter pylori seropositivity and its relationship with serum malondialdehyde and lipid profile in preeclampsia. J Clin Lab Anal (2009) 23:219–22. doi:10.1002/jcla.20330
354. Cardaropoli S, Rolfo A, Todros T. Helicobacter pylori and pregnancy-related disorders. World J Gastroenterol (2014) 20:654–64. doi:10.3748/wjg.v20.i3.654
355. Pugliese A, Beltramo T, Todros T, Cardaropoli S, Ponzetto A. Interleukin-18 and gestosis: correlation with Helicobacter pylori seropositivity. Cell Biochem Funct (2008) 26:817–9. doi:10.1002/cbf.1503
356. Cardaropoli S, Giuffrida D, Piazzese A, Todros T. Helicobacter pylori seropositivity and pregnancy-related diseases: a prospective cohort study. J Reprod Immunol (2015) 109:41–7. doi:10.1016/j.jri.2015.02.004
357. Cardaropoli S, Rolfo A, Piazzese A, Ponzetto A, Todros T. Helicobacter pylori’s virulence and infection persistence define pre-eclampsia complicated by fetal growth retardation. World J Gastroenterol (2011) 17:5156–65. doi:10.3748/wjg.v17.i47.5156
358. den Hollander WJ, Schalekamp-Timmermans S, Holster IL, Jaddoe VW, Hofman A, Moll HA, et al. Helicobacter pylori colonization and pregnancies complicated by preeclampsia, spontaneous prematurity, and small for gestational age birth. Helicobacter (2017) 22:e12364. doi:10.1111/hel.12364
359. Sansone M, Sarno L, Saccone G, Berghella V, Maruotti GM, Migliucci A, et al. Risk of preeclampsia in human immunodeficiency virus-infected pregnant women. Obstet Gynecol (2016) 127:1027–32. doi:10.1097/AOG.0000000000001424
360. McDonnold M, Dunn H, Hester A, Pacheco LD, Hankins GD, Saade GR, et al. High risk human papillomavirus at entry to prenatal care and risk of preeclampsia. Am J Obstet Gynecol (2014) 210(138):e131–5. doi:10.1016/j.ajog.2013.09.040
361. Hill JA, Devoe LD, Bryans CI Jr. Frequency of asymptomatic bacteriuria in preeclampsia. Obstet Gynecol (1986) 67:529–32.
362. Hsu CD, Witter FR. Urogenital infection in preeclampsia. Int J Gynaecol Obstet (1995) 49:271–5. doi:10.1016/0020-7292(95)02373-K
363. Mittendorf R, Lain KY, Williams MA, Walker CK. Preeclampsia. A nested, case-control study of risk factors and their interactions. J Reprod Med (1996) 41:491–6.
364. Easter SR, Cantonwine DE, Zera CA, Lim KH, Parry SI, Mcelrath TF. Urinary tract infection during pregnancy, angiogenic factor profiles, and risk of preeclampsia. Am J Obs Gynecol (2016) 214:387.e1–7. doi:10.1016/j.ajog.2015.09.101
365. Mazor-Dray E, Levy A, Schlaeffer F, Sheiner E. Maternal urinary tract infection: is it independently associated with adverse pregnancy outcome? J Matern Fetal Neonatal Med (2009) 22:124–8. doi:10.1080/14767050802488246
366. Minassian C, Thomas SL, Williams DJ, Campbell O, Smeeth L. Acute maternal infection and risk of pre-eclampsia: a population-based case-control study. PLoS One (2013) 8:e73047. doi:10.1371/journal.pone.0073047
367. Rezavand N, Veisi F, Zangane M, Amini R, Almasi A. Association between asymptomatic bacteriuria and pre-eclampsia. Glob J Health Sci (2016) 8:235–9. doi:10.5539/gjhs.v8n7p235
368. Karmon A, Sheiner E. The relationship between urinary tract infection during pregnancy and preeclampsia: causal, confounded or spurious? Arch Gynecol Obstet (2008) 277:479–81. doi:10.1007/s00404-008-0643-2
369. Villar J, Carroli G, Wojdyla D, Abalos E, Giordano D, Ba’aqeel H, et al. Preeclampsia, gestational hypertension and intrauterine growth restriction, related or independent conditions? Am J Obstet Gynecol (2006) 194:921–31. doi:10.1016/j.ajog.2005.10.813
370. Bánhidy F, Ács N, Puhó EH, Czeizel AE. Pregnancy complications and birth outcomes of pregnant women with urinary tract infections and related drug treatments. Scand J Infect Dis (2007) 39:390–7. doi:10.1080/00365540601087566
371. Ide M, Papapanou PN. Epidemiology of association between maternal periodontal disease and adverse pregnancy outcomes – systematic review. J Periodontol (2013) 84:S181–94. doi:10.1902/jop.2013.134009
372. Dunlop AL, Mulle JG, Ferranti EP, Edwards S, Dunn AB, Corwin EJ. Maternal microbiome and pregnancy outcomes that impact infant health: a review. Adv Neonatal Care (2015) 15:377–85. doi:10.1097/ANC.0000000000000218
373. Doron MW, Makhlouf RA, Katz VL, Lawson EE, Stiles AD. Increased incidence of sepsis at birth in neutropenic infants of mothers with preeclampsia. J Pediatr (1994) 125:452–8. doi:10.1016/S0022-3476(05)83294-9
374. Wei B-J, Chen Y-J, Yu L, Wu B. Periodontal disease and risk of preeclampsia: a meta-analysis of observational studies. PLoS One (2013) 8:e70901. doi:10.1371/journal.pone.0070901
375. Shetty M, Shetty PK, Ramesh A, Thomas B, Prabhu S, Rao A. Periodontal disease in pregnancy is a risk factor for preeclampsia. Acta Obstet Gynecol Scand (2010) 89:718–21. doi:10.3109/00016341003623738
376. Kumar A, Basra M, Begum N, Rani V, Prasad S, Lamba AK, et al. Association of maternal periodontal health with adverse pregnancy outcome. J Obstet Gynaecol Res (2013) 39:40–5. doi:10.1111/j.1447-0756.2012.01957.x
377. Amarasekara R, Jayasekara RW, Senanayake H, Dissanayake VHW. Microbiome of the placenta in pre-eclampsia supports the role of bacteria in the multifactorial cause of pre-eclampsia. J Obstet Gynaecol Res (2015) 41:662–9. doi:10.1111/jog.12619
378. Hlimi T. Association of anemia, pre-eclampsia and eclampsia with seasonality: a realist systematic review. Health Place (2015) 31:180–92. doi:10.1016/j.healthplace.2014.12.003
379. Brabin BJ, Johnson PM. Placental malaria and pre-eclampsia through the looking glass backwards? J Reprod Immunol (2005) 65:1–15. doi:10.1016/j.jri.2004.09.006
380. Anya SE. Seasonal variation in the risk and causes of maternal death in the Gambia: malaria appears to be an important factor. Am J Trop Med Hyg (2004) 70:510–3.
381. Sartelet H, Rogier C, Milko-Sartelet I, Angel G, Michel G. Malaria associated pre-eclampsia in Senegal. Lancet (1996) 347:1121. doi:10.1016/S0140-6736(96)90321-9
382. Silasi M, Cardenas I, Kwon JY, Racicot K, Aldo P, Mor G. Viral infections during pregnancy. Am J Reprod Immunol (2015) 73:199–213. doi:10.1111/aji.12355
383. Racicot K, Mor G. Risks associated with viral infections during pregnancy. J Clin Invest (2017) 127:1591–9. doi:10.1172/JCI87490
384. Keck C, Gerber-Schafer C, Clad A, Wilhelm C, Breckwoldt M. Seminal tract infections: impact on male fertility and treatment options. Hum Reprod Update (1998) 4:891–903. doi:10.1093/humupd/4.6.891
385. Ochsendorf FR. Sexually transmitted infections: impact on male fertility. Andrologia (2008) 40:72–5. doi:10.1111/j.1439-0272.2007.00825.x
386. Swidsinski A, Dörffel Y, Loening-Baucke V, Mendling W, Verstraelen H, Dieterle S, et al. Desquamated epithelial cells covered with a polymicrobial biofilm typical for bacterial vaginosis are present in randomly selected cryopreserved donor semen. FEMS Immunol Med Microbiol (2010) 59:399–404. doi:10.1111/j.1574-695X.2010.00688.x
387. Gallo MF, Warner L, King CC, Sobel JD, Klein RS, Cu-Uvin S, et al. Association between semen exposure and incident bacterial vaginosis. Infect Dis Obstet Gynecol (2011) 2011:842652. doi:10.1155/2011/842652
388. Paavonen J, Eggert-Kruse W. Chlamydia trachomatis: impact on human reproduction. Hum Reprod Update (1999) 5:433–47. doi:10.1093/humupd/5.5.433
389. Rando OJ, Simmons RA. I’m eating for two: parental dietary effects on offspring metabolism. Cell (2015) 161:93–105. doi:10.1016/j.cell.2015.02.021
390. Dehghan Marvast L, Aflatoonian A, Talebi AR, Ghasemzadeh J, Pacey AA. Semen inflammatory markers and Chlamydia trachomatis infection in male partners of infertile couples. Andrologia (2016) 48:729–36. doi:10.1111/and.12501
391. López-Hurtado M, Velazco-Fernández M, Pedraza-Sánchez MJE, Flores-Salazar VR, Villagrana Zesati R, Guerra-Infante FM. Molecular detection of Chlamydia trachomatis and semen quality of sexual partners of infertile women. Andrologia (2017) e12812. doi:10.1111/and.12812
392. Delwart EL, Mullins JI, Gupta P, Learn GH Jr, Holodniy M, Katzenstein D, et al. Human immunodeficiency virus type 1 populations in blood and semen. J Virol (1998) 72:617–23.
393. Winter AJ, Taylor S, Workman J, White D, Ross JD, Swan AV, et al. Asymptomatic urethritis and detection of HIV-1 RNA in seminal plasma. Sex Transm Infect (1999) 75:261–3. doi:10.1136/sti.75.4.261
394. Pilcher CD, Joaki G, Hoffman IF, Martinson FE, Mapanje C, Stewart PW, et al. Amplified transmission of HIV-1: comparison of HIV-1 concentrations in semen and blood during acute and chronic infection. AIDS (2007) 21:1723–30. doi:10.1097/QAD.0b013e3281532c82
395. Hladik F, McElrath MJ. Setting the stage: host invasion by HIV. Nat Rev Immunol (2008) 8:447–57. doi:10.1038/nri2302
396. Liu CM, Osborne BJW, Hungate BA, Shahabi K, Huibner S, Lester R, et al. The semen microbiome and its relationship with local immunology and viral load in HIV infection. PloS Path (2014) 10:e1004262. doi:10.1371/journal.ppat.1004262
397. Rametse CL, Olivier AJ, Masson L, Barnabas S, Mckinnon LR, Ngcapu S, et al. Role of semen in altering the balance between inflammation and tolerance in the female genital tract: does it contribute to HIV risk? Viral Immunol (2014) 27:200–6. doi:10.1089/vim.2013.0136
398. Gruber F, Lipozencic J, Kehler T. History of venereal diseases from antiquity to the renaissance. Acta Dermatovenerol Croat (2015) 23:1–11.
399. Sherman JK, Rosenfeld J. Importance of frozen-stored human semen in the spread of gonorrhea. Fertil Steril (1975) 26:1043–7. doi:10.1016/S0015-0282(16)41468-8
400. Zheng H. Analysis of the antigen-antibody specificity in the semen of patients with Neisseria gonorrhoeae. Chin Med Sci J (1997) 12:47–9.
401. Isbey SF, Alcorn TM, Davis RH, Haizlip J, Leone PA, Cohen MS. Characterisation of Neisseria gonorrhoeae in semen during urethral infection in men. Genitourin Med (1997) 73:378–82.
402. Kertséz G. A new method of inoculation to prove the infectivity of the semen in latent syphilis. Br J Dermatol Syph (1931) 43:588–92. doi:10.1111/j.1365-2133.1931.tb09454.x
403. Adeoba A. Interpretation of positive serological tests for syphilis in pregnancy. Br J Vener Dis (1967) 43:249–58.
404. Burchell AN, Allen VG, Gardner SL, Moravan V, Tan DHS, Grewal R, et al. High incidence of diagnosis with syphilis co-infection among men who have sex with men in an HIV cohort in Ontario, Canada. BMC Infect Dis (2015) 15:356. doi:10.1186/s12879-015-1098-2
405. Punjabi U, Wyns C, Mahmoud A, Vernelen K, China B, Verheyen G. Fifteen years of Belgian experience with external quality assessment of semen analysis. Andrology (2016) 4:1084–93. doi:10.1111/andr.12230
406. Trompoukis C, Kalaitzis C, Giannakopoulos S, Sofikitis N, Touloupidis S. Semen and the diagnosis of infertility in Aristotle. Andrologia (2007) 39:33–7. doi:10.1111/j.1439-0272.2006.00757.x
407. Jungwirth A, Diemer T, Dohle GR, Giwercman A, Kopa Z, Krausz C, et al. Guidelines on Male Infertility. Eur Assoc Urol (2015).
408. Mändar R, Punab M, Borovkova N, Lapp E, Kiiker R, Korrovits P, et al. Complementary seminovaginal microbiome in couples. Res Microbiol (2015) 166:440–7. doi:10.1016/j.resmic.2015.03.009
409. Fowlkes DM, Dooher GB, O’Leary WM. Evidence by scanning electron microscopy for an association between spermatozoa and T-mycoplasmas in men of infertile marriage. Fertil Steril (1975) 26:1203–11. doi:10.1016/S0015-0282(16)41536-0
410. Fowlkes DM, Macleod J, O’Leary WM. T-mycoplasmas and human infertility: correlation of infection with alterations in seminal parameters. Fertil Steril (1975) 26:1212–8. doi:10.1016/S0015-0282(16)41537-2
411. Rehewy MS, Hafez ES, Thomas A, Brown WJ. Aerobic and anaerobic bacterial flora in semen from fertile and infertile groups of men. Arch Androl (1979) 2:263–8. doi:10.3109/01485017908987323
412. Swenson CE, Toth A, Toth C, Wolfgruber L, O’Leary WM. Asymptomatic bacteriospermia in infertile men. Andrologia (1980) 12:7–11. doi:10.1111/j.1439-0272.1980.tb00567.x
413. Mogra N, Dhruva A, Kothari LK. Non-specific seminal tract infection and male infertility: a bacteriological study. J Postgrad Med (1981) 27:99–104.
414. Busolo F, Zanchetta R, Lanzone E, Cusinato R. Microbial flora in semen of asymptomatic infertile men. Andrologia (1984) 16:269–75. doi:10.1111/j.1439-0272.1984.tb00282.x
415. Naessens A, Foulon W, Debrucker P, Devroey P, Lauwers S. Recovery of microorganisms in semen and relationship to semen evaluation. Fertil Steril (1986) 45:101–5. doi:10.1016/S0015-0282(16)49105-3
416. Eggert-Kruse W, Rohr G, Strock W, Pohl S, Schwalbach B, Runnebaum B. Anaerobes in ejaculates of subfertile men. Hum Reprod Update (1995) 1:462–78. doi:10.1093/humupd/1.5.462
417. Merino G, Carranza-Lira S, Murrieta S, Rodriguez L, Cuevas E, Moran C. Bacterial infection and semen characteristics in infertile men. Arch Androl (1995) 35:43–7. doi:10.3109/01485019508987852
418. Jarvi K, Lacroix JM, Jain A, Dumitru I, Heritz D, Mittelman MW. Polymerase chain reaction-based detection of bacteria in semen. Fertil Steril (1996) 66:463–7. doi:10.1016/S0015-0282(16)58520-3
419. Lacroix JM, Jarvi K, Batra SD, Heritz DM, Mittelman MW. PCR-based technique for the detection of bacteria in semen and urine. J Microbiol Meth (1996) 26:61–71. doi:10.1016/0167-7012(96)00844-5
420. Byrn RA, Kiessling AA. Analysis of human immunodeficiency virus in semen: indications of a genetically distinct virus reservoir. J Reprod Immunol (1998) 41:161–76. doi:10.1016/S0165-0378(98)00056-4
421. Cardoso EM, Santoianni JE, De Paulis AN, Andrada JA, Predari SC, Arregger AL. Improvement of semen quality in infected asymptomatic infertile male after bacteriological cure. Medicina (B Aires) (1998) 58:160–4.
422. Köhn FM, Erdmann I, Oeda T, El Mulla KF, Schiefer HG, Schill WB. Influence of urogenital infections on sperm functions. Andrologia (1998) 30(Suppl 1):73–80. doi:10.1111/j.1439-0272.1998.tb02829.x
423. Onemu SO, Ibeh IN. Studies on the significance of positive bacterial semen cultures in male fertility in Nigeria. Int J Fertil Womens Med (2001) 46:210–4.
424. Esfandiari N, Saleh RA, Abdoos M, Rouzrokh A, Nazemian Z. Positive bacterial culture of semen from infertile men with asymptomatic leukocytospermia. Int J Fertil Womens Med (2002) 47:265–70.
425. Sanocka D, Fraczek M, Jedrzejczak P, Szumala-Kakol A, Kurpisz M. Male genital tract infection: an influence of leukocytes and bacteria on semen. J Reprod Immunol (2004) 62:111–24. doi:10.1016/j.jri.2003.10.005
426. Sanocka-Maciejewska D, Ciupińska M, Kurpisz M. Bacterial infection and semen quality. J Reprod Immunol (2005) 67:51–6. doi:10.1016/j.jri.2005.06.003
427. Gdoura R, Kchaou W, Chaari C, Znazen A, Keskes L, Rebai T, et al. Ureaplasma urealyticum, Ureaplasma parvum, Mycoplasma hominis and Mycoplasma genitalium infections and semen quality of infertile men. BMC Infect Dis (2007) 7:129. doi:10.1186/1471-2334-7-129
428. Ikechukwu O, George E, Sabinus AE, Florence O. Role of enriched media in bacterial isolation from semen and effect of microbial infection on semen quality: a study on 100 infertile men. Pak J Med Sci (2007) 23:885–8.
429. Kiessling AA, Desmarais BM, Yin HZ, Loverde J, Eyre RC. Detection and identification of bacterial DNA in semen. Fertil Steril (2008) 90:1744–56. doi:10.1016/j.fertnstert.2007.08.083
430. Pellati D, Mylonakis I, Bertoloni G, Fiore C, Andrisani A, Ambrosini G, et al. Genital tract infections and infertility. Eur J Obstet Gynecol Reprod Biol (2008) 140:3–11. doi:10.1016/j.ejogrb.2008.03.009
431. Moretti E, Capitani S, Figura N, Pammolli A, Federico MG, Giannerini V, et al. The presence of bacteria species in semen and sperm quality. J Assist Reprod Genet (2009) 26:47–56. doi:10.1007/s10815-008-9283-5
432. Kokab A, Akhondi MM, Sadeghi MR, Modarresi MH, Aarabi M, Jennings R, et al. Raised inflammatory markers in semen from men with asymptomatic chlamydial infection. J Androl (2010) 31:114–20. doi:10.2164/jandrol.109.008300
433. Onemu SO, Ogbimi AO, Ophori EA. Microbiology and semen indices of sexually-active males in Benin City, Edo State, Nigeria. J Bacteriol Res (2010) 2:55–9.
434. Uneke CJ, Ugwuoru CD. Antibiotic susceptibility of urogenital microbial profile of infertile men in South-eastern Nigeria. Andrologia (2010) 42:268–73. doi:10.1111/j.1439-0272.2009.00988.x
435. De Francesco MA, Negrini R, Ravizzola G, Galli P, Manca N. Bacterial species present in the lower male genital tract: a five-year retrospective study. Eur J Contracept Reprod Health Care (2011) 16:47–53. doi:10.3109/13625187.2010.533219
436. Hamada A, Agarwal A, Sharma R, French DB, Ragheb A, Sabanegh ES Jr. Empirical treatment of low-level leukocytospermia with doxycycline in male infertility patients. Urology (2011) 78:1320–5. doi:10.1016/j.urology.2011.08.062
437. Isaiah IN, Nche BT, Nwagu IG, Nnanna II. Current studies on bacterospermia the leading cause of male infertility: a protégé and potential threat towards mans extinction. N Am J Med Sci (2011) 3:562–4. doi:10.4297/najms.2011.3559
438. La Vignera S, Vicari E, Condorelli RA, D’agata R, Calogero AE. Male accessory gland infection and sperm parameters (review). Int J Androl (2011) 34:e330–47. doi:10.1111/j.1365-2605.2011.01200.x
439. Momoh ARM, Idonije BO, Nwoke EO, Osifo UC, Okhai O, Omoroguiwa A, et al. Pathogenic bacteria-a probable cause of primary infertility among couples in Ekpoma. J Microbiol Biotechnol Res (2011) 1:66–71.
440. Kaur S, Prabha V. Infertility as a consequence of spermagglutinating Staphylococcus aureus colonization in genital tract of female mice. PLoS One (2012) 7:e52325. doi:10.1371/journal.pone.0052325
441. Rusz A, Pilatz A, Wagenlehner F, Linn T, Diemer T, Schuppe HC, et al. Influence of urogenital infections and inflammation on semen quality and male fertility. World J Urol (2012) 30:23–30. doi:10.1007/s00345-011-0726-8
442. Salmeri M, Valenti D, La Vignera S, Bellanca S, Morello A, Toscano MA, et al. Prevalence of Ureaplasma urealyticum and Mycoplasma hominis infection in unselected infertile men. J Chemother (2012) 24:81–6. doi:10.1179/1120009X12Z.00000000021
443. Nabi A, Khalili MA, Halvaei I, Ghasemzadeh J, Zare E. Seminal bacterial contaminations: probable factor in unexplained recurrent pregnancy loss. Iran J Reprod Med (2013) 11:925–32.
444. Sleha R, Boštíková V, Salavec M, Mosio P, Kusáková E, Kukla R, et al. Bacterial infection as a cause of infertility in humans(paper in Czech). Epidemiol Mikrobiol Imunol (2013) 62:26–32.
445. La Vignera S, Condorelli RA, Vicari E, Salmeri M, Morgia G, Favilla V, et al. Microbiological investigation in male infertility: a practical overview. J Med Microbiol (2014) 63:1–14. doi:10.1099/jmm.0.062968-0
446. Weng SL, Chiu CM, Lin FM, Huang WC, Liang C, Yang T, et al. Bacterial communities in semen from men of infertile couples: metagenomic sequencing reveals relationships of seminal microbiota to semen quality. PLoS One (2014) 9:e110152. doi:10.1371/journal.pone.0110152
447. Fraczek M, Kurpisz M. Mechanisms of the harmful effects of bacterial semen infection on ejaculated human spermatozoa: potential inflammatory markers in semen. Folia Histochem Cytobiol (2015) 53:201–17. doi:10.5603/fhc.a2015.0019
448. Vander H, Prabha V. Evaluation of fertility outcome as a consequence of intravaginal inoculation with sperm-impairing micro-organisms in a mouse model. J Med Microbiol (2015) 64:344–7. doi:10.1099/jmm.0.000036
449. Fraczek M, Hryhorowicz M, Gill K, Zarzycka M, Gaczarzewicz D, Jedrzejczak P, et al. The effect of bacteriospermia and leukocytospermia on conventional and nonconventional semen parameters in healthy young normozoospermic males. J Reprod Immunol (2016) 118:18–27. doi:10.1016/j.jri.2016.08.006
450. Ruggeri M, Cannas S, Cubeddu M, Molicotti P, Piras GL, Dessole S, et al. Bacterial agents as a cause of infertility in humans. New Microbiol (2016) 39:206–9.
451. Shiadeh MN, Niyyati M, Fallahi S, Rostami A. Human parasitic protozoan infection to infertility: a systematic review. Parasitol Res (2016) 115:469–77. doi:10.1007/s00436-015-4827-y
452. Vicari LO, Castiglione R, Salemi M, Vicari BO, Mazzarino MC, Vicari E. Effect of levofloxacin treatment on semen hyperviscosity in chronic bacterial prostatitis patients. Andrologia (2016) 48:380–8. doi:10.1111/and.12456
453. Ahmadi MH, Mirsalehian A, Sadighi Gilani MA, Bahador A, Talebi M. Asymptomatic infection with Mycoplasma hominis negatively affects semen parameters and leads to male infertility as confirmed by improved semen parameters after antibiotic treatment. Urology (2017) 100:97–102. doi:10.1016/j.urology.2016.11.018
454. Kjaergaard N, Kristensen B, Hansen ES, Farholt S, Schønheyder HC, Uldbjerg N, et al. Microbiology of semen specimens from males attending a fertility clinic. APMIS (1997) 105:566–70. doi:10.1111/j.1699-0463.1997.tb05054.x
455. Hillier SL, Rabe LK, Muller CH, Zarutskie P, Kuzan FB, Stenchever MA. Relationship of bacteriologic characteristics to semen indices in men attending an infertility clinic. Obstet Gynecol (1990) 75:800–4.
456. Dieterle S. Urogenital infections in reproductive medicine. Andrologia (2008) 40:117–9. doi:10.1111/j.1439-0272.2008.00833.x
457. Vilvanathan S, Kandasamy B, Jayachandran AL, Sathiyanarayanan S, Tanjore Singaravelu V, Krishnamurthy V, et al. Bacteriospermia and its impact on basic semen parameters among infertile men. Interdiscip Perspect Infect Dis (2016) 2016:2614692. doi:10.1155/2016/2614692
458. Liversedge NH, Jenkins JM, Keay SD, Mclaughlin EA, Al-Sufyan H, Maile LA, et al. Antibiotic treatment based on seminal cultures from asymptomatic male partners in in-vitro fertilization is unnecessary and may be detrimental. Hum Reprod (1996) 11:1227–31. doi:10.1093/oxfordjournals.humrep.a019361
459. Bhandari P, Rishi P, Prabha V. Positive effect of probiotic Lactobacillus plantarum in reversing the LPS induced infertility in mouse model. J Med Microbiol (2016) 65:345–50. doi:10.1099/jmm.0.000230
460. Hou D, Zhou X, Zhong X, Settles ML, Herring J, Wang L, et al. Microbiota of the seminal fluid from healthy and infertile men. Fertil Steril (2013) 100:1261–9. doi:10.1016/j.fertnstert.2013.07.1991
461. Javurek AB, Spollen WG, Ali AMM, Johnson SA, Lubahn DB, Bivens NJ, et al. Discovery of a novel seminal fluid microbiome and influence of estrogen receptor alpha genetic status. Sci Rep (2016) 6. doi:10.1038/srep23027
462. Craig LB, Peck JD, Xu J, Sankaranarayanan K, Warinner C, Hansen KR, et al. Characterizing the semen microbiome and associations with semen parameters: the Chasm study. Fertil Steril (2015) 104:E66. doi:10.1016/j.fertnstert.2015.07.202
463. Deen GF, Knust B, Broutet N, Sesay FR, Formenty P, Ross C, et al. Ebola RNA persistence in semen of ebola virus disease survivors – preliminary report. N Engl J Med (2015). doi:10.1056/NEJMoa1511410
464. Soka MJ, Choi MJ, Baller A, White S, Rogers E, Purpura LJ, et al. Prevention of sexual transmission of ebola in Liberia through a national semen testing and counselling programme for survivors: an analysis of ebola virus RNA results and behavioural data. Lancet Glob Health (2016) 4:e736–43. doi:10.1016/S2214-109X(16)30175-9
465. Thorson A, Formenty P, Lofthouse C, Broutet N. Systematic review of the literature on viral persistence and sexual transmission from recovered ebola survivors: evidence and recommendations. BMJ Open (2016) 6:e008859. doi:10.1136/bmjopen-2015-008859
466. Sissoko D, Duraffour S, Kerber R, Kolie JS, Beavogui AH, Camara AM, et al. Persistence and clearance of ebola virus RNA from seminal fluid of ebola virus disease survivors: a longitudinal analysis and modelling study. Lancet Glob Health (2017) 5:e80–8. doi:10.1016/S2214-109X(16)30243-1
467. Klatt NR, Cheu R, Birse K, Zevin AS, Perner M, Noël-Romas L, et al. Vaginal bacteria modify HIV tenofovir microbicide efficacy in African women. Science (2017) 356:938–45. doi:10.1126/science.aai9383
468. Ma W, Li S, Ma S, Jia L, Zhang F, Zhang Y, et al. Zika virus causes testis damage and leads to male infertility in mice. Cell (2016) 167:1511–24.e1510. doi:10.1016/j.cell.2016.11.016
469. Baud D, Musso D, Vouga M, Alves MP, Vulliemoz N. Zika virus: a new threat to human reproduction. Am J Reprod Immunol (2017) 77:e12614. doi:10.1111/aji.12614
470. Hamer DH, Wilson ME, Jean J, Chen LH. Epidemiology, prevention, and potential future treatments of sexually transmitted zika virus infection. Curr Infect Dis Rep (2017) 19:16. doi:10.1007/s11908-017-0571-z
471. Ohri M, Prabha V. Isolation of a sperm-agglutinating factor from Staphylococcus aureus isolated from a woman with unexplained infertility. Fertil Steril (2005) 84:1539–41. doi:10.1016/j.fertnstert.2005.05.030
472. Diemer T, Huwe P, Ludwig M, Hauck EW, Weidner W. Urogenital infection and sperm motility. Andrologia (2003) 35:283–7. doi:10.1111/j.1439-0272.2003.tb00858.x
473. Prabha V, Sandhu R, Kaur S, Kaur K, Sarwal A, Mavuduru RS, et al. Mechanism of sperm immobilization by Escherichia coli. Adv Urol (2010) 2010:240268. doi:10.1155/2010/240268
474. Fraczek M, Wiland E, Piasecka M, Boksa M, Gaczarzewicz D, Szumala-Kakol A, et al. Fertilizing potential of ejaculated human spermatozoa during in vitro semen bacterial infection. Fertil Steril (2014) 102(711–719):e711. doi:10.1016/j.fertnstert.2014.06.002
475. Kaur K, Prabha V. Spermagglutinating Escherichia coli and its role in infertility: in vivo study. Microb Pathog (2014) 6(9–70):33–8. doi:10.1016/j.micpath.2014.03.010
476. Pretorius E, Mbotwe S, Bester J, Robinson CJ, Kell DB. Acute induction of anomalous and amyloidogenic blood clotting by molecular amplification of highly substoichiometric levels of bacterial lipopolysaccharide. J R Soc Interface (2016) 123:20160539. doi:10.1098/rsif.2016.0539
477. Kell DB, Pretorius E. Proteins behaving badly. Substoichiometric molecular control and amplification of the initiation and nature of amyloid fibril formation: lessons from and for blood clotting. Progr Biophys Mol Biol (2017) 123:16–41. doi:10.1016/j.pbiomolbio.2016.08.006
478. Pretorius E, Page MJ, Hendricks L, Nkosi NB, Benson SR, Kell DB. Both Lipopolysaccharide and Lipoteichoic Acids Potently Induce Anomalous Fibrin Amyloid Formation: Assessment with Novel Amytracker™ Stains. bioRxiv preprint. bioRxiv, 143867 (2017). doi:10.1101/143867
479. Gupta S, Prabha V. Human sperm interaction with Staphylococcus aureus: a molecular approach. J Pathog (2012) 2012:816536. doi:10.1155/2012/816536
480. Enwuru CA, Iwalokun B, Enwuru VN, Ezechi O, Oluwadun A. The effect of presence of facultative bacteria species on semen and sperm quality of men seeking fertility care. Afr J Urol (2016) 22:213–22. doi:10.1016/j.afju.2016.03.010
481. Anway MD, Cupp AS, Uzumcu M, Skinner MK. Epigenetic transgenerational actions of endocrine disruptors and mate fertility. Science (2005) 308:1466–9. doi:10.1126/science.1108190
482. Fenech M. Micronuclei and their association with sperm abnormalities, infertility, pregnancy loss, pre-eclampsia and intra-uterine growth restriction in humans. Mutagenesis (2011) 26:63–7. doi:10.1093/mutage/geq084
483. Pandian Z, Bhattacharya S, Templeton A. Review of unexplained infertility and obstetric outcome: a 10 year review. Hum Reprod (2001) 16:2593–7. doi:10.1093/humrep/16.12.2593
484. Trogstad L, Magnus P, Moffett A, Stoltenberg C. The effect of recurrent miscarriage and infertility on the risk of pre-eclampsia. BJOG (2009) 116:108–13. doi:10.1111/j.1471-0528.2008.01978.x
485. Basso O, Baird DD. Infertility and preterm delivery, birthweight, and Caesarean section: a study within the Danish National Birth Cohort. Hum Reprod (2003) 18:2478–84. doi:10.1093/humrep/deg444
486. Basso O, Weinberg CR, Baird DD, Wilcox AJ, Olsen J. Subfecundity as a correlate of preeclampsia: a study within the Danish National Birth Cohort. Am J Epidemiol (2003) 157:195–202. doi:10.1093/aje/kwf194
487. Sohrabvand F, Jafari M, Shariat M, Haghollahi F, Lotfi M. Frequency and epidemiologic aspects of male infertility. Acta Med Iran (2015) 53:231–5.
488. Dobson PD, Kell DB. Carrier-mediated cellular uptake of pharmaceutical drugs: an exception or the rule? Nat Rev Drug Disc (2008) 7:205–20. doi:10.1038/nrd2438
489. Kell DB, Dobson PD, Bilsland E, Oliver SG. The promiscuous binding of pharmaceutical drugs and their transporter-mediated uptake into cells: what we (need to) know and how we can do so. Drug Disc Today (2013) 18:218–39. doi:10.1016/j.drudis.2012.11.008
490. Kell DB, Oliver SG. How drugs get into cells: tested and testable predictions to help discriminate between transporter-mediated uptake and lipoidal bilayer diffusion. Front Pharmacol (2014) 5:231. doi:10.3389/fphar.2014.00231
491. Pajovic B, Radojevic N, Vukovic M, Stjepcevic A. Semen analysis before and after antibiotic treatment of asymptomatic Chlamydia- and Ureaplasma-related pyospermia. Andrologia (2013) 45:266–71. doi:10.1111/and.12004
492. Schoor RA. Prostatitis and male infertility: evidence and links. Curr Urol Rep (2002) 3:324–9. doi:10.1007/s11934-002-0058-8
493. Everaert K, Mahmoud A, Depuydt C, Maeyaert M, Comhaire F. Chronic prostatitis and male accessory gland infection – is there an impact on male infertility (diagnosis and therapy)? Andrologia (2003) 35:325–30. doi:10.1111/j.1439-0272.2003.tb00867.x
494. La Vignera S, Condorelli R, Vicari E, D’agata R, Calogero AE. High frequency of sexual dysfunction in patients with male accessory gland infections. Andrologia (2012) 44(Suppl 1):438–46. doi:10.1111/j.1439-0272.2011.01202.x
495. Alshahrani S, Mcgill J, Agarwal A. Prostatitis and male infertility. J Reprod Immunol (2013) 100:30–6. doi:10.1016/j.jri.2013.05.004
496. La Vignera S, Vicari E, Condorelli RA, Franchina C, Scalia G, Morgia G, et al. Prevalence of human papilloma virus infection in patients with male accessory gland infection. Reprod Biomed Online (2015) 30:385–91. doi:10.1016/j.rbmo.2014.12.016
497. Estemalik J, Demko C, Bissada NF, Joshi N, Bodner D, Shankar E, et al. Simultaneous detection of oral pathogens in subgingival plaque and prostatic fluid of men with periodontal and prostatic diseases. J Periodontol (2017) 88:823–29. doi:10.1902/jop.2017.160477
498. Hedger MP. Toll-like receptors and signalling in spermatogenesis and testicular responses to inflammation – a perspective. J Reprod Immunol (2011) 88:130–41. doi:10.1016/j.jri.2011.01.010
499. Bhushan S, Schuppe HC, Fijak M, Meinhardt A. Testicular infection: microorganisms, clinical implications and host-pathogen interaction. J Reprod Immunol (2009) 83:164–7. doi:10.1016/j.jri.2009.07.007
500. Bhushan S, Schuppe HC, Tchatalbachev S, Fijak M, Weidner W, Chakraborty T, et al. Testicular innate immune defense against bacteria. Mol Cell Endocrinol (2009) 306:37–44. doi:10.1016/j.mce.2008.10.017
501. Chen B, Yu L, Wang J, Li C, Zhao K, Zhang H. Involvement of prokineticin 2 and prokineticin receptor 1 in lipopolysaccharide-induced testitis in rats. Inflammation (2016) 39:534–42. doi:10.1007/s10753-015-0277-z
502. Lipsky BA. Prostatitis and urinary tract infection in men: what’s new; what’s true? Am J Med (1999) 106:327–34. doi:10.1016/S0002-9343(99)00017-0
503. Lipsky BA, Byren I, Hoey CT. Treatment of bacterial prostatitis. Clin Infect Dis (2010) 50:1641–52. doi:10.1086/652861
504. Vicari E, Calogero AE, Condorelli RA, Vicari LO, La Vignera S. Male accessory gland infection frequency in infertile patients with chronic microbial prostatitis and irritable bowel syndrome. Int J Androl (2012) 35:183–9. doi:10.1111/j.1365-2605.2011.01216.x
505. Wagenlehner FME, Pilatz A, Bschleipfer T, Diemer T, Linn T, Meinhardt A, et al. Bacterial prostatitis. World J Urol (2013) 31:711–6. doi:10.1007/s00345-013-1055-x
506. Krebs J, Bartel P, Pannek J. Bacterial persistence in the prostate after antibiotic treatment of chronic bacterial prostatitis in men with spinal cord injury. Urology (2014) 83:515–20. doi:10.1016/j.urology.2013.11.023
507. Krebs J, Bartel P, Pannek J. Chronic bacterial prostatitis in men with spinal cord injury. World J Urol (2014) 32:1579–85. doi:10.1007/s00345-013-1235-8
508. Wagenlehner FME, Weidner W, Pilatz A, Naber KG. Urinary tract infections and bacterial prostatitis in men. Curr Opin Infect Dis (2014) 27:97–101. doi:10.1097/QCO.0000000000000024
509. Videčnik Zorman J, Matičič M, Jeverica S, Smrkolj T. Diagnosis and treatment of bacterial prostatitis. Acta Dermatovenereol (2015) 24:25–9.
510. Condorelli RA, Vicari E, Mongioi LM, Russo GI, Morgia G, La Vignera S, et al. Human papilloma virus infection in patients with male accessory gland infection: usefulness of the ultrasound evaluation. Int J Endocrinol (2016) 2016:9174609. doi:10.1155/2016/9174609
511. Gill BC, Shoskes DA. Bacterial prostatitis. Curr Opin Infect Dis (2016) 29:86–91. doi:10.1097/QCO.0000000000000222
512. Krieger JN, Thumbikat P. Bacterial prostatitis: bacterial virulence, clinical outcomes, and new directions. Microbiol Spectr (2016) 4(1):UTI-0004-2012. doi:10.1128/microbiolspec.UTI-0004-2012
513. Alvarado-Esquivel C, Pacheco-Vega SJ, Hernández-Tinoco J, Centeno-Tinoco MM, Beristain-Garcia I, Sánchez-Anguiano LF, et al. Miscarriage history and Toxoplasma gondii infection: a cross-sectional study in women in Durango City, Mexico. Eur J Microbiol Immunol (Bp) (2014) 4:117–22. doi:10.1556/EuJMI.4.2014.2.4
514. Giakoumelou S, Wheelhouse N, Cuschieri K, Entrican G, Howie SEM, Horne AW. The role of infection in miscarriage. Hum Reprod Update (2015). doi:10.1093/humupd/dmv041
515. van der Eijk AA, Van Genderen PJ, Verdijk RM, Reusken CB, Mogling R, Van Kampen JJ, et al. Miscarriage associated with zika virus infection. N Engl J Med (2016) 375:1002–4. doi:10.1056/NEJMc1605898
516. McDonald HM, Chambers HM. Intrauterine infection and spontaneous midgestation abortion: is the spectrum of microorganisms similar to that in preterm labor? Infect Dis Obstet Gynecol (2000) 8:220–7. doi:10.1155/S1064744900000314
517. Romero R, Espinoza J, Mazor M. Can endometrial infection/inflammation explain implantation failure, spontaneous abortion, and preterm birth after in vitro fertilization? Fertil Steril (2004) 82:799–804. doi:10.1016/j.fertnstert.2004.05.076
518. Conde-Ferráez L, Chan May ADA, Carrillo-Martínez JR, Ayora-Talavera G, González-Losa MDR. Human papillomavirus infection and spontaneous abortion: a case-control study performed in Mexico. Eur J Obstet Gynecol Reprod Biol (2013) 170:468–73. doi:10.1016/j.ejogrb.2013.07.002
519. Wang H, Cao Q, Ge J, Liu C, Ma Y, Meng Y, et al. LncRNA-regulated infection and inflammation pathways associated with pregnancy loss: genome wide differential expression of lncRNAs in early spontaneous abortion. Am J Reprod Immunol (2014) 72:359–75. doi:10.1111/aji.12275
520. Ahmadi A, Khodabandehloo M, Ramazanzadeh R, Farhadifar F, Roshani D, Ghaderi E, et al. The relationship between Chlamydia trachomatis genital infection and spontaneous abortion. J Reprod Infertil (2016) 17:110–6.
521. Ambühl LMM, Baandrup U, Dybkaer K, Blaakaer J, Uldbjerg N, Sørensen S. Human papillomavirus infection as a possible cause of spontaneous abortion and spontaneous preterm delivery. Infect Dis Obstet Gynecol (2016) 2016:3086036. doi:10.1155/2016/3086036
522. Golding B, Scott DE, Scharf O, Huang LY, Zaitseva M, Lapham C, et al. Immunity and protection against Brucella abortus. Microbes Infect (2001) 3:43–8. doi:10.1016/S1286-4579(00)01350-2
523. Corbel MJ, Elberg SS, Cosivi O. Brucellosis in Humans and Animals. Geneva: World Health Organization (2006).
524. Oliveira SC, De Oliveira FS, Macedo GC, De Almeida LA, Carvalho NB. The role of innate immune receptors in the control of Brucella abortus infection: toll-like receptors and beyond. Microbes Infect (2008) 10:1005–9. doi:10.1016/j.micinf.2008.07.005
525. Kuster CE, Althouse GC. The impact of bacteriospermia on boar sperm storage and reproductive performance. Theriogenology (2016) 85:21–6. doi:10.1016/j.theriogenology.2015.09.049
526. Dorneles EMS, Sriranganathan N, Lage AP. Recent advances in Brucella abortus vaccines. Vet Res (2015) 46:76. doi:10.1186/s13567-015-0199-7
527. Brown VG, Schollum LM, Jarvis BDW. Microbiology of bovine semen and artificial breeding practices under New-Zealand conditions. NZ J Agric Res (1974) 17:431–2. doi:10.1080/00288233.1974.10421030
528. Schollum LM. The Microbiology of Bovine Serum and the Antimicrobial Activity of Bovine Seminal Plasma [PhD thesis]. Palmerston North: Massey University (1977).
529. Yániz JL, Marco-Aguado MA, Mateos JA, Santolaria P. Bacterial contamination of ram semen, antibiotic sensitivities, and effects on sperm quality during storage at 15 degrees C. Anim Reprod Sci (2010) 122:142–9. doi:10.1016/j.anireprosci.2010.08.006
530. Gaczarzewicz D, Udała J, Piasecka M, Błaszczyk B, Stankiewicz T. Bacterial contamination of boar semen and its relationship to sperm quality preserved in commercial extender containing gentamicin sulfate. Pol J Vet Sci (2016) 19:451–9. doi:10.1515/pjvs-2016-0057
531. Romero R, Mazor M, Wu YK, Sirtori M, Oyarzun E, Mitchell MD, et al. Infection in the pathogenesis of preterm labor. Semin Perinatol (1988) 12:262–79.
532. Toth M, Witkin SS, Ledger W, Thaler H. The role of infection in the etiology of preterm birth. Obstet Gynecol (1988) 71:723–6.
533. Cassell GH, Waites KB, Watson HL, Crouse DT, Harasawa R. Ureaplasma urealyticum intrauterine infection: role in prematurity and disease in newborns. Clin Microbiol Rev (1993) 6:69–87. doi:10.1128/CMR.6.1.69
534. McGregor JA, French JI, Jones W, Milligan K, Mckinney PJ, Patterson E, et al. Bacterial vaginosis is associated with prematurity and vaginal fluid mucinase and sialidase: results of a controlled trial of topical clindamycin cream. Am J Obstet Gynecol (1994) 170:1048–59; discussion 1059–60. doi:10.1016/S0002-9378(94)70098-2
535. Goldenberg RL, Hauth JC, Andrews WW. Intrauterine infection and preterm delivery. N Engl J Med (2000) 342:1500–7. doi:10.1056/NEJM200005183422007
536. Gonçalves LF, Chaiworapongsa T, Romero R. Intrauterine infection and prematurity. Ment Retard Dev Disabil Res Rev (2002) 8:3–13. doi:10.1002/mrdd.10008
537. Gerber S, Vial Y, Hohlfeld P, Witkin SS. Detection of Ureaplasma urealyticum in second-trimester amniotic fluid by polymerase chain reaction correlates with subsequent preterm labor and delivery. J Infect Dis (2003) 187:518–21. doi:10.1086/368205
538. Gardella C, Riley DE, Hitti J, Agnew K, Krieger JN, Eschenbach D. Identification and sequencing of bacterial rDNAs in culture-negative amniotic fluid from women in premature labor. Am J Perinatol (2004) 21:319–23. doi:10.1055/s-2004-831884
539. Espinoza J, Erez O, Romero R. Preconceptional antibiotic treatment to prevent preterm birth in women with a previous preterm delivery. Am J Obstet Gynecol (2006) 194:630–7. doi:10.1016/j.ajog.2005.11.050
540. Lee SE, Romero R, Kim CJ, Shim SS, Yoon BH. Funisitis in term pregnancy is associated with microbial invasion of the amniotic cavity and intra-amniotic inflammation. J Matern Fetal Neonatal Med (2006) 19:693–7. doi:10.1080/14767050600927353
541. Goldenberg RL, Culhane JF, Iams JD, Romero R. Epidemiology and causes of preterm birth. Lancet (2008) 371:75–84. doi:10.1016/S0140-6736(08)60074-4
542. Check JH. A practical approach to the prevention of miscarriage part 4-role of infection. Clin Exp Obstet Gyn (2010) 37:252–5.
543. Bastek JA, Gómez LM, Elovitz MA. The role of inflammation and infection in preterm birth. Clin Perinatol (2011) 38:385–406. doi:10.1016/j.clp.2011.06.003
544. Johnson HL, Ghanem KG, Zenilman JM, Erbelding EJ. Sexually transmitted infections and adverse pregnancy outcomes among women attending inner city public sexually transmitted diseases clinics. Sex Transm Dis (2011) 38:167–71. doi:10.1097/OLQ.0b013e3181f2e85f
545. Manzoni P, Rizzollo S, Decembrino L, Ruffinazzi G, Rossi Ricci A, Gallo E, et al. Recent advances in prevention of sepsis in the premature neonates in NICU. Early Hum Dev (2011) 87(Suppl 1):S31–3. doi:10.1016/j.earlhumdev.2011.01.008
546. Rours GIJG, Duijts L, Moll HA, Arends LR, De Groot R, Jaddoe VW, et al. Chlamydia trachomatis infection during pregnancy associated with preterm delivery: a population-based prospective cohort study. Eur J Epidemiol (2011) 26:493–502. doi:10.1007/s10654-011-9586-1
547. Jefferson KK. The bacterial etiology of preterm birth. Adv Appl Microbiol (2012) 80:1–22. doi:10.1016/B978-0-12-394381-1.00001-5
548. Lee SYR, Leung CW. Histological chorioamnionitis – implication for bacterial colonization, laboratory markers of infection, and early onset sepsis in very-low-birth-weight neonates. J Matern Fetal Neonatal Med (2012) 25:364–8. doi:10.3109/14767058.2011.579208
549. Shinar S, Skornick-Rapaport A, Rimon E. Placental abruption remote from term associated with Q fever infection. Obstet Gynecol (2012) 120:503–5. doi:10.1097/AOG.0b013e318260590f
550. Subramaniam A, Abramovici A, Andrews WW, Tita AT. Antimicrobials for preterm birth prevention: an overview. Infect Dis Obs Gynecol (2012). doi:10.1155/2012/157159
551. Aagaard K, Ma J, Antony KM, Ganu R, Petrosino J, Versalovic J. The placenta harbors a unique microbiome. Sci Transl Med (2014) 6:237ra265. doi:10.1126/scitranslmed.3008599
552. Joergensen JS, Kjaer Weile LK, Lamont RF. The early use of appropriate prophylactic antibiotics in susceptible women for the prevention of preterm birth of infectious etiology. Expert Opin Pharmacother (2014) 15:2173–91. doi:10.1517/14656566.2014.950225
553. Allen-Daniels MJ, Serrano MG, Pflugner LP, Fettweis JM, Prestosa MA, Koparde VN, et al. Identification of a gene in Mycoplasma hominis associated with preterm birth and microbial burden in intraamniotic infection. Am J Obstet Gynecol (2015) 212:779.e1–779.e13. doi:10.1016/j.ajog.2015.01.032
554. de Andrade Ramos B, Kanninen TT, Sisti G, Witkin SS. Microorganisms in the female genital tract during pregnancy: tolerance versus pathogenesis. Am J Reprod Immunol (2015) 73:383–9. doi:10.1111/aji.12326
555. Kacerovsky M, Vrbacky F, Kutova R, Pliskova L, Andrys C, Musilova I, et al. Cervical microbiota in women with preterm prelabor rupture of membranes. PLoS One (2015) 10:e0126884. doi:10.1371/journal.pone.0126884
556. Lamont RF. Advances in the prevention of infection-related preterm birth. Front Immunol (2015) 6:566. doi:10.3389/fimmu.2015.00566
557. Lis R, Rowhani-Rahbar A, Manhart LE. Mycoplasma genitalium infection and female reproductive tract disease: a meta-analysis. Clin Infect Dis (2015) 61:418–26. doi:10.1093/cid/civ312
558. Pammi M, Weisman LE. Late-onset sepsis in preterm infants: update on strategies for therapy and prevention. Expert Rev Anti Infect Ther (2015) 13:487–504. doi:10.1586/14787210.2015.1008450
559. Ueno T, Niimi H, Yoneda N, Yoneda S, Mori M, Tabata H, et al. Eukaryote-made thermostable DNA polymerase enables rapid PCR-based detection of mycoplasma, ureaplasma and other bacteria in the amniotic fluid of preterm labor cases. PLoS One (2015) 10:e0129032. doi:10.1371/journal.pone.0129032
560. Frey HA, Klebanoff MA. The epidemiology, etiology, and costs of preterm birth. Semin Fetal Neonatal Med (2016) 21:68–73. doi:10.1016/j.siny.2015.12.011
561. Nadeau HCG, Subramaniam A, Andrews WW. Infection and preterm birth. Semin Fetal Neonatal Med (2016) 21:100–5. doi:10.1016/j.siny.2015.12.008
562. Vinturache AE, Gyamfi-Bannerman C, Hwang J, Mysorekar IU, Jacobsson B; Preterm Birth International Collaborative (Prebic). Maternal microbiome – a pathway to preterm birth. Semin Fetal Neonatal Med (2016) 21:94–9. doi:10.1016/j.siny.2016.02.004
563. Yoneda S, Shiozaki A, Yoneda N, Ito M, Shima T, Fukuda K, et al. Antibiotic therapy increases the risk of preterm birth in preterm labor without intra-amniotic microbes, but may prolong the gestation period in preterm labor with microbes, evaluated by rapid and high-sensitive PCR system. Am J Reprod Immunol (2016) 75:440–50. doi:10.1111/aji.12484
564. García-Velasco JA, Menabrito M, Catalán IB. What fertility specialists should know about the vaginal microbiome: a review. Reprod Biomed Online (2017) 35:103–12. doi:10.1016/j.rbmo.2017.04.005
565. van Well GTJ, Daalderop LA, Wolfs T, Kramer BW. Human perinatal immunity in physiological conditions and during infection. Mol Cell Pediatr (2017) 4:4. doi:10.1186/s40348-017-0070-1
566. Zini A, Boman JM, Belzile E, Ciampi A. Sperm DNA damage is associated with an increased risk of pregnancy loss after IVF and ICSI: systematic review and meta-analysis. Hum Reprod (2008) 23:2663–8. doi:10.1093/humrep/den321
567. Gil-Villa AM, Cardona-Maya W, Agarwal A, Sharma R, Cadavid Á. Assessment of sperm factors possibly involved in early recurrent pregnancy loss. Fertil Steril (2010) 94:1465–72. doi:10.1016/j.fertnstert.2009.05.042
568. Brahem S, Mehdi M, Landolsi H, Mougou S, Elghezal H, Saad A. Semen parameters and sperm DNA fragmentation as causes of recurrent pregnancy loss. Urology (2011) 78:792–6. doi:10.1016/j.urology.2011.05.049
569. Shina A, Carp HJA. Recurrent pregnancy loss – beyond evidence based medicine. Gynecol Endocrinol (2012) 28:991–2. doi:10.3109/09513590.2012.683083
570. Lewis SEM, John Aitken R, Conner SJ, Iuliis GD, Evenson DP, Henkel R, et al. The impact of sperm DNA damage in assisted conception and beyond: recent advances in diagnosis and treatment. Reprod Biomed Online (2013) 27:325–37. doi:10.1016/j.rbmo.2013.06.014
571. Simon L, Proutski I, Stevenson M, Jennings D, Mcmanus J, Lutton D, et al. Sperm DNA damage has a negative association with live-birth rates after IVF. Reprod Biomed Online (2013) 26:68–78. doi:10.1016/j.rbmo.2012.09.019
572. Wang R, Zhou H, Zhang Z, Dai R, Geng D, Liu R. The impact of semen quality, occupational exposure to environmental factors and lifestyle on recurrent pregnancy loss. J Assist Reprod Genet (2013) 30:1513–8. doi:10.1007/s10815-013-0091-1
573. Belloc S, Benkhalifa M, Cohen-Bacrie M, Dalleac A, Chahine H, Amar E, et al. Which isolated sperm abnormality is most related to sperm DNA damage in men presenting for infertility evaluation. J Assist Reprod Genet (2014) 31:527–32. doi:10.1007/s10815-014-0194-3
574. Bonney EA, Brown SA. To drive or be driven: the path of a mouse model of recurrent pregnancy loss. Reproduction (2014) 147:R153–67. doi:10.1530/REP-13-0583
575. Kavitha P, Malini SS. Positive association of sperm dysfunction in the pathogenesis of recurrent pregnancy loss. J Clin Diagn Res (2014) 8:OC07–10. doi:10.7860/JCDR/2014/9109.5172
576. Zhao J, Zhang Q, Wang Y, Li Y. Whether sperm deoxyribonucleic acid fragmentation has an effect on pregnancy and miscarriage after in vitro fertilization/intracytoplasmic sperm injection: a systematic review and meta-analysis. Fertil Steril (2014) 102:998–1005.e1008. doi:10.1016/j.fertnstert.2014.06.033
577. Bronson R, editor. The Male Role in Pregnancy Loss and Embryo Implantation Failure. Berlin: Springer (2015).
578. Esteves SC, Sánchez-Martin F, Sánchez-Martin P, Schneider DT, Gosálvez J. Comparison of reproductive outcome in oligozoospermic men with high sperm DNA fragmentation undergoing intracytoplasmic sperm injection with ejaculated and testicular sperm. Fertil Steril (2015) 104:1398–405. doi:10.1016/j.fertnstert.2015.08.028
579. Zidi-Jrah I, Hajlaoui A, Mougou-Zerelli S, Kammoun M, Meniaoui I, Sallem A, et al. Relationship between sperm aneuploidy, sperm DNA integrity, chromatin packaging, traditional semen parameters, and recurrent pregnancy loss. Fertil Steril (2016) 105:58–64. doi:10.1016/j.fertnstert.2015.09.041
580. Simon L, Zini A, Dyachenko A, Ciampi A, Carrell DT. A systematic review and meta-analysis to determine the effect of sperm DNA damage on in vitro fertilization and intracytoplasmic sperm injection outcome. Asian J Androl (2017) 19:80–90. doi:10.4103/1008-682X.182822
581. Agarwal A, Majzoub A, Esteves SC, Ko E, Ramasamy R, Zini A. Clinical utility of sperm DNA fragmentation testing: practice recommendations based on clinical scenarios. Transl Androl Urol (2016) 5:935–50. doi:10.21037/tau.2016.10.03
582. Usmani S, Liu HC, Pilcher CD, Witkowska HE, Kirchhoff F, Greene WC, et al. HIV-enhancing amyloids are prevalent in fresh semen and are a determinant for semen’s ability to enhance HIV infection: relevance for HIV transmission. AIDS Res Hum Retroviruses (2014) 30:A183–4. doi:10.1089/aid.2014.5392.abstract
583. Binder NK, Sheedy JR, Hannan NJ, Gardner DK. Male obesity is associated with changed spermatozoa Cox4i1 mRNA level and altered seminal vesicle fluid composition in a mouse model. Mol Hum Reprod (2015) 21:424–34. doi:10.1093/molehr/gav010
584. Gil-Villa AM, Cardona-Maya W, Agarwal A, Sharma R, Cadavid Á. Role of male factor in early recurrent embryo loss: do antioxidants have any effect? Fertil Steril (2009) 92:565–71. doi:10.1016/j.fertnstert.2008.07.1715
585. Shiva M, Gautam AK, Verma Y, Shivgotra V, Doshi H, Kumar S. Association between sperm quality, oxidative stress, and seminal antioxidant activity. Clin Biochem (2011) 44:319–24. doi:10.1016/j.clinbiochem.2010.11.009
586. Agarwal A, Durairajanayagam D, Halabi J, Peng J, Vazquez-Levin M. Proteomics, oxidative stress and male infertility. Reprod Biomed Online (2014) 29:32–58. doi:10.1016/j.rbmo.2014.02.013
587. Durairajanayagam D, Agarwal A, Ong C, Prashast P. Lycopene and male infertility. Asian J Androl (2014) 16:420–5. doi:10.4103/1008-682X.126384
588. Ko EY, Sabanegh ES Jr, Agarwal A. Male infertility testing: reactive oxygen species and antioxidant capacity. Fertil Steril (2014) 102:1518–27. doi:10.1016/j.fertnstert.2014.10.020
589. Cruz DF, Lume C, Silva JV, Nunes A, Castro I, Silva R, et al. Oxidative stress markers: can they be used to evaluate human sperm quality? Turk J Urol (2015) 41:198–207. doi:10.5152/tud.2015.06887
590. Agarwal A, Roychoudhury S, Sharma R, Gupta S, Majzoub A, Sabanegh E. Diagnostic application of oxidation-reduction potential assay for measurement of oxidative stress: clinical utility in male factor infertility. Reprod Biomed Online (2017) 34:48–57. doi:10.1016/j.rbmo.2016.10.008
591. Agarwal A, Wang SM. Clinical relevance of oxidation-reduction potential in the evaluation of male infertility. Urology (2017) 104:84–9. doi:10.1016/j.urology.2017.02.016
592. Bouziat R, Hinterleitner R, Brown JJ, Stencel-Baerenwald JE, Ikizler M, Mayassi T, et al. Reovirus infection triggers inflammatory responses to dietary antigens and development of celiac disease. Science (2017) 356:44–50. doi:10.1126/science.aah5298
593. Verdu EF, Caminero A. How infection can incite sensitivity to food. Science (2017) 356:29–30. doi:10.1126/science.aan1500
594. Ludvigsson JF, Montgomery SM, Ekbom A. Celiac disease and risk of adverse fetal outcome: a population-based cohort study. Gastroenterology (2005) 129:454–63. doi:10.1016/j.gastro.2005.05.065
595. Wolf H, Ilsen A, Van Pampus MG, Sahebdien S, Pena S, Von Blomberg ME. Celiac serology in women with severe pre-eclampsia or delivery of a small for gestational age neonate. Int J Gynaecol Obstet (2008) 103:175–7. doi:10.1016/j.ijgo.2008.05.024
596. Bast A, O’Bryan T, Bast E. Celiac disease and reproductive health. Practical Gastroenterol (2009):10–21.
597. Özgör B, Selimoğlu MA. Coeliac disease and reproductive disorders. Scand J Gastroenterol (2010) 45:395–402. doi:10.3109/00365520903508902
598. Soni S, Badawy SZ. Celiac disease and its effect on human reproduction: a review. J Reprod Med (2010) 55:3–8.
599. Tersigni C, Castellani R, De Waure C, Fattorossi A, De Spirito M, Gasbarrini A, et al. Celiac disease and reproductive disorders: meta-analysis of epidemiologic associations and potential pathogenic mechanisms. Hum Reprod Update (2014) 20:582–93. doi:10.1093/humupd/dmu007
600. Moleski SM, Lindenmeyer CC, Veloski JJ, Miller RS, Miller CL, Kastenberg D, et al. Increased rates of pregnancy complications in women with celiac disease. Ann Gastroenterol (2015) 28:236–40.
601. Saccone G, Berghella V, Sarno L, Maruotti GM, Cetin I, Greco L, et al. Celiac disease and obstetric complications: a systematic review and metaanalysis. Am J Obstet Gynecol (2016) 214:225–34. doi:10.1016/j.ajog.2015.09.080
602. Byberg KK, Ogland B, Eide GE, Øymar K. Birth after preeclamptic pregnancies: association with allergic sensitization and allergic rhinoconjunctivitis in late childhood; a historically matched cohort study. BMC Pediatr (2014) 14:101. doi:10.1186/1471-2431-14-101
603. Liu AH. Revisiting the hygiene hypothesis for allergy and asthma. J Allergy Clin Immunol (2015) 136:860–5. doi:10.1016/j.jaci.2015.08.012
604. Stokholm J, Sevelsted A, Anderson UD, Bisgaard H. Preeclampsia associates with asthma, allergy, and eczema in childhood. Am J Respir Crit Care Med (2017) 195:614–21. doi:10.1164/rccm.201604-0806OC
605. Omer SB, Goodman D, Steinhoff MC, Rochat R, Klugman KP, Stoll BJ, et al. Maternal influenza immunization and reduced likelihood of prematurity and small for gestational age births: a retrospective cohort study. PLoS Med (2011) 8:e1000441. doi:10.1371/journal.pmed.1000441
606. Adedinsewo DA, Noory L, Bednarczyk RA, Steinhoff MC, Davis R, Ogbuanu C, et al. Impact of maternal characteristics on the effect of maternal influenza vaccination on fetal outcomes. Vaccine (2013) 31:5827–33. doi:10.1016/j.vaccine.2013.09.071
607. Richards JL, Hansen C, Bredfeldt C, Bednarczyk RA, Steinhoff MC, Adjaye-Gbewonyo D, et al. Neonatal outcomes after antenatal influenza immunization during the 2009 H1N1 influenza pandemic: impact on preterm birth, birth weight, and small for gestational age birth. Clin Infect Dis (2013) 56:1216–22. doi:10.1093/cid/cit045
608. Olsen SJ, Mirza SA, Vonglokham P, Khanthamaly V, Chitry B, Pholsena V, et al. The effect of influenza vaccination on birth outcomes in a cohort of pregnant women in Lao PDR, 2014–2015. Clin Infect Dis (2016) 63:487–94. doi:10.1093/cid/ciw290
609. Phadke VK, Steinhoff MC, Omer SB, Macdonald NE. Maternal influenza immunization and adverse birth outcomes: using data and practice to inform theory and research design. Am J Epidemiol (2016) 184:789–92. doi:10.1093/aje/kww110
610. Leslie M. Can flu shots help women get pregnant? Science (2017) 355:1247–8. doi:10.1126/science.355.6331.1247
611. Nordin JD, Kharbanda EO, Vazquez Benitez G, Lipkind H, Vellozzi C, Destefano F, et al. Maternal influenza vaccine and risks for preterm or small for gestational age birth. J Pediatr (2014) 164:1051–7.e1052. doi:10.1016/j.jpeds.2014.01.037
612. Bratton KN, Wardle MT, Orenstein WA, Omer SB. Maternal influenza immunization and birth outcomes of stillbirth and spontaneous abortion: a systematic review and meta-analysis. Clin Infect Dis (2015) 60:e11–9. doi:10.1093/cid/ciu915
613. Coenders A, Koopmans NK, Broekhuijsen K, Groen H, Karstenberg-Kramer JMA, Van Goor K, et al. Adjuvanted vaccines in pregnancy: no evidence for effect of the adjuvanted H1N1/09 vaccination on occurrence of preeclampsia or intra-uterine growth restriction. Eur J Obstet Gynecol Reprod Biol (2015) 187:14–9. doi:10.1016/j.ejogrb.2015.01.011
614. Munoz FM, Greisinger AJ, Wehmanen OA, Mouzoon ME, Hoyle JC, Smith FA, et al. Safety of influenza vaccination during pregnancy. Am J Obstet Gynecol (2005) 192:1098–106. doi:10.1016/j.ajog.2004.12.019
615. Mak TK, Mangtani P, Leese J, Watson JM, Pfeifer D. Influenza vaccination in pregnancy: current evidence and selected national policies. Lancet Infect Dis (2008) 8:44–52. doi:10.1016/S1473-3099(07)70311-0
616. Tamma PD, Ault KA, Del Rio C, Steinhoff MC, Halsey NA, Omer SB. Safety of influenza vaccination during pregnancy. Am J Obstet Gynecol (2009) 201:547–52. doi:10.1016/j.ajog.2009.09.034
617. Yamaguchi K, Hisano M, Isojima S, Irie S, Arata N, Watanabe N, et al. Relationship of Th1/Th2 cell balance with the immune response to influenza vaccine during pregnancy. J Med Virol (2009) 81:1923–8. doi:10.1002/jmv.21620
618. Bednarczyk RA, Adjaye-Gbewonyo D, Omer SB. Safety of influenza immunization during pregnancy for the fetus and the neonate. Am J Obstet Gynecol (2012) 207:S38–46. doi:10.1016/j.ajog.2012.07.002
619. Jamieson DJ, Kissin DM, Bridges CB, Rasmussen SA. Benefits of influenza vaccination during pregnancy for pregnant women. Am J Obstet Gynecol (2012) 207:S17–20. doi:10.1016/j.ajog.2012.06.070
620. Kharbanda EO, Vazquez-Benitez G, Shi WX, Lipkind H, Naleway A, Molitor B, et al. Assessing the safety of influenza immunization during pregnancy: the Vaccine Safety Datalink. Am J Obstet Gynecol (2012) 207:S47–51. doi:10.1016/j.ajog.2012.06.073
621. Moro PL, Tepper NK, Grohskopf LA, Vellozzi C, Broder K. Safety of seasonal influenza and influenza A (H1N1) 2009 monovalent vaccines in pregnancy. Expert Rev Vaccines (2012) 11:911–21. doi:10.1586/erv.12.72
622. Pasternak B, Svanström H, Mølgaard-Nielsen D, Krause TG, Emborg HD, Melbye M, et al. Risk of adverse fetal outcomes following administration of a pandemic influenza A(H1N1) vaccine during pregnancy. JAMA (2012) 308:165–74. doi:10.1001/jama.2012.6131
623. Pasternak B, Svanström H, Mølgaard-Nielsen D, Krause TG, Emborg HD, Melbye M, et al. Vaccination against pandemic A/H1N1 2009 influenza in pregnancy and risk of fetal death: cohort study in Denmark. BMJ (2012) 344:e2794. doi:10.1136/bmj.e2794
624. Beau AB, Hurault-Delarue C, Vidal S, Guitard C, Vayssière C, Petiot D, et al. Pandemic A/H1N1 influenza vaccination during pregnancy: a comparative study using the EFEMERIS database. Vaccine (2014) 32:1254–8. doi:10.1016/j.vaccine.2014.01.021
625. Keller-Stanislawski B, Englund JA, Kang G, Mangtani P, Neuzil K, Nohynek H, et al. Safety of immunization during pregnancy: a review of the evidence of selected inactivated and live attenuated vaccines. Vaccine (2014) 32:7057–64. doi:10.1016/j.vaccine.2014.09.052
626. Naleway AL, Irving SA, Henninger ML, Li DK, Shifflett P, Ball S, et al. Safety of influenza vaccination during pregnancy: a review of subsequent maternal obstetric events and findings from two recent cohort studies. Vaccine (2014) 32:3122–7. doi:10.1016/j.vaccine.2014.04.021
627. Vaughn DW, Seifert H, Hepburn A, Dewe W, Li P, Drame M, et al. Safety of AS03-adjuvanted inactivated split virion A(H1N1)pdm09 and H5N1 influenza virus vaccines administered to adults: pooled analysis of 28 clinical trials. Hum Vaccin Immunother (2014) 10:2942–57. doi:10.4161/21645515.2014.972149
628. Baum U, Leino T, Gissler M, Kilpi T, Jokinen J. Perinatal survival and health after maternal influenza A(H1N1)pdm09 vaccination: a cohort study of pregnancies stratified by trimester of vaccination. Vaccine (2015) 33:4850–7. doi:10.1016/j.vaccine.2015.07.061
629. Fabiani M, Bella A, Rota MC, Clagnan E, Gallo T, D’amato M, et al. A/H1N1 pandemic influenza vaccination: a retrospective evaluation of adverse maternal, fetal and neonatal outcomes in a cohort of pregnant women in Italy. Vaccine (2015) 33:2240–7. doi:10.1016/j.vaccine.2015.03.041
630. Fell DB, Platt RW, Lanes A, Wilson K, Kaufman JS, Basso O, et al. Fetal death and preterm birth associated with maternal influenza vaccination: systematic review. BJOG (2015) 122:17–26. doi:10.1111/1471-0528.12977
631. Ludvigsson JF, Ström P, Lundholm C, Cnattingius S, Ekbom A, Örtqvist Å, et al. Maternal vaccination against H1N1 influenza and offspring mortality: population based cohort study and sibling design. BMJ (2015) 351:h5585. doi:10.1136/bmj.h5585
632. Savitz DA, Fell DB, Ortiz JR, Bhat N. Does influenza vaccination improve pregnancy outcome? Methodological issues and research needs. Vaccine (2015) 33:6430–5. doi:10.1016/j.vaccine.2015.08.041
633. Walls T, Graham P, Petousis-Harris H, Hill L, Austin N. Infant outcomes after exposure to Tdap vaccine in pregnancy: an observational study. BMJ Open (2016) 6:e009536. doi:10.1136/bmjopen-2015-009536
634. Donegan K, King B, Bryan P. Safety of pertussis vaccination in pregnant women in UK: observational study. BMJ (2014) 349:g4219. doi:10.1136/bmj.g4219
635. Kharbanda EO, Vazquez-Benitez G, Lipkind HS, Klein NP, Cheetham TC, Naleway AL, et al. Maternal Tdap vaccination: coverage and acute safety outcomes in the vaccine safety datalink, 2007–2013. Vaccine (2016) 34:968–73. doi:10.1016/j.vaccine.2015.12.046
636. Petousis-Harris H, Walls T, Watson D, Paynter J, Graham P, Turner N. Safety of Tdap vaccine in pregnant women: an observational study. BMJ Open (2016) 6:e010911. doi:10.1136/bmjopen-2015-010911
637. Wheeler CM, Skinner SR, Del Rosario-Raymundo MR, Garland SM, Chatterjee A, Lazcano-Ponce E, et al. Efficacy, safety, and immunogenicity of the human papillomavirus 16/18 AS04-adjuvanted vaccine in women older than 25 years: 7-year follow-up of the phase 3, double-blind, randomised controlled VIVIANE study. Lancet Infect Dis (2016) 16:1154–68. doi:10.1016/S1473-3099(16)30120-7
638. Raj RS, Bonney EA, Phillippe M. Influenza, immune system, and pregnancy. Reprod Sci (2014) 21:1434–51. doi:10.1177/1933719114537720
639. Staff AC, Benton SJ, Von Dadelszen P, Roberts JM, Taylor RN, Powers RW, et al. Redefining preeclampsia using placenta-derived biomarkers. Hypertension (2013) 61:932–42. doi:10.1161/HYPERTENSIONAHA.111.00250
640. Scheminske M, Henninger M, Irving SA, Thompson M, Williams J, Shifflett P, et al. The association between influenza vaccination and other preventative health behaviors in a cohort of pregnant women. Health Educ Behav (2015) 42:402–8. doi:10.1177/1090198114560021
641. Steinhoff MC, Omer SB, Roy E, El Arifeen S, Raqib R, Dodd C, et al. Neonatal outcomes after influenza immunization during pregnancy: a randomized controlled trial. CMAJ (2012) 184:645–53. doi:10.1503/cmaj.110754
642. Porter TF, Lacoursiere Y, Scott JR. Immunotherapy for recurrent miscarriage. Cochrane Database Syst Rev (2006):CD000112. doi:10.1002/14651858.CD000112.pub2
643. Wong LF, Porter TF, Scott JR. Immunotherapy for recurrent miscarriage. Cochrane Database Syst Rev (2014):CD000112. doi:10.1002/14651858.CD000112.pub3
644. Jørgensen KT, Pedersen BV, Jacobsen S, Biggar RJ, Frisch M. National cohort study of reproductive risk factors for rheumatoid arthritis in Denmark: a role for hyperemesis, gestational hypertension and pre-eclampsia? Ann Rheum Dis (2010) 69:358–63. doi:10.1136/ard.2008.099945
645. Ebringer A, Rashid T, Wilson C. Rheumatoid arthritis, Proteus, anti-CCP antibodies and Karl Popper. Autoimmun Rev (2010) 9:216–23. doi:10.1016/j.autrev.2009.10.006
647. Ebringer A, Rashid T. Rheumatoid arthritis is caused by a Proteus urinary tract infection. APMIS (2014) 122:363–8. doi:10.1111/apm.12154
648. Pretorius E, Akeredolu O-O, Soma P, Kell DB. Major involvement of bacterial components in rheumatoid arthritis and its accompanying oxidative stress, systemic inflammation and hypercoagulability. Exp Biol Med (2017) 242:355–73. doi:10.1177/1535370216681549
649. Wang G, Li X, Wang Z. APD3: the antimicrobial peptide database as a tool for research and education. Nucleic Acids Res (2016) 44:D1087–93. doi:10.1093/nar/gkv1278
650. Zasloff M. Antimicrobial peptides of multicellular organisms. Nature (2002) 415:389–95. doi:10.1038/415389a
651. Auvynet C, Rosenstein Y. Multifunctional host defense peptides: antimicrobial peptides, the small yet big players in innate and adaptive immunity. FEBS J (2009) 276:6497–508. doi:10.1111/j.1742-4658.2009.07360.x
652. Gustafsson A, Olin AI, Ljunggren L. LPS interactions with immobilized and soluble antimicrobial peptides. Scand J Clin Lab Invest (2010) 70:194–200. doi:10.3109/00365511003663622
653. Lee SH, Jun HK, Lee HR, Chung CP, Choi BK. Antibacterial and lipopolysaccharide (LPS)-neutralising activity of human cationic antimicrobial peptides against periodontopathogens. Int J Antimicrob Agents (2010) 35:138–45. doi:10.1016/j.ijantimicag.2009.09.024
654. Kościuczuk EM, Lisowski P, Jarczak J, Strzałkowska N, Jóźwik A, Horbańczuk J, et al. Cathelicidins: family of antimicrobial peptides. A review. Mol Biol Rep (2012) 39:10957–70. doi:10.1007/s11033-012-1997-x
655. Seo MD, Won HS, Kim JH, Mishig-Ochir T, Lee BJ. Antimicrobial peptides for therapeutic applications: a review. Molecules (2012) 17:12276–86. doi:10.3390/molecules171012276
656. Zhao J, Zhao C, Liang G, Zhang M, Zheng J. Engineering antimicrobial peptides with improved antimicrobial and hemolytic activities. J Chem Inf Model (2013) 53:3280–96. doi:10.1021/ci400477e
657. Ashby M, Petkova A, Hilpert K. Cationic antimicrobial peptides as potential new therapeutic agents in neonates and children: a review. Curr Opin Infect Dis (2014) 27:258–67. doi:10.1097/QCO.0000000000000057
658. Waghu FH, Gopi L, Barai RS, Ramteke P, Nizami B, Idicula-Thomas S. CAMP: collection of sequences and structures of antimicrobial peptides. Nucleic Acids Res (2014) 42:D1154–8. doi:10.1093/nar/gkt1157
659. Wang G. Human antimicrobial peptides and proteins. Pharmaceuticals (Basel) (2014) 7:545–94. doi:10.3390/ph7050545
660. Kido EA, Pandolfi V, Houllou-Kido LM, Andrade PP, Marcelino FC, Nepomuceno AL, et al. Plant antimicrobial peptides: an overview of SuperSAGE transcriptional profile and a functional review. Curr Protein Pept Sci (2010) 11:220–30. doi:10.2174/138920310791112110
661. Li W, Tailhades J, O’brien-Simpson NM, Separovic F, Otvos L Jr, Hossain MA, et al. Proline-rich antimicrobial peptides: potential therapeutics against antibiotic-resistant bacteria. Amino Acids (2014) 46:2287–94. doi:10.1007/s00726-014-1820-1
662. Kosikowska P, Lesner A. Antimicrobial peptides (AMPs) as drug candidates: a patent review (2003–2015). Expert Opin Ther Pat (2016) 26:689–702. doi:10.1080/13543776.2016.1176149
663. Kang HK, Kim C, Seo CH, Park Y. The therapeutic applications of antimicrobial peptides (AMPs): a patent review. J Microbiol (2017) 55:1–12. doi:10.1007/s12275-017-6452-1
664. Yoshio H, Tollin M, Gudmundsson GH, Lagercrantz H, Jornvall H, Marchini G, et al. Antimicrobial polypeptides of human vernix caseosa and amniotic fluid: implications for newborn innate defense. Pediatr Res (2003) 53:211–6. doi:10.1203/01.PDR.0000047471.47777.B0
665. Frew L, Stock SJ. Antimicrobial peptides and pregnancy. Reproduction (2011) 141:725–35. doi:10.1530/REP-10-0537
666. Kai-Larsen Y, Gudmundsson GH, Agerberth B. A review of the innate immune defence of the human foetus and newborn, with the emphasis on antimicrobial peptides. Acta Paediatr (2014) 103:1000–8. doi:10.1111/apa.12700
667. Tribe RM. Small peptides with a big role: antimicrobial peptides in the pregnant female reproductive tract. Am J Reprod Immunol (2015) 74:123–5. doi:10.1111/aji.12379
668. Yarbrough VL, Winkle S, Herbst-Kralovetz MM. Antimicrobial peptides in the female reproductive tract: a critical component of the mucosal immune barrier with physiological and clinical implications. Hum Reprod Update (2015) 21:353–77. doi:10.1093/humupd/dmu065
669. Yedery RD, Reddy KVR. Antimicrobial peptides as microbicidal contraceptives: prophecies for prophylactics – a mini review. Eur J Contracept Reprod Health Care (2005) 10:32–42. doi:10.1080/13625180500035124
670. Zairi A, Tangy F, Bouassida K, Hani K. Dermaseptins and magainins: antimicrobial peptides from frogs’ skin-new sources for a promising spermicides microbicides-a mini review. J Biomed Biotechnol (2009) 2009:452567. doi:10.1155/2009/452567
671. Schulze M, Junkes C, Mueller P, Speck S, Ruediger K, Dathe M, et al. Effects of cationic antimicrobial peptides on liquid-preserved boar spermatozoa. PLoS One (2014) 9:e100490. doi:10.1371/journal.pone.0100490
672. Speck S, Courtiol A, Junkes C, Dathe M, Müller K, Schulze M. Cationic synthetic peptides: assessment of their antimicrobial potency in liquid preserved boar semen. PLoS One (2014) 9:e105949. doi:10.1371/journal.pone.0105949
673. Schulze M, Grobbel M, Müller K, Junkes C, Dathe M, Rüdiger K, et al. Challenges and limits using antimicrobial peptides in boar semen preservation. Reprod Domest Anim (2015) 50(Suppl 2):5–10. doi:10.1111/rda.12553
674. Schulze M, Dathe M, Waberski D, Müller K. Liquid storage of boar semen: current and future perspectives on the use of cationic antimicrobial peptides to replace antibiotics in semen extenders. Theriogenology (2016) 85:39–46. doi:10.1016/j.theriogenology.2015.07.016
675. Bussalleu E, Sancho S, Briz MD, Yeste M, Bonet S. Do antimicrobial peptides PR-39, PMAP-36 and PMAP-37 have any effect on bacterial growth and quality of liquid-stored boar semen? Theriogenology (2017) 89:235–43. doi:10.1016/j.theriogenology.2016.11.017
676. Easterhoff D, Ontiveros F, Brooks LR, Kim Y, Ross B, Silva JN, et al. Semen-derived enhancer of viral infection (SEVI) binds bacteria, enhances bacterial phagocytosis by macrophages, and can protect against vaginal infection by a sexually transmitted bacterial pathogen. Antimicrob Agents Chemother (2013) 57:2443–50. doi:10.1128/AAC.02464-12
677. Edström AML, Malm J, Frohm B, Martellini JA, Giwercman A, Mörgelin M, et al. The major bactericidal activity of human seminal plasma is zinc-dependent and derived from fragmentation of the semenogelins. J Immunol (2008) 181:3413–21. doi:10.4049/jimmunol.181.5.3413
678. Zhao H, Lee WH, Shen JH, Li H, Zhang Y. Identification of novel semenogelin I-derived antimicrobial peptide from liquefied human seminal plasma. Peptides (2008) 29:505–11. doi:10.1016/j.peptides.2008.01.009
679. Yenugu S, Hamil KG, Birse CE, Ruben SM, French FS, Hall SH. Antibacterial properties of the sperm-binding proteins and peptides of human epididymis 2 (HE2) family; salt sensitivity, structural dependence and their interaction with outer and cytoplasmic membranes of Escherichia coli. Biochem J (2003) 372:473–83. doi:10.1042/BJ20030225
680. Avellar MCW, Honda L, Hamil KG, Yenugu S, Grossman G, Petrusz P, et al. Differential expression and antibacterial activity of epididymis protein 2 isoforms in the male reproductive tract of human and rhesus monkey (Macaca mulatta). Biol Reprod (2004) 71:1453–60. doi:10.1095/biolreprod.104.031740
681. Sørensen OE, Gram L, Johnsen AH, Andersson E, Bangsbøll S, Tjabringa GS, et al. Processing of seminal plasma hCAP-18 to ALL-38 by gastricsin: a novel mechanism of generating antimicrobial peptides in vagina. J Biol Chem (2003) 278:28540–6. doi:10.1074/jbc.M301608200
683. Ayres JS, Freitag N, Schneider DS. Identification of Drosophila mutants altering defense of and endurance to Listeria monocytogenes infection. Genetics (2008) 178:1807–15. doi:10.1534/genetics.107.083782
684. Schneider DS, Ayres JS. Two ways to survive infection: what resistance and tolerance can teach us about treating infectious diseases. Nat Rev Immunol (2008) 8:889–95. doi:10.1038/nri2432
685. Råberg L, Graham AL, Read AF. Decomposing health: tolerance and resistance to parasites in animals. Philos Trans R Soc Lond B Biol Sci (2009) 364:37–49. doi:10.1098/rstb.2008.0184
686. Ayres JS, Schneider DS. Tolerance of infections. Annu Rev Immunol (2012) 30:271–94. doi:10.1146/annurev-immunol-020711-075030
687. Medzhitov R, Schneider DS, Soares MP. Disease tolerance as a defense strategy. Science (2012) 335:936–41. doi:10.1126/science.1214935
688. Råberg L. How to live with the enemy: understanding tolerance to parasites. PLoS Biol (2014) 12:e1001989. doi:10.1371/journal.pbio.1001989
689. Palaferri Schieber AM, Lee YM, Chang MW, Leblanc M, Collins B, Downes M, et al. Disease tolerance mediated by microbiome E. coli involves inflammasome and IGF-1 signaling. Science (2015) 350:558–63. doi:10.1126/science.aac6468
690. Kogut MH, Arsenault RJ. Immunometabolic phenotype alterations associated with the induction of disease tolerance and persistent asymptomatic infection of Salmonella in the chicken intestine. Front Immunol (2017) 8:372. doi:10.3389/fimmu.2017.00372
691. Meunier I, Kaufmann E, Downey J, Divangahi M. Unravelling the networks dictating host resistance versus tolerance during pulmonary infections. Cell Tissue Res (2017) 367:525–36. doi:10.1007/s00441-017-2572-5
692. Longo VD, Finch CE. Evolutionary medicine: from dwarf model systems to healthy centenarians? Science (2003) 299:1342–6. doi:10.1126/science.1077991
693. Gluckman P, Beedle A, Hanson M. Principles of Evolutionary Medicine. Oxford: Oxford University Press (2009).
694. Rühli FJ, Henneberg M. New perspectives on evolutionary medicine: the relevance of microevolution for human health and disease. BMC Med (2013) 11:115. doi:10.1186/1741-7015-11-115
695. Svensson EI, Råberg L. Resistance and tolerance in animal enemy-victim coevolution. Trends Ecol Evol (2010) 25:267–74. doi:10.1016/j.tree.2009.12.005
696. Ayres JS. Cooperative microbial tolerance behaviors in host-microbiota mutualism. Cell (2016) 165:1323–31. doi:10.1016/j.cell.2016.05.049
697. Ayres JS. Microbes dress for success: tolerance or resistance? Trends Microbiol (2017) 25:1–3. doi:10.1016/j.tim.2016.11.006
698. Rangan KJ, Pedicord VA, Wang YC, Kim B, Lu Y, Shaham S, et al. A secreted bacterial peptidoglycan hydrolase enhances tolerance to enteric pathogens. Science (2016) 353:1434–7. doi:10.1126/science.aaf3552
699. Than NG, Balogh A, Romero R, Kárpáti É, Erez O, Szilágyi A, et al. Placental protein 13 (PP13) – a placental immunoregulatory galectin protecting pregnancy. Front Immunol (2014) 5:348. doi:10.3389/fimmu.2014.00348
700. Than NG, Sumegi B, Than GN, Berente Z, Bohn H. Isolation and sequence analysis of a cDNA encoding human placental tissue protein 13 (PP13), a new lysophospholipase, homologue of human eosinophil Charcot-Leyden Crystal protein. Placenta (1999) 20:703–10. doi:10.1053/plac.1999.0436
701. Visegrády B, Than NG, Kilár F, Sümegi B, Than GN, Bohn H. Homology modelling and molecular dynamics studies of human placental tissue protein 13 (galectin-13). Protein Eng (2001) 14:875–80. doi:10.1093/protein/14.11.875
702. Bohn H, Kraus W, Winckler W. Purification and characterization of two new soluble placental tissue proteins (PP13 and PP17). Oncodev Biol Med (1983) 4:343–50.
703. Than NG, Pick E, Bellyei S, Szigeti A, Burger O, Berente Z, et al. Functional analyses of placental protein 13/galectin-13. Eur J Biochem (2004) 271:1065–78. doi:10.1111/j.1432-1033.2004.04004.x
704. Than NG, Romero R, Goodman M, Weckle A, Xing J, Dong Z, et al. A primate subfamily of galectins expressed at the maternal-fetal interface that promote immune cell death. Proc Natl Acad Sci U S A (2009) 106:9731–6. doi:10.1073/pnas.0903568106
705. Romero R, Kusanovic JP, Than NG, Erez O, Gotsch F, Espinoza J, et al. First-trimester maternal serum PP13 in the risk assessment for preeclampsia. Am J Obstet Gynecol (2008) 199:122.e121–122.e111. doi:10.1016/j.ajog.2008.01.013
706. Cowans NJ, Stamatopoulou A, Khalil A, Spencer K. PP13 as a marker of pre-eclampsia: a two platform comparison study. Placenta (2011) 32(Suppl):S37–41. doi:10.1016/j.placenta.2010.08.014
707. De Muro P, Capobianco G, Lepedda AJ, Nieddu G, Formato M, Tram NHQ, et al. Plasma PP13 and urinary GAGs/PGs as early markers of pre-eclampsia. Arch Gynecol Obstet (2016) 294:959–65. doi:10.1007/s00404-016-4111-0
708. Than NG, Abdul Rahman O, Magenheim R, Nagy B, Fule T, Hargitai B, et al. Placental protein 13 (galectin-13) has decreased placental expression but increased shedding and maternal serum concentrations in patients presenting with preterm pre-eclampsia and HELLP syndrome. Virchows Arch (2008) 453:387–400. doi:10.1007/s00428-008-0658-x
709. Bruiners N, Bosman M, Postma A, Gebhardt S, Rebello G, Sammar M, et al. Promoter variant-98A-C of the LGALS13 gene and pre-eclampsia. Proceedings of the 8th World Congress of Perinatal Medicine. Florence (2007). p. 371–4.
710. Than NG, Erez O, Wildman DE, Tarca AL, Edwin SS, Abbas A, et al. Severe preeclampsia is characterized by increased placental expression of galectin-1. J Matern Fetal Neonatal Med (2008) 21:429–42. doi:10.1080/14767050802041961
711. Vasta GR. Roles of galectins in infection. Nat Rev Microbiol (2009) 7:424–38. doi:10.1038/nrmicro2146
712. Poltorak A, He XL, Smirnova I, Liu MY, Van Huffel C, Du X, et al. Defective LPS signaling in C3H/HeJ and C57BL/10ScCr mice: mutations in Tlr4 gene. Science (1998) 282:2085–8. doi:10.1126/science.282.5396.2085
713. Hoshino K, Takeuchi O, Kawai T, Sanjo H, Ogawa T, Takeda Y, et al. Toll-like receptor 4 (TLR4)-deficient mice are hyporesponsive to lipopolysaccharide: evidence for TLR4 as the Lps gene product. J Immunol (1999) 162:3749–52.
714. Lien E, Means TK, Heine H, Yoshimura A, Kusumoto S, Fukase K, et al. Toll-like receptor 4 imparts ligand-specific recognition of bacterial lipopolysaccharide. J Clin Invest (2000) 105:497–504. doi:10.1172/JCI8541
715. Schwandner R, Dziarski R, Wesche H, Rothe M, Kirschning CJ. Peptidoglycan- and lipoteichoic acid-induced cell activation is mediated by toll-like receptor 2. J Biol Chem (1999) 274:17406–9. doi:10.1074/jbc.274.25.17406
716. Underhill DM, Ozinsky A, Hajjar AM, Stevens A, Wilson CB, Bassetti M, et al. The toll-like receptor 2 is recruited to macrophage phagosomes and discriminates between pathogens. Nature (1999) 401:811–5. doi:10.1038/44605
717. Ishii KJ, Akira S. Toll-like receptors and sepsis. Curr Infect Dis Rep (2004) 6:361–6. doi:10.1007/s11908-004-0034-1
718. Zähringer U, Lindner B, Inamura S, Heine H, Alexander C. TLR2 - promiscuous or specific? A critical re-evaluation of a receptor expressing apparent broad specificity. Immunobiology (2008) 213:205–24. doi:10.1016/j.imbio.2008.02.005
719. Kawai T, Akira S. Toll-like receptors and their crosstalk with other innate receptors in infection and immunity. Immunity (2011) 34:637–50. doi:10.1016/j.immuni.2011.05.006
720. Kumar H, Kawai T, Akira S. Pathogen recognition by the innate immune system. Int Rev Immunol (2011) 30:16–34. doi:10.3109/08830185.2010.529976
721. Oliveira-Nascimento L, Massari P, Wetzler LM. The role of TLR2 in infection and immunity. Front Immunol (2012) 3:79. doi:10.3389/fimmu.2012.00079
722. Alexander SPA, Benson HE, Faccenda E, Pawson AJ, Sharman JL, Spedding M, et al. The concise guide to pharmacology 2013/14: catalytic receptors. Br J Pharmacol (2013) 170:1676–705. doi:10.1111/bph.12449
723. Kumar S, Ingle H, Prasad DV, Kumar H. Recognition of bacterial infection by innate immune sensors. Crit Rev Microbiol (2013) 39:229–46. doi:10.3109/1040841X.2012.706249
724. Liu Y, Yin H, Zhao M, Lu Q. TLR2 and TLR4 in autoimmune diseases: a comprehensive review. Clin Rev Allergy Immunol (2014) 47:136–47. doi:10.1007/s12016-013-8402-y
725. Jiménez-Dalmaroni MJ, Gerswhin ME, Adamopoulos IE. The critical role of toll-like receptors – from microbial recognition to autoimmunity: a comprehensive review. Autoimmun Rev (2016) 15:1–8. doi:10.1016/j.autrev.2015.08.009
726. Mukherjee S, Karmakar S, Babu SP. TLR2 and TLR4 mediated host immune responses in major infectious diseases: a review. Braz J Infect Dis (2016) 20:193–204. doi:10.1016/j.bjid.2015.10.011
727. Tinsley JH, Chiasson VL, Mahajan A, Young KJ, Mitchell BM. Toll-like receptor 3 activation during pregnancy elicits preeclampsia-like symptoms in rats. Am J Hypertens (2009) 22:1314–9. doi:10.1038/ajh.2009.185
728. Akira S, Uematsu S, Takeuchi O. Pathogen recognition and innate immunity. Cell (2006) 124:783–801. doi:10.1016/j.cell.2006.02.015
729. Schroder K, Muruve DA, Tschopp J. Innate immunity: cytoplasmic DNA sensing by the AIM2 inflammasome. Curr Biol (2009) 19:R262–5. doi:10.1016/j.cub.2009.02.011
730. Sauer JD, Witte CE, Zemansky J, Hanson B, Lauer P, Portnoy DA. Listeria monocytogenes triggers AIM2-mediated pyroptosis upon infrequent bacteriolysis in the macrophage cytosol. Cell Host Microbe (2010) 7:412–9. doi:10.1016/j.chom.2010.04.004
731. Barber GN. Cytoplasmic DNA innate immune pathways. Immunol Rev (2011) 243:99–108. doi:10.1111/j.1600-065X.2011.01051.x
732. Konno H, Barber GN. The STING controlled cytosolic-DNA activated innate immune pathway and microbial disease. Microbes Infect (2014) 16:998–1001. doi:10.1016/j.micinf.2014.10.002
733. Paludan SR. Activation and regulation of DNA-driven immune responses. Microbiol Mol Biol Rev (2015) 79:225–41. doi:10.1128/MMBR.00061-14
734. Girling JE, Hedger MP. Toll-like receptors in the gonads and reproductive tract: emerging roles in reproductive physiology and pathology. Immunol Cell Biol (2007) 85:481–9. doi:10.1038/sj.icb.7100086
735. van Rijn BB, Franx A, Steegers EAP, De Groot CJM, Bertina RM, Pasterkamp G, et al. Maternal TLR4 and NOD2 gene variants, pro-inflammatory phenotype and susceptibility to early-onset preeclampsia and HELLP syndrome. PLoS One (2008) 3:e1865. doi:10.1371/journal.pone.0001865
736. Riley JK, Nelson DM. Toll-like receptors in pregnancy disorders and placental dysfunction. Clin Rev Allergy Immunol (2010) 39:185–93. doi:10.1007/s12016-009-8178-2
737. Pineda A, Verdin-Terán SL, Camacho A, Moreno-Fierros L. Expression of toll-like receptor TLR-2, TLR-3, TLR-4 and TLR-9 is increased in placentas from patients with preeclampsia. Arch Med Res (2011) 42:382–91. doi:10.1016/j.arcmed.2011.08.003
738. Panda B, Panda A, Ueda I, Abrahams VM, Norwitz ER, Stanic AK, et al. Dendritic cells in the circulation of women with preeclampsia demonstrate a pro-inflammatory bias secondary to dysregulation of TLR receptors. J Reprod Immunol (2012) 94:210–5. doi:10.1016/j.jri.2012.01.008
739. Zhang L, Yang H. Expression and localization of TLR4 and its negative regulator tollip in the placenta of early-onset and late-onset preeclampsia. Hypertens Pregnancy (2012) 31:218–27. doi:10.3109/10641955.2011.642434
740. Amirchaghmaghi E, Taghavi SA, Shapouri F, Saeidi S, Rezaei A, Aflatoonian R. The role of toll like receptors in pregnancy. Int J Fertil Steril (2013) 7:147–54.
741. Zhu Y, Wu M, Wu CY, Xia GQ. Role of progesterone in TLR4-MyD88-dependent signaling pathway in pre-eclampsia. J Huazhong Univ Sci Technolog Med Sci (2013) 33:730–4. doi:10.1007/s11596-013-1188-6
742. Koga K, Izumi G, Mor G, Fujii T, Osuga Y. Toll-like receptors at the maternal-fetal interface in normal pregnancy and pregnancy complications. Am J Reprod Immunol (2014) 72:192–205. doi:10.1111/aji.12258
743. Xue PP, Zheng MM, Gong P, Lin CM, Zhou JJ, Li YJ, et al. Single administration of ultra-low-dose lipopolysaccharide in rat early pregnancy induces TLR4 activation in the placenta contributing to preeclampsia. PLoS One (2015) 10:e0124001. doi:10.1371/journal.pone.0124001
744. Gong P, Liu M, Hong G, Li Y, Xue P, Zheng M, et al. Curcumin improves LPS-induced preeclampsia-like phenotype in rat by inhibiting the TLR4 signaling pathway. Placenta (2016) 41:45–52. doi:10.1016/j.placenta.2016.03.002
745. Kulikova GV, Nizyaeva NV, Nagovitsina MN, Lyapin VM, Loginova NS, Kan NE, et al. Specific features of TLR4 expression in structural elements of placenta in patients with preeclampsia. Bull Exp Biol Med (2016) 160:718–21. doi:10.1007/s10517-016-3259-8
746. Faas MM, Schuiling GA, Baller JF, Visscher CA, Bakker WW. A new animal model for human preeclampsia: ultra-low-dose endotoxin infusion in pregnant rats. Am J Obstet Gynecol (1994) 171:158–64. doi:10.1016/0002-9378(94)90463-4
747. Fujita Y, Mihara T, Okazaki T, Shitanaka M, Kushino R, Ikeda C, et al. Toll-like receptors (TLR) 2 and 4 on human sperm recognize bacterial endotoxins and mediate apoptosis. Hum Reprod (2011) 26:2799–806. doi:10.1093/humrep/der234
748. Li N, Wang T, Han D. Structural, cellular and molecular aspects of immune privilege in the testis. Front Immunol (2012) 3:152. doi:10.3389/fimmu.2012.00152
749. Saeidi S, Shapouri F, Amirchaghmaghi E, Hoseinifar H, Sabbaghian M, Sadighi Gilani MA, et al. Sperm protection in the male reproductive tract by toll-like receptors. Andrologia (2014) 46:784–90. doi:10.1111/and.12149
750. Hagan S, Khurana N, Chandra S, Abdel-Mageed AB, Mondal D, Hellstrom WJ, et al. Differential expression of novel biomarkers (TLR-2, TLR-4, COX-2, and Nrf-2) of inflammation and oxidative stress in semen of leukocytospermia patients. Andrology (2015) 3:848–55. doi:10.1111/andr.12074
751. Kunjara S, Greenbaum AL, Wang DY, Caro HN, Mclean P, Redman CWG, et al. Inositol phosphoglycans and signal transduction systems in pregnancy in preeclampsia and diabetes: evidence for a significant regulatory role in preeclampsia at placental and systemic levels. Mol Genet Metab (2000) 69:144–58. doi:10.1006/mgme.2000.2964
752. Williams PJ, Gumaa K, Scioscia M, Redman CW, Rademacher TW. Inositol phosphoglycan P-type in preeclampsia: a novel marker? Hypertension (2007) 49:84–9. doi:10.1161/01.HYP.0000251301.12357.ba
753. Scioscia M, Paine MA, Gumaa K, Rodeck CH, Rademacher TW. Release of inositol phosphoglycan P-type by the human placenta following insulin stimulus: a multiple comparison between preeclampsia, intrauterine growth restriction, and gestational hypertension. J Matern Fetal Neonatal Med (2008) 21:581–5. doi:10.1080/14767050802199934
754. Scioscia M, Gumaa K, Rademacher TW. The link between insulin resistance and preeclampsia: new perspectives. J Reprod Immunol (2009) 82:100–5. doi:10.1016/j.jri.2009.04.009
755. Scioscia M, Gumaa K, Selvaggi LE, Rodeck CH, Rademacher TW. Increased inositol phosphoglycan P-type in the second trimester in pregnant women with type 2 and gestational diabetes mellitus. J Perinat Med (2009) 37:469–71. doi:10.1515/JPM.2009.082
756. Paine MA, Scioscia M, Williams PJ, Gumaa K, Rodeck CH, Rademacher TW. Urinary inositol phosphoglycan P-type as a marker for prediction of preeclampsia and novel implications for the pathophysiology of this disorder. Hypertens Pregnancy (2010) 29:375–84. doi:10.3109/10641950903242667
757. Scioscia M, Williams PJ, Gumaa K, Fratelli N, Zorzi C, Rademacher TW. Inositol phosphoglycans and preeclampsia: from bench to bedside. J Reprod Immunol (2011) 89:173–7. doi:10.1016/j.jri.2011.03.001
758. Scioscia M, Robillard PY, Hall DR, Rademacher LH, Williams PJ, Rademacher TW. Inositol phosphoglycan P-type in infants of preeclamptic mothers. J Matern Fetal Neonatal Med (2012) 25:193–5. doi:10.3109/14767058.2011.557789
759. Scioscia M, Siwetz M, Fascilla F, Huppertz B. Placental expression of D-chiro-inositol phosphoglycans in preeclampsia. Placenta (2012) 33:882–4. doi:10.1016/j.placenta.2012.07.007
760. Scioscia M, Siwetz M, Campana C, Huppertz B. Differences in d-chiro-inositol-phosphoglycan expression between first and third trimester human placenta. Pregnancy Hypertens (2013) 3:1–2. doi:10.1016/j.preghy.2012.10.001
761. Scioscia M, Nigro M, Montagnani M. The putative metabolic role of d-chiro inositol phosphoglycan in human pregnancy and preeclampsia. J Reprod Immunol (2014) 101-102:140–7. doi:10.1016/j.jri.2013.05.006
762. Kunjara S, Mclean P, Rademacher L, Rademacher TW, Fascilla F, Bettocchi S, et al. Putative key role of inositol messengers in endothelial cells in preeclampsia. Int J Endocrinol (2016) 2016:7695648. doi:10.1155/2016/7695648
763. Pretorius E, Bester J, Kell DB. A bacterial component to Alzheimer-type dementia seen via a systems biology approach that links iron dysregulation and inflammagen shedding to disease. J Alzheimers Dis (2016) 53:1237–56. doi:10.3233/JAD-160318
764. Kell DB, Pretorius E. To what extent are the terminal stages of sepsis, septic shock, SIRS, and multiple organ dysfunction syndrome actually driven by a toxic prion/amyloid form of fibrin? Semin Thromb Hemost (2017). doi:10.1055/s-0037-1604108
765. Pretorius E, Mbotwe S, Kell DB. Lipopolysaccharide-binding protein (LBP) reverses the amyloid state of fibrin seen in plasma of type 2 diabetics with cardiovascular comorbidities. Sci Rep (2017) 7:9680. doi:10.1038/s41598-017-09860-4
766. Pretorius E, Page MJ, Engelbrecht L, Ellis GC, Kell DB. Substantial fibrin amyloidogenesis in type 2 diabetes assessed using amyloid-selective fluorescent stains. Cardiovasc Diabetol (2017) 16:141. doi:10.1186/s12933-017-0624-5
767. Cervera R, Balasch J. Bidirectional effects on autoimmunity and reproduction. Hum Reprod Update (2008) 14:359–66. doi:10.1093/humupd/dmn013
768. Heilmann L, Schorsch M, Hahn T, Fareed J. Antiphospholipid syndrome and pre-eclampsia. Semin Thromb Hemost (2011) 37:141–5. doi:10.1055/s-0030-1270341
769. Chen Q, Guo F, Hensby-Bennett S, Stone P, Chamley L. Antiphospholipid antibodies prolong the activation of endothelial cells induced by necrotic trophoblastic debris: implications for the pathogenesis of preeclampsia. Placenta (2012) 33:810–5. doi:10.1016/j.placenta.2012.07.019
770. Lefkou E, Mamopoulos A, Fragakis N, Dagklis T, Vosnakis C, Nounopoulos E, et al. Clinical improvement and successful pregnancy in a preeclamptic patient with antiphospholipid syndrome treated with pravastatin. Hypertension (2014) 63:e118–9. doi:10.1161/HYPERTENSIONAHA.114.03115
771. van Hoorn ME, Hague WM, Van Pampus MG, Bezemer D, De Vries JIP, Investigators F. Low-molecular-weight heparin and aspirin in the prevention of recurrent early-onset pre-eclampsia in women with antiphospholipid antibodies: the FRUIT-RCT. Eur J Obstet Gynecol Reprod Biol (2016) 197:168–73. doi:10.1016/j.ejogrb.2015.12.011
772. Asherson RA, Cervera R. Antiphospholipid antibodies and infections. Ann Rheum Dis (2003) 62:388–93. doi:10.1136/ard.62.5.388
773. Shoenfeld Y, Blank M, Cervera R, Font J, Raschi E, Meroni PL. Infectious origin of the antiphospholipid syndrome. Ann Rheum Dis (2006) 65:2–6. doi:10.1136/ard.2005.045443
774. Amin NM. Antiphospholipid syndromes in infectious diseases. Hematol Oncol Clin North Am (2008) 22:131–143, vii–viii. doi:10.1016/j.hoc.2007.10.001
775. Sène D, Piette JC, Cacoub P. Antiphospholipid antibodies, antiphospholipid syndrome and infections. Autoimmun Rev (2008) 7:272–7. doi:10.1016/j.autrev.2007.10.001
776. García-Carrasco M, Galarza-Maldonado C, Mendoza-Pinto C, Escarcega RO, Cervera R. Infections and the antiphospholipid syndrome. Clin Rev Allergy Immunol (2009) 36:104–8. doi:10.1007/s12016-008-8103-0
777. Zinger H, Sherer Y, Goddard G, Berkun Y, Barzilai O, Agmon-Levin N, et al. Common infectious agents prevalence in antiphospholipid syndrome. Lupus (2009) 18:1149–53. doi:10.1177/0961203309345738
778. Krone KA, Allen KL, Mccrae KR. Impaired fibrinolysis in the antiphospholipid syndrome. Curr Rheumatol Rep (2010) 12:53–7. doi:10.1007/s11926-009-0075-4
779. Martínez-Zamora MA, Tassies D, Carmona F, Espinosa G, Cervera R, Reverter JC, et al. Clot lysis time and thrombin activatable fibrinolysis inhibitor in severe preeclampsia with or without associated antiphospholipid antibodies. J Reprod Immunol (2010) 86:133–40. doi:10.1016/j.jri.2010.05.002
780. Lockshin MD. Anticoagulation in management of antiphospholipid antibody syndrome in pregnancy. Clin Lab Med (2013) 33:367–76. doi:10.1016/j.cll.2013.01.001
781. Lockshin MD. Pregnancy and antiphospholipid syndrome. Am J Reprod Immunol (2013) 69:585–7. doi:10.1111/aji.12071
782. Meroni PL, Chighizola CB, Rovelli F, Gerosa M. Antiphospholipid syndrome in 2014: more clinical manifestations, novel pathogenic players and emerging biomarkers. Arthritis Res Ther (2014) 16:209. doi:10.1186/ar4549
783. Buhimschi IA, Nayeri UA, Zhao G, Shook LL, Pensalfini A, Funai EF, et al. Protein misfolding, congophilia, oligomerization, and defective amyloid processing in preeclampsia. Sci Transl Med (2014) 6:245ra292. doi:10.1126/scitranslmed.3008808
784. Jonas SM, Deserno TM, Buhimschi CS, Makin J, Choma MA, Buhimschi IA. Smartphone-based diagnostic for preeclampsia: an mHealth solution for administering the Congo Red Dot (CRD) test in settings with limited resources. J Am Med Inform Assoc (2016) 23:166–73. doi:10.1093/jamia/ocv015
785. Kouza M, Banerji A, Kolinski A, Buhimschi IA, Kloczkowski A. Oligomerization of FVFLM peptides and their ability to inhibit beta amyloid peptides aggregation: consideration as a possible model. Phys Chem Chem Phys (2017) 19:2990–9. doi:10.1039/c6cp07145g
786. Clark EAS, Silver RM, Branch DW. Do antiphospholipid antibodies cause preeclampsia and HELLP syndrome? Curr Rheumatol Rep (2007) 9:219–25. doi:10.1007/s11926-007-0035-9
787. Saccone G, Berghella V, Maruotti GM, Ghi T, Rizzo G, Simonazzi G, et al. Antiphospholipid antibody profile based obstetric outcomes of primary antiphospholipid syndrome: the PREGNANTS study. Am J Obstet Gynecol (2017) 216:525.e521–525.e512. doi:10.1016/j.ajog.2017.01.026
788. Horvath SE, Daum G. Lipids of mitochondria. Prog Lipid Res (2013) 52:590–614. doi:10.1016/j.plipres.2013.07.002
789. Mejia EM, Nguyen H, Hatch GM. Mammalian cardiolipin biosynthesis. Chem Phys Lipids (2014) 179:11–6. doi:10.1016/j.chemphyslip.2013.10.001
790. Ren M, Phoon CKL, Schlame M. Metabolism and function of mitochondrial cardiolipin. Prog Lipid Res (2014) 55:1–16. doi:10.1016/j.plipres.2014.04.001
791. Hatch GM. Cardiolipin: biosynthesis, remodeling and trafficking in the heart and mammalian cells (review). Int J Mol Med (1998) 1:33–41.
792. Saini-Chohan HK, Holmes MG, Chicco AJ, Taylor WA, Moore RL, Mccune SA, et al. Cardiolipin biosynthesis and remodeling enzymes are altered during development of heart failure. J Lipid Res (2009) 50:1600–8. doi:10.1194/jlr.M800561-JLR200
793. Chaban Y, Boekema EJ, Dudkina NV. Structures of mitochondrial oxidative phosphorylation supercomplexes and mechanisms for their stabilisation. Biochim Biophys Acta (2014) 1837:418–26. doi:10.1016/j.bbabio.2013.10.004
794. Mileykovskaya E, Dowhan W. Cardiolipin-dependent formation of mitochondrial respiratory supercomplexes. Chem Phys Lipids (2014) 179:42–8. doi:10.1016/j.chemphyslip.2013.10.012
795. Paradies G, Paradies V, De Benedictis V, Ruggiero FM, Petrosillo G. Functional role of cardiolipin in mitochondrial bioenergetics. Biochim Biophys Acta (2014) 1837:408–17. doi:10.1016/j.bbabio.2013.10.006
796. Paradies G, Paradies V, Ruggiero FM, Petrosillo G. Cardiolipin and mitochondrial function in health and disease. Antioxid Redox Signal (2014) 20:1925–53. doi:10.1089/ars.2013.5280
797. Dolinsky VW, Cole LK, Sparagna GC, Hatch GM. Cardiac mitochondrial energy metabolism in heart failure: role of cardiolipin and sirtuins. Biochim Biophys Acta (2016) 1861:1544–54. doi:10.1016/j.bbalip.2016.03.008
798. Blank M, Krause I, Fridkin M, Keller N, Kopolovic J, Goldberg I, et al. Bacterial induction of autoantibodies to beta2-glycoprotein-I accounts for the infectious etiology of antiphospholipid syndrome. J Clin Invest (2002) 109:797–804. doi:10.1172/JCI12337
799. Harel M, Aron-Maor A, Sherer Y, Blank M, Shoenfeld Y. The infectious etiology of the antiphospholipid syndrome: links between infection and autoimmunity. Immunobiology (2005) 210:743–7. doi:10.1016/j.imbio.2005.10.004
800. Cruz-Tapias P, Blank M, Anaya JM, Shoenfeld Y. Infections and vaccines in the etiology of antiphospholipid syndrome. Curr Opin Rheumatol (2012) 24:389–93. doi:10.1097/BOR.0b013e32835448b8
801. Ebringer A, Rashid T. Rheumatoid arthritis is caused by Proteus: the molecular mimicry theory and Karl Popper. Front Biosci (Elite Ed) (2009) 1:577–86. doi:10.2741/e56
803. Bullerwell CE, Gray MW. Evolution of the mitochondrial genome: protist connections to animals, fungi and plants. Curr Opin Microbiol (2004) 7:528–34. doi:10.1016/j.mib.2004.08.008
804. Williams KP, Sobral BW, Dickerman AW. A robust species tree for the alphaproteobacteria. J Bacteriol (2007) 189:4578–86. doi:10.1128/JB.00269-07
805. Gray MW. Mitochondrial evolution. Cold Spring Harb Perspect Biol (2012) 4:a011403. doi:10.1101/cshperspect.a011403
806. Burger G, Gray MW, Forget L, Lang BF. Strikingly bacteria-like and gene-rich mitochondrial genomes throughout jakobid protists. Genome Biol Evol (2013) 5:418–38. doi:10.1093/gbe/evt008
807. Wang Z, Wu M. An integrated phylogenomic approach toward pinpointing the origin of mitochondria. Sci Rep (2015) 5:7949. doi:10.1038/srep07949
808. Ball SG, Bhattacharya D, Weber APM. Pathogen to powerhouse. Science (2016) 351:659–60. doi:10.1126/science.aad8864
809. John P, Whatley FR. Paracoccus denitrificans and the evolutionary origin of the mitochondrion. Nature (1975) 254:495–8. doi:10.1038/254495a0
810. Matsumoto K, Kusaka J, Nishibori A, Hara H. Lipid domains in bacterial membranes. Mol Microbiol (2006) 61:1110–7. doi:10.1111/j.1365-2958.2006.05317.x
811. Epand RM, Epand RF. Domains in bacterial membranes and the action of antimicrobial agents. Mol Biosyst (2009) 5:580–7. doi:10.1039/b900278m
812. Mileykovskaya E, Dowhan W. Cardiolipin membrane domains in prokaryotes and eukaryotes. Biochim Biophys Acta (2009) 1788:2084–91. doi:10.1016/j.bbamem.2009.04.003
813. Romantsov T, Guan Z, Wood JM. Cardiolipin and the osmotic stress responses of bacteria. Biochim Biophys Acta (2009) 1788:2092–100. doi:10.1016/j.bbamem.2009.06.010
814. Barák I, Muchová K. The role of lipid domains in bacterial cell processes. Int J Mol Sci (2013) 14:4050–65. doi:10.3390/ijms14024050
815. Mukamolova GV, Yanopolskaya ND, Votyakova TV, Popov VI, Kaprelyants AS, Kell DB. Biochemical changes accompanying the long-term starvation of Micrococcus luteus cells in spent growth medium. Arch Microbiol (1995) 163:373–9. doi:10.1007/BF00404211
816. Kell DB, Pretorius E. Serum ferritin is an important disease marker, and is mainly a leakage product from damaged cells. Metallomics (2014) 6:748–73. doi:10.1039/C3MT00347G
817. Agmon-Levin N, Rosário C, Katz BS, Zandman-Goddard G, Meroni P, Cervera R, et al. Ferritin in the antiphospholipid syndrome and its catastrophic variant (cAPS). Lupus (2013) 22:1327–35. doi:10.1177/0961203313504633
818. Rosário C, Zandman-Goddard G, Meyron-Holtz EG, D’cruz DP, Shoenfeld Y. The hyperferritinemic syndrome: macrophage activation syndrome, Still’s disease, septic shock and catastrophic antiphospholipid syndrome. BMC Med (2013) 11:185. doi:10.1186/1741-7015-11-185
819. Andrade SE, Gurwitz JH, Davis RL, Chan KA, Finkelstein JA, Fortman K, et al. Prescription drug use in pregnancy. Am J Obstet Gynecol (2004) 191:398–407. doi:10.1016/j.ajog.2004.04.025
820. Egen-Lappe V, Hasford J. Drug prescription in pregnancy: analysis of a large statutory sickness fund population. Eur J Clin Pharmacol (2004) 60:659–66. doi:10.1007/s00228-004-0817-1
821. Riley EH, Fuentes-Afflick E, Jackson RA, Escobar GJ, Brawarsky P, Schreiber M, et al. Correlates of prescription drug use during pregnancy. J Womens Health (Larchmt) (2005) 14:401–9. doi:10.1089/jwh.2005.14.401
822. Daw JR, Hanley GE, Greyson DL, Morgan SG. Prescription drug use during pregnancy in developed countries: a systematic review. Pharmacoepidemiol Drug Saf (2011) 20:895–902. doi:10.1002/pds.2184
823. Mitchell AA, Gilboa SM, Werler MM, Kelley KE, Louik C, Hernández-Díaz S, et al. Medication use during pregnancy, with particular focus on prescription drugs: 1976–2008. Am J Obstet Gynecol (2011) 205:51.e51–8. doi:10.1016/j.ajog.2011.02.029
824. Daw JR, Mintzes B, Law MR, Hanley GE, Morgan SG. Prescription drug use in pregnancy: a retrospective, population-based study in British Columbia, Canada (2001–2006). Clin Ther (2012) 34(239–249):e232. doi:10.1016/j.clinthera.2011.11.025
825. Cea-Soriano L, Garcia Rodríguez LA, Fernández Cantero O, Hernández-Díaz S. Challenges of using primary care electronic medical records in the UK to study medications in pregnancy. Pharmacoepidemiol Drug Saf (2013) 22:977–85. doi:10.1002/pds.3472
826. Palmsten K, Hernández-Díaz S, Chambers CD, Mogun H, Lai S, Gilmer TP, et al. The most commonly dispensed prescription medications among pregnant women enrolled in the U.S. Medicaid program. Obstet Gynecol (2015) 126:465–73. doi:10.1097/AOG.0000000000000982
827. Smolina K, Hanley GE, Mintzes B, Oberlander TF, Morgan S. Trends and determinants of prescription drug use during pregnancy and postpartum in British Columbia, 2002–2011: a population-based cohort study. PLoS One (2015) 10:e0128312. doi:10.1371/journal.pone.0128312
828. Valent F, Gongolo F, Deroma L, Zanier L. Prescription of systemic antibiotics during pregnancy in primary care in Friuli Venezia Giulia, Northeastern Italy. J Matern Fetal Neonatal Med (2015) 28:210–5. doi:10.3109/14767058.2014.906572
829. Heikkilä AM. Antibiotics in pregnancy – a prospective cohort study on the policy of antibiotic prescription. Ann Med (1993) 25:467–71. doi:10.3109/07853899309147314
830. Santos F, Oraichi D, Bérard A. Prevalence and predictors of anti-infective use during pregnancy. Pharmacoepidemiol Drug Saf (2010) 19:418–27. doi:10.1002/pds.1915
831. de Jonge L, Bos HJ, Van Langen IM, De Jong-Van Den Berg LTW, Bakker MK. Antibiotics prescribed before, during and after pregnancy in the Netherlands: a drug utilization study. Pharmacoepidemiol Drug Saf (2014) 23:60–8. doi:10.1002/pds.3492
832. Bookstaver PB, Bland CM, Griffin B, Stover KR, Eiland LS, Mclaughlin M. A review of antibiotic use in pregnancy. Pharmacotherapy (2015) 35:1052–62. doi:10.1002/phar.1649
833. Calogero AE, Condorelli RA, Russo GI, Vignera S. Conservative nonhormonal options for the treatment of male infertility: antibiotics, anti-inflammatory drugs, and antioxidants. Biomed Res Int (2017) 2017:4650182. doi:10.1155/2017/4650182
834. Kabir S. The current status of Helicobacter pylori vaccines: a review. Helicobacter (2007) 12:89–102. doi:10.1111/j.1523-5378.2007.00478.x
835. D’Elios MM, Czinn SJ. Immunity, inflammation, and vaccines for Helicobacter pylori. Helicobacter (2014) 19(Suppl 1):19–26. doi:10.1111/hel.12156
836. Sutton P, Chionh YT. Why can’t we make an effective vaccine against Helicobacter pylori? Expert Rev Vaccines (2013) 12:433–41. doi:10.1586/erv.13.20
837. Ng GZ, Chionh YT, Sutton P. Vaccine-mediated protection against Helicobacter pylori is not associated with increased salivary cytokine or mucin expression. Helicobacter (2014) 19:48–54. doi:10.1111/hel.12099
838. Grixti J, O’Hagan S, Day PJ, Kell DB. Enhancing drug efficacy and therapeutic index through cheminformatics-based selection of small molecule binary weapons that improve transporter-mediated targeting: a cytotoxicity system based on gemcitabine. Front Pharmacol (2017) 8:155. doi:10.3389/fphar.2017.00155
839. Borisy AA, Elliott PJ, Hurst NW, Lee MS, Lehar J, Price ER, et al. Systematic discovery of multicomponent therapeutics. Proc Natl Acad Sci U S A (2003) 100:7977–82. doi:10.1073/pnas.1337088100
840. Lehár J, Krueger AS, Avery W, Heilbut AM, Johansen LM, Price ER, et al. Synergistic drug combinations tend to improve therapeutically relevant selectivity. Nat Biotechnol (2009) 27:659–66. doi:10.1038/nbt.1549
841. Kell DB. Iron behaving badly: inappropriate iron chelation as a major contributor to the aetiology of vascular and other progressive inflammatory and degenerative diseases. BMC Med Genom (2009) 2:2. doi:10.1186/1755-8794-2-2
842. Kell DB. Towards a unifying, systems biology understanding of large-scale cellular death and destruction caused by poorly liganded iron: Parkinson’s, Huntington’s, Alzheimer’s, prions, bactericides, chemical toxicology and others as examples. Arch Toxicol (2010) 577:825–89. doi:10.1007/s00204-010-0577-x
844. Day J, Savani S, Krempley BD, Nguyen M, Kitlinska JB. Influence of paternal preconception exposures on their offspring: through epigenetics to phenotype. Am J Stem Cells (2016) 5:11–8.
845. Abbasi J. The paternal epigenome makes its mark. JAMA (2017) 317:2049–51. doi:10.1001/jama.2017.1566
846. Agarwal A, Sekhon LH. The role of antioxidant therapy in the treatment of male infertility. Hum Fertil (2010) 13:217–25. doi:10.3109/14647273.2010.532279
847. Nakata K, Yamashita N, Noda Y, Ohsawa I. Stimulation of human damaged sperm motility with hydrogen molecule. Med Gas Res (2015) 5:2. doi:10.1186/s13618-014-0023-x
848. Halliwell B, Cheah IK, Drum CL. Ergothioneine, an adaptive antioxidant for the protection of injured tissues? A hypothesis. Biochem Biophys Res Commun (2016) 470:245–50. doi:10.1016/j.bbrc.2015.12.124
849. Ahmed A, Singh J, Khan Y, Seshan SV, Girardi G. A new mouse model to explore therapies for preeclampsia. PLoS One (2010) 5:e13663. doi:10.1371/journal.pone.0013663
850. Bonney EA. Demystifying animal models of adverse pregnancy outcomes: touching bench and bedside. Am J Reprod Immunol (2013) 69:567–84. doi:10.1111/aji.12102
851. LaMarca B, Amaral LM, Harmon AC, Cornelius DC, Faulkner JL, Cunningham MW Jr. Placental ischemia and resultant phenotype in animal models of preeclampsia. Curr Hypertens Rep (2016) 18:38. doi:10.1007/s11906-016-0633-x
852. Kenny LC, Broadhurst DI, Dunn W, Brown M, Francis-Mcintyre S, North RA, et al. Robust early pregnancy prediction of later preeclampsia using metabolomic biomarkers. Hypertension (2010) 56:741–9. doi:10.1161/HYPERTENSIONAHA.110.157297
853. Kell DB, Oliver SG. The metabolome 18 years on: a concept comes of age. Metabolomics (2016) 12:148. doi:10.1007/s11306-016-1108-4
854. Infectious Diseases Society of America, Spellberg B, Blaser M, Guidos RJ, Boucher HW, Bradley JS, et al. Combating antimicrobial resistance: policy recommendations to save lives. Clin Infect Dis (2011) 52(Suppl 5):S397–428. doi:10.1093/cid/cir153
855. Gelband H, Laxminarayan R. Tackling antimicrobial resistance at global and local scales. Trends Microbiol (2015) 23:524–6. doi:10.1016/j.tim.2015.06.005
856. Laxminarayan R, Sridhar D, Blaser M, Wang M, Woolhouse M. Achieving global targets for antimicrobial resistance. Science (2016) 353:874–5. doi:10.1126/science.aaf9286
857. Coates ARM, Hu Y. New strategies for antibacterial drug design: targeting non-multiplying latent bacteria. Drugs R D (2006) 7:133–51. doi:10.2165/00126839-200607030-00001
858. Coates AR, Halls G, Hu Y. Novel classes of antibiotics or more of the same? Br J Pharmacol (2011) 163:184–94. doi:10.1111/j.1476-5381.2011.01250.x
859. Hu Y, Liu A, Ortega-Muro F, Alameda-Martin L, Mitchison D, Coates A. High-dose rifampicin kills persisters, shortens treatment duration, and reduces relapse rate in vitro and in vivo. Front Microbiol (2015) 6:641. doi:10.3389/fmicb.2015.00641
860. Broxmeyer L. Parkinson’s: another look. Med Hypotheses (2002) 59:373–7. doi:10.1016/S0306-9877(02)00188-3
861. Shen CH, Chou CH, Liu FC, Lin TY, Huang WY, Wang YC, et al. Association between tuberculosis and Parkinson disease: a nationwide, population-based cohort study. Medicine (Baltimore) (2016) 95:e2883. doi:10.1097/MD.0000000000002883
862. Berstad K, Berstad JER. Parkinson’s disease; the hibernating spore hypothesis. Med Hypotheses (2017) 104:48–53. doi:10.1016/j.mehy.2017.05.022
863. Raymond D, Peterson E. A critical review of early-onset and late-onset preeclampsia. Obstet Gynecol Surv (2011) 66:497–506. doi:10.1097/OGX.0b013e3182331028
864. Chaiworapongsa T, Romero R, Whitten A, Tarca AL, Bhatti G, Draghici S, et al. Differences and similarities in the transcriptional profile of peripheral whole blood in early and late-onset preeclampsia: insights into the molecular basis of the phenotype of preeclampsiaa. J Perinat Med (2013) 41:485–504. doi:10.1515/jpm-2013-0082
865. Lisonkova S, Joseph KS. Incidence of preeclampsia: risk factors and outcomes associated with early- versus late-onset disease. Am J Obstet Gynecol (2013) 209:544.e541–544.e512. doi:10.1016/j.ajog.2013.08.019
866. Lisonkova S, Sabr Y, Mayer C, Young C, Skoll A, Joseph KS. Maternal morbidity associated with early-onset and late-onset preeclampsia. Obstet Gynecol (2014) 124:771–81. doi:10.1097/AOG.0000000000000472
Keywords: preeclampsia, immunology, microbes, dormancy, semen, infection
Citation: Kenny LC and Kell DB (2018) Immunological Tolerance, Pregnancy, and Preeclampsia: The Roles of Semen Microbes and the Father. Front. Med. 4:239. doi: 10.3389/fmed.2017.00239
Received: 10 October 2017; Accepted: 12 December 2017;
Published: 04 January 2018
Edited by:
Issam Lebbi, Dream Center, TunisiaReviewed by:
Stefan Gebhardt, Stellenbosch University, South AfricaPeter Sedlmayr, Medical University of Graz, Austria
Copyright: © 2018 Kenny and Kell. This is an open-access article distributed under the terms of the Creative Commons Attribution License (CC BY). The use, distribution or reproduction in other forums is permitted, provided the original author(s) or licensor are credited and that the original publication in this journal is cited, in accordance with accepted academic practice. No use, distribution or reproduction is permitted which does not comply with these terms.
*Correspondence: Douglas B. Kell, ZGJrJiN4MDAwNDA7bWFuY2hlc3Rlci5hYy51aw==
†Paper 14 in the series “The dormant blood microbiome in chronic, inflammatory diseases.”