- Department of Biochemical Engineering, School of Chemical Engineering, Harcourt Butler Technical University, Kanpur, India
Carbon quantum dots (CQDs) have shown considerable interest in multiple fields including bioimaging, biosensing, photocatalysis, ion sensing, heavy metal detection, and therapy due to highly tunable photoluminescence and good photostability. Apart from having optical properties CQDs offer several advantages such as low toxicity, environmental friendliness, affordability, and simple synthesis methods. Furthermore, by modifying their surface and functionality, it's possible to precisely control their physical and chemical characteristics. Nevertheless, the growing utilization of carbon-based nanomaterials (CNMs) requires thorough examination of their potential toxicity and long-term impacts on human health and biological systems. In this study, carbon quantum dots (CQDs) were synthesized via a microwave-assisted method using citric acid and urea as precursors, resulting in an average particle diameter of 10.73 nm. The CQDs were further characterized using SEM and FTIR analysis. The CQDs exhibited an excitation wavelength of 320 nm, displaying an emission peak at 430 nm. The enzymatic biodegradation of CQDs by human myeloperoxidase enzyme has been thoroughly investigated here. It is very crucial to understand how these carbon quantum dots interact with the innate immune system that plays a vital role in recognizing and clearing foreign particles. Human myeloperoxidase (MPO), a key enzyme highly expressed in neutrophil granulocytes during inflammatory responses, has been shown to facilitate the biodegradation of carbon quantum dots and various carbon-based nanomaterials through oxidative processes. As a member of the peroxidase family, MPO produces hypochlorous acid (HOCl) and a range of reactive intermediates to eliminate pathogens. Consequently, the study of the biodegradability of CQDs within biological systems is essential for accelerating technological advancements. Here, we have assessed breakdown of CQDs through an oxidative process facilitated by a myeloperoxidase (MPO)-based peroxide system. The human MPO enzyme acted as a catalyst for the CQD degradation, and the addition of hydrogen peroxide (H2O2) and sodium chloride (NaCl) was found to accelerate the reaction.
1 Introduction
Carbon-derived nanomaterials, including carbon quantum dots (CQDs), fullerenes, graphene quantum dots, and carbon nanotubes, have garnered significant attention in recent times for a variety of applications within the fields of science and technology, attributable to their exceptional optical, mechanical, and photoluminescent characteristics (1, 2). With the pervasive utilization of carbon-derived nanomaterials, their inevitable release into the environment raises concerns regarding potential environmental contamination (3, 4). Consequently, the toxicity associated with nanomaterials has garnered increased scrutiny in recent years. When metallic carbon nanomaterials accumulate within cellular structures, they can produce free radicals that lead to DNA damage, inflammation, and oxidative stress (5). Thus, the potential hazards posed by engineered nanomaterials, including graphene quantum dots, carbon quantum dots, and metal oxide nanoparticles, remain a significant concern. In response to these issues, recent advancements have focused on developing engineered nanomaterials characterized by low toxicity and minimal environmental impact. Notably, carbon quantum dots (CQDs), representing a novel class of carbon-based engineered nanomaterials, have garnered substantial interest due to their ease of synthesis and modification, high biocompatibility, and versatility in application (4, 6).
Carbon nanoparticles (CQDs) are extremely small nanoparticles made of carbon that have undergone surface passivation or other modifications to become functionalized. The structure of CQDs can be both amorphous as well as crystalline (7). They exhibit sp2 carbon hybridization, however sp3 hybridization has occasionally also been observed (2, 8, 9).
CQDs are zero-dimensional carbon nanomaterials that are limited in size both in-plane and out-of-plane and distinguished by their relatively strong fluorescence properties and small size (less than 10 nm) (10). The fluorescence characters in CQDs are due to two types of sources one is fluorescence emission from the bandgap transitions within conjugated domains and fluorescence arising from surface defects. The CQDs are well-suited for biomedical applications due to their fluorescence emission in the near-infrared region (11). By changing the excitation wavelength, these structures allow for the tuning of the fluorescence. As the use of carbon quantum dots increases, it is crucial to closely examine their effects on human health and biological systems (12, 13). It is especially crucial to comprehend how they interact with innate immune system cells (12, 14, 15).
Carbon nanomaterials undergo biodegradation both in vivo and in vitro via myeloperoxidase (MPO), an essential enzyme that is secreted by neutrophils during inflammation (8, 16, 17). The capability of peroxidase enzymes to degrade carbon nanomaterials has emphasized the significance of using an ecologically safe enzymatic carbon nanomaterial degrading approach (18, 19). The human myeloperoxidase enzyme is a heme-containing complex that is well-known for having two glycosylated and two unglycosylated chains. This myeloperoxidase enzyme generates hypochlorous acid (HClO) molecules from hydrogen peroxide (H2O2) and chloride (Cl−) (20, 21). When carboxylated carbon nanomaterials are incubated with MPO, H2O2, and Cl– a variety of carbonaceous byproducts, such as CO, CO2, and various hydrocarbons are produced (8, 22). The structure and enzymatic characteristics of MPO enzyme is because of distinct prosthetic groups (23). When the enzyme MPO-Fe (III) is secreted, it undergoes a fast and reversible reaction with H2O2 to generate Compound I, an intermediate with a Fe group that has undergone two-electron oxidation. In the presence of H2O2, this redox intermediate transform halide (Cl−, Br−) and pseudohalides (SCN−) to their corresponding hypohalous acids (20, 24). This biochemical process, referred to as the halogenation cycle, generates Cl− ions as the preferred substrate and hypochlorous acid (HOCl), which serves as the dominant oxidant under physiological circumstances (23, 25).
However, carbon quantum dots (CQDs) are predominantly regarded as highly biocompatible and safe engineered nanomaterials (ENMs), it remains crucial to thoroughly investigate their potential toxicity before widespread use and mass production. Most of the research has conducted experiments at the cellular level to assess various types of CQDs, demonstrating that these structures exhibit lower cytotoxicity and enhanced biocompatibility compared to metallic ENMs (3, 5). Some investigations have employed animal models to assess the toxicity associated with CQDs (26). A considerable number of existing quantum dots are composed of hazardous metals such as cadmium and lead, which constrains their applicability in electronic and medical devices. This research focused on synthesizing carbon quantum dots through a single-step process, utilizing urea and citric acid as substrates and employing microwave-based synthesis method. Here, we have investigated various properties of the synthesized carbon quantum dots, including their size distribution, zeta potential, and chemical composition employing ultraviolet (UV) spectroscopy and dynamic light scattering (DLS) techniques. Additionally, we have examined the PL and UV absorption properties of CQDs using fluorescence and UV scans.
2 Materials and methods
2.1 Reagents
The citric acid and urea were obtained from HiMedia Laboratories Pvt. Ltd., India. The DMF (N, N-Dimethylformamide) was purchased from Merck Millipore. Hydrogen peroxide was obtained from SRL Pvt. Ltd., India. A Milli-Q device (Millipore, Burlington, MA, USA) provided the deionized water. Human origin myeloperoxidase (MPO) was purchased from Merck, Rahway, NJ, USA.
2.2 Preparation of urea and citric acid derived carbon quantum dots
The functionality and photoluminescence characteristics of carbon quantum dots can be precisely controlled by adjusting the surface functional groups and chemical configurations (27). A comprehensive review by (7) have explored into the synthesis of CQDs highlighting the potential for functionalization in accordance with their application requirements. Carbon quantum dots synthesis may be achieved through two distinct strategies: one is a top-down approach, where larger carbon-based materials are systematically reduced in size to form quantum dots. The second approach, called bottom-up, takes the opposite route by assembling quantum dots from smaller carbon-containing molecules or compounds (28, 29).
Here, the microwave-assisted synthesis method was utilized to prepare CQDs using urea and citric acid as the starting materials. Equal amounts of citric acid and urea, 5.0 grams each, were combined to create a 1:1 mixture for the synthesis of CQDs (30). Then this mixture was supplemented with 2 ml of N, N-dimethylformamide (DMF) solution. The prepared mixture was then microwaved at full power for two minutes using a standard domestic microwave. This process resulted in a charred substance with a chestnut brown color. After allowing this mixture to cool to room temperature, it was diluted with 50 ml of highly purified (Milli Q) water (31). The mixture was thoroughly blended using a high-speed homogenizer at 12,000 rpm for 15 min. The homogenized solution was centrifuged at 10,000 rpm for 40 min to remove larger particles, lumps, and residues from the CQDs. Following centrifugation, the pellet was discarded, and the supernatant was collected for subsequent processing (Figure 1). The supernatant was then dialyzed using 1 KDa dialyzer overnight at room temperature under continuous stirring. The dialyzed CQDs were then dried using a lyophilizer and were kept for long-term storage at 4°C.
2.3 Characterization methods for carbon quantum dots
For the physicochemical characterization of CQDs, 100 µg/ml of CQDs were suspended in Milli Q water to disperse the CQDs. The suspension was sonicated thoroughly in a bath sonicator (Ikon Industries) for 15 min with ice-cold water. Malvern Zetasizer Advance Series (Malvern, UK) instrument was used for the analysis of the particle size distribution and zeta potential at room temperature to maintain the stability of CQDs. The resulting graph and data were analyzed using zetasizer associated software.
2.4 Fluorescence spectroscopy
UV absorption scans (200–400 nm) and fluorescence spectrum scans (300–750 nm) of carbon quantum dots were conducted using an Envision device (PerkinElmer, Waltham, MO, USA). The resulting spectra were then analyzed with SpectraGryph software.
2.5 Fourier-transform infrared spectroscopy (FT-IR)
During this research, The KBr disk approach was specially used to generate Fourier-transform infrared spectra utilizing a Mattson 5,000 spectrometer (Unicam, United Kingdom).
2.6 Scanning electron microscopy
For surface topology, scanning electron microscope is used through the utilization of an accelerating voltage ranging from 10–15 kV.
2.7 Biodegradability of synthesized carbon quantum dots
When carrying out the enzymatic biodegradation of CQDs, we implemented the methodology outlined by (32). An aliquot of 1 ml of Milli-Q water (autoclaved), containing a concentration of 140 mM NaCl, was employed in the suspension of 5 μg/ml of human-derived MPO, which was subsequently combined with 50 μg of CQDs (resulting in a final concentration of 100 μg/ml). Subsequently, H2O2 was then added to the mixture at a rate of 100 μM h−1 for 24 h (32). Following an interval of six hours, the MPO enzyme was reintroduced, and the reaction mixture was sustained at a controlled temperature of 37°C (33). Subsequently, equivalent volumes of CQDs were dispersed in 1 ml of autoclaved Milli-Q H2O and subjected to a treatment duration of 24 h, with or without the addition of H2O2, at a rate of 100 M h−1. Upon the completion of reaction period, the samples were subjected to an analytical procedure to evaluate their absorbance within the wavelength range of 300–500 nm utilizing a UV-1800 spectrometer (Shimadzu, Kyoto, Japan). The final spectra were then processed using SpectraGryph software.
3 Result and discussion
3.1 Preparation and physicochemical characterization methods of carbon quantum dots
Carbon quantum dots were synthesized utilizing a 1:1 mixture of urea and citric acid through a simple single-step microwave-assisted approach using a 900-watt domestic microwave. The resulting CQDs were characterized using dynamic light scattering (DLS) to reveal a narrow size distribution with an average hydrodynamic diameter of 10.73 ± 0.18 nm (Figure 2), indicating the formation of uniform, nano-sized particles. The zeta potential was determined to be −11.9 ± 2.7 mV, suggesting colloidal stability of the CQDs in aqueous suspension (Figure 3). Furthermore, Carbon Quantum Dots (CQDs) were stimulated within the ultraviolet B (UVB) spectrum ranging from 280–320 nm. The resultant CQDs exhibited a photoluminescence (PL) emitting a blue color, as depicted in Figure 4 which is absent in standard white light sources. Neither chemical precursors nor dimethyl formamide shows any blue photoluminescence. This blue photoluminescence exhibited by CQDs arises from a combination of factors, including emissive traps, aromatic conjugate structures, triplet carbenes at zigzag edges, and surface functional groups such as carboxyl and oxygen-rich hydroxyl moieties (34).
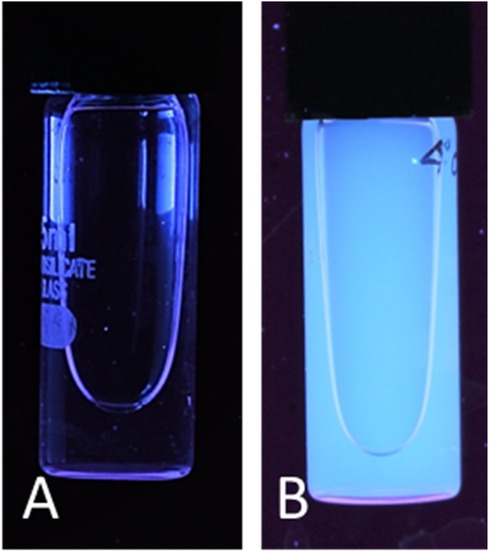
Figure 4. Carbon quantum dot photoluminescence in the presence of white light (A) and UV excitation (B).
3.2 Fluorescence spectroscopy analysis
The maximum photoluminescence emission observed from the citric acid derived carbon quantum dots was identified at an excitation wavelength of 320 nm, displaying an emission peak at 430 nm (Figure 5). The photoluminescence characterized by blue color observed in carbon dots (CDs) is significantly modulated by the existence of emissive trapping sites, the presence of triplet carbenes situated at the zigzag boundaries, the configuration of aromatic conjugated frameworks, and the incorporation of functional moieties such as carboxyl groups and hydroxyl groups that are abundant in oxygen, all of which contribute to this intricate phenomenon (31).
3.3 Fourier-transform infrared spectroscopy (FT-IR) analysis
The FTIR analysis conducted on the citric acid-derived carbon quantum dots (CQDs) elucidated the existence of N–H and O–H functional groups within the spectral range of 3,463–3,186 cm−1, along with a distinctive C=C bond, observed at 1,662 cm−1, while C–H stretching revealed at 2,920 cm−1. The spectrum also exhibited C–O–H vibration bands at 1,412 cm−1, C=O stretching at 1,600 cm−1, and C–O–C linkages at 1,069 cm−1 (Figure 6).
3.4 Scanning electron microscope analysis
SEM images illustrated the citric acid derived carbon quantum dots exhibited crumpled structures formed by precipitation, devoid of any visible dots due to their minute particle size and the tendency to agglomerate because of prolonged sample storage (Figure 7).
3.5 Enzymatic biodegradability of CQDs
In addition to demonstrating their biocompatibility, it is necessary to investigate the biodegradability of carbon quantum dots. Human myeloperoxidase, along with various other peroxidases, possesses the enzymatic capability to decompose the graphitic matrix of carbon quantum dots (CQDs) and other carbon-based nanomaterials via an oxidative mechanism (35). Therefore, an MPO-based peroxide system was used to investigate the oxidative biodegradation of CQDs. When CQDs were incubated for 24 h with 140 mM NaCl, 5 μg/ml MPO, and 100 μM H2O2. In addition, we also performed control experiments where we incubated the CQDs exclusively with H2O2 to investigate any potential structural changes. Now the aliquots of the various samples were taken at 0, 4, 12, and 24 h intervals during incubation. Using UV-Vis spectroscopy, the absorbance of these aliquots was measured at various time intervals, and it was discovered that absorption decreased with time (Figures 8, 9).
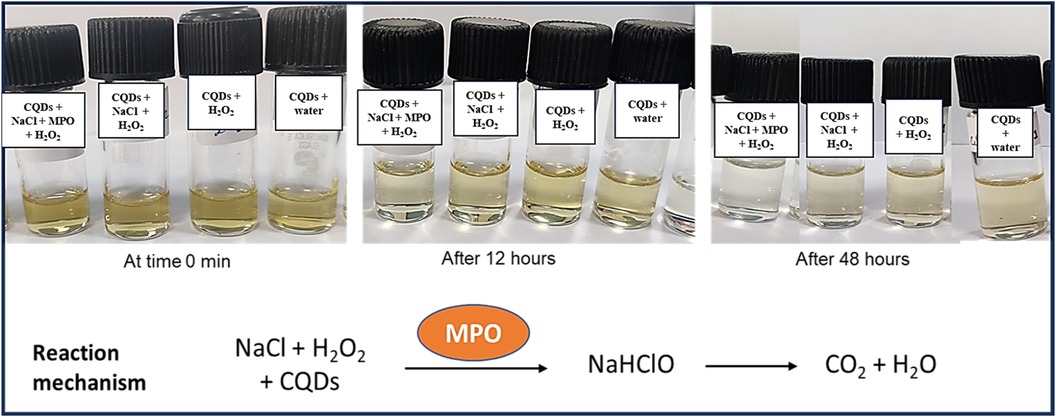
Figure 8. Incubation of CQDs with MPO, NaCl and H2O2 for different time intervals (0 min, 12 h, and 48 h).
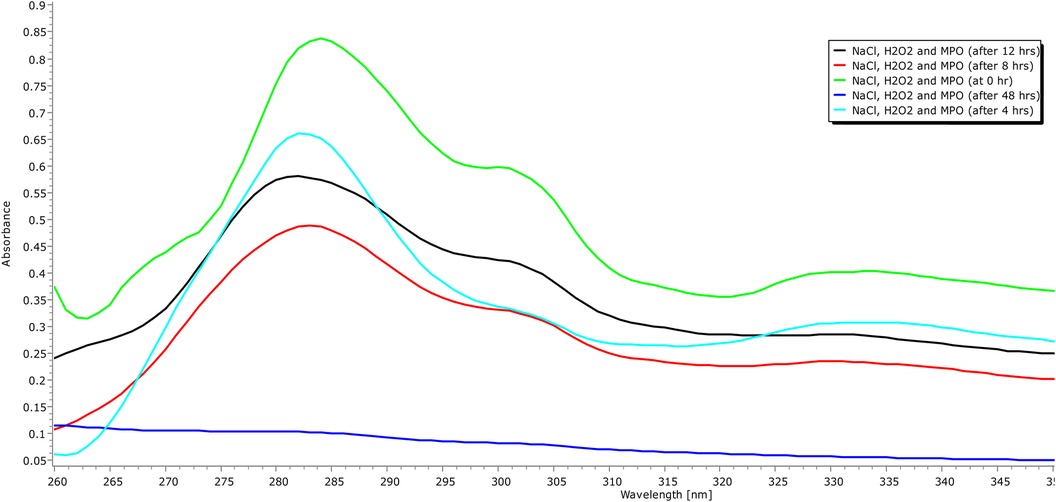
Figure 9. UVB absorption spectra (260–350 nm) of carbon quantum dots treated with H2O, H2O2, and human origin MPO, with NaCl, H2O2 for 24 h.
The oxidative degradation of the CQDs caused by H2O2 alone with NaCl was comparatively less than that caused by the H2O2-driven peroxide system, MPO, and NaCl (Figure 8). To facilitate the synthesis of sodium hypochlorous acid (NaHOCl), which subsequently disintegrates the graphitic lattice, myeloperoxidase (MPO) interacts with the hydroxyl (OH) groups located on the surface of CQDs situated in proximity to defect sites. The OH group that present on the surface of the CQDs had come from citric acid verified by FTIR spectra (Figure 6). This observation is particularly relevant in the context of the body's innate immune defence mechanism. The primary barrier against invading pathogens and foreign materials consists of resident macrophages and neutrophils, which produce a myeloperoxidase-mediated peroxide system (22).
Additionally, the relative fluorescence intensity of CQDs was assessed over time, revealing a significant reduction in each MPO enzyme-treated sample's fluorescent intensity compared to its respective control (Figure 10). The most significant decrease in fluorescence intensity occurred during the first 4 h of incubation, in contrast to the subsequent time intervals. This phenomenon can likely be attributed to the high concentration of oxygenated functional groups present on the surface of the CQDs, which enhances their water dispersibility and facilitates interaction with the enzyme (35). We propose that after degrading the defective regions of the CQDs, the enzyme began to break down the more graphitic areas, potentially explaining the decreased rate of degradation after 4 h.
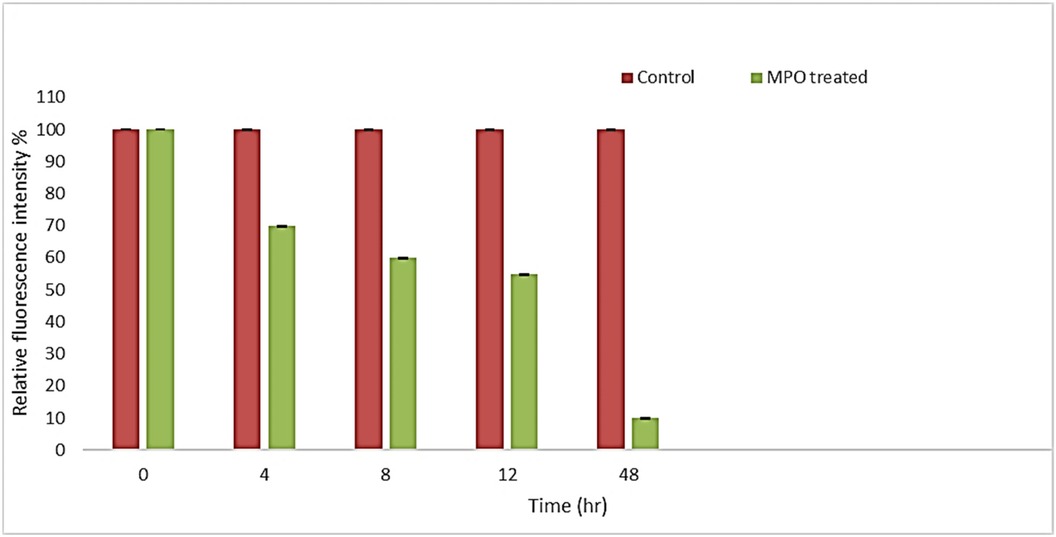
Figure 10. Fluorescence intensity of MPO treated and their respective control samples at different time intervals.
4 Conclusions
It was discovered that MPO based peroxide system significantly degraded the carbon quantum dots in the presence of H2O2 and MPO in the duration of the proposed experiment, (48 h). By using UV-vis spectroscopy, it was possible to confirm that the CQDs were biodegrading over time. At first, after 4 h of incubation, signs of degradation were seen, but after 48 h, they became more evident. Additionally, fluorescence intensity graph also confirmed that CQDs were degraded, and the fluorescence intensity decreased over time. Therefore, the results support the biocompatibility of CQDs for their use in various fields like biomedical and therapeutic applications.
Data availability statement
The original contributions presented in the study are included in the article/Supplementary Material, further inquiries can be directed to the corresponding author.
Author contributions
PS: Writing – original draft, Conceptualization, Methodology, Writing – review & editing. LS: Writing – review & editing.
Funding
The author(s) declare that no financial support was received for the research, authorship, and/or publication of this article.
Acknowledgments
The authors acknowledge the Department of Biochemical Engineering, School of Chemical Engineering, Harcourt Butler Technical University, Kanpur for providing infrastructure support to conduct the research work.
Conflict of interest
The authors declare that the research was conducted in the absence of any commercial or financial relationships that could be construed as a potential conflict of interest.
Publisher's note
All claims expressed in this article are solely those of the authors and do not necessarily represent those of their affiliated organizations, or those of the publisher, the editors and the reviewers. Any product that may be evaluated in this article, or claim that may be made by its manufacturer, is not guaranteed or endorsed by the publisher.
References
1. Bhattacharya K, Mukherjee SP, Gallud A, Burkert SC, Bistarelli S, Bellucci S. Biological interactions of carbon-based nanomaterials: from coronation to degradation. Nanomedicine. (2016) 12(2):333–51. doi: 10.1016/j.nano.2015.11.011
2. Zheng XT, Ananthanarayanan A, Luo KQ, Chen P. Glowing graphene quantum dots and carbon dots: properties, syntheses, and biological applications. Small. (2015) 11(14):1620–36. doi: 10.1002/smll.201402648
3. Nel A, Xia T, Madler L, Li N. Toxic potential of materials at the nanolevel. Science. (2006) 311(5761):622–7. doi: 10.1126/science.1114397
4. Chung CY, Chen YJ, Kang CH, Lin HY, Huang CC, Hsu PH, et al. Toxic or not toxic, that is the carbon quantum dot’s question: a comprehensive evaluation with zebrafish embryo, eleutheroembryo, and adult models. Polymers. (2021) 13(10):1598. doi: 10.3390/polym13101598
5. Attia H, Nounou H, Shalaby M. Zinc oxide nanoparticles induced oxidative DNA damage, inflammation and apoptosis in rat’s brain after oral exposure. Toxics. (2018) 6(2):29. doi: 10.3390/toxics6020029
6. Zhu S, Meng Q, Wang L, Zhang J, Song Y, Jin H, et al. Highly photoluminescent carbon dots for multicolor patterning, sensors, and bioimaging. Angew Chem. (2013) 125(14):4045–9. doi: 10.1002/ange.201300519
7. Dordevic L, Arcudi F, Cacioppo M, Prato M. A multifunctional chemical toolbox to engineer carbon dots for biomedical and energy applications. Nat Nanotechnol. (2022) 17(2):112–30. doi: 10.1038/s41565-021-01051-7
8. Vlasova II, Kapralov AA, Michael ZP, Burkert SC, Shurin MR, Star A, et al. Enzymatic oxidative biodegradation of nanoparticles: mechanisms, significance, and applications. Toxicol Appl Pharmacol. (2016) 299:58–69. doi: 10.1016/j.taap.2016.01.002
9. Jafar M. Carbon quantum dots and their biomedical and therapeutic applications: a review. RSC Adv. (2019) 9:6460–81. doi: 10.1039/C8RA08088G
10. Azam N, Najabat Ali M, Javaid Khan T. Carbon quantum dots for biomedical applications: review and analysis. Front Mater. (2021) 8:700403. doi: 10.3389/fmats.2021.700403
11. Butkevich E, Verma NC, Oleksiievets N, Gregor I, Schmidt CF, Enderlein J, et al. Carbon dots for studying muscle architecture. ACS Appl Nano Mater. (2019) 2(12):7466–72. doi: 10.1021/acsanm.9b01815
12. Madannejad R, Shoaie N, Jahanpeyma F, Darvishi MH, Azimzadeh M, Javadi H. Toxicity of carbon-based nanomaterials: reviewing recent reports in medical and biological systems. Chem Biol Interact. (2019) 307:206–22. doi: 10.1016/j.cbi.2019.04.036
13. Singh P, Singh S, Nara S. Nanotechnology as a tool for contaminants detection in milk or milk products. In: Nascimento RFd, Neto VdOS, Fechine PBA, Freire PdTC, editors. Nanomaterials and Nanotechnology. Materials Horizons: From Nature to Nanomaterials. Singapore: Springer (2021). doi: 10.1007/978-981-33-6056-3_5
14. Fadeel B, Bussy C, Merino S, Vázquez E, Flahaut E, Mouchet F, et al. Safety assessment of graphene-based materials: focus on human health and the environment. ACS Nano. (2018) 12(11):10582–620. doi: 10.1021/acsnano.8b04758
15. Boraschi D, Italiani P, Palomba R, Decuzzi P, Duschl A, Fadeel B, et al. Nanoparticles and innate immunity: new perspectives on host defence. Semin Immun. (2017) 34:33–51. doi: 10.1016/j.smim.2017.08.013
16. Kotchey GP, Hasan SA, Kapralov AA, Ha SH, Kim K, Shvedova AA, et al. A natural vanishing act: the enzyme-catalyzed degradation of carbon nanomaterials. Acc Chem Res. (2012) 45(10):1770–81. doi: 10.1021/ar300106h
17. Kurapati R, Russier J, Squillaci MA, Treossi E, Ménard-Moyon C, Esaú A, et al. Dispersibility-dependent biodegradation of graphene oxide by myeloperoxidase. Small. (2015) 11(32):3985–94. doi: 10.1002/smll.201500038
18. Lalwani G, Xing W, Sitharaman B. Enzymatic degradation of oxidized and reduced graphene nanoribbons by lignin peroxidase. J Mater Chem B. (2014) 2(37):6354–62. doi: 10.1039/C4TB00976B
19. Allen BL, Kotchey GP, Chen Y, Yanamala NV, Klein-Seetharaman J, Kagan VE, et al. Mechanistic investigations of horseradish peroxidase-catalyzed degradation of single-walled carbon nanotubes. J Am Chem Soc. (2009) 131(47):17194–205. doi: 10.1021/ja9083623
20. Rizo-Téllez SA, Sekheri M, Filep JG. Myeloperoxidase: regulation of neutrophil function and target for therapy. Antioxidants. (2022) 11(11):2302. doi: 10.3390/antiox11112302
21. Azuara Tuexi G, Méndez JA, Munoz-Sandoval E, Guirado-Lopez R. Myeloperoxidase-induced degradation of N-doped carbon nanotubes: revealing possible atomistic mechanisms underlying hypochlorite-driven damage of nanotube walls. Carbon. (2021) 175:387–402. doi: 10.1016/j.carbon.2021.01.117
22. Kagan VE, Konduru NV, Feng W, Allen BL, Conroy J, Volkov Y, et al. Carbon nanotubes degraded by neutrophil myeloperoxidase induce less pulmonary inflammation. Nat Nanotechnol. (2010) 5(5):354–9. doi: 10.1038/nnano.2010.44
23. Klebanoff SJ. Myeloperoxidase: friend and foe. J Leukoc Biol. (2005) 77(5):598–625. doi: 10.1189/jlb.1204697
24. Malle E, Furtmüller PG, Sattler W, Obinger C. Myeloperoxidase: a target for new drug development? Br J Pharmacol. (2007) 152(6):838–54. doi: 10.1038/sj.bjp.0707358
25. Parker H, Albrett AM, Kettle AJ, Winterbourn CC. Myeloperoxidase associated with neutrophil extracellular traps is active and mediates bacterial killing in the presence of hydrogen peroxide. J Leukocyte Biol. (2011) 91(3):369–76. doi: 10.1189/jlb.0711387
26. Jiang C, Wu H, Song X, Ma X, Wang J, Tan M. Presence of photoluminescent carbon dots in Nescafe® original instant coffee: applications to bioimaging. Talanta. (2014) 127:68–74. doi: 10.1016/j.talanta.2014.01.046
27. Shukla D, Pandey FP, Kumari P, Basu N, Tiwari MK, Lahiri J, et al. Label-free fluorometric detection of adulterant malachite green using carbon dots derived from the medicinal plant source ocimum tenuiflorum. Chem Select. (2019) 4(17):4839–47. doi: 10.1002/slct.201900530
28. Wu J, Chen T, Ge S, Fan W, Wang H, Zhang Z, et al. Synthesis and applications of carbon quantum dots derived from biomass waste: a review. Environ Chem Lett. (2023) 21(6):3393–424. doi: 10.1007/s10311-023-01636-9
29. Qu S, Wang X, Lu Q, Liu X, Wang L. A biocompatible fluorescent ink based on water-soluble luminescent carbon nanodots. Angew Chem Int Ed. (2012) 51(49):12215. doi: 10.1002/anie.201206791
30. Sendao R, de Yuso MDVM, Algarra M, da Silva JCE, da Silva LP. Comparative life cycle assessment of bottom-up synthesis routes for carbon dots derived from citric acid and urea. J Cleaner Prod. (2020) 254:120080. doi: 10.1016/j.jclepro.2020.120080
31. Balkrishna A, Tomar M, Bhattacharya K, Varshney A. Withania somnifera-derived carbon dots protect human epidermal cells against UVB-induced cell death and support growth factor-mediated wound healing. Nanoscale Adv. (2023) 5(5):1331–44. doi: 10.1039/D2NA00545J
32. Mukherjee SP, Gliga AR, Lazzaretto B, Brandner B, Fielden M, Vogt C, et al. Graphene oxide is degraded by neutrophils and the degradation products are non-genotoxic. Nanoscale. (2018) 10(3):1180–8. doi: 10.1039/C7NR03552G
33. Hampton MB, Kettle AJ, Winterbourn CC. Inside the neutrophil phagosome: oxidants, myeloperoxidase, and bacterial killing. Blood. (1998) 92(9):3007–17. doi: 10.1182/blood.V92.9.3007
34. Mochalin VN, Gogotsi Y. Wet chemistry route to hydrophobic blue fluorescent nanodiamond. J Am Chem Soc. (2009) 131(13):4594–5. doi: 10.1021/ja9004514
Keywords: myeloperoxidase enzyme, photoluminescence, hypochlorous acid, biodegradation, peroxidase, neutrophil granulocytes, carbon quantum dots
Citation: Singh P and Singh LK (2024) Myeloperoxidase enzyme-catalyzed breakdown of zero-dimension carbon quantum dots. Front. Med. Technol. 6:1493288. doi: 10.3389/fmedt.2024.1493288
Received: 8 September 2024; Accepted: 31 October 2024;
Published: 28 November 2024.
Edited by:
Bijayananda Panigrahi, Biopioneer Pvt. Ltd., IndiaReviewed by:
Bhaskar Das, KIIT University, IndiaAkhilesh Kumar Gupta, University of Nebraska Omaha, United States
Copyright: © 2024 Singh and Singh. This is an open-access article distributed under the terms of the Creative Commons Attribution License (CC BY). The use, distribution or reproduction in other forums is permitted, provided the original author(s) and the copyright owner(s) are credited and that the original publication in this journal is cited, in accordance with accepted academic practice. No use, distribution or reproduction is permitted which does not comply with these terms.
*Correspondence: Lalit Kumar Singh, bGt1bWFyQGhidHUuYWMuaW4=