- Artificial Cells & Organs Research Centre, Department of Physiology, Medicine and Biomedical Engineering, Faculty of Medicine and Health Sciences, McGill University, Montreal, QC, Canada
Editorial on the Research Topic
Innovative medical technology based on artificial cells, including its different configurations
1. Introduction
Artificial cell is a highly interdisciplinary area involving medicine, chemistry, bioengineering, biotechnology, biochemistry, and other areas. The highly interdisciplinary approach is such that this Research Topic includes the following participating Frontiers journals: Frontiers in Medical Technology, Frontiers in Bioengineering and Biotechnology, Frontiers in Medicine, Frontiers in Oncology, Frontiers in Molecular Biosciences, and Frontiers in Pediatrics.
Red blood cells (rbc) are one of the most important cells since without them, our organs, tissues and cells cannot survive. The first artificial cell prepared is artificial rbc (1, 2). (Figure 1). By going outside the box, we and others are able to extend this to prepare artificial cells of unlimited configurations and contents (2–6). (Figure 1) resulting in large areas of applications (2–6) (Figure 1).
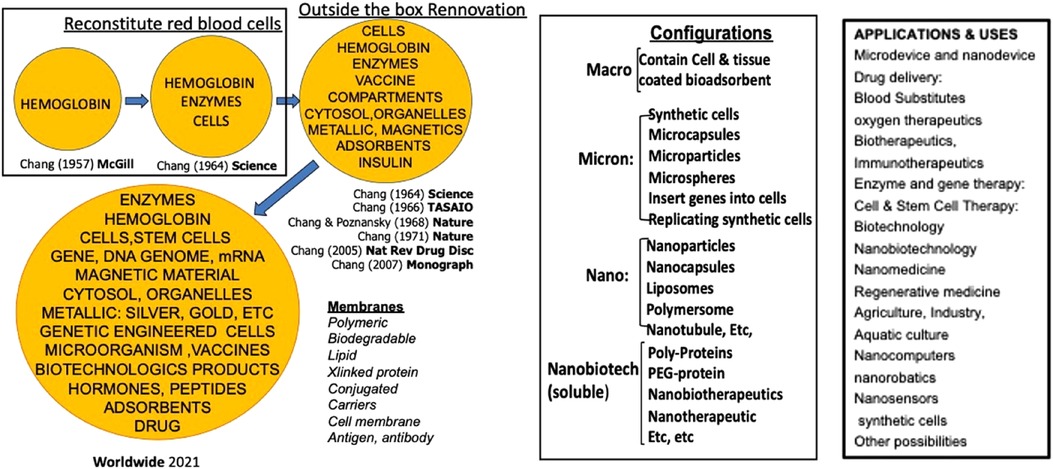
Figure 1. Left: the basic idea of artificial cells evolved into “out of the box variation in contents and membrane materials.” middle: the basic idea of artificial cells extended into different configurations. Right: Examples of uses of artificial cells [Figure from Chang (5) with written copyright permission from the publisher Taylor and Frances].
Many of the ideas of artificial cells are being extensively applied and extended by us and by researchers worldwide, resulting in exciting progress and applications (Figure 1). Artificial cell is too large an area to be covered under this journal Research Topic. Artificial red blood cells or blood substitute alone already require a >1,000-page multi-author books (7). Many other areas of artificial cells are in development or routine clinical use. For this Research Topic we shall concentrate on artificial red blood cells as the basis for novel and innovative medical technology and showing how outside the box approaches can lead to innovation application.
2. Artificial red blood cell (blood substitutes)
Red blood cells have 3 major functions: 1. Oxygen Carrier 2. Antioxidant functions and 3. transport of carbon dioxide. There was no initial public interest to develop artificial rbc when it was published in 1964 (2). It was the 1980s HIV contamination of donor blood that led to the belated effort to develop a suitable blood substitute.
2.1. Oxygen carrier
The urgency was such that researchers concentrated on just an oxygen carrier. In the form of hemoglobin-based oxygen carriers (7). Hemoglobin, a tetramer, is an excellent oxygen carrier. However, in the body it is converted into toxic dimers. Chang used diacid (2) or glutaraldehyde (8) to crosslink hemoglobin into polyhemoglobin (PolyHb) and prevent its breakdown into toxic dimers. This has been developed and tested in clinical trials. A glutaraldehyde crosslinked bovine polyhemoglobin has been approved for routine clinical use for surgical procedures in South Africa and Russia to avoid the use of HIV contaminated donor blood (9). Thus, the original aim has been reached as described in Jahr's article on Blood substitutes: Basic science, translational studies and clinical trials.
Polyhemoglobins do not have blood group. Moore et al. reported their clinical trials with glutaraldehyde crosslinked human PolyHb. They showed that this could be given right In the ambulance for patients with hemorrhagic shock without the need for cross matching. This was more effective than the saline control group. They reported a very slight increase in non-fatal myocardial ischemia (10). This could be due to a number of reasons as discussed by Alayash in their article Oxidation reactions of cellular and acellular hemoglobins: Implications for human health and Williams et al. in their article Renal glomerular and tubular responses to glutaraldehyde- polymerized human hemoglobin.
2.2. Oxygen carrier with antioxidant functions
For those conditions with ischemia-reperfusion, one would need an oxygen carrier with antioxidant properties. Thus, our approach of polyhemoglobin-catalase-superoxide dismutase (PolyHb-CAT-SOD) prevents cerebral edema in hemorrhagic shock with cerebral ischemia (11, 12). Another oxygen carrier with antioxidant showed similar results. This is reported by Jun Wang et al.'s group in Polynitroxylated PEGylated hemoglobin protects pig brain neocortical gray and white matter after traumatic brain injury and hemorrhagic shock.
2.3. Oxygen carrier with antioxidant property and CO2 transport
Another possible problem is an increase in intracellular pCO2 in severe hemorrhagic shock (13, 14). We therefore added an enhanced level of carbonic anhydrase (CA) to prepare a PolyHb-CAT-SOD-CA. The result is an oxygen carrier with enhanced Carbonic Anhydrase for CO2 transport and enhanced Catalase and Superoxide Dismutase for antioxidant functions (15). This is reported by Bian and Chang in this Research Topic. Hoq and Chang used more stable chemical CAT, SOD and CA instead of biological enzymes for certain uses as reported in the Research Topic article Preliminary feasibility study using a solution of synthetic enzymes to replace the natural enzymes in polyhemoglobin-catalase-superoxide dismutase-carbonic anhydrase: effect on warm ischemic hepatocyte cell culture.
2.4. Other approaches
Many other approaches are being explored (7). This Research Topic shows two examples. One is Kettisen et al.'s group in Structural and oxidative investigation of a recombinant high-yielding fetal hemoglobin mutant. Another is Sakai et al.'s group in Research of storable and ready-to-use artificial red blood cells (hemoglobin vesicles) for emergency medicine and other clinical applications. Biodegradable polymeric membrane nano-rbc is also possible (16).
2.5. Preservation of pretransplant cells and organs: regenerative medicine
A very promising and exciting area is the use of blood substitute in the preservation of cells and organs for transplantation. Zal's murine HEMARINA has been approved in the EU for the pretransplant preservation of human kidneys (17).
Active research continues in this exciting application. A number of years ago we show that oxygen carrier with antioxidants is effective for the preservation of rat small intestine and hepatocytes (18). Andrijevic et al. reported their detailed study in Nature (2022) (19). In this Research Topic, Li et al. describe their work in Application of polymerized porcine hemoglobin in the ex vivo normothermic machine perfusion of rat livers alongside Shen et al. in The role of normothermic machine perfusion (NMP) in the preservation of ex-vivo liver before transplantation: A review.
3. Other areas
Artificial rbc or blood substitutes is but a very small area of artificial cells. Much larger areas of uses have been reviewed elsewhere (Figure 1) (4, 5). A very brief summary of some of these follows.
1. Hemoperfusion: Artificial cells containing bioactive material for hemoperfusion (20–22). One example is the. routine clinical use of artificial cells containing adsorbent for the removal of waste metaboletes and drug poisoning (21,22) and in cytokine storm of severe COVID-19 (23). It also has other uses like the treatment for immunological disease as reported in this Research Topic in The development of immunosorbents for the treatment of systemic lupus erythematosus via hemoperfusion by Yu and Ou.
2. Delivery system: Artificial cells in different configurations have used successfully as drug carriers (4, 5, 24, 25). A recent report is Dhasmana et al.’s Fabrication and evaluation of herbal beads to slow cell ageing.
3. Artificial Cells in the fight against COVID: The potential of artificial cells as carrier for vaccine has been proposed many years ago (24, 26, 27). Artificial cells prevent mRNA from inactivation by body enzymes and allow it to carry out its function as COVID-19 vaccine (26, 28). Artificial cells based hemoperfusion has also been used to lower the elevated toxic level of cytokines in patients with severe COVID-19 (23, 26).
4. Hereditary enzyme deficiency: We first show that catalase artificial cells can replace the deficient enzyme in hereditary catalase deficient mice (29). Use in patients only became possible when stable simple enzymes became available for artificial cells. This allowed us to treat a patient with Lesch Nyhan disease using artificial cells contain xanthine oxidase (30, 31). We also use artificial cells containing Phenylalanine ammonia lyase (PAL) to treat rats with Phenylketonuria (32). A company collaborated with us to prepared less expensive recombinant PAL which was used by another company to prepare PEG-PAL that has been approved by FDA for adult PKU patients (33).
5. Cancer therapy: We first reported the use of artificial cells containing asparaginase for the suppression of lymphosarcoma in mice (35). This is now being used in patients in the form of PEG-asparaginase. We are using artificial cell containing polyHb-tyrosinase in mice with melanoma (37, 38) Soltys et al.’s group reports in this Research topic in Inhibition of metastatic brain cancer in Sonic Hedgehog medulloblastoma using caged nitric oxide albumin nanoparticles.
6. Other areas: Other areas of uses include cell/stem cell therapy and regenerative medicine (39–43), encapsulated microbe (44–46) industry, agriculture, aquatic culture (4, 5, 49), synthetic cells (47), nanomedicine, biotherapeutics, gene therapy, nano-robotics and others (Figure 1) (4, 5, 49).
Summary
Artificial cells can be prepared with extensive variations in terms of their contents, membranes, dimensions, and configurations. This allows for an array of promising and innovative medical applications. In this Research Topic, we will start with a detailed discussion of the current status and future prospects of artificial red blood cells. Notable developments in this area include hemoglobin-based oxygen carriers, which have been approved for use in surgical patients by two countries. Additionally, researchers are using natural and synthetic enzymes to form oxygen carriers with antioxidant properties or those with CO2 transport and antioxidant properties. Other approaches include bioengineered hemoglobin and hemoglobin vesicles. Another active area of research centers on the preservation of tissues and cells, with Hemarina having received approval in the EU for the pretransplant preservation of human kidneys. Brief discussion of other notable innovative applications of artificial cells encompasses hemoperfusion, delivery systems, COVID-19 vaccines, cancer therapy, and hereditary enzyme defects. The possibilities extend beyond these, as listed in Figure 1, encompassing nanomedicine, biotherapeutics, gene therapy, regenerative medicine, cell and stem cell therapies, and nanorobotics.
Author contributions
TC: Conceptualization, Data curation, Formal analysis, Investigation, Methodology, Project administration, Resources, Supervision, Validation, Writing – original draft, Writing – review & editing, Funding acquisition.
Funding
The author(s) declare that no financial support was received for the research, authorship, and/or publication of this article.
Conflict of interest
The author declare that the research was conducted in the absence of any commercial or financial relationships that could be construed as a potential conflict of interest.
Publisher's note
All claims expressed in this article are solely those of the authors and do not necessarily represent those of their affiliated organizations, or those of the publisher, the editors and the reviewers. Any product that may be evaluated in this article, or claim that may be made by its manufacturer, is not guaranteed or endorsed by the publisher.
References
1. Chang TMS. (1957) 1957 Report on “method for preparing artificial hemoglobin corpuscles”. BSc thesis. McGill University: Montreal, QC. Available at: http://www.medicine.mcgill.ca/artcell/514.pdf
2. Chang TMS. Semipermeable microcapsules. Science. (1964) 146:524–5. doi: 10.1126/science.146.3643.524
3. Chang TMS. Artificial cells. Springfield, IL: Charles C Thomas Publisher. (1972). p. 1–207. Available at: www.artcell.mcgill.ca/1972bookcovercr.pdf
4. Chang TMS. Monograph on “ARTIFICIAL CELLS: Biotechnology, nanotechnology, blood substitutes, regenerative medicine, bioencapsulation, cell/stem cell therapy” Singapore/London: World Scientific Publisher/Imperial College Press (2007). p. 435. Available at: http://www.medicine.mcgill.ca/artcell/2007%20ebook%20artcell%20web.pdf
5. Chang TMS. ARTIFICIAL CELL evolves into nanomedicine, biotherapeutics, blood substitutes, drug delivery, enzyme/gene theraphy, cancer therapy, cell/stem cell therapy, nanoparticles, liposomes, bioencapsulation, replicating synthetic cells, cell encapsulation/scaffold, biosorbent/immunosorbent haemoperfusion/plasmapheresis, regenerative medicine, encapsulated microbe, nanobiotechnology, nanotechnology. Artif Cells Nanomed Biotechnol. (2019) 47(1):997–1013. doi: 10.1080/21691401.2019.1577885
6. Abuchowski A, Kazo GM, Jr VC, Van Es T, Kafkewitz D, Nucci ML, et al. Cancer therapy with chemically modified enzymes. I. antitumor properties of polyethylene glycol-asparaginase conjugates. Cancer Biochem Biophys. (1984) 7(2):175–86.6467175
7. Chang TMS, Bulow L, Jahr J, Sakai H, Yang CM. (editors) Nanobiotherapeutic based blood substitutes. World Scientific Publisher/Imperial College (2021). p. 1040 doi: 10.1142/12054
8. Chang TMS. Stabilisation of enzymes by microencapsulation with a concentrated protein solution or by microencapsulation followed by cross-linking. BBRC. (1971) 44(6):1531–36. doi: 10.1016/S0006-291X(71)80260-7
9. Mer M, Hodgson E, Wallis L, Jacobson B, Levien L, Snyman J, et al. Hemoglobin glutamer-250 (bovine) in South Africa: consensus usage guidelines from clinician experts who have treated patients. Transfusion. (2016) 56(10):2631–6. doi: 10.1111/trf.13726
10. Moore EE, Moore FA, Fabian TC, Bernard AC, Fulda GJ, Hoyt DB, et al. Human polymerized hemoglobin for the treatment of hemorrhagic shock when blood is unavailable: the USA multicenter trial. J Am Coll Surg. (2009) 208(1):1–13. doi: 10.1016/j.jamcollsurg.2008.09.023
11. D'Agnillo F, Chang TM. Polyhemoglobin-superoxide dismutase-catalase as a blood substitute with antioxidant properties. Nat Biotechnol. (1998) 16(7):667–71. doi: 10.1038/nbt0798-667
12. Powanda DD, Chang TM. Cross-linked polyhemoglobin-superoxide dismutase-catalase supplies oxygen without causing blood-brain barrier disruption or brain edema in a rat model of transient global brain ischemia-reperfusion. Artif Cells Blood Substit Immobil Biotechnol. (2002) 30(1):23–37. doi: 10.1081/bio-120002725
13. Sims C, Seigne P, Menconi M, Monarca J, Barlow C, Pettit J, et al. Skeletal muscle acidosis correlates with the severity of blood volume loss during shock and resuscitation. J Trauma. (2001) 51(6):1137–45. doi: 10.1097/00005373-200112000-00020
14. Tronstad C, Pischke SE, Holhjem L, Tønnessen TI, Martinsen OG, Grimnes S. Early detection of cardiac ischemia using a conductometric pCO(2) sensor: real-time drift correction and parameterization. Physiol Meas. (2010) 31(9):1241–55. doi: 10.1088/0967-3334/31/9/013
15. Bian Y, Chang TMS. A novel nanobiotherapeutical poly-[hemoglobin-superoxide dismutase-catalase-carbonic anhydrase] with no cardiac toxicity for the resuscitation of a 90 min sustained severe hemorrhagic shock rat model with 2/3 blood volume loss. Artif Cells Nanomedicine and Biotechnology. (2015) 43(1):1–9. doi: 10.1142/9789811228698_0025
16. Chang TM, Powanda D, Yu WP. Analysis of polyethylene-glycol-polylactide nano-dimension artificial red blood cells in maintaining systemic hemoglobin levels and prevention of methemoglobin formation. Artif Cells Blood Substit Immobil Biotechnol. (2003) 31(3):231–47. doi: 10.1081/bio-120023155
17. Atlanpole Biotherapies and Zal F. (2022) The biotechnology company HEMARINA obtains the CE mark for its HEMO2life® technology. Available at: https://www.atlanpolebiotherapies.eu/news/the-biotechnology-company-hemarina-obtains-the-ce-mark-for-its-hemo2life-technology/
18. Chang TMS, Razack S, Jiang W, D’Agnillo F. Nanobiotherapeutics as preservation fluids for organs and cells. In: Chang TMS, Jiang W, Razack S, editors. Nanobiotherapeutic based blood substitutes. Singapore/London: World Science Publisher (2021). p. 959–76. doi: 10.1142/9789811228698_0044
19. Andrijevic D, Vrselja Z, Lysyy T, Zhang S, Skarica M, Spajic A, et al. Cellular recovery after prolonged warm ischaemia of the whole body. Nature. (2022) 608(7922):405–12. doi: 10.1038/s41586-022-05016-1
20. Chang TMS. Semipermeable aqueous microcapsules (“artificial cells”): with emphasis on experiments in an extracorporeal shunt system. Trans Am Soc Artif Intern Organs. (1966) 12:13–9.5960694
21. Chang TMS, Coffey JF, Barre P, Gonda A, Dirk J, Levy M, et al. Microcapsule artificial kidney:treatment of patients with acute drug intoxication. Can Med Assoc J. (1973) 108:429–33.4405716
22. Chang TMS, Endo Y, Nicolaev VG, Tani T, Zheng Y, editors. Hemoperfusion and plasma-perfusion and other clinical uses of general, biospecific, immune and leucocyte adsorbents. Regenerative medicine, artificial cells and nanomedicine chapter 1. World Scientific Publisher/Imperial College Press (2017). p. 1004. Available at: www.medicine.mcgill.ca/artcell/HPBk_Ch1.pdf
23. Zhou F, Yu T, Du R, Fan G, Liu Y, Liu Z, et al. Clinical course and risk factors for mortality of adult inpatients with COVID-19 in Wuhan, China: a retrospective cohort study. Lancet. (2020) 395(10229):1054–62. doi: 10.1016/S0140-6736(20)30566-3
24. Chang TM. Biodegradable semipermeable microcapsules containing enzymes, hormones, vaccines, and other biologicals. J Bioeng. (1976) 1(1):25–32.1052520
25. Torchilin VP. Recent advances with liposomes as pharmaceutical carriers. Nat Rev Drug Discov. (2005) 4(2):145–60. doi: 10.1038/nrd1632
26. Chang TMS. The role of artificial cells in the fight against COVID-19: deliver vaccine, hemoperfusion removes toxic cytokines, nanobiotherapeutics lower free radicals and pCO2 and replenish blood supply. Artif Cells Nanomed Biotechnol. (2022) 50(1):240–51. doi: 10.1080/21691401.2022.2126491
27. Chang TMS. The one-shot vaccine. In: HEDEN C-G, editors. “Socio-Economic and ethical implications of enzyme engineering”, Stockholm, Sweden: International Federation of Institutes for Advanced Studies (1975). p. 17–8.
28. Kulkarni JA, Witzigmann D, Thomson SB, Chen S, Leavitt BR, Cullis PR, et al. The current landscape of nucleic acid therapeutics. Nat Nanotechnol. (2021) 16(6):630–43. doi: 10.1038/s41565-021-00898-0
29. Chang TM, Poznansky MJ. Semipermeable microcapsules containing catalase for enzyme replacement in acatalasaemic mice. Nature. (1968) 218(5138):243–5. doi: 10.1038/218243a0
30. Chang TM. Preparation and characterisation of xanthine oxidase immobilised by microencapsulation in artificial cells for the removal of hypoxanthine. Biomater Artif Cells Artif Organs. (1989) 17(5):611–6. doi: 10.3109/10731198909117640
31. Palmour RM, Goodyer P, Reade T, Chang TM. Microencapsulated xanthine oxidase as experimental therapy in lesch-nyhan disease. Lancet. (1989) 2(8664):687–8. doi: 10.1016/s0140-6736(89)90939-2
32. Bourget L, Chang TM. Phenylalanine ammonia-lyase immobilized in microcapsules for the depletion of phenylalanine in plasma in phenylketonuric rat model. Biochim Biophys Acta. (1986) 883(3):432–8. doi: 10.1016/0304-4165(86)90281-3
33. U.S. Food and Drug Administration. FDA approves a new treatment for PKU, a rare and serious genetic disease (2018). Available at: https://www.fda.gov/news-events/press-announcements/fda-approves-new-treatment-pku-rare-and-serious-genetic-disease
34. Mahan KC, Gandhi MA, Anand S. Pegvaliase: a novel treatment option for adults with phenylketonuria. Curr Med Res Opin. (2019) 35(4):647–51. doi: 10.1080/03007995.2018.1528215
35. Chang TM. The in vivo effects of semipermeable microcapsules containing L-asparaginase on 6C3HED lymphosarcoma. Nature. (1971) 229(5280):117–8. doi: 10.1038/229117a0
36. Wetzler M, Sanford BL, Kurtzberg J, DeOliveira D, Frankel SR, Powell BL, et al. Effective asparagine depletion with pegylated asparaginase results in improved outcomes in adult acute lymphoblastic leukemia: cancer and leukemia group B study 9511. Blood. (2007) 109(10):4164–7. doi: 10.1182/blood-2006-09-045351
37. Yu B, Swi Chang TM. In vitro and in vivo effects of polyhaemoglobin-tyrosinase on murine B16F10 melanoma. Melanoma Res. (2004) 14(3):197–202. doi: 10.1097/01.cmr.0000131013.71638.c0
38. Wang Y, Chang TMS. A polymer–lipid membrane artificial cell nanocarrier containing enzyme–oxygen biotherapeutic inhibits the growth of B16F10 melanoma in 3D culture and in a mouse model. Artificial Cells, Nanomedicine & Biotechnology. (2021) 49:461–70. doi: 10.1080/21691401.2021.1918134
39. Lim F, Sun AM. Microencapsulated islets as bioartificial endocrine pancreas. Science. (1980) 210(4472):908–10. doi: 10.1126/science.6776628
40. Wong H, Chang TM. Bioartificial liver: implanted artificial cells microencapsulated living hepatocytes increases survival of liver failure rats. Int J Artif Organs. (1986) 9(5):335–6. doi: 10.1177/039139888600900515
41. Liu ZC, Chang TM. Transdifferentiation of bioencapsulated bone marrow cells into hepatocyte-like cells in the 90% hepatectomized rat model. Liver Transpl. (2006) 12(4):566–72. doi: 10.1002/lt.20635
42. Liu ZC, Chang TMS. Intrasplenic transplantation of bioencapsulated mesenchymal stem cells enhances the regeneration of remnant livers and improves the recovery rates of 90% partial hepatectomized rats. Stem Cells Int. (2012) 12:697094. doi: 10.1155/2012/697094
43. Grant R, Hay D, Callanan A. From scaffold to structure: the synthetic production of cell derived extracellular matrix for liver tissue engineering. Biomed Phys Eng Express. (2018) 4(6):065015. doi: 10.1088/2057-1976/aacbe1
44. Prakash S, Chang TM. Microencapsulated genetically engineered live E. coli DH5 cells administered orally to maintain normal plasma urea level in uremic rats. Nat Med. (1996) 2(8):883–7. doi: 10.1038/nm0896-883
45. Chow KM, Liu ZC, Prakash S, Chang TM. Free and microencapsulated Lactobacillus and effects of metabolic induction on urea removal. Artif Cells Blood Substit Immobil Biotechnol. (2003) 31(4):425–34. doi: 10.1081/bio-120025412
46. Iqbal U, Westfall S, Prakash S. Novel microencapsulated probiotic blend for use in metabolic syndrome: design and in-vivo analysis. Artificial Cells Nanomedicine and Biotechnology. (2018) 46(1):1–9.
47. Hutchison CA 3rd, Chuang RY, Noskov VN, Assad-Garcia N, Deerinck TJ, Ellisman MH, et al. Design and synthesis of a minimal bacterial genome. Science. (2016) 351(6280):aad6253. doi: 10.1126/science.aad6253
48. Poncelet D, Neufeld R. Bioencapsulation Research Group on industrial and agricultural applications. Available at: http://bioencapsulation.net
49. Chang TMS (2023) Artificial Cells, Blood Substitutes and Nanomedicine website containing reviews and monographs for free online viewing or download: Available at: www.medicine.mcgill.ca/artcell
Keywords: artificial cells, hemoglobin, antioxidant, carbonic anhydrase, nanobiotherapeutic, regeneration, enzyme therapy, COVID-19
Citation: Chang TMS (2023) Editorial: Innovative medical technology based on artificial cells, including its different configurations. Front. Med. Technol. 5:1306419. doi: 10.3389/fmedt.2023.1306419
Received: 3 October 2023; Accepted: 30 October 2023;
Published: 10 November 2023.
Edited and Reviewed by: Sarah Harriet Cartmell, The University of Manchester, United Kingdom
© 2023 Chang. This is an open-access article distributed under the terms of the Creative Commons Attribution License (CC BY). The use, distribution or reproduction in other forums is permitted, provided the original author(s) and the copyright owner(s) are credited and that the original publication in this journal is cited, in accordance with accepted academic practice. No use, distribution or reproduction is permitted which does not comply with these terms.
*Correspondence: Thomas M. Chang YXJ0Y2VsbC5tZWRAbWNnaWxsLmNh