- 1Biomedical Engineering, Gannon University, Erie, PA, United States
- 2Electrical and Computer Engineering, Gannon University, Erie, PA, United States
Introduction: Archers face a notable annual risk of injury attributed to the repetitive strain exerted on their upper body muscles and joints. This groundbreaking study marks the first utilization of real-time motion tracking combined with ergonomic analysis in archery research, eliminating the need for extensive equipment setups and streamlining data collection.
Methods: Thirteen participants were involved, undertaking four tasks with varying draw weights of bows and target positions.
Results: The precise recording of human movements revealed distinctive postures adopted by both genders across different tasks. Furthermore, an assessment of exposed spinal force and its correlation with anatomical variables was conducted, providing valuable insights into injury risks during archery performances.
1 Introduction
Prolonged and intense training, coupled with insufficient rest and recovery, is a known precursor to musculoskeletal injuries in athletes (Aicale et al., 2018). In the realm of archery, the repetitive strain placed on the upper body muscles and joints significantly increases the risk of injuries, such as cumulative fatigue and diminished neuromuscular control (Legend, 2023b). Furthermore, the combination of repetitive stress and inadequate time for tissue repair escalates the potential for both acute and chronic lesions (Barr and Barbe, 2002). These injuries not only impact archers’ immediate performance but also pose enduring threats to their physical health (Niestroj et al., 2017). Reports from the archery trade association (Management, 2015) indicate a relatively high annual injury rate, exceeding one percent per 1,000 participants. The continuous drawing and releasing of the bowstring, coupled with inadequate rest, can give rise to conditions such as rotator cuff injuries, tendonitis, string slap, and muscle strain injuries (Legend, 2023b). In elite archers, overuse injuries are most commonly observed in the shoulder, particularly in the drawing arm (Niestroj et al., 2017). Consequently, it is imperative to implement proper training techniques, incorporate sufficient rest periods, and adopt injury prevention strategies to optimize archers’ performance and mitigate the risk of musculoskeletal injuries.
Numerous prior studies have focused on analyzing the mechanical loads placed on the shoulders during archery. Electromyographic (EMG) techniques, as seen in some studies, were employed to gauge upper and lower trapezius activity (Liao et al., 2022). Advanced methodologies, including 3D modeling with CT and MRI imaging data, were utilized to simulate and reconstruct bone and rotator cuff muscles, providing insights into its mechanisms, due to the mechanical loading asymmetrically placed on both arms (Guo et al., 2022). Another study aimed to enhance archer performance by examining muscle activity and comparing shoulder kinematics between archers with shoulder impingement syndrome and uninjured counterparts (Shinohara et al., 2014).
Moreover, certain investigations focused on the back injuries incurred by archers. Notably, one study observed archers employing excessive arch in their lower back, leading to the overuse of incorrect lower-back muscles, ultimately impacting shot strength and technique. Another report emphasized the significance of back tension in archery, particularly in enhancing elite archers’ performance (Yi et al., 2007). However, we observed that there was a lack of comparison with alternative training interventions and potential performance bias making it more difficult to fully reflect a real archery scenario (Liao et al., 2022).
Furthermore, limitations such as a restricted sample size and lack of diversity reduced the statistical power and increased the sample error and the risk of bias. For instance, a study conducted solely on a 22-year-old male exemplifies the limited demographic representation (Guo et al., 2022). Additionally, a cross-sectional design in another study constrained the ability to establish a temporal relationship between shoulder kinematics and time (Shinohara et al., 2014).
The systematic review conducted by Vendrame et al. (2022) encompasses a comprehensive analysis of prior studies, which focus on utilizing EMG to explore the shooting movement of archers, maintaining optimal emotional conditions to enhance performance, tracking heart rate and nervous system responses during the shooting gesture, examining the archer’s balance both pre and post arrow release, and assessing the reaction time of archers. However, there remains a lack of studies involving the use of DHM specifically in archery.
Currently, the digital human modeling (DHM) technique finds widespread application across diverse research domains. In sports biomechanics, DHM has proven invaluable for analyzing and optimizing the biomechanics of athletes’ movements, encompassing the assessment of joint forces, muscle activation factors, and body kinematics in sports like golf, tennis, and swimming (Maurice et al., 2019). Beyond athletics, DHM has been employed in studies focusing on workplace ergonomics to prevent work-related musculoskeletal injuries by evaluating human performance (Ji et al., 2023c). Additionally, the DHM technique has played a crucial role in research related to automotive safety, simulating human responses in car crashes (Wang et al., 2021).
DHM stands out as a tool capable of creating highly detailed virtual human models that simulate real anatomical features, joint motions, and muscle activation properties (Duffy, 2012). Moreover, DHM provides an alternative to traditional data collection methods by avoiding the need for extensive equipment setups and reducing the time required for data collection (Schall Jr et al., 2018).
However, the setup process can extend to several hours in the traditional DHM technique even though a single simulation task may only demand a few seconds (Rhén et al., 2018), as every joint movement must manually input into the program. Thus, many of the previous studies were primarily focused on static pose analysis (Cao et al., 2013; Paul and Quintero Duran, 2015; Quintero-Duran and Paul, 2018), because it is a time-consuming process to imitate the realistic human movement. Additionally, the susceptibility to errors during setup is noteworthy, and even a minor deviation in joint angles within DHM posturing can lead to substantial discrepancies in estimated forces exposed on the lower back (Chaffin, 2005).
To comprehensively assess injury risks in the sports sector, it is imperative to use a simulation that represents full-body kinematics rather than relying on a sequence of disjointed static poses. This approach facilitates the identification of all potential awkward postures that could lead to injuries during task performance. Therefore, our study marks the pioneering effort in concentrating on injury assessment during archers’ performances, utilizing advanced motion tracking techniques and ergonomic tools. Employing the DHM technique to simulate the intricate motions involved in drawing and releasing the bowstring enables a precise identification of mechanisms that may contribute to archers’ injuries. Additionally, the integration of kinematic data onto the model significantly reduces the setup time compared to traditional DHM manual posturing. This technology effectively addresses a previously existing gap in the analysis of injury risks within the sports sector. Furthermore, this research not only offers archers the prospect of enhancing their performance through adjustments to improper poses but also lays the foundation for developing injury prevention programs. The analysis will focus on establishing correlations between key kinematic motion data and predicted spinal forces or joint torques, providing valuable insights for injury mitigation strategies.
2 Methods
2.1 Subjects and system setup
Participants must be in good health and free from any conditions that could impede the movement of their legs or arms. We recruited a total of thirteen college students with varying levels of experience in archery, primarily from the archery club. The participants’ ages ranged from 19 to 26 years. Each subject underwent guidance through the shooting sequence and was instructed to perform at their best, regardless of prior archery training. Each shooter was provided with an opportunity to warm up, and this warm-up routine was repeated every time they switched bows until they felt adequately prepared to begin. The range was overseen by a certified archery coach who provided guidance on the shooting procedure, ensuring participants were well-instructed on safe and methodical shooting practices beforehand.
This study enrolled thirteen participants, with average body weights of 75.4 ± 20.4 kg for males and 66.2 ± 17.2 kg for females. The average body heights were 174.7 ± 9.6 cm for males and 162.5 ± 7.5 cm for females. In the orientation phase, key segment lengths were measured to facilitate the creation of digital human models (DHMs). These measurements included arm span, shoulder and hip widths, as well as the lengths of the upper and lower limbs for each participant.
For each participant, two DHMs were created to analyze their kinematic outcomes and assess forces applied to the fourth/fifth (L4/L5) lumbar spine. The DHM_Xsens was generated within the Xsens MVN Analyze software (Xsens, 2022) to capture movements, while the DHM_JACK was generated through the JACK Siemens PLM software (Siemens, 2018) for ergonomics analysis. There are 17 inertial sensors secured to the body segments for each subject, which include head, shoulders, upper arms, forearms, hands, sternum, hip, upper legs, lower legs, and feet, followed by Xsens instructions (Xsens, 2022). Seamlessly integrating both software platforms ensured alignment between the skeletal segments of DHM_Xsens and DHM_JACK to achieve the replication of real human motion, as depicted in Figure 1.
2.2 Operational tasks
Four tasks were designed in this study. Each participant was tasked with employing two bows, featuring draw weights of 20 lbs (net weight: 9 kg) and 25 lbs (net weight: 11 kg), as illustrated in Figure 2A, while aiming at two targets positioned at distances of 1.0 and 1.4 m from the center of the targets to the floor, as illustrated in Figure 2B. Additionally, the subjects were situated 10 yards away from both targets. Each of the four tasks underwent repetition five times. Every participant was instructed to utilize a bow with a draw weight of 20 lbs initially and aim at both the bottom and top targets. Subsequently, participants were directed to switch to a bow with a draw weight of 25 lbs and repeat the aiming process at both the bottom and top targets.
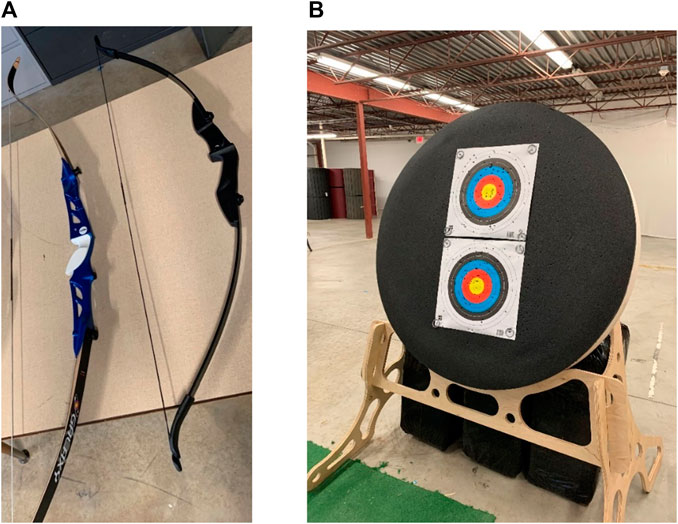
FIGURE 2. (A) Two bows with the draw weights of 20 lbs (right) and 25 lbs (left). (B) Two targets positioned at distances of 1.0 and 1.4 m from the center of the targets to the floor.
Task#1: pulled a bow with the draw weight of 20 lbs and aimed at the bottom target.
Task#2: pulled a bow with the draw weight of 20 lbs and aimed at the top target.
Task#3: pulled a bow with the draw weight of 25 lbs and aimed at the bottom target.
Task#4: pulled a bow with the draw weight of 25 lbs and aimed at the top target.
To achieve a successful shot, the subjects should follow a sequence of movements, which include 1) stance; 2) nock; 3) hook and grip; 4) posture/alignment; 5) raise bow; 6) draw; 7) anchor; 8) transfer to hold; 9) aim; 10) release/follow through; 11) feedback.
2.3 Data analysis
The forces applied to pull the bowstrings were measured using a digital force gauge (SF-500). During each participant’s performance, we employed a band tape to measure the distance between the bowstring and the center of the bow handle. Subsequently, the applied force was measured using a force gauge as the bowstring was pulled to a consistent length.
The posture illustrated in Figure 3 was identified as potentially posing an injury risk due to the significant force applied on the bow through both hands during the shooting sequence. This was monitored through real-time readings obtained from the JACK Siemens software.
To evaluate the injury risk associated with this posture, this study examined the compressive spinal force at the L4/L5 level, along with pertinent joint angles including shoulders, elbows, and trunk. The compressive forces applied to the fourth/fifth lumbar spine were calculated based on the posture adopted by participants and the load exerted by each hand, as shown in Figure 4.
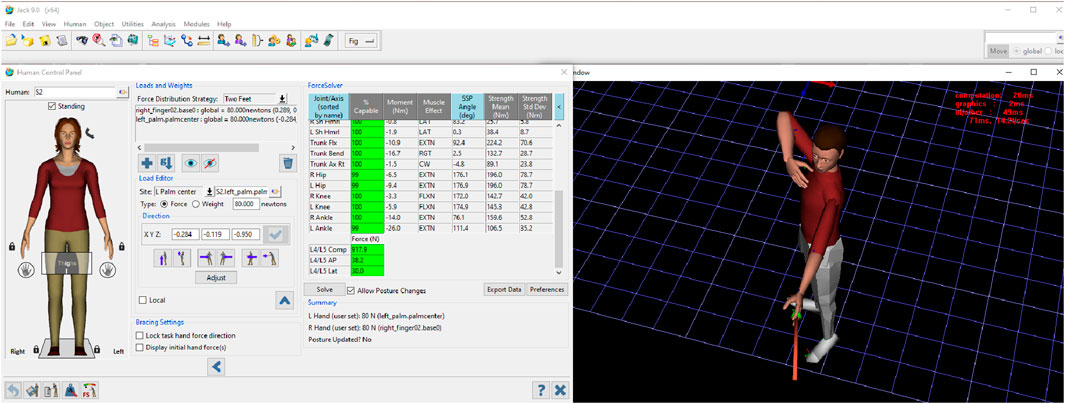
FIGURE 4. The spinal force was estimated based on the force exerted by each hand and the posture adopted by each participant.
2.4 Statistical analysis
A two-way analysis of variance (ANOVA) was performed to evaluate anatomical joints and forces exerted on the lower back, considering both tasks and genders as factors. The statistical significance threshold was set at 0.05. Furthermore, cross-correlation (r value) calculations were employed to explore potential relationships between any two of the main variables, offering insights into potential injury risks.
3 Results
Table 1 presents the anatomical joint angles and the estimated spinal force exerted on the lower back. The results were average across five trails. The abbreviations “20B,” “20T,” “25B,” and “25T” denote bows with draw weights of 20 lbs and 25 lbs aimed at bottom and top targets, respectively. “AVE_F” and “AVE_M” represent average results for females and males. “Comp” indicates the exposed compressive force on the lower back, while “R_Shoulder,” “L_Shoulder,” “R_Elbow,” and “L_Elbow” signify the right and left shoulders and elbows. Additionally, “F/E,” “Abd/Add,” and “Rot” denote the movements of flexion/extension, abduction/adduction, and rotation, respectively.
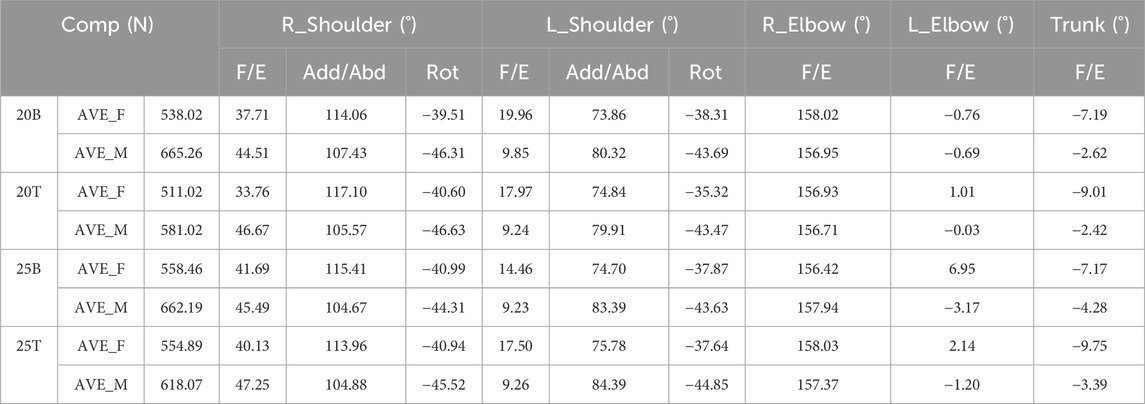
TABLE 1. The anatomical joint angles and the estimated exposed spinal forces at the pose where a high injury risk may exist.
The maximum and minimum values of compressive forces and the corresponding joint angles were graphically depicted in Figure 5 for both males and females in each testing scenario (20B, 20T, 25B and 25T). Additionally, the mean value and standard deviations were incorporated into the plots.
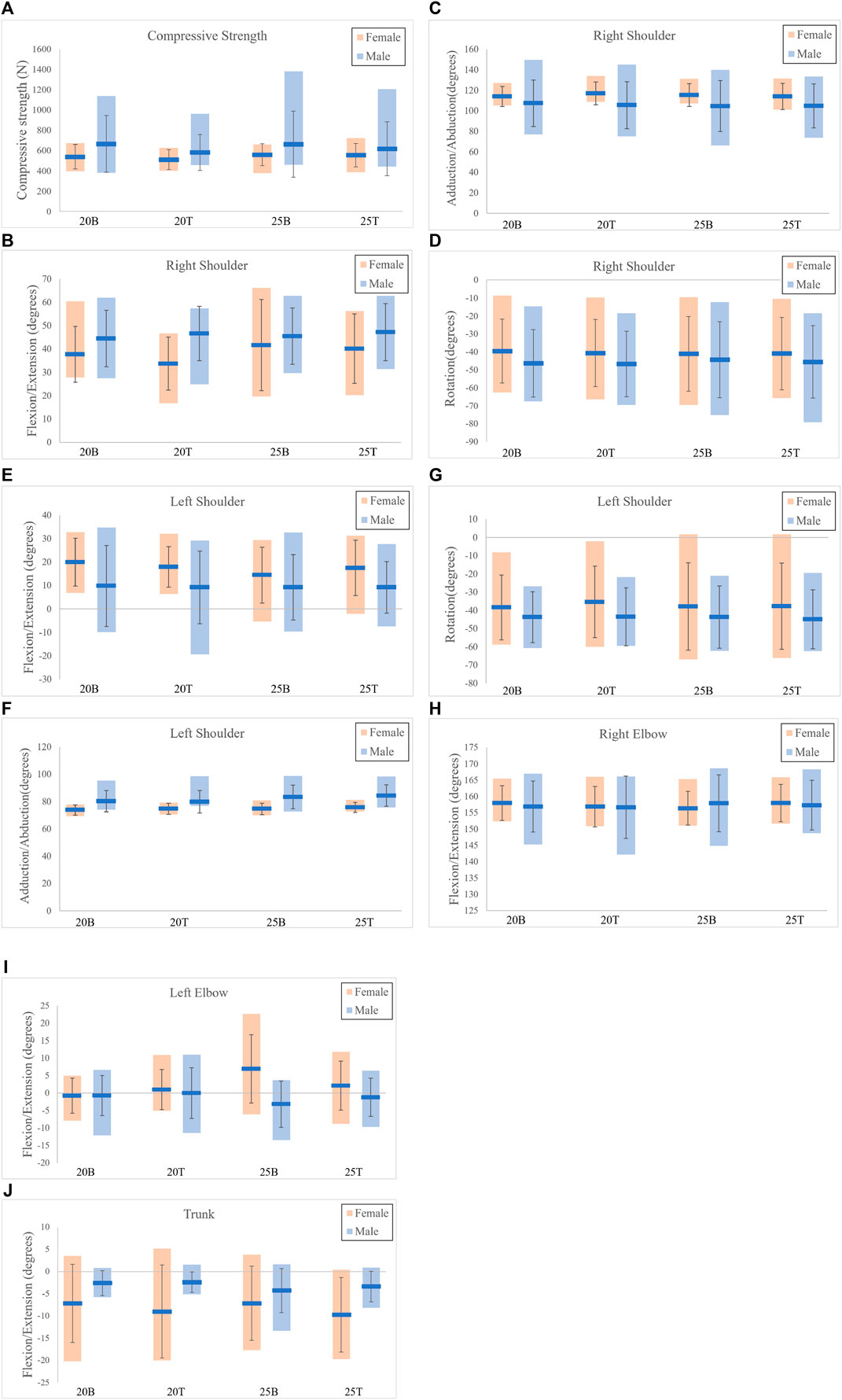
FIGURE 5. The results for each of test scenarios. (A) Compressive force; (B) R_Shoulder_F/E; (C) R_Shoulder_Abd/Add; (D) R_Shoulder_Rot; (E) L_Shoulder_F/E; (F) L_Shoulder_Abd/Add; (G) L_Shoulder_Rot; (H) R_Elbow_F/E; (I) L_Elbow_F/E; (J) Trunk_F/E.
In the results of the statistical analysis, while the p-value for flexion/extension in the right shoulder between genders was 0.06 when drawing the 20 lbs bow and aiming at the top target (20T), the observed difference was substantial, reaching 13° (AVE_F= 33.76°; AVE_M=46.67°). Furthermore, a significant gender difference was identified for abduction/adduction in the left shoulder during the drawing of the 25 lbs bow and aiming at both targets (25B: p=0.04; 20T: p=0.03). Specifically, in the 25B scenario, the AVE_F was 74.70°, and the AVE_M was 83.39°. Similarly, in the 25T scenario, the AVE_F was 75.78°, and the AVE_M was 84.39°. Besides, no significant gender differences were observed in the comparison of other variables.
In examining the correlation coefficients between variables, a moderate correlation emerged between trunk flexion/extension and compressive force, indicated by an r value of 0.40. Additionally, a notable high correlation was identified between body weight and compressive force, with an r value of 0.62.
4 Discussion
This study provided archers the chance to comprehend potential injury risks during their shooting performances. The adopted postures and the corresponding applied force on the lower back were examined using advanced motion tracking technique and ergonomics tools.
When comparing anatomical joint angles between genders, a significant difference was observed in the abduction/adduction of the left shoulder when drawing the 25 lbs bow at both top and bottom targets. Males exhibited approximately a 10° larger abduction angle than females while pulling the bowstring and aiming at the targets. However, no significant gender difference was noted when using the 20 lbs draw weight bow. A relatively small abduction of the left shoulder indicates that the left upper limb was distant from the horizontal axis, while a large abduction suggests it was close to or aligned with the horizontal axis. Notably, there was minimal variance in the abduction/adduction of the left shoulder among females when using both 20 lbs and 25 lbs bows. In contrast, males exhibited increased abduction of the left shoulder when transitioning from the 20 lbs to the 25 lbs bow. This could be attributed to differences in muscle strength between males and females (Patel and Parmar, 2022). As the draw weight increased, females may find it challenging to fully draw the bowstring in comparison to males.
In the comparison of the right shoulder, males exhibited a 10° greater flexion than females when using a 20 lbs draw weight bow. However, when transitioning to the 25 lbs draw weight, females had to apply increased force to pull the bowstring. Consequently, the exerted pulling force by females led to a corresponding increase in the adopted flexion angle, resulting in a similar flexion of the right shoulder between males and females when both used the 25 lbs bow. A substantial flexion of the right shoulder indicates that the right upper limb was positioned far from the subject’s frontal plane, whereas a relatively small flexion suggests it was close to or aligned with the frontal plane. Considering the variance in isometric muscle force between genders, males could maintain the same pose with bows of 20 lbs and 25 lbs, respectively. In contrast, females had to exert more force to pull the bowstring, directly influencing one of the most crucial archery postures, the Anchor (Legend, 2023a), used by archers to aim at targets.
Moreover, in comparing the force exerted on the lower back between females and males, there was an approximate difference of 80 N, with males exerting more force than females when using both 20 lbs and 25 lbs bows. This disparity could be attributed to gender differences in trunk flexion. Our findings align with previous studies (Ji et al., 2023a; Ji et al., 2023b; Ji et al., 2023c), indicating that a significant trunk flexion may lead to an increased exposed spinal force. Another contributing factor to the higher exposed spinal force in males compared to females might be the variation in body weight. We observed a moderate correlation (r=0.62) between body weight and compressive spinal force, suggesting that greater body weight supported by the lower trunk may elevate the load on the spinal discs. This aligns with previous research (Ghezelbash et al., 2016). Consequently, individuals with greater body weight, coupled with awkward postures and higher draw weight bows, may increase the risk of lower back injuries (WA, 2023).
5 Conclusion
This study employed the advanced Xsens motion tracking system and ergonomic tools to estimate the exposed spinal force on the lower back of archers. Anthropometric variables, such as body weight and trunk flexion/extension, were identified as potential contributors to significant differences in exerted spinal force. Additionally, variations in muscle biomechanics emerged as another factor leading to noticeable biases in adopted postures between males and females. To mitigate the risk of lower back (Prine et al., 2023) and shoulder (Gimigliano et al., 2021) injuries among archers and enhance their performance skills, maintaining upper body strength through training, controlling body weight, and ensuring a straight and natural position of the spine and shoulders are crucial measures.
Enhancing the study could involve recruiting a larger number of volunteers and expanding the variety of archery bows with different draw weights. This augmentation would enable a more comprehensive assessment of Rapid Upper Limb Assessment (RULA). Specifically, with regard to bows featuring larger draw weights, there is a potential for an elevated risk of upper limb issues among archers that warrants careful consideration.
Data availability statement
The original contributions presented in the study are included in the article/Supplementary Material, further inquiries can be directed to the corresponding author.
Ethics statement
The studies involving humans were approved by Gannon University Institutional Review Board (IRB) Committee. The studies were conducted in accordance with the local legislation and institutional requirements. The participants provided their written informed consent to participate in this study.
Author contributions
XJ: Conceptualization, Formal Analysis, Methodology, Software, Writing–original draft. IA: Software, Writing–original draft. XG: Formal Analysis, Validation, Writing–review and editing. ZA: Software, Writing–review and editing. ES: Data curation, Writing–review and editing. DP: Conceptualization, Methodology, Writing–review and editing.
Funding
The author(s) declare that no financial support was received for the research, authorship, and/or publication of this article.
Conflict of interest
The authors declare that the research was conducted in the absence of any commercial or financial relationships that could be construed as a potential conflict of interest.
Publisher’s note
All claims expressed in this article are solely those of the authors and do not necessarily represent those of their affiliated organizations, or those of the publisher, the editors and the reviewers. Any product that may be evaluated in this article, or claim that may be made by its manufacturer, is not guaranteed or endorsed by the publisher.
References
Aicale, R., Tarantino, D., and Maffulli, N. (2018). Overuse injuries in sport: a comprehensive overview. J. Orthop. Surg. Res. 13 (1), 309–311. doi:10.1186/s13018-018-1017-5
Barr, A. E., and Barbe, M. F. (2002). Pathophysiological tissue changes associated with repetitive movement: a review of the evidence. Phys. Ther. 82 (2), 173–187. doi:10.1093/ptj/82.2.173
Cao, W., Jiang, M., Han, Y., and Khasawneh, M. T. (2013). “Ergonomic assessment of patient barrow lifting technique using digital human modeling,” in Digital Human Modeling and Applications in Health, Safety, Ergonomics, and Risk Management. Human Body Modeling and Ergonomics: 4th International Conference, DHM 2013, Held as Part of HCI International 2013, Las Vegas, NV, USA, July 2013 (Springer), 20–29.
Chaffin, D. B. (2005). Improving digital human modelling for proactive ergonomics in design. Ergonomics 48 (5), 478–491. doi:10.1080/00140130400029191
Duffy, V. G. (2012). Human digital modeling in design. Handb. Hum. factors ergonomics, 1016–1030. doi:10.1002/9781118131350.ch35
Ghezelbash, F., Shirazi-Adl, A., Arjmand, N., El-Ouaaid, Z., Plamondon, A., and Meakin, J. (2016). Effects of sex, age, body height and body weight on spinal loads: sensitivity analyses in a subject-specific trunk musculoskeletal model. J. biomechanics 49 (14), 3492–3501. doi:10.1016/j.jbiomech.2016.09.026
Gimigliano, F., Resmini, G., Moretti, A., Aulicino, M., Gargiulo, F., Gimigliano, A., et al. (2021). Epidemiology of musculoskeletal injuries in adult athletes: a scoping review. Medicina 57 (10), 1118. doi:10.3390/medicina57101118
Guo, C., Liu, X., Yang, Y., Zhang, D., Yang, D., and Yin, J. (2022). Analysis of rotator cuff muscle injury on the drawing side of the recurve bow: a finite element method. Comput. Math. Methods Med. 2022, 1–10. doi:10.1155/2022/8572311
Ji, X., Hernandez, J., Schweitzer, E., Wang, W., and Piovesan, D. (2023). The assessment of injury risk in the healthcare sector via integrating motion tracking techniques with digital human modelling ergonomic tools. Int. J. Hum. Factors Model. Simul. 8 (1), 1–20. doi:10.1504/ijhfms.2023.130120
Ji, X., Hettiarachchige, R. O., Littman, A. L., Lavery, N. L., and Piovesan, D. (2023). Prevent workers from injuries in the brewing company via using digital human modelling technology. Appl. Sci. 13 (6), 3593. doi:10.3390/app13063593
Ji, X., Hettiarachchige, R. O., Littman, A. L., and Piovesan, D. (2023). Using digital human modelling to evaluate the risk of musculoskeletal injury for workers in the healthcare industry. Sensors 23 (5), 2781. doi:10.3390/s23052781
Legend (2023). All you need to know about archery anchor points. Available at: https://legendarchery.com/blogs/archery-bowhunting-blog/all-you-need-to-know-about-archery-anchor-points#:∼:text=Start%20by%20placing%20the%20tip,observe%20where%20the%20arrow%20lands (Accessed October 25, 2023).
Legend (2023). The most common archery injuries and how you can avoid them. Available at: https://legendarchery.com/blogs/archery-bowhunting-blog/the-most-common-archery-injuries-and-how-you-can-avoid-them (Accessed January 5, 2023).
Liao, C.-N., Fan, C.-H., Hsu, W.-H., Chang, C. F., Yu, P. A., Kuo, L.-T., et al. (2022). Twelve-week lower trapezius-centred muscular training regimen in university archers. Healthcare, 10, 171. doi:10.3390/healthcare10010171
Management, R. (2015). “Archery Participation Among Adult United States Residents in 2014,”(Harrisonburg, VA, USA: Responsive Management).
Maurice, P., Padois, V., Measson, Y., and Bidaud, P. (2019). Assessing and improving human movements using sensitivity analysis and digital human simulation. Int. J. Comput. Integr. Manuf. 32 (6), 546–558. doi:10.1080/0951192x.2019.1599432
Niestroj, C. K., Schöffl, V., and Küpper, T. (2018). Acute and overuse injuries in elite archers. J. Sports Med. Phys. Fit. 58 (7-8), 1063–1070. doi:10.23736/s0022-4707.17.07828-8
Patel, P., and Parmar, L. (2022). Comparison between genders for trunk mobility in normal adults: a cross sectional study. Int. J. Health Sci., 6, (4), 1564–1573. doi:10.53730/ijhs.v6ns4.6284
Paul, G., and Quintero Duran, M. (2015). “Ergonomic assessment of hospital bed moving using DHM Siemens JACK,” in Proceedings of the 19thTriennial Congress of the International Ergonomics Association (IEA 2015), Australia, August 2015, 1–6.
Paul, G., and Quintero Duran, M. (2018). Ergonomic assessment of a physical task using two different digital human modelling systems: a case study. Int. J. Hum. Factors Model. Simul. 6 (4), 298–313. doi:10.1504/ijhfms.2018.10017421
Prine, B. R., Pazik, M. N., Prine, A., Haley, H., Bruner, M. L., and Vincent, H. K. (2023). Characteristics and reported injuries of recreational and competitive archers. J. Sports Med. Phys. Fit. 63, 1202–1207. doi:10.23736/s0022-4707.23.15257-1
Rhén, I.-M., Forsman, M., Örtengren, R., Högberg, D., Keyvani, A., Lämkull, D., et al. (2018). Ergonomic risk assessment in DHM tools employing motion data-exposure calculation and comparison to epidemiological reference data. Int. J. Hum. Factors Model. Simul. 6 (1), 31–64. doi:10.1504/ijhfms.2018.091356
Schall Jr, M. C., Fethke, N. B., and Roemig, V. (2018). Digital human modeling in the occupational safety and health process: an application in manufacturing. IISE Trans. Occup. ergonomics Hum. factors 6 (2), 64–75. doi:10.1080/24725838.2018.1491430
Shinohara, H., Urabe, Y., Maeda, N., Xie, D., Sasadai, J., and Fujii, E. (2014). Does shoulder impingement syndrome affect the shoulder kinematics and associated muscle activity in archers. J. Sports Med. Phys. Fit. 54 (6), 772–779.
Siemens (2018). Siemens JACK software. Available at: https://www.plm.automation.siemens.com/media/store/en_us/4917_tcm1023-4952_tcm29-1992.pdf (Accessed May 5, 2022).
Vendrame, E., Belluscio, V., Truppa, L., Rum, L., Lazich, A., Bergamini, E., et al. (2022). Performance assessment in archery: a systematic review. Sports Biomech., 1–23. doi:10.1080/14763141.2022.2049357
WA (2023). Equipment compound. World archery. Available at: https://www.worldarchery.sport/sport/equipment/compound#:∼:text=Drawing%20a%20competitive%20compound%20bow,speeds%20of%20over%20350%20kph (Accessed October 25, 2023).
Wang, Q., Lou, Y., Li, T., and Jin, X. (2021). Development and application of digital human models in the field of vehicle collisions: a review. Ann. Biomed. Eng. 49 (7), 1619–1632. doi:10.1007/s10439-021-02794-z
Xsens (2022). Xsens 3D motion tracking technology. Available at: https://www.movella.com/products/motion-capture/xsens-mvn-awinda (Accessed May 1, 2022).
Keywords: archery, injuries, Xsens Awinda MVN, digital human modeling, JACK Siemens
Citation: Ji X, Arzalluz I, Gao X, Al Tamimi Z, Swierski E and Piovesan D (2024) Exploring injury assessment using motion tracking technology and ergonomic tools in archery performance. Front. Med. Eng. 2:1375520. doi: 10.3389/fmede.2024.1375520
Received: 24 January 2024; Accepted: 20 February 2024;
Published: 01 March 2024.
Edited by:
Victor Prybutok, University of North Texas, United StatesReviewed by:
Hongwu Wang, University of Florida, United StatesAbhijeet Kumar, Missouri Southern State University, United States
Copyright © 2024 Ji, Arzalluz, Gao, Al Tamimi, Swierski and Piovesan. This is an open-access article distributed under the terms of the Creative Commons Attribution License (CC BY). The use, distribution or reproduction in other forums is permitted, provided the original author(s) and the copyright owner(s) are credited and that the original publication in this journal is cited, in accordance with accepted academic practice. No use, distribution or reproduction is permitted which does not comply with these terms.
*Correspondence: Xiaoxu Ji, amkwMDFAZ2Fubm9uLmVkdQ==