- Laboratory of Biomechanical Orthopedics, Institute of Bioengineering, EPFL, Lausanne, Switzerland
During daily activities, cartilage encounters complex biophysical cues upon loading. Foremost among these is the coupled stimulation of hydrostatic pressure (HP) and loading-induced temperature increase (T), which encompasses both mechanical and thermal aspects of biophysical stimulations in cartilage. While prior research on this subject has been initiated in our laboratory, the detailed mechanisms of combined HP-T effects on chondrocytes in their natural environment remain largely unexplored. Using a custom bioreactor, we applied both isolated and combined HP-T stimuli to cartilage explants obtained from a non-inflammatory adolescent knee joint. Tissue and cellular responses were evaluated through histochemical staining and transcriptomic analyses, employing bulk RNA-sequencing complemented with signaling enrichment analyses. Our findings reveal that the thermal component of the coupled HP-T stimulation predominantly regulates the chondrocytes’ transcriptional profile during the stimulation period. When coupled with HP stimulation, a peak in chondroinduction was observed. This coupling process notably boosted chondroprotection in a synergistic manner, as demonstrated by the corresponding enhanced negative regulation of apoptotic processes and increased levels of Heat Shock Protein 70 (HSPA). Our study suggests that the upregulation in protein translation and processing, triggered by thermal stimulation, may serve as an adaptive mechanism in chondrocytes to mechanical simulations, thereby contributing to the observed synergy during the coupling of these two biophysical stimuli. The results highlight the potential of integrating thermal stimulation, a natural accompanying process during cartilage deformation, in tissue engineering, cell therapy or physiotherapy.
1 Introduction
Unraveling the intricacies of cellular responses within the knee joint to external physiological biophysical stimuli poses inherent challenges. These challenges come from the simultaneous manifestation of two key biological phenomena, mechanobiology and thermobiology, arising during repetitive physical activities. Previous research has revealed an association between repetitive activities such as jogging and a consequential elevation in the local cartilage temperature of the knee (Becher et al., 2008). This coupling is of considerable interest, particularly as chondrocytes, the primary cellular constituents of knee cartilage, demonstrate a distinct sensitivity to both temperature fluctuations and mechanical stimuli (Ito et al., 2014; Ito et al., 2015a; Ito et al., 2015b). The benefits of mechanical stimuli, composed of a combination of compressive strain, shear strain, and hydrostatic pressure (HP), extend beyond simply orchestrating the differentiation of stem cells into mature chondrocytes (Fahy et al., 2017). These mechanical stimuli also enhance the synthesis of chondrogenic markers and extra cellular matrix (ECM), pivotal elements in the constitution of mature chondrocytes and functional cartilage (Wong and Carter, 2003; Elder and Athanasiou, 2009; Anderson and Johnstone, 2017; Li et al., 2017; Salinas et al., 2018). In terms of thermal cues, under normal resting conditions, the intra-articular temperature of the knee maintains a steady equilibrium at around 32.5°C (Becher et al., 2008). However, when exposed to a temperature increase within the physiological range up to 38.7°C [mirroring the temperature profile within a knee joint during 1.5 h of jogging (Becher et al., 2008)], in vitro cultured chondrocytes displayed enhanced chondrogenic ability and an elevated capacity to produce ECM (Nasrollahzadeh et al., 2022; Stampoultzis et al., 2023). Intriguingly, these same studies showed that this temperature alteration also intensifies the chondrocytes’ response to compressive loading. The individual benefits and the intricate interplay between temperature and mechanical stimuli underscores the necessity of considering both these factors in investigations seeking to unravel the complexities of chondrocyte function and cartilage homeostasis.
Hydrostatic pressure and the loading-induced thermal increase are both direct consequences of compressive cyclic loading. Intriguingly, while these elements are naturally coupled during normal knee activity in healthy cartilage, this coupling may disappear under degenerative conditions (Setton et al., 1999; Martin and Buckwalter, 2001; Abdel-Sayed et al., 2014), indicating the potential importance of HP-thermal coupling in regulating cartilage homeostasis. Previous in vitro investigations using 3D chondrocyte-hydrogel constructs revealed that chondrocytes can react to HP-T stimulation, thereby maximizing their chondroinductive and chondroprotective capacities (Guo et al., 2023). However, synthetic in vitro 3D chondrocyte models might limit a comprehensive understanding of the underlying synergy. Despite their high biocompatibility, biomaterials may not completely replicate the native ECM environment or the zonal structure of actual cartilage (Sophia Fox et al., 2009; Antons et al., 2018), complicating the elucidation of the mechanisms underpinning the effects of external stimuli (Ahearne, 2014; Chaudhuri et al., 2016; Mohammed et al., 2019). Approaches employing osteochondral units or cartilage explants can circumvent the use of biomaterials, providing a more realistic environment for studying chondrocyte reactions to external biophysical stimulation (Kotelsky et al., 2020; McCreery et al., 2020; Szczesny, 2020).
In this study, we aim to investigate the synergistic behavior of hydrostatic pressure (referred to as “HP” in this article) and physiological load-induced gradual temperature increase (referred to as “T” or “thermal stimulation” in this article) within a human cartilage tissue environment. We seek to elucidate the mechanisms by which chondrocytes respond to this coupled HP-T stimulation. Moreover, we aim to understand the distinct contributions of each form of isolated stimulation, HP and T, within the coupled interplay that dictates cartilage homeostasis. To this end, we procured human cartilage explants, incubating them in our customized bioreactor to apply either isolated or coupled HP-T stimuli. To investigate the chondrocyte cellular response to either uncoupled or coupled HP-T stimuli, we conducted a comprehensive range of histological staining and transcriptomic analyses using bulk RNA-sequencing complemented with signaling enrichment analyses. Our findings reveal that in cartilage explants subjected to repetitive loading, the thermal component within the coupled HP-T stimulation primarily influences the short-term transcriptional profile of chondrocytes. When coupled with HP stimulation, a peak in chondroinduction is observed. Furthermore, the coupling process notably enhances chondroprotection synergistically, as evidenced by an increase in Heat Shock Protein 70 (HSPA) levels and augmented negative regulation of apoptosis. The upregulation of protein translation and protein processing, triggered by thermal stimulation and demonstrated by the activation of various related biological processes, may provide key insights into the observed synergy during the coupling of these two biophysical stimuli.
2 Material and methods
2.1 Human explant preparation and culture
Cartilage explants were obtained from the femoral trochlea of the left knee of a 14-year-old male patient following a traumatic Osteochondritis Dissecans (OCD) event at CHUV (Centre hospitalier universitaire vaudois, Switzerland). In particular, clinical cartilage samples were anonymized, stored, and logged in a Musculoskeletal Department Biobank, complying with internal regulations thereof and following an approved protocol (i.e., Vaud cantonal State Ethics Committee reference N°264/12). The extracted tissue was immediately preserved in 0.9% NaCl and subsequently sectioned into uniform cylindrical pieces using a 5 mm diameter biopsy punch, with an approximate thickness of 4 mm per explant. Each explant was then washed three times in DMEM (ThermoFisher, US) medium supplemented with 1% penicillin-streptomycin (P/S) (ThermoFisher, US) and 1% L-Glutamine (L-Glu) (Gibco, US). To ensure reliability of the results, the samples were randomized based on their original position in the tissue (Supplementary Figure S1). They were then cultured in the same medium at 32.5°C for 24 h.
2.2 Biophysical stimulation
Explant samples were placed in customized bioreactor wells filled with culture medium consisting of DMEM, 1% P/S, 1% L-Glu, 10% insulin transferrin selenium (ITS-IV) (PAN-biotech, Germany), and 1% ascorbic acid (VC) (Sigma, US). Using a previously developed customized bioreactor, various biophysical stimulation protocols were administered for 1.5 h daily over five consecutive days (Guo et al., 2023). Four experimental groups were considered and the specific stimulation details for each group are (Figure 1): 1) Control group (“C”): Maintained at a steady 32.5°C within the bioreactor, replicating the resting knee temperature, with no applied hydrostatic pressure (HP) or temperature increase. 2) Isolated pressure group (“P”): While kept consistently at 32.5°C, samples underwent sinusoidal cyclic HP loading with a 5 MPa amplitude at 1 Hz. 3) Isolated temperature increase group (“T”): Samples were exposed to a gradual temperature rise from 32.5°C to 38.7°C without any HP loading. This temperature profile mirrors the intra-articular temperature evolution observed during a 1.5-h jogging (Becher et al., 2008; Nasrollahzadeh et al., 2022). 4) Coupled HP-T stimulation group (“PT”): Samples were concurrently subjected to a 5 MPa cyclic HP loading (as in the “P” group) and a temperature increase from 32.5°C to 38.7°C (as in the “T” group) for the entire 1.5-h stimulation duration. In each group, the stimulation medium was replaced every other day. For each biophysical stimulation condition, we analyzed three explants, chosen randomly based on their position within the knee. We performed histological examinations on all three explants from each group and conducted RNA-sequencing on two explants from each condition. Samples of all conditions were harvested immediately after the last stimulation and processed accordingly to the analysis described below.
2.3 Histology and bio-imaging
Explant were fixed in formalin solution (Sigma-Aldrich, US) for 48 h, then dehydrated and embedded in paraffin. These samples were subsequently sectioned at 4 µm using a microtome. Standard histochemical staining methods were employed using Hematoxylin and Eosin (HE), Alcian Blue (AB), Sirius Red (SR), and Safranin’O with Fast Green (SO) at the EPFL Histology Core Facility. All slides were consistently imaged using an Olympus VS120 whole slide scanner, with uniform exposure settings. For polarized light imaging, the LEICA DM 5500 was utilized.
2.4 RNA extraction
Explant samples were snap-frozen in liquid nitrogen and subsequently ground into powder using a mortar and pestle, ensuring the continued presence of liquid nitrogen. The tissue powder was then combined with 300 µL of TRIzol (ThermoFisher, US), followed by the addition of 100 µL of chloroform (Sigma-Aldrich, US). After centrifugation, the RNA-rich aqueous phase was separated. RNA isolation and purification were carried out using the Nucleospin® RNA XS kit (Macherey-Nagel, Germany) according to the manufacturer’s guidelines. RNA concentrations were determined using the Nanodrop Lite Spectrophotometer (Thermo Scientific, US).
2.5 Bulk RNA sequencing and statistics analyses
RNA quality was controlled on the TapeStation 4200 (Agilent, US). RNAs were ranging from being partially degraded to heavily degraded, with RIN (RNA Integrity Number) values varying from 1.8 to 6.9. This value, along with the mRNA profiling results from the TapeStation 4200, served to determine the protocol chosen for subsequent library preparation. First, non-ribosomal RNAs were enriched using the NEBNext rRNA Depletion Kit (New England Biolabs, US), starting from 15 ng RNA. Then, libraries were prepared with NEBNext Ultra II Directional RNA Library Prep Kit (New England Biolabs, US), according to manufacturer’s instructions. Libraries, all bearing unique dual indexes, were subsequently loaded on a NovaSeq 6000 flow cell (Illumina, US) and sequenced according to manufacturer instructions, yielding pairs of 60 nucleotides-long reads. Reads were trimmed of their adapters with bclconvert v3.9.3 (Illumina, US) and quality-controlled with fastQC v0.11.9.
For data analyses, Truseq adapter sequences were trimmed from FASTQ files using bcl2fastq (Illumina, US). Sequences were aligned to the human genome (GRCh38) using STAR (Dobin et al., 2013). Duplicates were marked with Picard (Picard Tools, 2023) and then FeatureCounts (Liao et al., 2014) was used to count reads in features annotated in Gencode (v42) (Frankish et al., 2019) with the following parameters: paired end mode (-p), --ignoreDup, reverse-strand (-s 2), minimum quality (-Q 10).
Genes were considered expressed if they had greater than 10 reads in at least 2 of the samples. Differential expression was performed using DESeq2 (v1.36.0) default parameters (Love et al., 2014) and genes were considered differentially expressed if they were significantly (adjusted p-value
Gene regulation pattern analysis was conducted using DEGreport (v1.37.0) (Lorena Pantano Aut, 2017). Ontology analysis was carried out with gprofiler2 (v0.2.1) (Kolberg et al., 2020), and biological process maps were created through the KEGG online interface. Heatmaps and other data visualizations were generated using custom R codes. Significance was represented by adjusted p-values from DESeq2 or p-values from gprofiler2: * (p < 0.05), ** (p < 0.01), and *** (p < 0.001).
3 Results
3.1 Promotion of isogenous group formation in cartilage through thermal stimulation
After 5 days of varied stimulations within the bioreactor (Figure 2A, left), histological staining showed tissues in a normal condition with preserved structural integrity (Figure 2B). Tile-scanned images revealed chondrocytes encased within lacunae, with occasional groupings into isogenous clusters (Figure 2A, right). The color absorbance across various groups did not indicate significant variations in the quantity of various ECM components, such as glycosaminoglycan and collagen (Supplementary Figure S2).
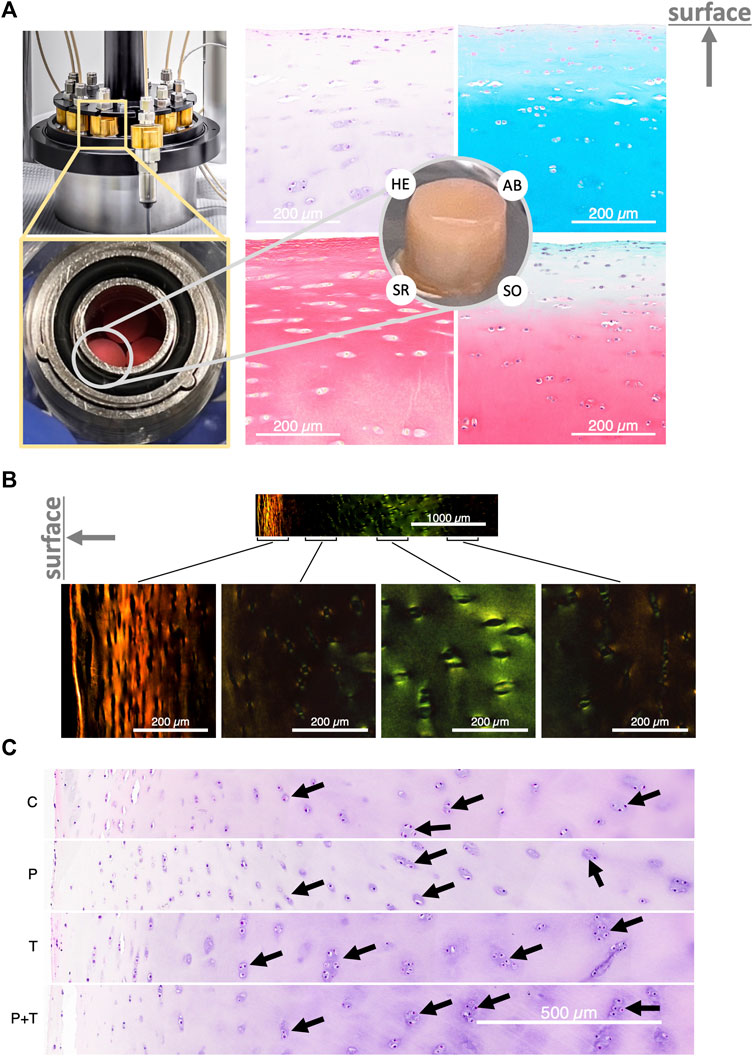
FIGURE 2. (A) Left: The bioreactor housing the cylindrical explant samples. Right: Cross-section of the explant stained with Hematoxylin and Eosin (HE), Alcian Blue (AB), Sirius Red (SR), and Safranin’O with Fast Green (SO), showing normal cartilage structure. The direction of the cartilage surface is indicated by an upward-pointing arrow. (B) Polarized light image of Sirius Red-stained cartilage, displaying a typical zonal organization. (C) HE-stained explants following control and isolated or couple HP-thermal biophysical stimulation; black arrows highlight the isogenous group. “C” stands for unstimulated control incubated at 32.5°C. “P” stands for isolated cyclic HP stimulation at 5 MPa and 1 Hz; “T” stands for gradual temperature increase from 32.5°C to 38.7°C mimicking the temperature evolution profile seen in the human intra-articular space during jogging, and “PT” stands for the simultaneous application of the previous two types of stimulations. The direction of the cartilage surface in (B,C) is indicated by a left-pointing arrow.
Intriguingly, a consistent and noticeable enlargement of isogenous groups was observed in samples subjected to thermal stimulation compared to other conditions. This pattern was particularly prominent when analyzing cell distributions at comparable depths from the cartilage surface (Figure 2C). The isolated thermal stimulation group disclosed a greater count of chondrocytes within isogenous groups, suggesting a potential surge in mitotic division. In contrast, the isolated hydrostatic pressure (HP) group appeared to display a slight decrease in isogenous group sizes relative to the control. This observation remained constant across all triplicate samples examined under each condition. For the group subjected to combined pressure and thermal stimuli, the cell distribution seemed to reside between the patterns observed for the isolated HP and thermal stimulation groups. These findings suggest a potential alteration in metabolic activity in samples subjected to thermal stimulation, either standalone or in combination with hydrostatic pressure.
3.2 Dominance of temperature in coupled HP-T regulation on chondrocyte transcriptomics
To evaluate the individual and collective contributions of hydrostatic pressure and temperature increase to cartilage homeostasis, we performed bulk RNA-sequencing to scrutinize the transcriptomic profiles of chondrocytes within the explants subjected to various stimulation conditions. Our primary focus was on the genes altered by the naturally occurring, coupled HP-thermal stimulation. Additionally, we aimed to delineate the individual contributions of HP and thermal stimulation to these transcriptomic changes within the context of their coupling.
Hierarchical clustering, using the top 2,000 genes with the highest adjusted p-values from coupled stimulation, showed that those exposed to thermal stimulation (i.e., “T” and “PT”) grouped together, suggesting a closer transcriptomic similarity among samples subjected to isolated thermal and coupled stimulation (Figure 3A). To comprehensively evaluate the contribution of individual stimulations, we contrasted differentially expressed (DE) genes across various stimulation conditions using Venn diagrams (Figure 3B). Interestingly, out of the 1003 upregulated and 1109 downregulated DE genes in the coupled stimulation, 87% and 80%, respectively, corresponded to genes differentially expressed under isolated thermal stimulation. In contrast, HP stimulation accounted for a small portion of the DE genes seen in the coupled stimulation, highlighting the dominant influence of transient thermal stimulation on the chondrocytes’ transcriptomic profile during the combined HP-thermal process. Notably, there was no gene that was upregulated by pressure and downregulated by temperature (or vice versa), indicating that, in the short term, HP and thermal stimulations do not counteract each other under the naturally coupled HP-thermal conditions within a physiological range.
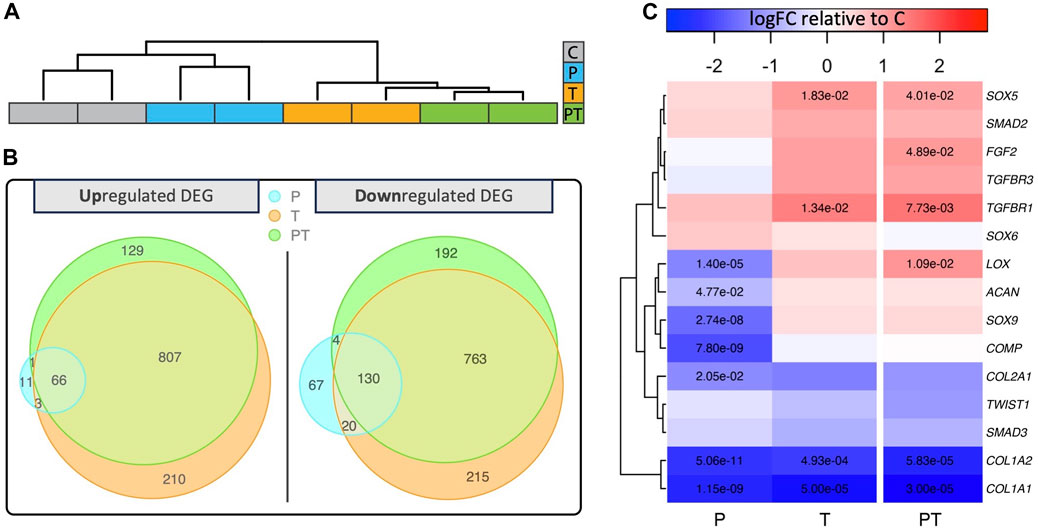
FIGURE 3. (A) Hierarchical clustering and dendrograms reveal similarities across samples under different biophysical stimulations. (B) Venn diagrams revealing different numbers of up or downregulated differentially expressed genes (DEG) in various biophysical stimulation groups. (C) Heatmap displaying log10 relative fold change of chondrocyte-associated genes compared to the unstimulated control with significant fold changes denoted by adjusted p-values in the respective boxes.
Next, we evaluated 15 important genes associated with structural proteins, signaling proteins, and growth factor receptors in chondrocytes across each stimulation condition (Figure 3C). Notably, most of these genes, including SOX9, ACAN, COL2, and COMP, remained statistically unaffected by the coupled stimulation. However, the level of COL1A demonstrated a significant decrease across all stimulation groups.
Recognizing the significance of thermal stimulation in shaping the transcriptomic profiles of chondrocytes in cartilage, we sought to further delve into its role by investigating how genes are influenced by naturally occurring coupled HP-thermal stimulation, and how isolated forms of stimulation contribute to this influence. Initially, we examined the regulatory patterns of all differentially expressed (DE) genes modified by naturally occurring HP-T stimulation (Figure 4A). The dot plot for each group displays the z-score for a specific set of genes, which exhibit consistent regulation patterns across various stimulation conditions. Each box represents the gene expressions of that set of genes under its respective stimulation condition. Regulatory pattern group 1 and 2 had the highest number of genes, occupying 43.7% and 41.3% of the total number of analyzed genes respectively. Genes in these two groups showed an incremental increase or decrease across control, uncoupled HP, T stimulation, and coupled HP-T conditions. This pattern suggests that the majority of genes influenced by the coupled HP-T stimulation are also similarly impacted albeit in a less pronounced or comparable manner by individual HP or T stimulations. To gain a more comprehensive understanding of the transcriptional influence of the genes present within these clusters, we then examined the activation of signaling cascades pertinent to cartilage homeostasis using all the DE genes from the coupled stimulation (Figure 4B). We observed that biological processes associated with the “extracellular space,” “extracellular matrix structural constituent conferring compression resistance,” and “cartilage development” (quoted terms denote standard biological processes of interests) were significantly enriched by coupled stimulation. The first two biological processes mentioned above were also activated by isolated thermal stimulation, albeit less significantly. Concurrently, with the inclusion of thermal stimulation, biological processes related to negative regulation of apoptosis were significantly enriched, implying an enhanced chondroprotective ability at the transcriptomic level (Jiang and Tuan, 2015). Conversely, none of the mentioned biological processes were significantly increased by isolated HP stimulation under the duration of our experiments. Collectively, these findings highlight the considerable contribution of thermal stimulation to the effects of coupled HP-T stimulation, maximizing both chondroinduction and chondroprotection in human cartilage tissue at a transcriptional level.
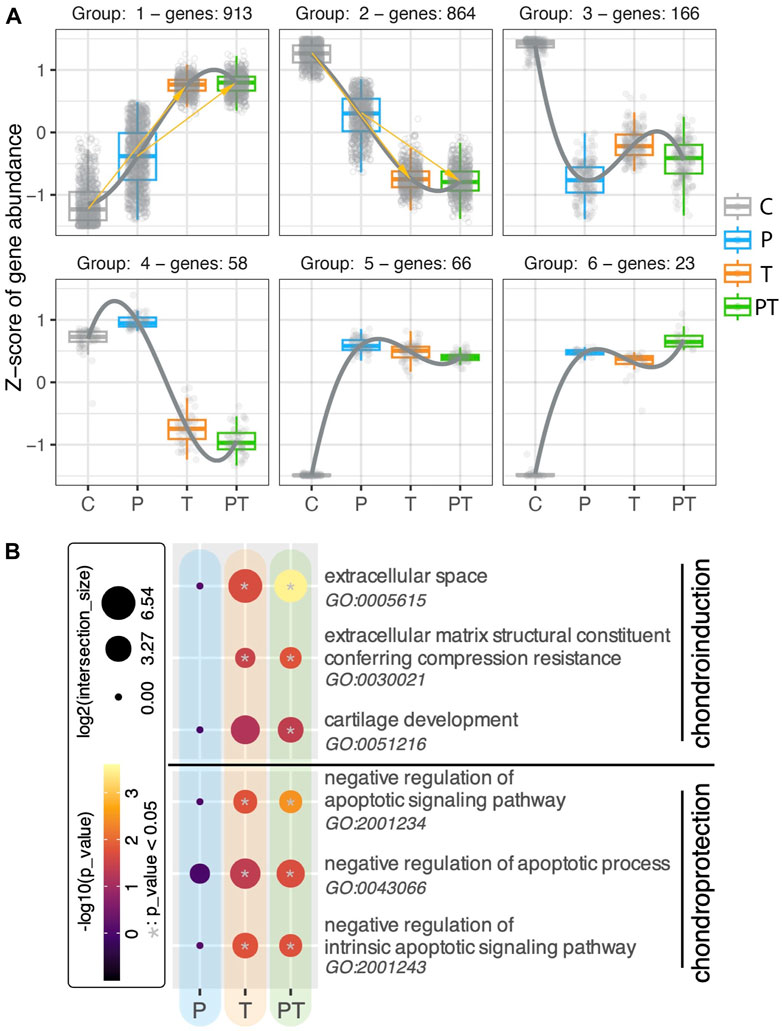
FIGURE 4. (A) Regulation patterns in six clusters, using all DE genes identified from the coupled HP-T stimulation compared to the control. Each gene is represented with a small circle. The gene level (represented with z-score) of all the genes within one cluster (meaning these genes shared the same regulation pattern across different stimulation conditions) are plotted in forms of box plots for each stimulation. (B) Gene Ontology (GO) analyses of isolated and combined HP-thermal stimulation, highlighting chondroinduction (top) and chondroprotection (bottom). The color inside each circle corresponds to the -log10 of adjusted p-values, while circle size denotes the log2 of intersection with the GO term. Significant hits with p-values smaller than 0.05 are denoted with a gray star in the center of the circle.
3.3 Synergistic transcriptomic activation of HSPA and protein translation and processing biological processes by HP-T coupling
Heat shock protein 70 (HSPA) is a family of chaperone proteins known for their critical role in chondroprotection (Grossin et al., 2006; Tonomura et al., 2006; Etienne et al., 2008). Motivated by our preliminary findings on the synergistic responses of HSPA in a 3D chondrocyte construct under HP-T stimuli (Guo et al., 2023), we undertook a deeper investigation into the transcriptional behavior of the HSPA family within our explants. Notably, many members of the HSPA family were significantly upregulated in response to thermal stimulation (Figure 5A). Some genes, such as HSPA1A/B, demonstrated further enhancement upon coupling of HP with thermal stimulation, although the difference between isolated thermal stimulation and coupled HP-thermal stimulation was not statistically significant.
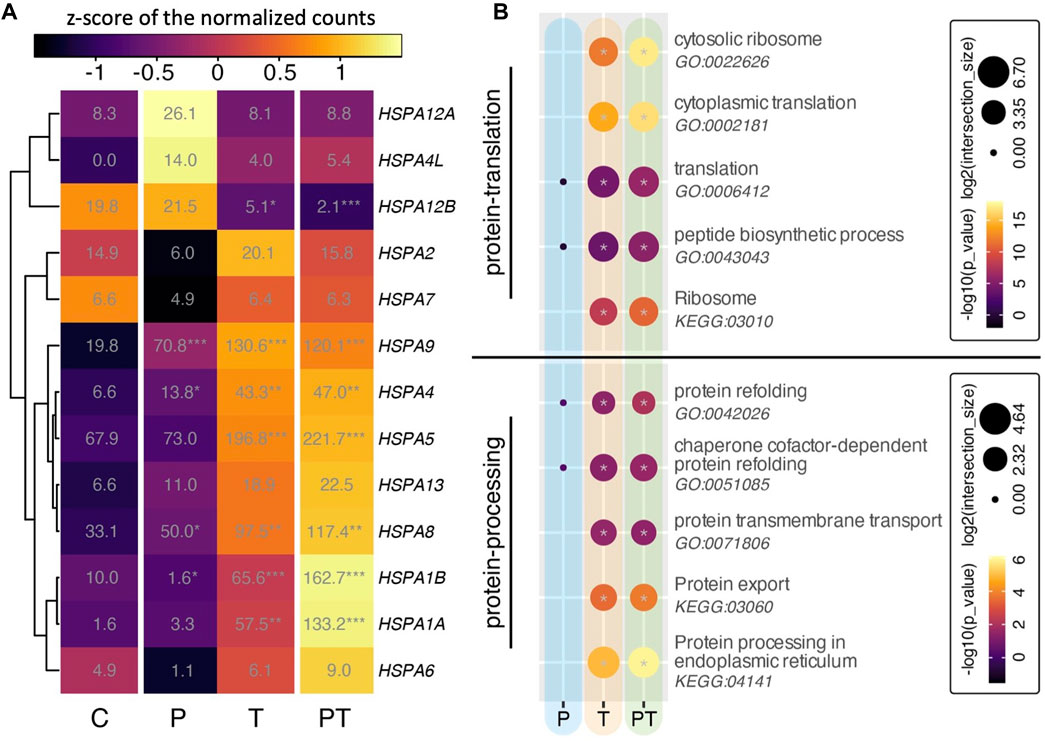
FIGURE 5. (A) Heatmap of all HSPA family isoforms. Colors denote z-scores of normalized gene counts, values represent normalized gene counts, and stars indicate statistical significance (adjusted p values) compared to control with p < 0.05 (*), p < 0.01 (**), p < 0.001 (***). (B) Gene ontology (GO) analyses for different biophysical stimulation groups, focusing on protein translation (top) and protein processing (bottom). Circle color and size correspond to -log10 of p-values and log2 of GO term intersection, respectively. Significant hits with p-values smaller than 0.05 are denoted with a gray star in the center of the circle.
Considering the critical role of HSPA in ensuring proper protein folding, stabilization, and protection against cellular stressors after translation, we conducted ontology analyses associated with protein translation and protein processing in our experimental groups (Figure 5B). Protein translation occurs when ribosomes synthesize proteins using mRNA. These proteins move to the endoplasmic reticulum (ER) for folding and processing. Some of these proteins, intended for secretion, then travel via vesicles to the Golgi for further modification before being released from the cell. In chondrocytes, this process is pivotal for maintaining cartilage health and stability. Under thermal stimulation conditions (i.e., both isolated thermal and coupled HP-thermal stimulation), the GO terms related to protein translation, encompassing activities related to ribosomes, translation, and peptide biosynthesis, were notably enhanced (Figure 5B, top). Concurrently, protein processing, which includes protein folding, transport, export, and protein processing in the endoplasmic reticulum (ER), also saw a significant rise (Figure 5B, bottom). It was also observed that, compared to the isolated T stimulation, the combined HP-T resulted in a more pronounced activation of these biological processes.
Alongside HSPA, other genes implicated in these aforementioned processes, such as protein processing in ER, also showed upregulation in the presence of thermal stimulation (Figure 6A). These included SEC23 and SAR1, key components of COPII involved in vesicle formation and the transport of synthesized proteins and lipids from the ER to the Golgi apparatus, as well as numerous other translocation proteins such as SEC11/62/63 and signaling recognition proteins (SRP), all critical for peptide synthesis and processing in the ER. Of particular interest were the behaviors of ribosomal proteins. We observed that the transcriptional levels of ribosomal proteins from both large and small subunits were significantly enhanced under thermal stimulation (Figure 6B). Consistent with our previous observations on the synergistic effects of coupled HP and thermal stimulation, HP stimulation alone did not yield any significant changes in the previously mentioned biological processes. However, when coupled with thermal stimulation, it conferred a more pronounced activation, than that coming from the thermal stimulation alone.
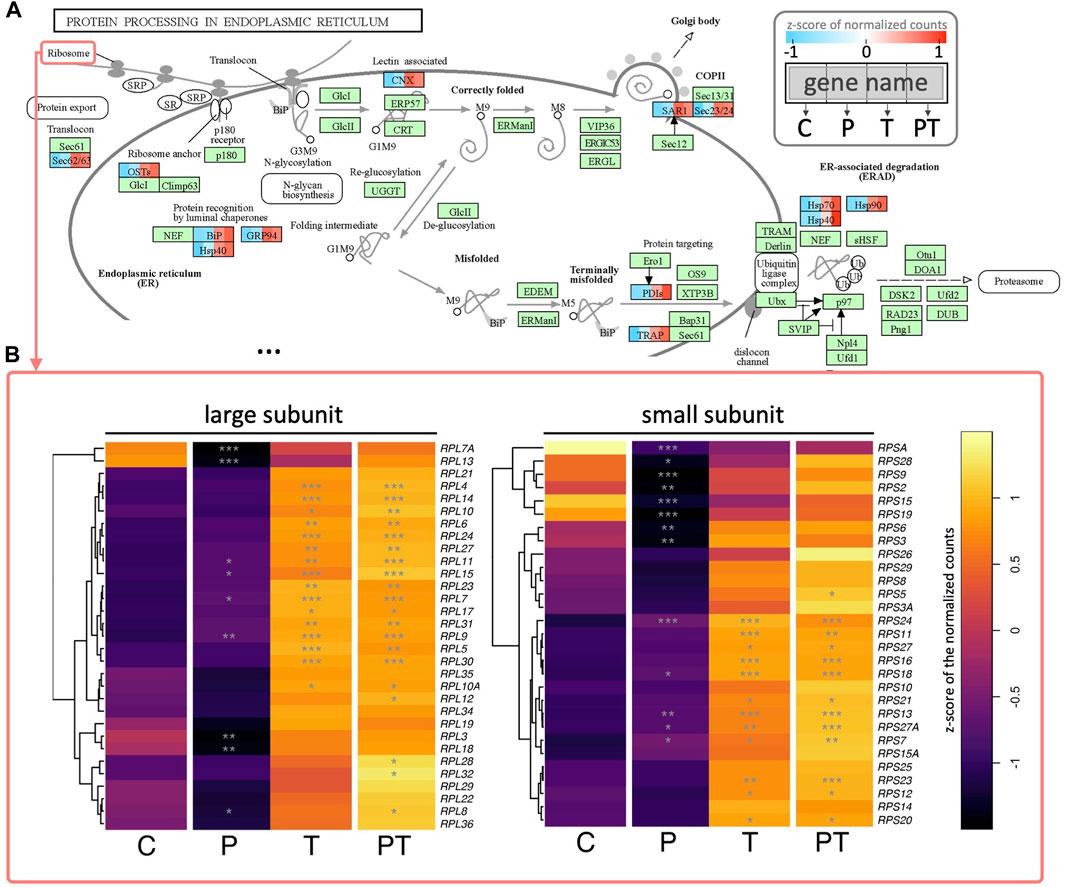
FIGURE 6. (A) Colored KEGG pathway map showing gene names per rectangle. Colored rectangles represent genes significantly enhanced during coupled HP-thermal stimulation, with colors divided into four to represent z-scores of normalized gene counts for different groups. Genes not detected as differentially expressed in coupled HP-thermal stimulation are colored in green. (B) Heatmap of detected ribosomal proteins (from two ribosome subunits). Colors depict z-scores of normalized gene counts, and stars denote statistical significance (based on adjusted p values) compared to control.
Taken together, these results shows that thermal stimulation activated a majority of HSPA members, including the inducible HSPA1A and the constitutively expressed HSPA8, as well as biological processes related to multiple aspects of protein translation and protein processing. These effects appear to be maximized by the coupling of HP and thermal stimulation.
4 Discussion
4.1 Unraveling the effects of HP-thermal biophysical stimuli on chondrocyte homeostasis within native cartilage environment
Cartilage, the load-bearing tissue, and its resident chondrocytes consistently experience external biophysical stimuli throughout our daily activities. While prior investigations utilizing 2D chondrocyte cell cultures or 3D chondrocyte-laden constructs have shed light on the beneficial effects of external biophysical stimuli, these studies are hampered by their inability to fully replicate the native cellular environment.
To unveil the mechanisms by which chondrocytes maintain homeostasis within their native environment, we employed human cartilage explants and an in vitro biophysical stimulation platform. This platform emulates essential external biophysical stimuli present within a healthy native environment, including transient temperature increases and cyclic hydrostatic pressure, which are both significant by-products of repetitive loadings. We employed three distinct application patterns to discern the individual contributions of these stimuli to cartilage homeostasis: the naturally occurring coupled stimulation (“PT”), and their uncoupled counterparts (“P”, “T”). Our findings suggest that transient thermal increase plays a dominant role in orchestrating the transcriptomic changes observed in naturally coupled HP-thermal stimulation. Although no significant enhancement of extracellular matrix production was detected at the histological level, which is probably due to the short-term nature of the study, the coupling of thermal and hydrostatic pressure stimulation resulted in the highest observed chondroprotection, as evidenced by the augmented negative regulation of apoptosis signaling pathways. Furthermore, the expression of multiple HSPA isoforms was significantly amplified with coupled stimulation, as indicated by the highest normalized gene counts. Thermal stimulation activated various biological processes related to protein translation and protein processing at the transcriptomic level, suggesting its crucial role in regulating cartilage homeostasis.
Our findings underscore the potential benefits of incorporating thermal stimulation into tissue engineering, cell therapies, and physiotherapy. As thermal stimulation naturally accompanies mechanical stimulation in healthy knee cartilage, its strategic use could enhance treatment effectiveness. Moreover, the upregulation in Heat Shock Protein 70 (HSPA) expression and enhancement of biological processes related to protein translation and processing due to the involvement of thermal stimulation, indicate their potential roles in modulating cartilage homeostasis. Future studies should further investigate HSPA and its related biological processes activities, with the objective of uncovering innovative approaches to improve therapeutic outcomes in cartilage repair and regenerative medicine. Potential therapeutic interventions might include warming the knee cartilage to mimic loading-induced temperature increase during physiotherapy sessions to boost HSPA expression, or employing pharmaceutical agents to elevate HSPA levels and enhance protein translation and processing activity. Such strategies might optimize the benefits derived from treatment sessions.
4.2 Reflections on experimental design
In this investigation, we employed bulk RNA sequencing on explants derived from the cartilage of a 14-year-old donor, with the aim to investigate the response of chondrocytes to naturally coupled HP-thermal biophysical stimuli. Though the explants were harvested from a region affected by traumatic Osteochondritis Dissecans (OCD), the surgeon did not identify it as inflammatory. Histological staining further confirmed the normal appearance of our explant, resembling healthy tissue without any significant loss of extracellular matrix. These observations affirm the structural integrity of our biological tissue, suggesting its biochemical composition aligns closely with that of native healthy knee cartilage. To mitigate potential biases from initial sample variations, biopsies were grouped and randomized based on their original locations. For our analyses, we utilized triplicates in histological examinations and two samples per group for RNA-sequencing. When analyzing results, we emphasized significant findings to ensure a reliable and generalizable interpretation of the data.
The explants we used represented an array of cartilage zones—superficial, middle, deep, and calcified—encompassing the inherent heterogeneity of chondrocytes in various stages of cellular differentiation. However, it is important to note that all cells within our explant, regardless of their zonal origin, belong to the chondrocyte lineage. This methodology aligns with previous studies, which also adopted a similar approach, utilizing bulk tissue samples for RNA sequencing to investigate the overall tissue response to varying stimuli (Lv et al., 2019; Li and Wang, 2021; Hegenbarth et al., 2022). This tissue-level perspective offers a more comprehensive understanding of the coupled HP-thermal effects on cartilage, enhancing the relevance of our findings to clinical settings such as drug discovery or physiotherapy. We anticipate future studies could build upon our findings by adopting single-cell sequencing approaches to delineate the cell-type-specific responses. This will allow for even more nuanced insights into chondrocyte responses to these stimuli.
Our investigation focused on a relatively brief period of stimulation, i.e., 5 days, a strategy determined by our objective to probe the activation of biological processes at the transcriptomic level. Conventional in vitro studies necessitate longer culture periods to discern changes at the structural protein level. However, a more compact study timeframe was deemed sufficient for our goals, while also ensuring the preservation of cellular viability in their native environment. Throughout our experiment, we observed no significant variation in extracellular matrix production across different groups, as demonstrated by histology images. Similarly, gene expressions of COL2A and aggrecan remained stable. This observation is congruous with our expectations since healthy cartilage, characterized by a low metabolic rate and well-established ECM, is unlikely to manifest marked ECM changes under physiologically relevant external stimulations during our selected timeframe (Goldring and Marcu, 2009; Sophia Fox et al., 2009; Goldring, 2012).
4.3 Thermal stimulation may offer an adaptive mechanism for chondroprotection and chondroinduction
Contrasting with the effects of thermal stimulation, our data demonstrated no substantial impact of isolated hydrostatic pressure. We propose that the transient thermal increase, potentially sensed via thermally sensitive ion channels (such as TRPV4) or HSP70-associated pathways, generates a more immediate response by swiftly activating pertinent biological cascades (Nasrollahzadeh et al., 2022). Our observations suggest that short-term thermal stimulation has the potential to positively influence chondroinduction and chondroprotection, possibly by amplifying protein translation and processing. This enhancement might serve as an adaptive mechanism, making the cells to be more sensitive to mechanical stimulations over extended periods. Further experiments are needed to verify this hypothesis.
HSPA, also known as HSP70, belongs to a protective chaperone protein family crucial for protecting cells from various stresses (Ohtsuka and Hata, 2000; Rérole et al., 2011; Rosenzweig et al., 2019). Numerous studies have highlighted the chondroprotective role of HSPA in both mitigating osteoarthritis and reducing chondrocyte apoptosis (Grossin et al., 2003; Etienne et al., 2008; Kubo et al., 2001; Zhang et al., 2022). Our current research revealed that isolated transient thermal increase stimulates HSPA upregulation, a phenomenon that is further amplified when coupled with hydrostatic pressure stimulation, as demonstrated by the upregulation of genes like HSPA1A and HSPA1B. These results resonate with our earlier research using 3D chondrocyte-laden GelMA constructs (Guo et al., 2023). Notably, HSF, the upstream regulator of HSP70, was not elevated by thermal stimulation. We speculate that the HSP70 increase may stem from greater HSF trimerization and nuclear entry, fostering enhanced HSP70 transcription. This process could be an outcome of the improved protein translation and processing biological processes observed when thermal stimulation is applied to the explants. A more comprehensive study of the HSP70-related signaling pathway is needed to definitively determine if HSP70 facilitates the synergistic support of cartilage homeostasis engendered by the coupling of hydrostatic pressure and thermal stimulation.
The observed thermomechanical synergy and the critical role of thermal stimulation on chondrocytes behavior partially align with our previous research (Nasrollahzadeh et al., 2022; Guo et al., 2023; Stampoultzis et al., 2023). In those studies, the introduction of thermal stimulation to mechanical stimulation resulted in a synergistic enhancement of both chondroinductive and chondroprotective capabilities, at both gene and protein levels. In the present study using human cartilage explants, however, we did not observe notable differences in main structural proteins at either gene or protein expression levels. The discrepancy with the previous studies can be attributed to fundamental differences between hydrogel structures and native tissue.
5 Conclusion
In this study we delved deeply into the intricate interplay between the naturally coupled hydrostatic pressure and loading-induced thermal stimulation on chondrocytes in tissue level. We found that the thermal component predominantly influences the transcriptional profile of chondrocytes during short-term combined thermal-HP stimulation. When this temperature rise was coupled with HP, we noted a significant boost in chondroinduction and chondroprotection compared to singular stimulations. These effects were evidenced by relatively enhanced biological processes and raised levels of Heat Shock Protein 70 (HSPA) compared to isolated stimulations. Moreover, the simultaneous increase in protein translation and protein processing biological processes, was triggered by thermal stimulation. Our insights highlight the potential of incorporating thermal stimulation, which is inherent in healthy cartilage during repetitive loading, into therapeutic approaches like tissue engineering and physiotherapy. Potential treatments could involve heating the knee cartilage during physiotherapy sessions based on temperature patterns found in healthy cartilage, or using medications to boost HSPA levels and protein translation and processing activity, aiming to optimize therapeutic outcomes.
Data availability statement
The datasets presented in this study can be found in online repositories. The names of the repository/repositories and accession number(s) can be found below: https://www.ncbi.nlm.nih.gov/geo/, GSE242710.
Ethics statement
The studies involving humans were approved by Vaud cantonal State Ethics Committee reference No 264/12. The studies were conducted in accordance with the local legislation and institutional requirements. The human samples used in this study were acquired from Clinical cartilage samples were anonymized, stored, and logged in a Musculoskeletal Department Biobank, complying with internal regulations thereof and following an approved protocol (i.e., Vaud cantonal State Ethics Committee reference No 264/12). Written informed consent for participation was not required from the participants or the participants’ legal guardians/next of kin in accordance with the national legislation and institutional requirements.
Author contributions
YG: Conceptualization, Data curation, Formal Analysis, Methodology, Visualization, Writing–original draft, Writing–review and editing. TS: Methodology, Writing–review and editing. DP: Conceptualization, Supervision, Writing–review and editing.
Funding
The author(s) declare that no financial support was received for the research, authorship, and/or publication of this article.
Acknowledgments
Doctor Robin MARTIN for suppling the explants used in this study, EPFL HCF facility for their assistance in histology staining, EPFL GECF facility for their assistance in RNA-sequencing, EPFL BICC center for their assistance in bioinformatic analyses, Virginie PHILIPPE and Sandra JACCOUD for their technical assistance.
Conflict of interest
The authors declare that the research was conducted in the absence of any commercial or financial relationships that could be construed as a potential conflict of interest.
Publisher’s note
All claims expressed in this article are solely those of the authors and do not necessarily represent those of their affiliated organizations, or those of the publisher, the editors and the reviewers. Any product that may be evaluated in this article, or claim that may be made by its manufacturer, is not guaranteed or endorsed by the publisher.
Supplementary material
The Supplementary Material for this article can be found online at: https://www.frontiersin.org/articles/10.3389/fmede.2023.1291191/full#supplementary-material
References
Abdel-Sayed, P., Moghadam, M. N., Salomir, R., Tchernin, D., and Pioletti, D. P. (2014). Intrinsic viscoelasticity increases temperature in knee cartilage under physiological loading. J. Mech. Behav. Biomed. Mater. 30, 123–130. doi:10.1016/j.jmbbm.2013.10.025
Ahearne, M. (2014). Introduction to cell–hydrogel mechanosensing. Interface Focus 4, 20130038. doi:10.1098/rsfs.2013.0038
Anderson, D. E., and Johnstone, B. (2017). Dynamic mechanical compression of chondrocytes for tissue engineering: a critical review. Front. Bioeng. Biotechnol. 5, 76. doi:10.3389/fbioe.2017.00076
Antons, J., Marascio, M. G. M., Nohava, J., Martin, R., Applegate, L. A., Bourban, P. E., et al. (2018). Zone-dependent mechanical properties of human articular cartilage obtained by indentation measurements. J. Mat. Sci. Mat. Med. 29, 57. doi:10.1007/s10856-018-6066-0
Becher, C., Springer, J., Feil, S., Cerulli, G., and Paessler, H. H. (2008). Intra-articular temperatures of the knee in sports – an in-vivo study of jogging and alpine skiing. BMC Musculoskelet. Disord. 9, 46. doi:10.1186/1471-2474-9-46
Chaudhuri, O., Gu, L., Klumpers, D., Darnell, M., Bencherif, S. A., Weaver, J. C., et al. (2016). Hydrogels with tunable stress relaxation regulate stem cell fate and activity. Nat. Mater. 15, 326–334. doi:10.1038/nmat4489
Dobin, A., Davis, C. A., Schlesinger, F., Drenkow, J., Zaleski, C., Jha, S., et al. (2013). STAR: ultrafast universal RNA-seq aligner. Bioinformatics 29, 15–21. doi:10.1093/bioinformatics/bts635
Elder, B. D., and Athanasiou, K. A. (2009). Hydrostatic pressure in articular cartilage tissue engineering: from chondrocytes to tissue regeneration. Tissue Eng. Part B Rev. 15, 43–53. doi:10.1089/ten.teb.2008.0435
Etienne, S., Gaborit, N., Henrionnet, C., Pinzano, A., Galois, L., Netter, P., et al. (2008). Local induction of heat shock protein 70 (Hsp70) by proteasome inhibition confers chondroprotection during surgically induced osteoarthritis in the rat knee. Bio-Medical Mater. Eng. 18, 253–260. doi:10.3233/BME-2008-0534
Fahy, N., Alini, M., and Stoddart, M. J. (2017). Mechanical stimulation of mesenchymal stem cells: implications for cartilage tissue engineering. J. Orthop. Res. 36, 52–63. doi:10.1002/jor.23670
Frankish, A., Diekhans, M., Ferreira, A.-M., Johnson, R., Jungreis, I., Loveland, J., et al. (2019). GENCODE reference annotation for the human and mouse genomes. Nucleic Acids Res. 47, D766–D773. doi:10.1093/nar/gky955
Goldring, M. B. (2012). Chondrogenesis, chondrocyte differentiation, and articular cartilage metabolism in health and osteoarthritis. Ther. Adv. Musculoskelet. Dis. 4, 269–285. doi:10.1177/1759720X12448454
Goldring, M. B., and Marcu, K. B. (2009). Cartilage homeostasis in health and rheumatic diseases. Arthritis Res. Ther. 11, 224. doi:10.1186/ar2592
Grossin, L., Cournil-Henrionnet, C., Pinzano, A., Gaborit, N., Dumas, D., Etienne, S., et al. (2006). Gene transfer with HSP 70 in rat chondrocytes confers cytoprotection in vitro and during experimental osteoarthritis. FASEB J. 20, 65–75. doi:10.1096/fj.04-2889com
Grossin, L., Cournil-Henrionnet, C., Watrin-Pinzano, A., Terlain, B., Jouzeau, J., Netter, P., et al. (2003). Overexpression and induction of heat shock protein 70 protect chondrocytes from cell death in vitro and in vivo. Arthritis Res. Ther. 5, 53. doi:10.1186/ar854
Guo, Y., Stampoultzis, T., Nasrollahzadeh, N., Karami, P., Rana, V., Applegate, L. A., et al. (2023). Unraveling cartilage degeneration through synergistic effects of hydrostatic pressure and biomimetic temperature increase. iScience. doi:10.1016/j.isci.2023.108519
Hegenbarth, J.-C., Lezzoche, G., De Windt, L. J., and Stoll, M. (2022). Perspectives on bulk-tissue RNA sequencing and single-cell RNA sequencing for cardiac transcriptomics. Front. Mol. Med. 2, 839338. doi:10.3389/fmmed.2022.839338
Ito, A., Aoyama, T., Iijima, H., Nagai, M., Yamaguchi, S., Tajino, J., et al. (2014). Optimum temperature for extracellular matrix production by articular chondrocytes. Int. J. Hyperth. 30, 96–101. doi:10.3109/02656736.2014.880857
Ito, A., Aoyama, T., Iijima, H., Tajino, J., Nagai, M., Yamaguchi, S., et al. (2015b). Culture temperature affects redifferentiation and cartilaginous extracellular matrix formation in dedifferentiated human chondrocytes: culture Temperature in Chondrocytes. J. Orthop. Res. 33, 633–639. doi:10.1002/jor.22808
Ito, A., Nagai, M., Tajino, J., Yamaguchi, S., Iijima, H., Zhang, X., et al. (2015a). Culture temperature affects human chondrocyte messenger RNA expression in monolayer and pellet culture systems. PLOS ONE 10, e0128082. doi:10.1371/journal.pone.0128082
Jiang, Y., and Tuan, R. S. (2015). Origin and function of cartilage stem/progenitor cells in osteoarthritis. Nat. Rev. Rheumatol. 11, 206–212. doi:10.1038/nrrheum.2014.200
Kolberg, L., Raudvere, U., Kuzmin, I., Vilo, J., and Peterson, H. (2020). gprofiler2– an R package for gene list functional enrichment analysis and namespace conversion toolset g:Profiler. F1000Research 9, 709. doi:10.12688/f1000research.24956.1
Kotelsky, A., Carrier, J. S., Aggouras, A., Richards, M. S., and Buckley, M. R. (2020). Evidence that reduction in volume protects in situ articular chondrocytes from mechanical impact. Connect. Tissue Res. 61, 360–374. doi:10.1080/03008207.2020.1711746
Kubo, T.O.S.H.I.K.A.Z.U., Arai, Y.U.J.I., Takahashi, K.E.N.J.I., Ikeda, T.A.K.U.M.I., Ohashi, S.U.Z.U.Y.O., Kitajima, I.S.A.O., Mazda, O.S.A.M., Takigawa, M.A.S.A.H.A.R.U., Imanishi, J.I.R.O., and Hirasawa, Y.A.S.U.S.U.K.E. (2001). Expression of transduced HSP70 gene protects chondrocytes from stress. The Journal of Rheumatology, 28 (2), 330–335.
Li, K., Zhang, C., Qiu, L., Gao, L., and Zhang, X. (2017). Advances in application of mechanical stimuli in bioreactors for cartilage tissue engineering. Tissue Eng. Part B Rev. 23, 399–411. doi:10.1089/ten.teb.2016.0427
Li, X., and Wang, C.-Y. (2021). From bulk, single-cell to spatial RNA sequencing. Int. J. Oral Sci. 13, 36. doi:10.1038/s41368-021-00146-0
Liao, Y., Smyth, G. K., and Shi, W. (2014). featureCounts: an efficient general purpose program for assigning sequence reads to genomic features. Bioinformatics 30, 923–930. doi:10.1093/bioinformatics/btt656
Love, M. I., Huber, W., and Anders, S. (2014). Moderated estimation of fold change and dispersion for RNA-seq data with DESeq2. Genome Biol. 15, 550. doi:10.1186/s13059-014-0550-8
Lv, M., Zhou, Y., Polson, S. W., Wan, L. Q., Wang, M., Han, L., et al. (2019). Identification of chondrocyte genes and signaling pathways in response to acute joint inflammation. Sci. Rep. 9, 93. doi:10.1038/s41598-018-36500-2
Martin, J. A., and Buckwalter, J. A. (2001). Roles of articular cartilage aging and chondrocyte senescence in the pathogenesis of osteoarthritis. Iowa Orthop. J. 21, 1–7.
McCreery, K. P., Calve, S., and Neu, C. P. (2020). Ontogeny informs regeneration: explant models to investigate the role of the extracellular matrix in cartilage tissue assembly and development. Connect. Tissue Res. 61, 278–291. doi:10.1080/03008207.2019.1698556
Mohammed, D., Versaevel, M., Bruyère, C., Alaimo, L., Luciano, M., Vercruysse, E., et al. (2019). Innovative Tools for mechanobiology: unraveling outside-in and inside-out mechanotransduction. Front. Bioeng. Biotechnol. 7, 162. doi:10.3389/fbioe.2019.00162
Nasrollahzadeh, N., Karami, P., Wang, J., Bagheri, L., Guo, Y., Abdel-Sayed, P., et al. (2022). Temperature evolution following joint loading promotes chondrogenesis by synergistic cues via calcium signaling. eLife 11, e72068. doi:10.7554/eLife.72068
Ohtsuka, K., and Hata, M. (2000). Molecular chaperone function of mammalian Hsp70 and Hsp40-a review. Int. J. Hyperth. 16, 231–245. doi:10.1080/026567300285259
Picard Tools (2023). By broad Institute. Available at: http://broadinstitute.github.io/picard/index.html.
Rérole, A.-L., Jego, G., and Garrido, C. (2011). “Hsp70: anti-apoptotic and tumorigenic protein,” in Molecular chaperones methods in molecular biology. Editors S. K. Calderwood, and T. L. Prince (United States: Humana Press), 205–230. doi:10.1007/978-1-61779-295-3_16
Rosenzweig, R., Nillegoda, N. B., Mayer, M. P., and Bukau, B. (2019). The Hsp70 chaperone network. Nat. Rev. Mol. Cell Biol. 20, 665–680. doi:10.1038/s41580-019-0133-3
Salinas, E. Y., Hu, J. C., and Athanasiou, K. (2018). A guide for using mechanical stimulation to enhance tissue-engineered articular cartilage properties. Tissue Eng. Part B Rev. 24, 345–358. doi:10.1089/ten.teb.2018.0006
Setton, L. A., Elliott, D. M., and Mow, V. C. (1999). Altered mechanics of cartilage with osteoarthritis: human osteoarthritis and an experimental model of joint degeneration. Osteoarthr. Cartil. 7, 2–14. doi:10.1053/joca.1998.0170
Sophia Fox, A. J., Bedi, A., and Rodeo, S. A. (2009). The basic science of articular cartilage: structure, composition, and function. Sports Health A Multidiscip. Approach 1, 461–468. doi:10.1177/1941738109350438
Stampoultzis, T., Guo, Y., Nasrollahzadeh, N., Karami, P., and Pioletti, D. P. (2023). Mimicking loading-induced cartilage self-heating in vitro promotes matrix formation in chondrocyte-laden constructs with different mechanical properties. ACS Biomater. Sci. Eng. 9, 651–661. doi:10.1021/acsbiomaterials.2c00723
Szczesny, S. E. (2020). Ex vivo models of musculoskeletal tissues. Connect. Tissue Res. 61, 245–247. doi:10.1080/03008207.2020.1742418
Tonomura, H., Takahashi, K. A., Mazda, O., Arai, Y., Inoue, A., Terauchi, R., et al. (2006). Glutamine protects articular chondrocytes from heat stress and NO-induced apoptosis with HSP70 expression. Osteoarthr. Cartil. 14, 545–553. doi:10.1016/j.joca.2005.12.008
Wong, M., and Carter, D. R. (2003). Articular cartilage functional histomorphology and mechanobiology: a research perspective. Bone 33, 1–13. doi:10.1016/S8756-3282(03)00083-8
Keywords: cartilage, homeostasis, bioreactor, hydrostatic pressure (HP), thermal stimulation, biophysical stimulation, RNA-sequencing, chondrocyte
Citation: Guo Y, Stampoultzis T and Pioletti DP (2023) Synergetic support of cartilage homeostasis via coupled thermal-pressure stimuli: a transcriptomic study in human cartilage explants. Front. Med. Eng. 1:1291191. doi: 10.3389/fmede.2023.1291191
Received: 08 September 2023; Accepted: 18 October 2023;
Published: 05 December 2023.
Edited by:
Lorenzo Vannozzi, Sant’Anna School of Advanced Studies, ItalyReviewed by:
Cristina Manferdini, Rizzoli Orthopedic Institute (IRCCS), ItalySebastian L. Vega, Rowan University, United States
Copyright © 2023 Guo, Stampoultzis and Pioletti. This is an open-access article distributed under the terms of the Creative Commons Attribution License (CC BY). The use, distribution or reproduction in other forums is permitted, provided the original author(s) and the copyright owner(s) are credited and that the original publication in this journal is cited, in accordance with accepted academic practice. No use, distribution or reproduction is permitted which does not comply with these terms.
*Correspondence: Dominique P. Pioletti, dominique.pioletti@epfl.ch
†These authors have contributed equally to this work and share first authorship