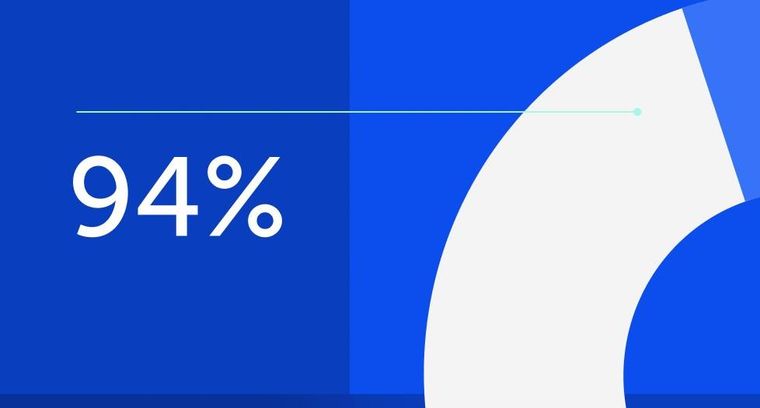
94% of researchers rate our articles as excellent or good
Learn more about the work of our research integrity team to safeguard the quality of each article we publish.
Find out more
ORIGINAL RESEARCH article
Front. Mech. Eng., 04 April 2025
Sec. Biomechanical Engineering
Volume 11 - 2025 | https://doi.org/10.3389/fmech.2025.1505969
This article is part of the Research TopicAdvancing Musculoskeletal Health: Bridging Basic and Clinical Research on Biomechanical Properties of Joints, Ligaments, Tendons, and Associated StructuresView all 3 articles
The shoulder joint in the human body is a complex anatomical structure composed of diverse biological tissues that connect and stabilize the joint. This complexity allows the shoulder to perform a wide range of movements and develop specific skills compared to other joints. However, activities such as heavy lifting, forceful jerks of the arm, rapid or aggressive movements of the arm above the shoulder, or sports activities involving repetitive motions can lead to a shoulder labral tear. This injury, known as superior labrum anterior to posterior (SLAP) type II tear, damages the joint, weakens its stability, and limits its motion. The tear diagnosis is based on physical examination tests such as the O'Brien test, the Jobe relocation test, or the internal impingement sign. This research evaluated the shoulder joint from a biomechanical perspective by applying the finite element method to a virtual complex shoulder joint biomodel and analyzing the main elements of the joint, including the bones (cortical and cancellous), ligaments (labrum and joint capsule), and articular cartilage. The main objective was to analyze the effects of loading on the labrum and joint capsule tissues by applying an external load on the humerus, simulating the shoulder abduction movement, and obtaining stress and von Mises stress results. A case study of a healthy shoulder joint structure is developed for comparison. Subsequently, a biomodel modification is proposed to virtually represent the SLAP type II tear in the labral tissue, allowing for numerical analysis of the three-dimensional biomodel. This study investigated the regions of the labrum and capsule most susceptible to tears in the presence of a SLAP type II tear. The labrum does not effectively deepen the socket of the humeral head in the glenoid cavity, resulting in increased mobility of the humeral head. The simulation result is shown to align with observations noted in clinical practice.
The shoulder is the joint where the arm starts and connects the upper limb and the torso, forming the shoulder girdle (Chang et al., 2023); it is essential to outline its anatomical structure, which includes the clavicle, scapula, and humerus. The labrum is a fibrocartilaginous tissue that surrounds the glenoid cavity edge, enhancing its depth and improving the fit of the humeral head (Brzóska et al., 2017). Its main function is to increase the depth of the glenoid cavity, enhancing its depth and improving the humeral head fit. The joint capsule is a loose tissue that allows for a wide range of motion in the glenohumeral joint. This shoulder joint capsule is a tight sac made of fibrous lax tissue that encases the glenohumeral joint, extending from the scapula to the humerus (Saccomanno et al., 2013).
One of the most prevalent injuries affecting the labrum is the superior labrum anterior to posterior (SLAP) tear (Figure 1). It is primarily diagnosed using a combination of clinical examination and imaging studies. Physical examinations may include specific tests such as the O'Brien’s test, the Jobe relocation test, or the internal impingement sign, which help identify the presence of an SLAP tear through pain or internal tissue impingement sensation during shoulder movements (Mathew and Lintner, 2018). This study focuses on developing a virtual simulation of the SLAP lesion based on an analysis conducted from January 1995 to June 1998 (Bencardino et al., 2000). The research included data from 159 patients, consisting of 123 men and 36 women, to improve understanding of this shoulder injury. Among the patients, a subset experienced chronic pain or instability in the shoulder and underwent magnetic resonance arthrography for diagnosis. It is important to note that while magnetic resonance procedures are commonly used, they may not provide an optimal diagnostic assessment because they often fail to accurately depict the extent of the lesion. Consequently, surgical procedures were necessary to diagnose 28 patients (19 showed SLAP type I tears, and nine showed SLAP type II tears).
Figure 1. On the left, shoulder joint anatomy. On the right is an example of an SLAP type II tear and quadrants in the sagittal plane.
In this research, a complex biomodel of the shoulder joint is developed based on information from computed tomography (CT) of the upper joint, which is stored in a DICOM file. A methodology described in previous publications (Hernández-Vázquez et al., 2020; Marquet-Rivera et al., 2021; Jang et al., 2020; Martinez-Mondragon et al., 2022) was followed to develop a virtual model that accurately represents the shoulder morphology. Different process stages were developed, where the surfaces of the elements that build the shoulder (bone, ligaments, joint capsule, and articular cartilage) are developed. These components were refined through a process that modifies their volume, smooths their surfaces, and produces a solid model. The first case study considers a healthy shoulder joint to establish a baseline criterion for the biomodel joint behavior, serving as a comparative reference. Additionally, modifications were made to the upper area of the labrum to depict the disinsertion of the tissue from its normal position, resembling an SLAP type II tear. Both biomodels (a healthy shoulder biomodel and the SLAP type II tear biomodel) were exported to a finite element method (FEM) software to perform a numerical simulation to obtain the numerical data. Comparisons were made between both cases (a healthy shoulder biomodel and the SLAP type II tear biomodel) to understand the labrum and capsule behaviors numerically during abduction motion when the lesion is present.
Computational biomodels are characterized by replicating diverse biological structures of the human body based on medical images, using modeling techniques for elaboration (Zheng et al., 2017). They are characterized by their precision and accurate representation of the human anatomy. They represent an alternative for the study of different biological tissues that avoid ethical, legal, and moral problems because invasive techniques that affect the patient’s physical health are not used to obtain them, saving time and economic and material resources. Biomodels are used in simulations where the behavior of biological structures is analyzed numerically (Lohfeld et al., 2005). CT is a non-invasive scanning technique that provides image information on tissue geometry stored in a DICOM file (Kramme et al., 2011). The file goes through post-processing computer programs to generate the volumetric model (Figure 2e), applying a methodology that simplifies model development regardless of complexity.
Figure 2. Steps for developing a 3D biomodel of the anatomical structures of the shoulder joint. (a) Data acquisition from a tomography scan. (b) DICOM file imported into Mimics Research®. (c) Image processing: the model structures are delimited. (d) Surface repair and geometry smoothing from Materialise 3-matic®. (e) Final shoulder joint biomodel.
The methodology that was to be followed for the development of the shoulder joint biomodel used in this research is presented below (Correa-Corona et al., 2023; Dhanopia and Bhargava, 2017; Trejo-Enriquez et al., 2023; Serrato-Pedrosa et al., 2024):
• Data acquisition: CT images of a 23-year-old female volunteer’s torso area are used to create the DICOM file to develop the biomodel. These images provide information about the dimensions and position of the body’s internal structures (Figure 2a).
• Image processing consists of importing a DICOM file into a CAD-type computer program for image processing, where the elements forming part of the three-dimensional model are outlined and delimited (Figures 2b, c). Mimics Research® (version 21.0) software was used to develop the model.
• File refinement for generation of the 3D biomodel: The process continues by improving the element surfaces, using tools to remove repeated surfaces, repair, and smoothing geometry (Figure 2d). Once the refinement process is finished, the volumetric model is generated for further analysis. Each of the biomodel elements is saved with an STL extension. Finally, a biomodel of the shoulder joint is obtained. The refinement process was conducted within Materialise 3-matic® (version 13.0) software.
• Exporting CAD model: The biomodel was developed in CAD software and exported for performing structural numerical analysis into an FEM-method software ANSYS Workbench® (Figure 2e).
The bone segmentation of cortical and cancellous surfaces was performed using a combination of automatic thresholding and manual labeling techniques. Ligament and joint capsule segmentation were performed manually, according to what is reported in the literature, based on ligament origin and insertions in the bones (Kadi et al., 2017). For the humeral head cartilage, a 1 mm outward offset of the humeral head surface was performed (Yeh et al., 1998). For the glenoid cartilage, a 2-mm extrusion of the glenoid cavity surface was performed along the lateral direction, and a Boolean operation cut was applied between the glenoid cartilage, labrum, and articular capsule to ensure consistency between them (Favre et al., 2012).
The three-dimensional shoulder joint biomodel developed has 19 structural components. These include six components (humerus, scapula, and clavicle) that are rigid structures of cortical bone and cancellous bone and two components (glenoid cartilage and cartilage humeral head) for articular cartilage. The remaining 11 components correspond to soft tissues (glenohumeral ligaments, acromioclavicular ligament, coracoacromial ligament, trapezoidal and conoid ligaments, superior transverse scapular ligament, labrum, and joint capsule) (Figure 3) (Suarez-Hernandez et al., 2024).
Figure 3. Shoulder joint biomodel with soft tissues, including bones (cortical and cancellous) and the ligaments that hold the bones together.
Different types of biological material (cortical bone and cancellous bone) are applied in this research. It is assumed that bone is rigid (although cortical bone is more rigid than cancellous bone), homogeneous, isotropic, and behaves in a linear-elastic manner. In the case of ligament and cartilage, despite having very different characteristics from bone, it will be assumed that they have the same homogeneity, are isotropic, and are linear-elastic. In the case of biological tissues, there are no exact values due to aspects such as lifestyle, injuries, physiology, and age, among other factors. Therefore, approximations of the mechanical properties can be made based on data from previous studies (Favre et al., 2012; Hayes et al., 1991; Abril et al., 2015; Clavert et al., 2006; Büchler et al., 2002). The characterization of biological tissues (cortical bone, cancellous bone, ligaments, and cartilage) relies on these prior works and is summarized in Table 1. For this study, the mechanical properties of the labrum and capsule joint were classified as equivalent to those of a ligament. This simplification allows for a focus on the geometry of the model.
Two case studies are considered in this research. Initially, the biomodel considers the biological tissue structure of the healthy shoulder joint (especially the labrum component); this case will serve as a comparison case. Subsequently, a labrum with deterioration due to wear is simulated to consider an SLAP type II tear lesion in the numerical analysis and compare it against the data results from a healthy biological system.
The identification of the SLAP type II tear lesion is commonly performed by physical examination. It is not the best option for diagnosis because morphologically fibrocartilaginous tissue presents variations, which can be confused with other types of injuries (Kanatli et al., 2010). In addition, no apparent symptoms exist to establish a difference against other conditions (O’Kane and Toresdahl, 2014). In the second case, a modification into the biomodel components in the upper area of the labrum is produced by eliminated tissue, recreating the SLAP type II tear, which corresponds to the degeneration and partial detachment of the upper part of the labrum and the tendon head of the biceps (Fitzpatrick et al., 2021). Figure 4 compares the model of the healthy shoulder in contrast to the model of the shoulder with an injury. Likewise, for the development of the numerical simulation, the material’s mechanical properties, boundary conditions, and applied load are replicated in both cases.
Figure 4. Numerical biomodel comparison. On the left is a healthy biomodel, and on the right is a biomodel with an SLAP type II tear.
Previously developed works on analyzing structures part of the shoulder consider only a few tissues as part of the joint complex, imposing boundary conditions (Yeh et al., 2005; Ellis et al., 2007; Adams et al., 2007) and loading conditions (Suarez-Hernandez et al., 2024; Luo et al., 1998; Ellis et al., 2007; Sano et al., 2006; Seki et al., 2008; Webb et al., 2014). For both numerical simulations, the boundary conditions have embedded characteristics (Figure 5) according to the union between the shoulder and the torso, between the clavicle and the sternum (the sternoclavicular joint), and the scapula on the medial border, where muscle attachments occur.
Figure 5. Boundary conditions application and loading. On the left, mark A is the sternal facet on the sternal end of the clavicle. Mark B is the medial margin of the scapula.
Both numerical evaluations are performed by applying structural-static concepts, considering linear-elastic behavior, isotropic considerations, and mechanical properties from Table 1. The biomodel discretization is developed in an accessible manner, producing triangular elements. For bones, the discretization is generated by a triangle length of 2 mm; for soft tissues, the discretization considers a triangle length of 1 mm. The biomodel comprises 19 solids (Figure 6) (Suarez-Hernandez, 2023).
Figure 6. Shoulder discretization joint biomodel with soft tissues in the ANSYS Workbench® software for numerical analysis.
The research focuses on learning about the effects of sudden abduction movement on the static stabilizers of the shoulder. The shoulder joint numerical simulation data represent the abduction movement, allowing us to evaluate the stress and the von Mises stress. Figure 7 compares von Mises’s results between the healthy biomodel labrum and the labrum of the biomodel that presents the SLAP tear. The results are divided into the quadrants in which the labrum is divided, which allows us to observe the region where the joint is most stressed when the joint performs the abduction movement. It is observed that the labrum that presents the SLAP lesion presents less stress than the healthy labrum, except for the anteroinferior zone, which presents a stress of 10.52 MPa. The joint labrum does not present any lesion and has a stress of 11.5 MPa in the posteroinferior zone. Figure 8 shows the von Mises results obtained from the numerical simulation.
Figure 7. von Mises stress results comparison that presents the labrum areas during abduction movement.
Figure 8. Maximum von Mises stress results of the labrum. On the left side is a healthy labrum, and on the right side is the biomodel labrum with an SLAP tear.
Figure 9 shows a comparison of the results of the distribution of stress in the labrum, which is not uniform. The highest value, 10.16 MPa, was presented in the anteroinferior zone. However, in the injured labrum, the values obtained in the different areas are doubled, reaching a maximum value of 22.33 MPa in the anteroinferior zone. Meanwhile, Figure 10 shows the stress results obtained from the numerical analysis simulation.
Figure 10. Stress results of the labrum. On the left side is a healthy labrum, and on the right side is the biomodel labrum with an SLAP tear.
Figure 11 shows the von Mises stress results obtained in the joint capsule, as well as in the axillary recess and the labral area. In the articular capsule, when there is a lesion in the labrum, a value of 10.65 MPa was obtained in the healthy articular capsule. In contrast, in the capsule corresponding to the model with the lesion, values of 15.75 MPa were obtained. The axillary recess is the region where there is a marked difference because, in the model, lesion values of 19.84 MPa were obtained, while in the healthy capsule, values of 14.67 MPa were obtained. Comparing the biomodel results, the healthy model shows a 13.15 MPa von Mises stress value, while the injured model slightly increases to 13.95 MPa. Meanwhile, Figure 12 shows the von Mises results from the numerical analysis simulation.
Figure 11. von Mises stress results comparison that presents different areas of the shoulder articular capsule joint.
Figure 12. Maximum von Mises stress results from the shoulder joint articular capsule. On the left side is a healthy articular capsule, and on the right side is the biomodel articular capsule with an SLAP tear.
While Figure 13 shows that the stress in the capsule is lower in the model with the lesion, values of 5.7 MPa were obtained; on the other hand, the capsule of the healthy model obtained values of 13.39 MPa. There was a notable difference in the axillary recess area because, in the healthy model of the articular capsule, values of 7.9 MPa were obtained. In contrast, lesion values of 16.71 MPa were obtained in the model capsule. In the area shared with the labrum, values of 5.12 MPa were obtained in the healthy model, while values of 4.05 MPa, lower than the healthy model, were obtained in the model with the lesion. Figure 14 shows the stress results obtained from the numerical analysis simulation.
Figure 14. Stress results of the joint capsule. On the left side is a healthy articular capsule, and on the right side is the biomodel articular capsule with an SLAP tear.
Certain studies address a numerical analysis of SLAP type II tears. This work aimed to determine the areas of the labrum and joint capsule most prone to injury during the abduction movement while the SLAP tear is present. The results indicated a reduction in stress distribution because the labrum tissue does not effectively deepen the socket of the humeral head in the glenoid cavity, resulting in increased mobility of the humeral head (Kadi et al., 2017). In the model with injury, the shoulder joint capsule in the axillary recess area presents the highest stress, which could be related to thickening signs of adhesive capsulitis (Sernik et al., 2019).
The labrum results show that in the biomodel without a lesion, the greatest von Mises stress is presented in the posteroinferior area (11.5 MPa). In the other areas, the von Mises stress results are similar. However, the most affected region in the labrum with a lesion is the anteroinferior area, which suffers a 66% increase relative to the healthy labrum. In contrast, the posteroinferior area decreases, with a 1.7 MPa von Misses stress. The biomodel with lesion capsule exhibited a 47% increase in von Mises stress compared to the healthy biomodel capsule. Additionally, the area of the axillary recess increased by 35% compared with the healthy biomodel, but the region where the capsule and the labrum overlap showed only a 5.3% increase in von Mises stress compared to the healthy biomodel.
The results of this study are highly significant, demonstrating that the stress present in the capsule tissues plays a crucial role in maintaining the balance in the joint. Moreover, the axillary recess of the biomodel is essential for joint movement, supporting the humeral head as the capsule does. In contrast, the labral tissue in the anteroinferior area shows high-stress values, with a healthy labrum at 1.6 MPa and an injured labrum soaring to 22.33 MPa, suggesting that, without prompt treatment, a significant tear is probable in this area. The rest of the labrum areas revealed a stress reduction in distribution, indicating instability within the joint. This instability occurs because the humeral head does not fully engage with the glenoid, which diminishes the effectiveness of humeral shock absorption during joint abduction movement.
The findings presented in this article align with the results obtained by Maldonado et al. (2023), who conducted a numerical evaluation of the SLAP type II lesion. Their study indicates that the area of the axillary recess is particularly vulnerable to injury, highlighting its critical role during joint movement. Furthermore, it is validated that the capsule is essential for maintaining joint stability by restricting the movement of the humerus.
Numerical simulations using numerical models allow the investigation of aspects that would otherwise be difficult to study, overcoming technical and ethical limits. Improving software and computational power has been fundamental for modeling, developing, and resolving increasingly complex models. The article explains the methodological steps to replicate the shoulder joint biomodel for its study in patients with similar lesions using medical images such as CT. The presented methodology can replicate other body joints or biological tissues for numerical analysis. This research uses a CT scan of an uninjured shoulder to develop a finite element model to investigate the SLAP lesion. To represent this SLAP type II lesion, an alteration in the model’s geometry was performed to identify vulnerable areas of the tissue, indicating the potential for additional conditions. This section provides knowledge of the impact of natural shoulder motion on joint function, emphasizing the role of static stabilizers such as the joint capsule and labrum.
The numerical representation of biological tissue materials was treated as isotropic rather than anisotropic because the tension along the fiber direction was not considered. Soft tissues were assumed to have isotropic properties, which means their characteristics are directionally independent. This simplification allowed a more straightforward approach to formulating the numerical model and problem resolution.
The shoulder joint has a range of movements and morphological variations, and predictions from a single model may have limitations, so it is fundamental to consider that the results presented in this research are from a single, specific patient model. Because variables such as age, lifestyle, gender, impact of complex daily activities, anatomical variations (Kadi et al., 2017), biomechanical variability due to previous pathologies, and simplified material properties, among others, are not taken into consideration, it cannot be concluded that they apply to the population with this type of injury. In addition, this analysis was developed from a simple and primary representation of the SLAP lesion.
This study sets the tone for interdisciplinary collaboration between clinicians and biomechanical engineers to improve its comprehensiveness and relevance to clinical practice. It offers a promising avenue for simulation testing and treatment exploration and provides valuable insights into conditions before surgical intervention.
The case studies presented in this work have helped to understand the effects of the shoulder’s natural movement on the functioning of the joint, emphasizing the interaction of the static limiters that give stability to the joint, highlighting the results of the effect of internal forces that produce soft tissue displacement (ligaments). The study shows the areas susceptible to presenting a tear in the healthy model due to the concentrations of stresses and the intensification of these, which agree with the clinical observations published in previous articles. In addition, the model with lesions shows the areas where the tissue could be susceptible to presenting another condition. The difference in biomechanics was due to the alteration of the geometry of the tissue representing the labrum to represent the SLAP type II tear because the mechanical properties of the tissues and boundary conditions were the same in both models. It is essential to point out that currently, the development of biomodels is a representation with a high level of characterization of the human body, which is why they represent a viable and effective alternative to carry out simulation tests for the knowledge and treatment of conditions before reaching surgery.
The original contributions presented in the study are included in the article/supplementary material; further inquiries can be directed to the corresponding authors.
MS-H: Formal Analysis, Investigation, Methodology, Writing – original draft, Writing – review and editing. GU-S: Software, Supervision, Writing – original draft, Writing – review and editing. BR-Á: Formal Analysis, Software, Writing – original draft, Writing – review and editing. FC-H: Formal Analysis, Software, Writing – original draft, Writing – review and editing. JM-R: Methodology, Software, Writing – original draft, Writing – review and editing. FG-F: Formal Analysis, Validation, Writing – original draft, Writing – review and editing. EV-L: Conceptualization, Supervision, Validation, Writing – original draft, Writing – review and editing. MC-C: Conceptualization, Data curation, Formal Analysis, Software, Writing – original draft, Writing – review and editing. JG-C: Conceptualization, Investigation, Writing – original draft, Writing – review and editing. GU-C: Methodology, Project administration, Visualization, Writing – original draft, Writing – review and editing.
The author(s) declare that no financial support was received for the research and/or publication of this article.
The authors gratefully acknowledge the Instituto Politécnico Nacional and CONHCYT for their support.
The authors declare that the research was conducted in the absence of any commercial or financial relationships that could be construed as a potential conflict of interest.
The author(s) declare that no Generative AI was used in the creation of this manuscript.
All claims expressed in this article are solely those of the authors and do not necessarily represent those of their affiliated organizations, or those of the publisher, the editors and the reviewers. Any product that may be evaluated in this article, or claim that may be made by its manufacturer, is not guaranteed or endorsed by the publisher.
Abril, H. A. C., Ramírez, A., and Silva, L. (2015). Modelo de elementos finitos del hombro: comparación de los esfuerzos mecánicos de un hombro sano y un hombro con síndrome del manguito rotador. Rev. Investig. 8 (1), 42–50. doi:10.29097/2011-639X.6
Adams, C. R., Baldwin, M. A., Laz, P. J., Rullkoetter, P. J., and Langenderfer, J. E. (2007). Effects of rotator cuff tears on muscle moment arms: a computational study. J. biomechanics 40 (15), 3373–3380. doi:10.1016/j.jbiomech.2007.05.017
Bencardino, J. T., Beltran, J., Rosenberg, Z. S., Rokito, A., Schmahmann, S., Mota, J., et al. (2000). Superior labrum anterior-posterior lesions: diagnosis with MR arthrography of the shoulder. Radiology 214 (1), 267–271. doi:10.1148/radiology.214.1.r00ja22267
Brzóska, R., Milano, G., Randelli, P. S., and Kovačič, L. (2017). 360° around shoulder instability. History, 7–16. doi:10.1007/978-3-662-61074-9
Büchler, P., Ramaniraka, N. A., Rakotomanana, L. R., Iannotti, J. P., and Farron, A. (2002). A finite element model of the shoulder: application to the comparison of normal and osteoarthritic joints. Clin. Biomech. 17 (9-10), 630–639. doi:10.1016/S0268-0033(02)00106-7
Chang, L. R., Anand, P., and Varacallo, M. A. (2023). Anatomy, shoulder and upper limb, glenohumeral joint. StatPearls.
Clavert, P., Zerah, M., Krier, J., Mille, P., Kempf, J. F., and Kahn, J. L. (2006). Finite element analysis of the strain distribution in the humeral head tubercles during abduction: comparison of young and osteoporotic bone. Surg. Radiologic Anat. 28, 581–587. doi:10.1007/s00276-006-0140-x
Correa-Corona, M. I., Urriolagoitia-Sosa, G., Romero-Ángeles, B., Urriolagoitia-Luna, A., Maya-Anaya, D., Suarez-Hernández, M. de la L., et al. (2023). Application of the finite element model using 3D modeling of a human bone system with osteoporosis for biomedical and mechanical analysis. MOJ Appl. Biomech. 7 (1), 14–15. doi:10.15406/mojabb.2023.07.00169
Dhanopia, A., and Bhargava, M. (2017). Finite element analysis of human fractured femur bone implantation with PMMA thermoplastic prosthetic plate. Procedia Eng. 173, 1658–1665. doi:10.1016/j.proeng.2016.12.190
Ellis, B. J., Debski, R. E., Moore, S. M., McMahon, P. J., and Weiss, J. A. (2007). Methodology and sensitivity studies for finite element modeling of the inferior glenohumeral ligament complex. J. biomechanics 40 (3), 603–612. doi:10.1016/j.jbiomech.2006.01.024
Favre, P., Senteler, M., Hipp, J., Scherrer, S., Gerber, C., and Snedeker, J. G. (2012). An integrated model of active glenohumeral stability. J. biomechanics 45 (13), 2248–2255. doi:10.1016/j.jbiomech.2012.06.010
Fitzpatrick, S., Bishop, J. Y., and Cvetanovich, G. L. (2021). SLAP tear diagnosis and management. Manag. Biceps Pathology A Clin. Guide Shoulder Elb., 125–136. doi:10.1007/978-3-030-63019-5_9
Hayes, W. C., Piazza, S. J., and Zysset, P. K. (1991). Biomechanics of fracture risk prediction of the hip and spine by quantitative computed tomography. Radiologic Clin. N. Am. 29 (1), 1–18. doi:10.1016/S0033-8389(22)02675-6
Hernández-Vázquez, R. A., Urriolagoitia-Sosa, G., Marquet-Rivera, R. A., Romero-Angeles, B., Mastache-Miranda, O. A., Vázquez-Feijoo, J. A., et al. (2020). High-biofidelity biomodel generated from three-dimensional imaging (Cone-Beam computed tomography): a methodological proposal. Comput. Math. Methods Med. 2020 (1), 4292501. doi:10.1155/2020/4292501
Jang, S. W., Yoo, Y. S., and Kim, Y. S. (2020). A new method of contact stress measurement for analyzing internal impingement syndrome of the shoulder: potentials and preliminary evaluation. Appl. Sci. 10 (12), 4165. doi:10.3390/app10124165
Kadi, R., Milants, A., and Shahabpour, M. (2017). Shoulder anatomy and normal variants. J. Belg. Soc. Radiology 101 (Suppl. 2), 3. doi:10.5334/jbr-btr.1467
Kanatli, U., Ozturk, B. Y., and Bolukbasi, S. (2010). Anatomical variations of the anterosuperior labrum: prevalence and association with type II superior labrum anterior-posterior (SLAP) lesions. J. shoulder Elb. Surg. 19 (8), 1199–1203. doi:10.1016/j.jse.2010.07.016
Kramme, R., Hoffmann, K. P., and Pozos, R. S. (2011). Springer handbook of medical technology. Springer Sci. and Bus. Media. doi:10.1007/978-3-540-74658-4_16
Lohfeld, S., Barron, V., and McHugh, P. E. (2005). Biomodels of bone: a review. Ann. Biomed. Eng. 33, 1295–1311. doi:10.1007/s10439-005-5873-x
Luo, Z. P., Hsu, H. C., Grabowski, J. J., Morrey, B. F., and An, K. N. (1998). Mechanical environment associated with rotator cuff tears. J. shoulder Elb. Surg. 7 (6), 616–620. doi:10.1016/S1058-2746(98)90010-6
Maldonado, J. A., Puentes, D. A., Quintero, I. D., González-Estrada, O. A., and Villegas, D. F. (2023). Image-based numerical analysis for isolated type II SLAP lesions in shoulder abduction and external rotation. Diagnostics 13 (10), 1819. doi:10.3390/diagnostics13101819
Marquet-Rivera, R. A., Urriolagoitia-Sosa, G., Romero-Ángeles, B., Hernández-Vázquez, R. A., Mastache-Miranda, O. A., Cruz-López, S., et al. (2021). Numerical analysis of the ACL, with sprains of different degrees after trauma. Comput. Math. methods Med. 2021 (1), 2109348. doi:10.1155/2021/2109348
Martinez-Mondragon, M., Urriolagoitia-Sosa, G., Romero-Ángeles, B., Maya-Anaya, D., Martínez-Reyes, J., Gallegos-Funes, F. J., et al. (2022). Numerical analysis of zirconium and titanium implants under the effect of critical masticatory load. Materials 15 (21), 7843. doi:10.3390/ma15217843
Mathew, C. J., and Lintner, D. M. (2018). Superior labral anterior to posterior tear management in athletes. Open Orthop. J. 12, 303–313. doi:10.2174/1874325001812010303
O’Kane, J. W., and Toresdahl, B. G. (2014). The evidenced-based shoulder evaluation. Curr. sports Med. Rep. 13 (5), 307–313. doi:10.1249/JSR.0000000000000090
Saccomanno, M. F., Fodale, M., Capasso, L., Cazzato, G., and Milano, G. (2013). Generalized joint laxity and multidirectional instability of the shoulder. Joints 1 (04), 171–179. doi:10.11138/jts/2013.1.4.171
Sano, H., Wakabayashi, I., and Itoi, E. (2006). Stress distribution in the supraspinatus tendon with partial-thickness tears: an analysis using two-dimensional finite element model. J. shoulder Elb. Surg. 15 (1), 100–105. doi:10.1016/j.jse.2005.04.003
Seki, N., Itoi, E., Shibuya, Y., Wakabayashi, I., Sano, H., Sashi, R., et al. (2008). Mechanical environment of the supraspinatus tendon: three-dimensional finite element model analysis. J. Orthop. Sci. 13, 348–353. doi:10.1007/s00776-008-1240-8
Sernik, R. A., Vidal Leão, R., Luis Bizetto, E., Sanford Damasceno, R., Horvat, N., and Guido Cerri, G. (2019). Thickening of the axillary recess capsule on ultrasound correlates with magnetic resonance imaging signs of adhesive capsulitis. Ultrasound 27 (3), 183–190. doi:10.1177/1742271x19840063
Serrato-Pedrosa, J. A., Urriolagoitia-Sosa, G., Romero-Ángeles, B., Carrasco-Hernández, F., Gallegos-Funes, F. J., Trejo-Enriquez, A., et al. (2024). Numerical analysis of the plantar pressure points during the stance phases for the design of a 3D-printable patient-specific insole. Prosthesis 6 (3), 429–456. doi:10.3390/prosthesis6030032
Suarez-Hernandez, M. de la L., Urriolagoitia-Sosa, G., Romero-Ángeles, B., Estrada-Salazar, K. M., Guereca-Ibarra, J. R., Gomez-Niebla, J. A., et al. (2024). “Analysis developed from an extremely complex system of the human shoulder based on finite element method (FEM),” in Engineering design applications VI: structures, materials and processes (Cham: Springer Nature Switzerland), 255–269. doi:10.1007/978-3-031-60920-6_17
Suarez-Hernandez, M. L. (2023). Determinación por medio del Método de Elemento Finito de posibles lesiones de labrum en el hombro. M. Sc. Thesis Mexico: CDMX: Instituto Politécnico Nacional, SEPI ESIME Zacatenco.
Trejo-Enriquez, A., Urriolagoitia-Sosa, G., Romero-Ángeles, B., García-Laguna, M. Á., Guzmán-Baeza, M., Martínez-Reyes, J., et al. (2023). Numerical evaluation using the finite element method on frontal craniocervical impact directed at intervertebral disc wear. Appl. Sci. 13 (21), 11989. doi:10.3390/app132111989
Webb, J. D., Blemker, S. S., and Delp, S. L. (2014). 3D finite element models of shoulder muscles for computing lines of actions and moment arms. Comput. methods biomechanics Biomed. Eng. 17 (8), 829–837. doi:10.1080/10255842.2012.719605
Yeh, L., Kwak, S., Kim, Y. S., Chou, D. S., Muhle, C., Skaf, A., et al. (1998). Evaluation of articular cartilage thickness of the humeral head and the glenoid fossa by MR arthrography: anatomic correlation in cadavers. Skelet. Radiol. 27, 500–504. doi:10.1007/s002560050427
Yeh, M. L., Lintner, D., and Luo, Z. P. (2005). Stress distribution in the superior labrum during throwing motion. Am. J. sports Med. 33 (3), 395–401. doi:10.1177/0363546504268404
Keywords: finite element method, numerical analysis, SLAP, biomodel, tomography
Citation: Suarez-Hernandez MdlL, Urriolagoitia-Sosa G, Romero-Ángeles B, Carrasco-Hernández F, Martínez-Reyes J, Gallegos-Funes FJ, Velázquez-Lozada E, Correa-Corona MI, German-Carcaño JM and Urriolagoitia-Calderón GM (2025) Numerical evaluation for SLAP type II tear in shoulder abduction applying the finite element method. Front. Mech. Eng. 11:1505969. doi: 10.3389/fmech.2025.1505969
Received: 04 October 2024; Accepted: 10 March 2025;
Published: 04 April 2025.
Edited by:
Xin Jin, Huazhong University of Science and Technology, ChinaReviewed by:
Yoshitaka Nakanishi, Kumamoto University, JapanCopyright © 2025 Suarez-Hernandez, Urriolagoitia-Sosa, Romero-Ángeles, Carrasco-Hernández, Martínez-Reyes, Gallegos-Funes, Velázquez-Lozada, Correa-Corona, German-Carcaño and Urriolagoitia-Calderón. This is an open-access article distributed under the terms of the Creative Commons Attribution License (CC BY). The use, distribution or reproduction in other forums is permitted, provided the original author(s) and the copyright owner(s) are credited and that the original publication in this journal is cited, in accordance with accepted academic practice. No use, distribution or reproduction is permitted which does not comply with these terms.
*Correspondence: Maria de la Luz Suarez-Hernandez, bHV6c3VhcmV6Mzk4QGdtYWlsLmNvbQ==; Guillermo Urriolagoitia-Sosa, Z3VpdXJyaUBob3RtYWlsLmNvbQ==; Beatriz Romero-Ángeles, cm9tZXJvYmVhdHJpejk3QGhvdG1haWwuY29t
Disclaimer: All claims expressed in this article are solely those of the authors and do not necessarily represent those of their affiliated organizations, or those of the publisher, the editors and the reviewers. Any product that may be evaluated in this article or claim that may be made by its manufacturer is not guaranteed or endorsed by the publisher.
Research integrity at Frontiers
Learn more about the work of our research integrity team to safeguard the quality of each article we publish.