- 1Department of Mechanical and System Engineering, Kyoto Institute of Technology, Kyoto, Japan
- 2Department of Mechanical Engineering and Science, Graduate School of Engineering, Kyoto University, Kyoto, Japan
In boundary lubrication, adsorbed molecular films formed by lubricant additives on the metal surfaces of sliding parts effectively reduce friction and wear. A method is presented for simultaneously measuring friction and the gap at a metal–lubricant interface under boundary lubrication conditions using atomic force microscopy. In this method, line-and-space patterns are microfabricated in Cu films on Si substrates, and the gap is evaluated from the step height change when scanning in base oil and in base oil with an additive. Neutron reflectometry showed that whereas both stearic acid and stearyl alcohol formed molecular film about 2 nm thick on Cu film in a static state, the gap increased only with stearic acid due to maintaining a molecular film on the Cu film. This demonstrates the feasibility of the proposed method as means for visualizing the gap and shows that there is a difference between the two additives in their film-forming ability in a static state and their durability against friction. The proposed method for simultaneously measuring friction and the gap at a metal–lubricant interface is thus an effective way to investigate the tribological performance of additives under boundary lubrication conditions.
1 Introduction
Additives in lubricants generally improve the tribological properties of machine sliding parts by acting on metal surfaces (Yu et al., 2023; Cyriac et al., 2024; Nozue et al., 2024; Shu et al., 2024; Xu et al., 2024). Organic lubricating additives suppress friction and wear by simply adsorbing physically or chemically on the metal surface and forming a molecular film without complex chemical reaction due to temperature, pressure, or frictional shear force. This molecular film protects the surface from damage and reduces friction.
There are many types of organic lubricating additives such as fatty acids, amines, alcohols, and polymers (Tang and Li, 2014; Fry et al., 2020; Cyriac et al., 2021), as well as additives with improved adsorption properties (Desanker et al., 2017; Desanker et al., 2018; He et al., 2018; Yamashita et al., 2022; Hou et al., 2023; Oshio et al., 2023). Although the thickness of the adsorbed molecular film is generally on the nanometer scale, the film often dramatically changes the tribological properties of the sliding parts. Several studies have shown that the frictional properties of the film depend on the molecular structure of the additive, such as its alkyl chain length and the type and number of polar groups (Ries and Cook, 1954; Block and Simms, 1967; Jahanmir, 1985; Tatsumi et al., 2020; Song et al., 2024). These parameters are strongly related to the thickness, stiffness, durability against friction, or stability against temperature rise of the adsorbed molecular film and affect the friction and wear properties.
To understand the tribological properties exhibited by additives, it is necessary to understand the state of the adsorbed film and base oil at the narrow gap between two surfaces in friction. Many studies focused on understanding the mechanism of low friction by simultaneously measuring friction and gap at the metal–lubricant interface. Generally, the measurements have been carried out under elastohydrodynamic lubrication using optical interferometry (Spikes, 1996; Ratoi et al., 2000). However, to accurately measure the gap using optical interferometry, the glass disk must be coated with thin films, such as chromium and silica, and the measurement must be performed under rolling lubrication conditions with a low slip rate to avoid damaging the coating film. A completely different approach is to use a surface force apparatus, which uses optical interferometry for gap measurement (Yamada et al., 2015). This apparatus uses semi-cylindrical glass disks arranged at right angles to each other, and the viscoelasticity of the solid-liquid interface in a narrow gap is investigated in detail by vibrating one of the disks while moving it closer to the other disks. This approach is normally limited to glass or mica specimens to ensure surface smoothness, but recent experiments have been performed using smooth specimens coated with thin metal films (Kasuya et al., 2017; Kurihara, 2019; Song et al., 2023). However, the set-up for the measurements is difficult and requires skilled operation.
Boundary lubrication is a severe lubrication condition in which only a thin oil film is maintained between two sliding surfaces, so the role of the adsorbed molecular film formed by the additive is particularly important. However, few studies have attempted to precisely characterize the tribological properties of the films formed by additives while measuring the gap at the metal–lubricant interface under boundary lubrication conditions with high contact pressure. In-situ atomic force microscopy (AFM) observation is now widely used for simultaneous measurement of friction and surface topography in lubricant (Gosvami et al., 2015; Yamashita and Hirayama, 2024). Friction created by AFM probes with sharp tips simulates the single asperity contact of protrusions due to the surface roughness of mechanical sliding parts under boundary lubrication conditions. AFM has been used to evaluate the amount of wear and the reaction rate of anti-wear agents that form a thicker film. However, AFM can measure only the relative change in surface topography, not the absolute thickness of the film. For example, if an adsorbed molecular film is formed over the entire metal surface, not only is it impossible to measure the thickness of the film, but it is also impossible to determine whether a film has actually formed because there is no reference plane corresponding to a height of zero. Campen et al. successfully measured the absolute thickness of island-like adsorbed molecular films formed by physisorption of fatty acids and amines on mica by using dynamic mode AFM observations with low loads to minimize damage to the film structure (Campen et al., 2015a; Campen et al., 2015b). However, contact mode AFM observations showed that the films on the mica were easily removed by friction although a low friction coefficient derived from the film was initially observed. These studies are very interesting in that they evaluated the thickness and durability of the adsorbed molecular films during friction. However, they did not adequately simulate the lubrication conditions of a machine composed mainly of metal, and the adsorption form of the additives was limited to physisorption.
To understand how nanoscale adsorbed films formed by organic lubricant additives reduce friction and wear on metal surfaces under boundary lubrication, it is essential to correlate the durability and load dependence of the film thickness with the tribological properties. Therefore, the aim of this study was to establish a method for measuring the nanometer gap at the metal–lubricant interface with friction under boundary lubrication conditions. There are two challenges in measuring the gap by using AFM. The first is to provide a reference plane invariably corresponding to a height of zero without additive adsorption. The second is to minimize the drift that typically occurs with wide range observation since drift prevents precise height measurement due to the waviness of the observed image. To overcome the first challenge, inactivated Si was used for the reference plane because additives do not easily absorb on it. To overcome the second challenge, a fine metal line pattern was formed to keep the metal plane to be evaluated within a narrow observation range. This was done by microfabricating line-and-space patterns in Cu thin films on Si substrates. The AFM tip was scanned over the pattern in poly-alpha-olefin (PAO) base oil and in PAO containing stearic acid or stearyl alcohol. Since an adsorbed molecular film is not formed on the inactivated Si and the AFM friction measurement is performed under boundary lubrication conditions with almost no oil film between two sliding surfaces, the change in the measured step height from Si to Cu for PAO with/without an additive is equivalent to the gap increase, which reflects the effect of the adsorbed molecular film formed on the Cu film. In this study, friction was measured while measuring the gap between the metal-lubricant interface under boundary lubrication conditions of high contact pressure, which is difficult to achieve conventionally. The proposed method, which utilizes a fine metal line pattern, has the capability to simulate the boundary lubrication of metal sliding parts in machines. Consequently, the relationship between the durability of the adsorbent additive film formed on the Cu surface and the contact pressure dependence of the gap with tribological properties was investigated.
2 Fabrication of line-and-space specimens
The specimen fabrication process is schematically shown in Figure 1. ➀A 4-inch Si (100) wafer was cleaned using a sulfuric acid-hydrogen peroxide mixture and ultrasonic jet water in a cleaning system (KSC-150CBU, Kanamex). ➁The native Si oxide layer on the wafer surface was then removed using a diluted buffered hydrofluoric acid (HF) solution. ➂Next, a photoresist (PMGI-SF5S) with a film thickness of 240 nm was deposited as a 1st layer resist using a spin coater, and the Si wafer was baked on a hot plate at 180°C for 5 min ➃Then, a 2nd layer resist (TDMR-AR80) with a film thickness of 0.95 µm was deposited using a spin coater, and the Si wafer was baked at 90°C for 90 s. ➄The resist layers were exposed using a UV laser in a direct laser writing system (DWL2000, Heidelberg Instruments), thereby creating a 3 µm line-and-space pattern. The Si wafer was then baked on a hot plate at 110°C for 90 s. ➅The Si wafer was immersed in 2.38 wt% tetramethyl ammonium hydroxide (TMAH) aqueous solution for 70 s to develop the pattern. Finally, the Si wafer was rinsed with pure water for 60 s and dried with N2 blow. All the processes described above were carried out in a clean room with yellow light.
➆Next, a vacuum evaporation system (RD-1400, SANVAC) was used to deposit a 2 nm Cr adhesion layer and a 15 nm Cu film on top of it. The deposition rates for both metals were adjusted to be approximately 0.03 nm/s. The Si wafer was stored in a vacuum desiccator until friction measurements to prevent excessive oxidation. Before friction measurement, the wafer was cut into small chips, which were immersed in a 1-methyl-2-pyrrolidinone (NMP) solution at room temperature for 60 min to lift-off the resists and then immersed in another NMP solution heated on a hot plate at 60°C for 60 min to remove the resists more thoroughly. Finally, the chips were ultrasonically cleaned for 10 s in NMP, isopropanol, and ultrapure water and then dried with N2 blow.
➇To completely remove the photoresists, the chips were immersed in a 1% aqueous solution of TMAH for 1 min and rinsed in ultrapure water for 1 min ➈The oxide film on the chips, which re-formed during the fabrication process, was again removed by rinsing with HF specially developed to minimize damaging the Cu film (Pure Etch ZE202, Hayashi Pure Chemical) for 1 min, rinsed in ultrapure water and isopropanol, and then dried with N2. As the adsorption of lubricant additives on Cu can vary with the degree of oxidation, the native Cu oxide layer was etched by immersion in glacial acetic acid for 1 min and then dried directly in N2 to improve the reproducibility of the experiment (Chavez and Hess, 2001).
3 Experiments
3.1 Neutron reflectometry
The structure of the adsorbed additive film formed on the Cu surface in a static state was evaluated by neutron reflectometry. Since hydrogen and deuterium are clearly distinguishable in neutron reflectometry, the thickness and film density of the adsorbed additive film can be evaluated by converting the hydrogen atoms in the additive to deuterium atoms (Hirayama et al., 2012; Hirayama and Yamashita, 2020). This was done using a lubricant with deuterated stearic acid (C17D35COOH) or deuterated stearyl alcohol (C18D37OH) with a 3 mM concentration, which corresponds to approximately 0.1 wt% in terms of the non-deuterated additive in PAO (30 mm2/s@40°C, Idemitsu Kosan). A mirror-polished Si block (50 × 50 mm, t10 mm) was prepared, and a Cu film was deposited on its surface using an ion beam sputtering system (KUR-IBS) to a 30 nm thickness (Hino et al., 2015).
Neutron reflectivity measurements were performed using the SOFIA time-of-flight neutron reflectometer in Beamline 16 at the Materials and Life Science Experimental Facility of the Japan Proton Accelerator Research Complex (Yamada et al., 2011; Mitamura et al., 2013). Figure 2 schematically illustrates the neutron reflectometry measurement. The theoretical value of the scattering length density (SLD), which is the coherent nuclear scattering length multiplied by the number density of the constituent atoms, is also shown in the figure. First, a Si block with a Cu film was placed in a holder, and the neutron reflectivity was measured while the Cu surface was immersed in PAO base oil. The base oil was then replaced with PAO containing deuterated stearic acid or deuterated stearyl alcohol. The reflectivity was measured again after 12 h at room temperature. The thickness of the Cu film and the thickness and film density of the adsorbed molecular film formed by each additive were evaluated by fitting to the reflectivity profiles obtained from each measurement using analysis software (Nelson, 2006).
3.2 AFM observations
AFM observations were first carried out to check that the proposed line-and-space pattern had formed correctly in the Cu film. The observations were carried out using an AFM (SPM-9700HT, Shimadzu) in dynamic mode in air. The maximum operating range of this AFM is 10 μm in the XY plane and 1 μm in the Z direction. The AFM exhibits a resolution of 0.2 nm in the XY plane and 0.01 nm in the Z direction. A point probe type Si cantilever (TAP300AL-G, BudgetSensors; spring constant = 40 N/m, tip radius <10 nm) was used.
A schematic illustration of the simultaneous measurement of friction and gap at the metal–lubricant interface using a specimen with a Cu line-and-space pattern is shown in Figure 3. A sphere-probe-type cantilever made of Si (SD-Sphere-CONT-L, NANOSENSORS), which typically has a 2 µm tip radius, was used for this contact mode AFM observation. Before the experiment, the cantilever was rinsed with specially developed HF for 1 min to inactivate its surface. A 3 µm wide Cu line was formed in the center of the 7.5 µm observation area, and observation was carried out in 50 µL PAO base oil. The applied loads were 25, 75, and 125 nN, with the maximum Hertzian contact pressures on the Cu film corresponding to 200, 280, and 330 MPa, respectively. Next, 50 µL of PAO containing 0.2 wt% of stearic acid or stearyl alcohol was supplied to the same substrate. The lubricant was gently stirred with a pipette, and the concentration of the additive in the lubricant on the substrate was adjusted to 0.1 wt%, after which the same measurements were carried out as for PAO base oil. For the simultaneous measurement of friction and gap at the metal–lubricant interface, 15 successive observations were performed at a load of 75 nN. From each obtained image, the average friction coefficients on the Si block and the Cu film and the step height from the Si reference plane to the Cu line were evaluated.
The AFM images were analyzed using Gwyddion open-source software for AFM data analysis (Nečas and Klapetek, 2012). The height distribution in each image was calculated, and the mean step heights from Si to Cu were found by Gaussian fitting to each height distribution peak. The friction coefficient was obtained by dividing the friction force mechanically calculated from the detected cantilever torsion by the total load of the pushing force calculated from the cantilever deflection and the adhesion force detected in the force curve measurement.
4 Results
4.1 Neutron reflectometry
The thickness and density of the adsorbed molecular film on the Cu thin film were evaluated by neutron reflectometry. The reflectivity profiles obtained from the measurements are shown in Figure 4. Scattering vector Qz (x-axis) is expressed as the following Equation 1.
where λ is the wavelength of the neutron beam and θ is the angle of incidence (Mitamura et al., 2013).
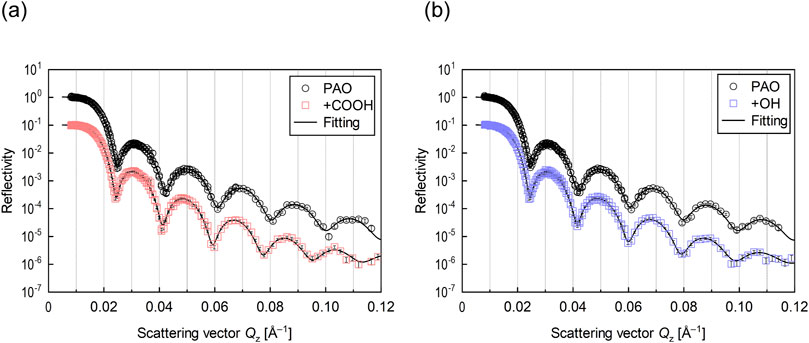
Figure 4. Neutron reflectivity profiles for interface of Cu and lubricant (A) with stearic acid (+COOH) and (B) with stearyl alcohol (+OH).
The SLD profiles obtained by fitting analysis for each reflectivity profile are shown in Figure 5. As the SLD is unique to each material, the film structure can be determined from the depth distribution of the SLD. The thickness of both Cu films formed on the Si blocks was around 30 nm, indicating that the films were successfully deposited at the desired thickness. The thickness of the adsorption film formed on the Cu surface by deuterated stearic acid was 1.9 nm and the film density was 41%. The molecular film formed by deuterated stearyl alcohol had a thickness of 2.2 nm and a film density of 14%. Both the stearic acid and stearyl alcohol has linear chains with 18 carbons, and their molecular length was approximately 2 nm. Therefore, both molecules were in a monolayer state.
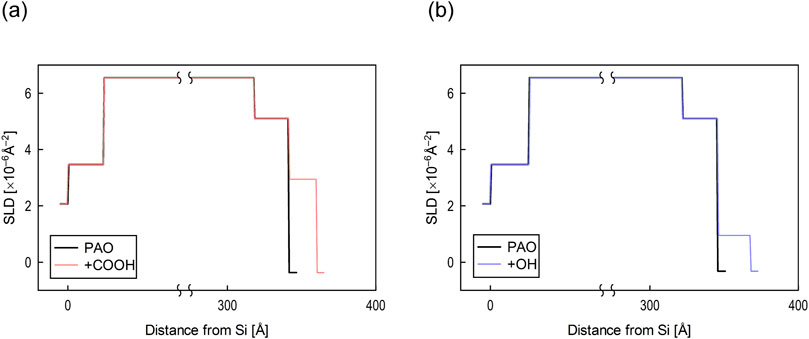
Figure 5. SLD profiles obtained by optimum fitting analysis for (A) stearic acid (+COOH) and (B) stearyl alcohol (+OH).
4.2 Fabricated specimens
The line-and-space patterns observed by optical microscopy and dynamic mode AFM in air with a point probe cantilever are shown in Figure 6. These images show that there was no photoresist or metal residue on the specimen surface and that the target line-and-space pattern was successfully fabricated.
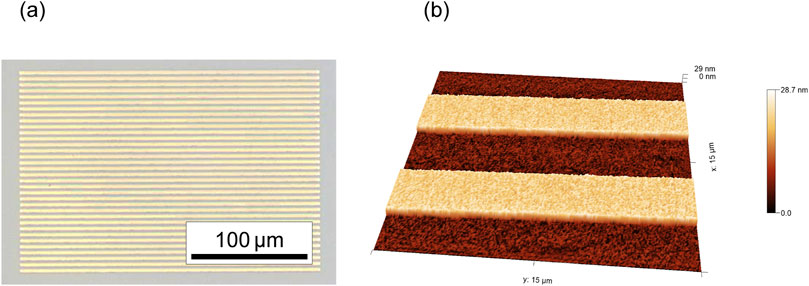
Figure 6. Line-and-space patterns observed by (A) optical microscopy and (B) dynamic mode AFM in air.
Studies on Si/Si and Si/SiO2 friction measured by AFM have been mostly carried out in air with controlled humidity and in water and have shown that Si-based materials are subject to abnormal wear due to interfacial reactions (Chen et al., 2013; Zhang et al., 2017; Zhang et al., 2018). Although this has not been demonstrated for non-polar solvents such as PAO, the moisture in lubricants may affect wear. In this study, the ambient atmosphere around the lubricant was not controlled, and the moisture content in the lubricant was not monitored. As a result, wear with an average depth of about 0.4 nm was rarely observed in the friction area, but data from such cases were not used for evaluation in subsequent experiments. Even if there was sub-nanometer wear that became invisible in the image processing phase, its depth was small compared with the molecular size of the additive and therefore had little effect on the step measurement.
4.3 Load dependence of gap with use of additives
Typical examples of AFM image and height distributions obtained for PAO base oil are shown in Figures 7A, B, respectively. The results of Gaussian fitting to the Si and Cu height distributions in the observed images are shown in Figures 7C, D, respectively. The distances between the peaks correspond to the measured thickness of the Cu film since the minimum oil film thickness calculated using the Hamrock–Dowson equation (Hamrock and Dowson, 1977) is usually extremely small (<10 p.m.). The same analysis was applied to all observed images to obtain an average value. The Cu films used in the experiments were around 17 nm thick for PAO base oil. Experiments were conducted using at least five specimens, and observations were made at five locations for each specimen.
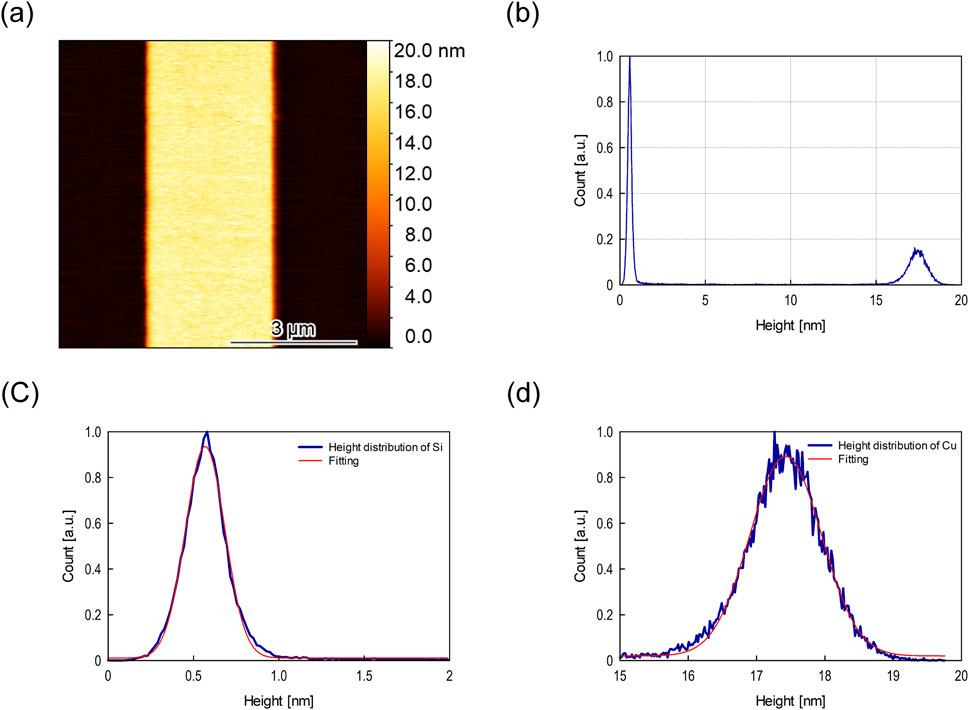
Figure 7. Typical example of (A) AFM image and (B) height distribution in PAO base oil, and Gaussian fittings to height distributions of (C) Si and (D) Cu.
Due to differences in the thickness of the deposited Cu film on each substrate, the average step height for PAO base oil was subtracted from that for PAO with an additive using the same substrate. Since no adsorbed molecular film of an additive was formed on the inactivated Si and since the AFM friction measurements were performed under boundary lubrication conditions with little or no oil film between the two sliding surfaces, the change in the step height from Si to Cu measured for PAO with or without an additive corresponds to the gap generated by the effect of the adsorbed additive film on Cu.
The average gap at the metal–lubricant interface obtained from the observations for each load is shown in Figure 8. Gap formation was clearly observed for PAO containing stearic acid, and the gap was greater at lower loads. At the lowest load the gap was approximately the same as the thickness of the adsorbed stearic acid film observed in neutron reflectometry, whereas at a load of 125 nN the gap was about half the thickness. In contrast, PAO containing stearyl alcohol showed a negative gap for the two lowest loads and only a slight gap increase for the highest load. A negative gap, even if the absolute value is small, indicates that stearyl alcohol may have adsorbed on the Si rather than the Cu or that there may have been a small measurement or statistical error.
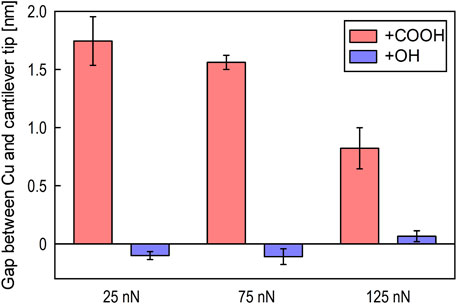
Figure 8. Average gap at metal–lubricant interface for each load for PAO with stearic acid (+COOH) or stearyl alcohol (+OH).
4.4 Simultaneously measurement of friction and gap
Representative friction coefficients for Si and Cu extracted from each 7.5 × 7.5 µm image captured during 15 friction cycles measurement are shown in Figures 9A, B, respectively. The simultaneously measured step heights for PAO containing stearic acid or stearyl alcohol and those for PAO before supplying lubricant for the same specimen are shown in Figure 10. The results for PAO containing stearic acid are shown as three separate results (COOH 1–3) in Figures 9, 10 because the step height showed more variability than those measured for PAO containing stearyl alcohol and PAO base oil.
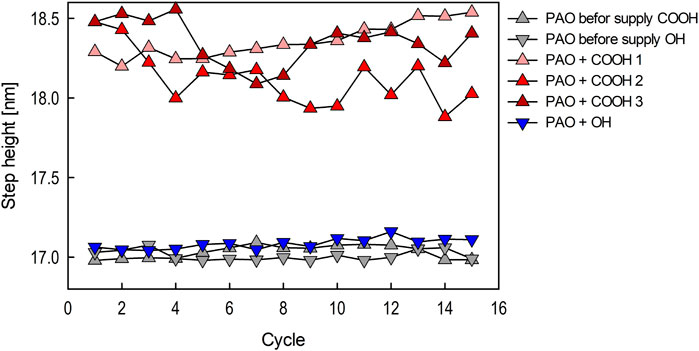
Figure 10. Step heights over 15 friction cycles before and after supplying stearic acid or stearyl alcohol.
The step height measurement for PAO base oil showed that the step height was almost unchanged from the initial value, indicating that wear of the Cu does not occur at a load of 75 nN (Figure 10). It can also be seen that stearyl alcohol had no effect on the step height change on Cu. There was no clear difference in the friction coefficient on Cu for PAO base oil and for PAO containing stearyl alcohol (the differences ranged from 0.17 to 0.15). These results indicate stearyl alcohol has insufficient load-bearing capacity against friction for a 75 nN load.
The friction coefficient for Si in PAO containing stearic acid was slightly lower (around 0.19), whereas for PAO base oil and PAO containing stearyl alcohol it was generally around 0.21. According to Campen et al., there is significant friction reduction for lubricants containing stearic acid on mica despite the absence of film formation on its surface (Campen et al., 2015a; Campen et al., 2015b). In this study, the stearic acid slightly reduced the friction coefficient on Si, but the degree of reduction was smaller. This may be because HF treatment inactivates Si surfaces, which makes adsorption of additives more difficult.
For Cu, in PAO containing stearic acid, the friction coefficient was about 0.07, which is a drastic reduction in friction. When stearic acid was used, the step heights were larger than those for Cu film in PAO base oil, indicating that adsorbed stearic acid film on the Cu film increases the gap at the interface. Since the gap varied by 1–1.5 nm during the 15 friction cycles, which is less than the thickness of the molecular film obtained by neutron reflectivity, the tip of the AFM cantilever is considered to have scanned on or inside the film.
5 Discussion
5.1 Film properties and gap evaluation
Neutron reflectometry showed that both stearic acid and stearyl alcohol formed adsorbed molecular film about 2 nm thick under static conditions, but the AFM measurements showed no significant step height change on the Cu film when stearyl alcohol was used, even with the lowest load used (Figure 8). Neutron reflectometry revealed that the film density with stearyl alcohol was 14%, which is clearly lower than that of stearic acid (41%). In general, adsorbed molecular film is stronger at higher film densities due to larger interactions between the alkyl chains of the adsorbed additives (Studt, 1981; Hironaka, 1988). Films with lower densities may have a larger contact area with the AFM cantilever probe, resulting in a stronger interaction and thus a higher shear force per unit area and higher friction. It has been suggested that sliding between loosely packed molecular films may cause molecular deformation and greater energy dissipation (Lee et al., 2000). In this study, there were no differences in the friction coefficient and step height for Cu with or without stearyl alcohol. Since the molecular film formed by stearyl alcohol has quite low density, it can be concluded that either the load-bearing capacity was low and could not bear the cantilever tip or that was removed from the Cu surface without any positive or negative effect on friction.
As the results of neutron reflectometry show, the density of the film formed by the stearic acid was relatively high (41%). Furthermore, fatty acids have a high adsorption capacity for Cu, forming a high density molecular film with chemical bonds (Greenhill, 1949). Many studies using steel as a sliding material have shown that fatty acids exhibit a lower friction coefficient than alcohols (Jahanmir, 1985; Studt, 1989; Simic and Kalin, 2013) and that this tendency appears to be basically the same for Cu. The carboxy groups of fatty acids include –OH and C=O groups. Stearic acid can be adsorbed on Cu via–OH and C=O groups in a mono- or bidentate mode, and bidentate adsorption increases after the friction-induced tribochemical reaction (Hu et al., 1992; Fischer et al., 1997). Therefore, the adsorption capacity of fatty acids is stronger than that of alcohols, which in principle can only be adsorbed in monodentate mode. For methyl and ethyl esters of fatty acids, the adsorption capacity is reported to be small compared with that of the original fatty acid (Moore, 1951; Studt, 1981; Liascukiene et al., 2012). It is important to have both C=O and –OH groups, and in this study, a stable chemically adsorbed film was formed on Cu, providing substantial friction reduction and a gap increase even under severe friction conditions beneath the AFM cantilever tip.
Figure 8 shows that the durability of the molecular film formed by stearic acid is load dependent. At a load of 25 nN, the change in step height for PAO with/without stearic acid was about 1.7 nm, which is comparable, as revealed by neutron reflectometry, to the film thickness in a static state. This indicates that the molecular film is strong enough to bear an average contact pressure of 200 MPa, in accordance with the Hertzian contact between the Si cantilever and the Cu film, with a contact diameter of 16 nm. At a load of 125 nN, the gap decreased to about 1 nm. That the gap became smaller than the thickness of the molecular film formed in a static state suggests that the film may have been compressed or tilted or that the alkyl chains may have been bent (Salmeron, 2001). However, the gap was still well maintained, indicating that the molecular film formed by stearic acid had a high load-bearing capacity.
In the friction measurement at a load of 75 nN using stearic acid, the friction coefficient remained stable regardless of the number of friction cycles (Figure 9B), and the gap did not decrease due to, for example, removal of the adsorbed molecular film by friction. However, the step height fluctuated around 18–18.5 nm during measurement. Since the step height for PAO base oil exhibited a constant value of around 17 nm (Figure 10), the gap fluctuated significantly with the formation of the adsorbed stearic acid film. Although this measurement technique cannot determine the molecular conformation of the adsorbed stearic acid film during friction, the results indicate that the state of the film may change from moment to moment. The stearic acid adsorbed molecular film on Cu changed to a softer metallic soap state due to friction (Hirayama et al., 2017), suggesting that the cantilever can scan on and within the film.
5.2 Effectiveness of proposed method
In the experiments with stearyl alcohol, the neutron reflectometry confirmed film formation under static state conditions, whereas AFM observation simulating single asperity contact did not show any gap increase due to film formation. This indicates that there is a clear difference between film formation ability under static state conditions and maintaining the film under friction conditions. Therefore, to investigate the tribological performance of additives under boundary lubrication conditions, it is highly effective to simultaneously measure the friction and gap at the metal–lubricant interface, as proposed here, in addition to using such methods as neutron reflectometry and quartz crystal microbalance to evaluate adsorption on the metal surface.
Adsorption of an additive can occur to a small extent as long as there is no repulsive force between the substrate and additive. That there was little friction reduction on Si when additives were supplied (Figure 9A) means that weakly and sparsely adsorbed additives are easily removed by applied friction with virtually no effect on the frictional properties. The adsorption of fatty acids, alcohols, and amines on active SiO2 surfaces has been reported in experiments and molecular simulations (Fry et al., 2020; Shi et al., 2020). During the feasibility phase of this study, measurements of friction in lubricant containing stearic acid were performed using Si without HF treatment, and friction reduction was obvious.
In this study, the stearic acid was successfully prevented from adsorbing on Si by removing the Si native oxide film and hydrogen termination by treatment with a special HF, which does not damage Cu, immediately before starting the experiment (as described in Section 2). This indicates that the adsorption of the additive can be suppressed by using inactivated Si. A slight decrease in the friction coefficient was still observed for Si in PAO containing stearic acid (Figure 9A). However, the degree of reduction was much smaller than that for Cu, and no difference in surface topography was observed, suggesting that the amount of adsorption was small.
The feasibility of the proposed method was evaluated using Cu, which can maintain a line-and-space pattern without dissolving or peeling during HF treatment and is active to the adsorption of additives. In future experiments with other kind of metals, such as iron, it will be necessary to improve the selection of the base material, e.g., by using diamond-like carbon coated Si, which is inactive to additive adsorption (Simič et al., 2014). Future work includes a more detailed evaluation of the contact pressure dependence of the gap at the metal–lubricant interface formed by adsorbed molecular films created by various type of additives on metal films and visualization of the formation of tribofilms and metallic soaps under friction conditions. Simultaneous measurement of friction and gap under various tribological conditions provides a deep understanding of additive properties, facilitating the selection and development of additives that strongly adsorb to metals, resulting in low friction and wear. This method is useful for parameter studies in the field of tribology and contributes to improving the energy efficiency of machines.
6 Conclusion
In the proposed method for simultaneously measuring the friction and gap at the metal–lubricant interface of adsorbed molecular films formed on metal surfaces under boundary lubrication conditions using AFM, line-and-space patterns are microfabricated in Cu film on a Si substrate. The gap is evaluated on the basis of the step height change when scanning in base oil and in lubricant with an additive. Neutron reflectometry showed film formation with both stearic acid and stearyl alcohol as additives, but the film density of stearyl alcohol was less than half that formed by stearic acid. The proposed method using AFM confirmed that the molecular film on the Cu film is maintained only with stearic acid and the gap increases under boundary lubrication conditions. This indicates that the adsorbed molecular film of stearyl alcohol, which has low film strength due to low film density and weak adsorption, is easily removed under boundary lubrication conditions and does not contribute to friction reduction. These results are reasonable and support the validity of the evaluation by the proposed method. Therefore, the proposed visualization technique is an effective analytical method for investigating the durability of adsorbed molecular films and the contact pressure dependence of gap at the metal–lubricant interface.
Data availability statement
The raw data supporting the conclusions of this article will be made available by the authors, without undue reservation.
Author contributions
NY: Conceptualization, Formal Analysis, Funding acquisition, Investigation, Methodology, Validation, Visualization, Writing–original draft. TH: Methodology, Project administration, Supervision, Writing–review and editing.
Funding
The author(s) declare that financial support was received for the research, authorship, and/or publication of this article. This study was supported by Transmission Research Association for Mobility Innovation (TRAMI) and NSK Foundation for Advancement of Mechatronics. This work was also supported by JSPS KAKENHI Grant Number 24K07288.
Acknowledgments
The neutron experiment at the Materials and Life Science Experimental Facility of the J-PARC was performed under a user program (Proposal No. 2023MP-S001). This work was supported by Kyoto University Nanotechnology Hub in “Advanced Research Infrastructure for Materials and Nanotechnology Project” sponsored by the Ministry of Education, Culture, Sports, Science and Technology (MEXT), Japan. We express our sincere gratitude to Professor Masahiro Hino at the Institute for Integrated Radiation and Nuclear Science, Kyoto University, for kindly preparing the Cu films used in the neutron reflectometry experiments (Proposal No. R6114).
Conflict of interest
The authors declare that the research was conducted in the absence of any commercial or financial relationships that could be construed as a potential conflict of interest.
Publisher’s note
All claims expressed in this article are solely those of the authors and do not necessarily represent those of their affiliated organizations, or those of the publisher, the editors and the reviewers. Any product that may be evaluated in this article, or claim that may be made by its manufacturer, is not guaranteed or endorsed by the publisher.
References
Block, A., and Simms, B. B. (1967). Desorption and exchange of adsorbed octadecylamine and stearic acid on steel and glass. J. Colloid Interface Sci. 25, 514–518. doi:10.1016/0021-9797(67)90062-8
Campen, S., Green, J. H., Lamb, G. D., and Spikes, H. A. (2015a). In situ study of model organic friction modifiers using liquid cell AFM; saturated and mono-unsaturated carboxylic acids. Tribol. Lett. 57, 18. doi:10.1007/s11249-015-0465-x
Campen, S., Green, J. H., Lamb, G. D., and Spikes, H. A. (2015b). In situ study of model organic friction modifiers using liquid cell AFM: self-assembly of octadecylamine. Tribol. Lett. 58, 39. doi:10.1007/s11249-015-0514-5
Chavez, K. L., and Hess, D. W. (2001). A novel method of etching copper oxide using acetic acid. J. Electrochem. Soc. 148, G640. doi:10.1149/1.1409400
Chen, L., Kim, S. H., Wang, X., and Qian, L. (2013). Running-in process of Si-SiOx/SiO2 pair at nanoscale—sharp drops in friction and wear rate during initial cycles. Friction 1, 81–91. doi:10.1007/s40544-013-0007-1
Cyriac, F., Tee, X. Y., and Chow, P. S. (2024). Tribological performance of polymeric friction modifiers under sliding rolling contact condition. Lubr. Sci. 36, 119–134. doi:10.1002/ls.1678
Cyriac, F., Tee, X. Y., Poornachary, S. K., and Chow, P. S. (2021). Influence of structural factors on the tribological performance of organic friction modifiers. Friction 9, 380–400. doi:10.1007/s40544-020-0385-0
Desanker, M., He, X., Lu, J., Johnson, B. A., Liu, Z., Delferro, M., et al. (2018). High-performance heterocyclic friction modifiers for boundary lubrication. Tribol. Lett. 66, 50. doi:10.1007/s11249-018-0996-z
Desanker, M., He, X., Lu, J., Liu, P., Pickens, D. B., Delferro, M., et al. (2017). Alkyl-cyclens as effective sulfur- and phosphorus-free friction modifiers for boundary lubrication. ACS Appl. Mater. Interfaces 9, 9118–9125. doi:10.1021/acsami.6b15608
Fischer, D. A., Hu, Z. S., and Hsu, S. M. (1997). Tribochemical and thermochemical reactions of stearicacid on copper surfaces in air as measured by ultra-soft X-rayabsorption spectroscopy. Tribol. Lett. 3, 35–40. doi:10.1023/A:1019109407863
Fry, B. M., Moody, G., Spikes, H. A., and Wong, J. S. S. (2020). Adsorption of organic friction modifier additives. Langmuir 36, 1147–1155. doi:10.1021/acs.langmuir.9b03668
Gosvami, N. N., Bares, J. A., Mangolini, F., Konicek, A. R., Yablon, D. G., and Carpick, R. W. (2015). Mechanisms of antiwear tribofilm growth revealed in situ by single-asperity sliding contacts. Science 348, 102–106. doi:10.1126/science.1258788
Greenhill, E. B. (1949). The adsorption of long chain polar compounds from solution on metal surfaces. Trans. Faraday Soc. 45, 625–631. doi:10.1039/TF9494500625
Hamrock, B. J., and Dowson, D. (1977). Isothermal elastohydrodynamic lubrication of point contacts: Part III—fully flooded results. J. Lubr. Technol. 99, 264–275. doi:10.1115/1.3453074
He, X., Lu, J., Desanker, M., Invergo, A. M., Lohr, T. L., Ren, N., et al. (2018). Boundary lubrication mechanisms for high-performance friction modifiers. ACS Appl. Mater. Interfaces 10, 40203–40211. doi:10.1021/acsami.8b11075
Hino, M., Oda, T., Kitaguchi, M., Yamada, N. L., Tasaki, S., and Kawabata, Y. (2015). The ion beam sputtering facility at KURRI: coatings for advanced neutron optical devices. Nucl. Instrum. Methods Phys. Res. Sect. A Accel. Spectrom. Detect. Assoc. Equip. 797, 265–270. doi:10.1016/j.nima.2015.06.046
Hirayama, T., Kawamura, R., Fujino, K., Matsuoka, T., Komiya, H., and Onishi, H. (2017). Cross-sectional imaging of boundary lubrication layer formed by fatty acid by means of frequency-modulation atomic force microscopy. Langmuir 33, 10492–10500. doi:10.1021/acs.langmuir.7b02528
Hirayama, T., Torii, T., Konishi, Y., Maeda, M., Matsuoka, T., Inoue, K., et al. (2012). Thickness and density of adsorbed additive layer on metal surface in lubricant by neutron reflectometry. Tribol. Int. 54, 100–105. doi:10.1016/j.triboint.2012.04.012
Hirayama, T., and Yamashita, N. (2020). Combined use of neutron reflectometry and frequency-modulation atomic force microscopy for deeper understanding of tribology. Jpn. J. Appl. Phys. 59, SN0803. doi:10.35848/1347-4065/ab9c43
Hironaka, S. (1988). Friction properties of C18-fatty acids. J. Jpn. Petroleum Inst. 31, 216–220. doi:10.1627/jpi1958.31.216
Hou, J., Tsukamoto, M., Hor, S., Chen, X., Yang, J., Zhang, H., et al. (2023). Molecules with a TEMPO-based head group as high-performance organic friction modifiers. Friction 11, 316–332. doi:10.1007/s40544-022-0610-0
Hu, Z.-S., Hsu, S. M., and Wang, P. S. (1992). Tribochemical and thermochemical reactions of stearic acid on copper surfaces studied by infrared microspectroscopy. Tribol. Trans. 35, 189–193. doi:10.1080/10402009208982108
Jahanmir, S. (1985). Chain length effects in boundary lubrication. Wear 102, 331–349. doi:10.1016/0043-1648(85)90176-0
Kasuya, M., Tomita, K., Hino, M., Mizukami, M., Mori, H., Kajita, S., et al. (2017). Nanotribological characterization of lubricants between smooth iron surfaces. Langmuir 33, 3941–3948. doi:10.1021/acs.langmuir.7b00148
Kurihara, K. (2019). Surface forces measurement for materials science. Pure Appl. Chem. 91, 707–716. doi:10.1515/pac-2019-0101
Lee, S., Shon, Y.-S., Colorado, R., Guenard, R. L., Lee, T. R., and Perry, S. S. (2000). The influence of packing densities and surface order on the frictional properties of alkanethiol self-assembled monolayers (SAMs) on gold: a comparison of SAMs derived from normal and spiroalkanedithiols. Langmuir 16, 2220–2224. doi:10.1021/la9909345
Liascukiene, I., Aissaoui, N., Asadauskas, S. J., Landoulsi, J., and Lambert, J.-F. (2012). Ordered nanostructures on a hydroxylated aluminum surface through the self-assembly of fatty acids. Langmuir 28, 5116–5124. doi:10.1021/la2051542
Mitamura, K., Yamada, N. L., Sagehashi, H., Torikai, N., Arita, H., Terada, M., et al. (2013). Novel neutron reflectometer SOFIA at J-PARC/MLF for in-situ soft-interface characterization. Polym. J. 45, 100–108. doi:10.1038/pj.2012.156
Moore, A. C. (1951). The adsorption of lubricant films: a study by radioactive tracers. Br. J. Appl. Phys. 2, 54–56. doi:10.1088/0508-3443/2/S1/320
Nečas, D., and Klapetek, P. (2012). Gwyddion: an open-source software for SPM data analysis. Open Phys. 10, 181–188. doi:10.2478/s11534-011-0096-2
Nelson, A. (2006). Co-refinement of multiple-contrast neutron/X-ray reflectivity data using MOTOFIT. J. Appl. Cryst. 39, 273–276. doi:10.1107/S0021889806005073
Nozue, T., Itoh, S., Okubo, N., Fukuzawa, K., Zhang, H., and Azuma, N. (2024). Temperature dependence of viscoelasticity of lubricating oil with adsorptive polymer additives sheared in nanogaps. Tribol. Lett. 72, 83. doi:10.1007/s11249-024-01884-y
Oshio, T., Minfray, C., Dassenoy, F., Galipaud, J., and Yagishita, K. (2023). Dialkyl phosphonate with carboxylic acid as antiwear additives for ester-base lubricants. Wear 530-531, 205042. doi:10.1016/j.wear.2023.205042
Ratoi, M., Anghel, V., Bovington, C., and Spikes, H. A. (2000). Mechanisms of oiliness additives. Tribol. Int. 33, 241–247. doi:10.1016/S0301-679X(00)00037-2
Ries, H. E., and Cook, H. D. (1954). Monomolecular films of mixtures: I. Stearic acid with isostearic acid and with tri-p-cresyl phosphate. Comparison of components with octadecylphosphonic acid and with tri-o-xenyl phosphate. J. Colloid Sci. 9, 535–546. doi:10.1016/0095-8522(54)90056-2
Salmeron, M. (2001). Generation of defects in model lubricant monolayers and their contribution to energy dissipation in friction. Tribol. Lett. 10, 69–79. doi:10.1023/A:1009026312732
Shi, J., Zhou, Q., Sun, K., Liu, G., and Zhou, F. (2020). Understanding adsorption behaviors of organic friction modifiers on hydroxylated SiO2 (001) surfaces: effects of molecular polarity and temperature. Langmuir 36, 8543–8553. doi:10.1021/acs.langmuir.0c01386
Shu, J., Espejo, C., Kalin, M., and Morina, A. (2024). Tribological performance of fatty acid, acid/amine additive mixture and ionic liquid. Proc. Institution Mech. Eng. Part J J. Eng. Tribol., 13506501241251524. doi:10.1177/13506501241251524
Simic, R., and Kalin, M. (2013). Comparison of alcohol and fatty acid adsorption on hydrogenated DLC coatings studied by AFM and tribological tests. Strojniski Vestn. 59, 707–718. doi:10.5545/sv-jme.2013.1228
Simič, R., Kalin, M., Hirayama, T., Korelis, P., and Geue, T. (2014). Fatty acid adsorption on several DLC coatings studied by neutron reflectometry. Tribol. Lett. 53, 199–206. doi:10.1007/s11249-013-0257-0
Song, W., Campen, S., Shiel, H., Gattinoni, C., Zhang, J., and Wong, J. S. S. (2024). Position of carbonyl group affects tribological performance of ester friction modifiers. ACS Appl. Mater. Interfaces 16, 14252–14262. doi:10.1021/acsami.3c16432
Song, Y., Fukuzawa, K., Hirayama, T., Yamashita, N., Yamada, N. L., Itoh, S., et al. (2023). Effects of polarity of polymers on conformation and lubricating film formation of adsorbed films. Macromolecules 56, 1954–1964. doi:10.1021/acs.macromol.2c02238
Spikes, H. A. (1996). Direct observation of boundary layers. Langmuir 12, 4567–4573. doi:10.1021/la950899b
Studt, P. (1981). The influence of the structure of isomeric octadecanols on their adsorption from solution on iron and their lubricating properties. Wear 70, 329–334. doi:10.1016/0043-1648(81)90353-7
Studt, P. (1989). Boundary lubrication: adsorption of oil additives on steel and ceramic surfaces and its influence on friction and wear. Tribol. Int. 22, 111–119. doi:10.1016/0301-679X(89)90171-0
Tang, Z., and Li, S. (2014). A review of recent developments of friction modifiers for liquid lubricants (2007–present). Curr. Opin. Solid State Mater. Sci. 18, 119–139. doi:10.1016/j.cossms.2014.02.002
Tatsumi, G., Ratoi, M., Shitara, Y., Sakamoto, K., and Mellor, B. G. (2020). Effect of organic friction modifiers on lubrication of PEEK-steel contact. Tribol. Int. 151, 106513. doi:10.1016/j.triboint.2020.106513
Xu, X., Yang, F., Yang, H., Zhao, Y., Sun, X., and Tang, Y. (2024). Preparation and tribological behaviors of sulfur- and phosphorus-free organic friction modifier of amide–ester type. Lubricants 12, 196. doi:10.3390/lubricants12060196
Yamada, N. L., Torikai, N., Mitamura, K., Sagehashi, H., Sato, S., Seto, H., et al. (2011). Design and performance of horizontal-type neutron reflectometer SOFIA at J-PARC/MLF. Eur. Phys. J. Plus 126, 108. doi:10.1140/epjp/i2011-11108-7
Yamada, S., Fujihara, A., Yusa, S., Tanabe, T., and Kurihara, K. (2015). Low-friction adsorbed layers of a triblock copolymer additive in oil-based lubrication. Langmuir 31, 12140–12147. doi:10.1021/acs.langmuir.5b03620
Yamashita, N., and Hirayama, T. (2024). Effect of atmospheric gas on ZDDP tribofilm formation. Tribol. Int. 193, 109400. doi:10.1016/j.triboint.2024.109400
Yamashita, N., Hirayama, T., L. Yamada, N., Shimizu, Y., Oda, K., and Kawamoto, H. (2022). Interfacial structure and tribological property of adsorbed layer formed by dibasic acid ester derivative. Tribol. Online 17, 246–256. doi:10.2474/trol.17.246
Yu, H., Chen, H., Zheng, Z., Qiao, D., Feng, D., Gong, Z., et al. (2023). Effect of functional groups on tribological properties of lubricants and mechanism investigation. Friction 11, 911–926. doi:10.1007/s40544-022-0630-9
Zhang, P., Chen, C., Xiao, C., Chen, L., Jiang, L., and Qian, L. (2018). Effects of surface chemical groups and environmental media on tribochemical running-in behaviors of silicon surface. Tribol. Int. 128, 174–180. doi:10.1016/j.triboint.2018.07.032
Keywords: tribology, lubricant additive, boundary lubrication, visualization technique, atomic force microscopy, neutron reflectometry, microfabrication, line-and-space pattern
Citation: Yamashita N and Hirayama T (2024) A method for simultaneously measuring friction and gap at metal–lubricant interface by combined use of atomic force microscopy and line-and-space patterned metal films. Front. Mech. Eng. 10:1470775. doi: 10.3389/fmech.2024.1470775
Received: 26 July 2024; Accepted: 28 August 2024;
Published: 06 September 2024.
Edited by:
Taisuke Maruyama, NSK Ltd., JapanCopyright © 2024 Yamashita and Hirayama. This is an open-access article distributed under the terms of the Creative Commons Attribution License (CC BY). The use, distribution or reproduction in other forums is permitted, provided the original author(s) and the copyright owner(s) are credited and that the original publication in this journal is cited, in accordance with accepted academic practice. No use, distribution or reproduction is permitted which does not comply with these terms.
*Correspondence: Naoki Yamashita, naoki@kit.ac.jp