- 1Institute for product development and machine elements, Technical University of Darmstadt, Darmstadt, Germany
- 2Department of Production Engineering, Escola Politécnica da Universidade de São Paulo, São Paulo, Brazil
Introduction: The demand to increase reliability and reduce maintenance costs drives the search for new condition monitoring solutions of machines. Bearings are of special interest in this matter as they are main contributors to machine downtime. Therefore, this article investigates the electric behavior of a hydrodynamic journal bearing using impedance measurement.
Methods: For that purpose measurements are taken in boundary lubrication, mixed lubrication and hydrodynamic lubrication of a hydrodynamic journal bearing. In order to interpret the behaviour observed in these measurements, a phenomenological model is developed using the quantitative working space model. The measurement results are also interpreted using analogies to the Stribeck curve as a known tribological model. Additionally, measurements in mixed lubrication are analysed in detail.
Results: These measurements show that the electric behaviour of journal bearings changes significantly within the mixed lubrication regime. This change in behaviour shows three distinct states within mixed lubrication which helps to identify destructive operating conditions. Furthermore, the measurements potentially allow the detection of deformation or damage of the bearing in mixed lubrication.
Discussion: The quantitative analysis of the electric behaviour of journal bearings is possible in the mixed lubrication regime and can be used in future to extract condition and operating information from a system during operation.
1 Introduction
Within the context of energy crises, efficiency increases emerge as a major requirement for most technical systems. Moreover, retrofitting existing systems, especially productive or energy converting plants, is of great interest for industry and research (Jaspert et al., 2021). As existing technical systems of large scale lack the efficiency of recently developed systems, it is a promising approach to integrate additional functionalities into the system to either increase the efficiency by operating in the most efficient conditions or to prevent damage due to unexpected failure of components (Mourtzis et al., 2020). The approach of functional integration as a measure of optimization and increase of reliability drives the research field of condition monitoring (CM). New products as well as existing systems are a focus of research and industry in order to implement CM functions (Surucu et al., 2023). The advancing digitisation of engineering products enables the much needed more reliable and precise solutions for CM (Baszenski et al., 2023). These CM solutions have to provide at least the minimum information necessary to detect and diagnose wear and component failure in the system (Tavner et al., 2008). While some failures can only be detected after their occurrence, others show a time variant behavior (e.g., due to the accumulation of wear) making the prediction of future machine failures especially promising.
Bearings are of special interest for condition monitoring as they show a varying behavior over their lifetime, and their failures are one of the main contributors to machine downtime (Amirat et al., 2009; Dą et al., 2015). Bearing applications comprise a number of characteristics, making them a promising option for implementing various CM functions (Saruhan et al., 2014). Bearings are load carrying elements and, therefore, naturally in the flow of force, which is often function determining and a valuable source of condition information. As bearings run in a variety of operating conditions ranging from high load applications in mixed lubrication to high speed applications in hydrodynamic lubrication, the occurring wear becomes an inherent aspect of their behavior and varies over time and lubrication condition. Additionally, the dimensioning of technical systems is often determined by the dimensioning of bearings and their maintenance cycles. Figure 1 depicts two examples of hydrodynamic bearing segments showing different wear scenarios. The left image is the result of the operation in mixed lubrication, causing significant wear of the gliding surface. This ultimately results in bearing failure and consequently in machine downtime and possibly additional damage to the machine due to increased temperature, wear of the shaft, and vibrations. The right image illustrates the situation of a tilted shaft, visible in the tilted wear pattern. The effects of this wear scenario are similar and have to be avoided in order to avoid a potential bearing or system failure.
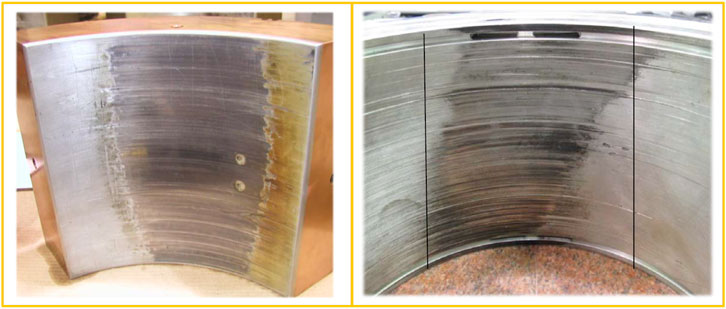
Figure 1. Journal bearing segment; left: wear due to operation in mixed lubrication; right: wear due to tilted shaft during operation (Branagan, 2015)
Regarding the realization of CM functions, the measurement of state variables, such as temperature or pressure of, e.g., gaseous media, within the system’s critical subsystems is a prerequisite (Vorwerk-Handing et al., 2018). A measurement as close as possible to the quantity of interest is called in situ measurement (Kraus et al., 2021). Many publications outline the outstanding suitability of bearings for the monitoring of machines as the behavior of bearings is a steady time variant function of the state of wear. Therefore, this behavior can be used as an indicator for the remaining useful life (Okoh et al., 2014) and the need for maintenance. For instance, Manjunath et al. (2024) describe not only the relevance of monitoring bearing conditions for rolling element bearings, but exploring condition monitoring strategies using the impedance of the bearing and the temperature. Both are quantities that are easy to measure compared to measuring the film thickness directly. Other publications such as Fett et al. (2024) show a practical application of the sensor bearing concept in industrial context based on a case study. The influence of material choice is addressed by Kandeva et al. (2016) and Pastukhov and Timashov, (2023). While the importance of monitoring bearing operating and wear condition as a research field becomes apparent based on these publications, the way how to implement a reliable monitoring strategy remains obscure. Bearings can be used to implement reliable measurement functions without altering the predefined and standardized interfaces of machine elements and thus maintaining the same design as a conventional sole mechanical system. The resulting components are referred to as sensing machine elements (Vorwerk-Handing et al., 2020). According to Vorwerk-Handing et al. (2020) both rolling element bearing and hydrodynamic journal bearings can be classified as sensory utilizable machine elements as both machine elements show a capacitive electrical behavior. This inherent electric behavior can be measured to provide information about the state of the system.
These bearing types are common in mechanical systems and the choice between them is often ambiguous. Both hydrodynamic journal bearings and rolling element bearings can operate in a wide range of applications (Childs, 2019). However, hydrodynamic journal bearings offer distinct advantages over rolling element bearings in terms of assembly space and durability in applications with limited start-stop operations and high rotation rates, such as in aircraft turbines (Mokhtari Molk Abadi, 2020), steam turbines (aus der Wiesche and Joos, 2018; Pennacchi and Tanuma, 2022) and ship drive trains (Weychardt et al., 2008). Applications like the ones mentioned benefit from accurate CM solutions that detect dangerous operating conditions before a bearing failure occurs in order to prevent long downtime and ensure the safety of the system.
Therefore, the objective of this contribution is to generate the basis for a CM approach for journal bearings as central components of safety-critical systems, such as aircraft turbines. However, present condition monitoring approaches for journal bearings focus on the detection of already occurred wear (Mokhtari Molk Abadi, 2020). To this point, the classification of the operating conditions based on the electric properties of journal bearings is limited to the description of hydrodynamic lubrication as done by Prashad (Prashad, 1991; Prashad and Rao, 1994; Prashad, 2006) and Furtmann, (2017). However, the detection of mixed lubrication is not discussed as it is assumed that the metal-to-metal contact would invalidate all models of the capacitive behavior of the bearing. It is therefore assumed that the differentiation between hydrodynamic and mixed lubrication is possible as a binary decision. While this differentiation is of considerable value to avoid a high friction coefficient in the boundary lubrication regime, it lacks any kind of information of the mixed lubrication regime. As many high-load applications are constantly or partly operated in mixed lubrication conditions, this regime is of considerable interest.
As the current state of research does not provide a consistent model describing the electric behavior of journal bearings operated in mixed lubrication, this contribution thrives to develop an explanatory approach of how mixed lubrication would propagate over an increasing relative speed of the contact surfaces and how the electric behavior of the bearing is affected by the varying lubrication conditions. To address the lack of information regarding the mixed lubrication regime, a new, volume-based modeling approach, called quantitative working space model (qWSM), is utilized to describe the lubrication film as a three-dimensional space where the physical domains of mechanics, hydrodynamics, and electrostatics interact.
The qWSM is a system representation based on three-dimensional sub-volumes of the observed systems, called working spaces (WS), and it derives the behavior of the supersystem as a function of connected subsystems or working spaces. The behavior of a working space is described by balancing conservation quantities introduced into the working space, e.g., energy, mass or charge. Additionally, the effects of conservation quantities affecting the state of a working space are described, such as the increasing temperature of the lubricant due to friction energy for the working space “lubricant film” (Schmitt and Kirchner, 2022; Schösser et al., 2022). A schematic view of the bearing arrangement is depicted in Figure 2 while a simple qWSM is depicted in Figure 3.
The principles of the qWSM applied to the journal bearing system help to derive an explanatory approach for the electric behavior observed at a journal bearing test rig while operated in a variety of lubrication conditions, ranging from solid state friction throughout the mixed lubrication regime to a hydrodynamic lubrication state. Two mixed lubrication models are derived from the qWSM which offer alternative explanation approaches for effects observed in measurements as described in section 3. This way, the contribution provides new insights in the electrical characteristics of journal bearings within the mixed lubrication regime.These insights are intended to enable users to develop new condition monitoring solutions based on the electric behavior of hydrodynamic journal bearings focusing on the mixed lubrication regime. The visualisation of the operating conditions in mixed lubrication by means of the impedance can be used to optimise the operating strategies. It further allows the detection of improper operating conditions and thus mitigating damage during these operations. The objective of this contribution can therefore be summed up as: Deriving a new approach to visualize and model the lubrication conditions of hydrodynamic journal bearings.
The following research hypotheses addressing this objective are underlying this research and will be verified throughout this publication.
H1: The qWSM serves as a modeling approach to derive a new model of mixed lubrication in hydrodynamic journal bearing in an electric circuit
H2: The electric behavior of journal bearings can be described with a continuous function over the relative surface speed rather than a binary distinction between ohmic and capacitive behavior
In order to evaluate present research approaches on journal bearing CM, the qWSM, and system modeling, the state of research is discussed in the following section.
2 Materials and methods
In this section, firstly, the state of research about CM and the electrical behavior of journal bearings is presented. Secondly, the modeling approach using the quantitative working space model is explained. Thirdly, the journal bearing test bench used for this contribution is described and modelled. Finally, potential modeling approaches for mixed lubrication are outlined.
2.1 State of research
Recent publications show that CM is an increasingly important topic to optimise maintenance strategies, improve machine availability, and increase the interconnectivity of machines in relation to Industry 4.0 (Fritz et al., 2001; Tavner et al., 2008; Amirat et al., 2009; Goyal et al., 2019; Baszenski et al., 2023; Sanchez-Londono et al., 2023). Bearings are of special interest for CM as their failure results in downtime and often expensive maintenance (Dą et al., 2015; Rolink et al., 2020). Two established solutions for failure detection are temperature monitoring and acoustic monitoring (Fritz et al., 2001; AlShorman et al., 2021; Baszenski et al., 2023). Both methods can detect faults in a bearing, however, information about the remaining useful life (RUL) cannot be obtained easily (AlShorman et al., 2021). Both approaches are usually applied within corrective maintenance strategies, when a bearing fault has already occurred but before it leads to an increasingly critical failure in adjoining components of the system. An alternative strategy for CM in rolling element bearings consists of using the bearing’s impedance as a measurand. The impedance is dependent on the lubrication film thickness and therefore on temperature, bearing load and rotational rate. These state variables can supply information about the bearing’s operating state and condition (Jablonka et al., 2018; Maruyama et al., 2019; Schirra et al., 2019; Schneider et al., 2022; Puchtler et al., 2023). However, this research is limited to rolling element bearings and recent publications do not cover the electric behavior of journal bearings.
Another advantage of measuring the impedance of the bearing is that the measuring takes place in situ of the process that needs to be assessed. In-situ measuring has become increasingly important in recent years with the demand for precise and reliable sensor data (Kraus et al., 2021). Measuring a measurand directly at the point of interest allows the reduction of disturbances and improves data quality (Kraus et al., 2021). Thus, the sensor placement in relation to the process that needs to be measured has to be considered when implementing a measurement strategy (Goyal et al., 2019). However, the majority of existing technical systems have not been designed to provide sufficient, accurate, and reliable information about the system (Vorwerk-Handing, 2021). Moreover, the fitting of additional sensors to machines is often difficult and does not allow the ideal sensor placement. Even in newly designed systems, the available installation space often does not allow the integration of sensors in close proximity to the point of interest (Vorwerk-Handing et al., 2018). The measurement of the bearing impedance does not require the integration of an additional sensor and thus circumvents the problem. In terms of integration complexity, measuring the impedance requires only the insulation of either the bearing’s outer ring or the shaft and an individual contact for each electric potential. Thus the insulation can be challenging as well but potentially allows more flexibility in finding appropriate solutions.
Journal bearings and rolling element bearings generally overlap in their use cases. However, journal bearings have advantages over rolling element bearings especially in applications with limited packaging space, rare stoppages, and high rotational rates due to their higher efficiency and smaller dimensions compared to rolling element bearings. Examples of these use cases are steam turbines, aircraft turbines, ship drive trains, and recently also main bearings of wind turbines (aus der Wiesche and Joos, 2018; Pennacchi and Tanuma, 2022; Weychardt et al., 2008; Mokhtari Molk Abadi, 2020; Rolink et al., 2020; Rolink et al., 2022). In the first three use cases, the failure of the bearing directly impacts the safety of the steam turbine, aircraft or shipping vessel and thus CM equipment is important. In the latter case, the failure of a wind turbine main bearing may not pose a threat to human life but causes long downtime and financial losses (Dą et al., 2015; Peng et al., 2023). Additionally, information about the lubrication condition of journal bearings can be obtained by the impedance measurement and thus allows the operator to detect and avoid wear-intensive and inefficient operating conditions such as mixed lubrication.
The operation in mixed lubrication leads to a higher abrasion within the bearing and should thus be avoided as it affects the otherwise very low wear in hydrodynamic bearings compared to rolling bearings. However, the operation in mixed lubrication can not be avoided totally for hydrodynamic bearings, especially when they are facing transient operating conditions during run up or high dynamic loads. Therefore, recent publications investigate mixed lubrication models, in order to describe the mechanical behavior in the mixed lubrication regime more accurately or to derive certain design solutions fit to mitigate the effects of operation in mixed lubrication (Xiao et al., 2021; Guo et al., 2022; Patel et al., 2022; Tang et al., 2023). CM strategies that can detect failures in journal bearings, are among others, the aforementioned temperature and acoustics related fault detection strategies (Fritz et al., 2001; AlShorman et al., 2021; Baszenski et al., 2023). However, they can only detect faults after they have occurred. In order to assess operating conditions and the remaining useful life of the bearing, additional sensors are required to measure load, ambient temperature and rotational rates. With this sensor information an assessment of the remaining useful life can be made (Okoh et al., 2014).
The most critical failure modes of journal bearings occur in relation to mixed lubrication where the corresponding wear is usually caused by insufficient lubrication (Muzakkir et al., 2015). Using the impedance of the bearing for CM offers the chance to directly gain information about the lubrication film thickness and the state of the lubricant over the bearing’s lifetime. If, for example, viscosity stabilizing additives are slowly used up over the bearing’s lifetime, a reduction of lubrication film thickness would be detected before a failure of the lubricant occurs. Furthermore, the impedance of the bearing is dependent on the bearing load (Furtmann, 2017). Therefore, measuring the impedance allows a qualitative and potentially quantitative assessment of the bearing load.
The capacitive behavior of journal bearings has been known for decades, as described by Prashad, (2006) and Bittner and Kluth, (1995). One addition to Prashad’s work is the work of Furtmann, (2017) who extends the capacitive equation of journal bearings with tilt angles. However, Furtmann only covers the electrical behavior and neglects the effects that the tilting has on the hydrodynamic behavior of the bearing. Furthermore, these publications do not create a relation between CM and the journal bearing impedance. Instead they exclusively focus on the electric behavior of the bearing. The work of Furtmann, Prashad and Bittner et al. is of relevance regarding the electrification of automotive drive trains, which also involves electric leakage currents that can damage bearings and need to be investigated. But this does not take advantage of the CM potential of the electric behavior of the bearing.
In order to analyse the literature in this field, a systematic literature review has been performed using “Web of Science”. The string used in “Web of Science” was capacitance journal bearing OR capacity journal bearing and electric. This resulted in 32 results which were screened using the abstract. Additionally, google scholar was used to extend the results using the string capacitance “journal bearing” which resulted in 2,280 results. Of these results, 100 were selected according to their fit to the string and title screened. After this screening 16 results remained which were screened using their abstract. After reviewing the abstracts of the literature found, the literature regarding the load capacity of journal bearings in electrical machines or similar results were discarded as this does not fall into the scope of this contribution. Additionally, eight papers deal with the use of magnetorheological fluids in active journal bearings (Nikolakopoulos and Papadopoulos, 1997; Peng and Zhu, 2006; Bouzidane and Thomas, 2008; Lee, 2015; Zapoměl and Ferfecki, 2021; Kardoudi et al., 2022; Zapoměl and Ferfecki, 2022; Singh et al., 2023). In these papers magnetic fields are used to influence the local viscosity of the lubricant which is not within the scope of this contribution. Two papers cover the electrical damaging of journal bearings as a result of shaft voltages. Other papers offer works on electrical damaging of journal bearings which is also not in the scope of this article (Prashad and Rao, 1994; Muetze, 2010; Golkhandan and Torkaman, 2021). Two works also cover the electric coupling through hydrodynamic journal bearings but do not address condition monitoring opportunities (Dai et al., 2017; Dai et al., 2019). Prashad presents multiple theoretical works on the capacitance of journal bearings (Prashad, 1991) and evaluations of dynamics on two-lobe (Prashad, 1996) and multi-lobe (Prashad, 2006) journal bearings. However, these remain theoretical and do not work towards condition monitoring solutions. Furthermore, the mixed lubrication regime is not covered by these contributions. Similarly, Cui et al. (2014), Cho et al. (1999), Kataoka et al. (2012) and Paranjpe et al. (2000) deal with the measurement of the lubrication film thickness in journal bearings using the electrical capacitance. But they do not cover the systematic behaviour of journal bearings, nor the behaviour in the mixed lubrication regime, nor condition monitoring applications.
The reviewed literature shows a lack of coverage of condition monitoring approaches for journal bearings using the capacitance. Investigating the potential of utilizing the electrical behavior of journal bearings for CM and measurements of the lubrication state and operational parameters is the purpose of this paper. In order to evaluate this potential, a systematic understanding of the electric behaviour of the journal bearing in different lubrication regimes has to be supported. For this purpose, measurements are taken on an existing journal bearing test bench and analyzed. Furthermore, a quantitative working space model of the test bench is created to allow the phenomenological explanation of the behaviour observed in the measurements.
2.2 Quantitative working space model
The tool that is used to derive the electric model of the journal bearing is the qWSM. This modelling approach uses finite volumes, so-called working spaces, that are distributed throughout the system that is being modelled, which can contain fluids and solids. In the first iteration, the geometry of the working spaces (WS) can resemble the geometry of the individual parts. In later iterations, it may be beneficial to use smaller volumes where needed, leading to a higher resolution by subdividing the working spaces. Between each working space the working space surfaces interact and form working surface pairs (WSP). This interaction is characterized by any kind of energy flow that can be electrical, mechanical, thermal, or volumetric. Therefore, functions can only be conducted, if a WSP is formed, as every functional relation requires a form of energy exchange. In essence, each working space can be considered a subsystem of the overall system with a subsystem boundary. The overall system can be analysed by analysing the energy flow via the WSPs and identifying intended flows, unintended flows, and intended but missing flows. These different categories of energy flow result in intended functions, malfunctions and so-called non-functions. The detection of non-functions by identifying an energy flow is a unique mechanism of the qWSM in the context of product development. The model elements of the qWSM are illustrated in Figure 4.
The quantitative description of the qWSM is achieved by assigning state variables to each WS. State variables are typically quantities such as pressure, charge or temperature of the WS’ contents but can also represent more abstract quantities like the lubrication state. Furthermore, coupling equations based on the conservation of energy are assigned to the WSPs as coupling conditions. With these variables and coupling conditions, the state equation of each WS can be formulated dependent on the coupling variables. The resulting model represents the system with state equations and coupling equations for each WS and WSP respectively. The underlying theory is that the state of the overall system can be derived by calculating the state of each individual WS and their interaction (Schmitt and Kirchner, 2022). Assessing uncertainties within the specifications of the detailed embodiment using the qWSM are addressed within (Schmitt et al., 2023) for the example of additively manufactured cooling channels, e.g., used to realize advanced cooling strategies of water-jacked cooled electric drives. Thus, the qWSM provides a modelling approach to characterize energy based functions as well as system inherent properties such as surface roughness.
It allows the assessment of varying surface qualities on the system behavior, which becomes of significant relevance for journal bearing applications both for the determination of the detachment point as well as the characterization of mixed lubrication operation and thus is applicable as a model element of the journal bearing qWSM.
2.3 Journal bearing test bench
In the following section first the mechanical setup and thereafter the electrical setup of the journal bearing test bench is described.
2.3.1 Mechanical setup
The test bench used to operate the journal bearing for measurements is depicted in Figure 5. The test bench consists of a shaft that is supported by two spherical rolling element bearings and powered by an electric motor. The journal bearing is located between the spherical rolling element bearings inside a free swinging housing. The housing is supported only by two spring mechanisms in z and y direction and by a torque stanchion. In real applications of journal bearings, the bearings are used to support a shaft. The weight of the shaft and process-induced forced causes a compressive force in the bottom of the journal bearing. At the test bench applying a tensile force onto the journal bearing housing is necessary to mimic the compressive force inside the journal bearing in real applications. For the purpose of applying tensile forces, the spring mechanisms in z and y direction are used. The torque stanchion only supports the frictional torque inside the journal bearing. The operating parameters measured in the test bench are the horizontal and vertical forces at the spring mechanisms, the force measured at the torque support, the temperature of the journal bearing shell and the position of the journal bearing housing relative to the shaft using four eddy-current sensors. With this setup the test bench is able to operate at up to
2.3.2 Electrical setup
The purpose of the test bench is to measure the electrical behaviour of the journal bearing. Therefore, the bearing is connected to an electrical circuit as shown in Figure 6. The electrical circuit is a serial connection between a voltage generator, a reference resistor, the journal bearing housing, the shaft, the electric contact element and again the signal generator. The electrical behaviour can be expected to be capacitive in hydrodynamic lubrication. Therefore, an AC voltage needs to be generated to measure the impedance of the bearing. The frequency and amplitude selection for the voltage signal has to be picked in accordance with an optimization problem. An increase in voltage amplitude may result in discharge despite the oil film which may cause damage to the bearing. Therefore, the voltage should be minimised. However, this makes the signal more sensitive to disturbance voltages from the environment. An assessment of the disturbance voltages in the system results in a maximum disturbance voltage of
Lastly, the magnitude of the reference resistor
In order to calculate the impedance of the bearing, the voltage drop over the bearing
2.4 Modelling mixed lubrication using the qWSM
This section presents two approaches that allow the electrical modelling of the journal bearing using the qWSM. In literature, the modelling of mixed lubrication is usually presented through stochastic modelling (Dobrica and Fillon, 2013). The deterministic finite element modelling of mixed lubrication is possible but impractical for large surface applications due to the necessity to map the surface roughness of the contact surfaces. Figure 7 shows the proposed micro-structural model that can accommodate a stochastic surface roughness model. In this model, the metal to metal contact can be represented with one working space each. These working spaces are expected to show a resistive behavior. However, these contact surfaces are expected to carry foreign particles and oil residue. Therefore, the calculation of the resistance of these contact surfaces is currently not possible due to the complexity of the contaminated contact (Vinaricky, 2016). The sections with hydrodynamic lubrication can be described with another working space each. These working spaces are expected to show a capacitive behavior dependent on the distance between the two surfaces and can be modelled as a capacitor. The distribution and number of electric contact working spaces can be calculated stochastically using the models of Greenwood and Williamson (Greenwood and J, 1966) as well as Patir and Cheng (Patir and Cheng, 1979).
However, the measurement results in Section 3.1 show a dynamic electrical behavior that is generally operating with high impedances. These high impedances are frequently disrupted by low impedances. As a result of this observation, a time-varying working space model for mixed lubrication is proposed and depicted in Figure 8. This model largely follows the logic of Heemskerk et al. (Heemskerk et al., 1982) who introduced the percent contact time (PCT) value when measuring the impedance of ball bearings. In this time-varying model, it is assumed that the lubrication condition can exist in either of two states. In state 0 (Figure 8A), it is assumed that boundary lubrication occurs with the asperities of the two contacting surfaces being in direct mechanical contact with each other. This results in a low impedance. In state 1 (Figure 8B), the bearing operates in hydrodynamic lubrication.
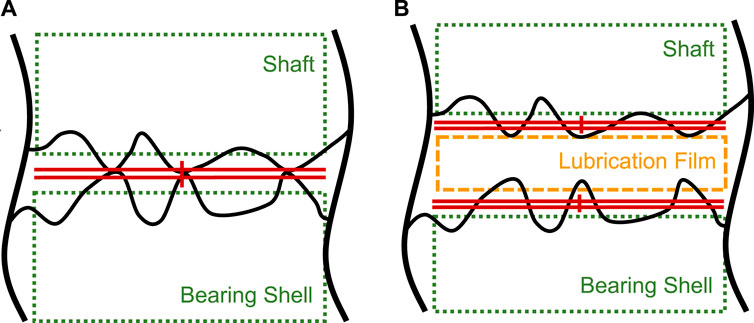
Figure 8. Timed Working Space model for (A) the state of contact and (B) the state of hydrodynamic lubrication.
To apply these states the time line can be split into constant time windows. To differentiate between the two states, a probability
The impedance of the bearing during the time windows of hydrodynamic lubrication
3 Results
In the following section the experimental results will be described. Initially an example measurement will be described to explain the analysis process. Thereafter, the results are taken into context of the known tribological behaviour of journal bearings. Furthermore, the results in mixed lubrication are analysed in detail and interpreted using the qWSM. Each operating condition presented in this contribution has been investigated three times in random order to ensure no statistical inaccuracies in the behaviour. The results presented in this section are a selection of the measurements to improve readability and are representative of the behaviour observed in each of the three test runs.
3.1 Determination of the lubrication regime
The measurements in this section are taken over the whole operating regime of the journal bearing including boundary lubrication, mixed lubrication and hydrodynamic lubrication. For the measurements the voltage drops over the bearing
Figure 9 shows the results for the FFT, with each point plotted being the result of one time window. For each measurement, the resulting amplitude of the voltage signals over the reference resistor and the bearing is plotted. Additionally, the phase angle between both voltages is plotted. When the bearing is behaving like a capacitor, the expected phase shift is
Figure 9A shows the behavior of the bearing at
Figure 9B) shows the bearing behavior at
3.2 Interpretation of results using the known stribeck model
Figure 10 shows the mean and standard deviation of the measured impedance’s presented in Figure 9 for varying rotation rates at a constant temperature and load. Given this experimental data a good qualitative fit of the measured impedance and the well known model of the Stribeck curve can be observed. This shows a qualitative correlation between the electrical impedance and the lubrication condition within lubricated contacts. This correlation is inverse as the impedance of the bearing increases with increasing revolutions while the friction coefficient decreases with an increase in revolutions until reaching the detachment point. However, this observation is only valid until the detachment point. After the detachment point the impedance signal continues to increase with an increase in revolutions while the friction coefficient reaches its minimum at the detachment point.
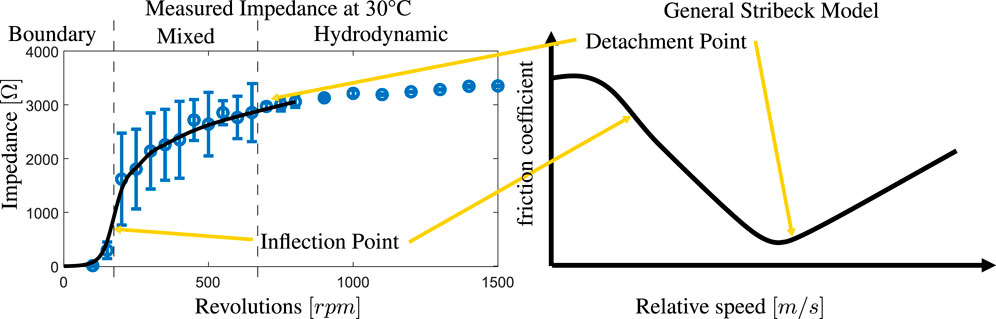
Figure 10. Comparison of the measured impedance and standard deviation of the Journal bearing test bench and the Stribeck model according to (Cabrera, 2024) for a run up test at
It can further be observed that the standard deviation in the mixed lubrication regime remains significant. Considering the measurements presented in Figure 9, it can be assumed that the lubrication regimes can be determined by mainly using the standard deviation as a determining factor. This is due to the standard deviation increasing in mixed lubrication and tending towards zero in boundary and hydrodynamic lubrication. The absolute impedance of a journal bearing on the other hand is dependent on bearing dimensions and the electrical properties of the lubricant. However, a precise detachment point cannot be determined as the reduction in standard deviation from mixed lubrication to hydrodynamic lubrication is continuous and not sudden.
It is further noteworthy, that the observed detachment point of the journal bearing used in this contribution is determined to be at
An additional problem of the impedance measurement is that the clear distinction of individual points, i.e., detachment point, is not possible. Instead the measurements show a gradual reduction of standard deviation and a gradual reduction of individual contacts while increasing the rotation rate of the shaft. It is therefore not possible to clearly identify the transition point from boundary lubrication to mixed lubrication and from mixed lubrication to hydrodynamic lubrication using the measurements taken for Figures 9, 10. In the traditional Stribeck model the detachement point is usually described to be reached when the lubrication film thickness reaches three times the root mean square (RMS) of the surface roughness. However, depending on the book or paper, sometimes the ratio between the film thickness and RMS surface roughness is mentioned to be
3.3 Results in mixed lubrication
In this section the electrical behaviour of the journal bearing in the mixed lubrication regime will be analysed in more detail. For this purpose Figure 11 shows measurements taken between
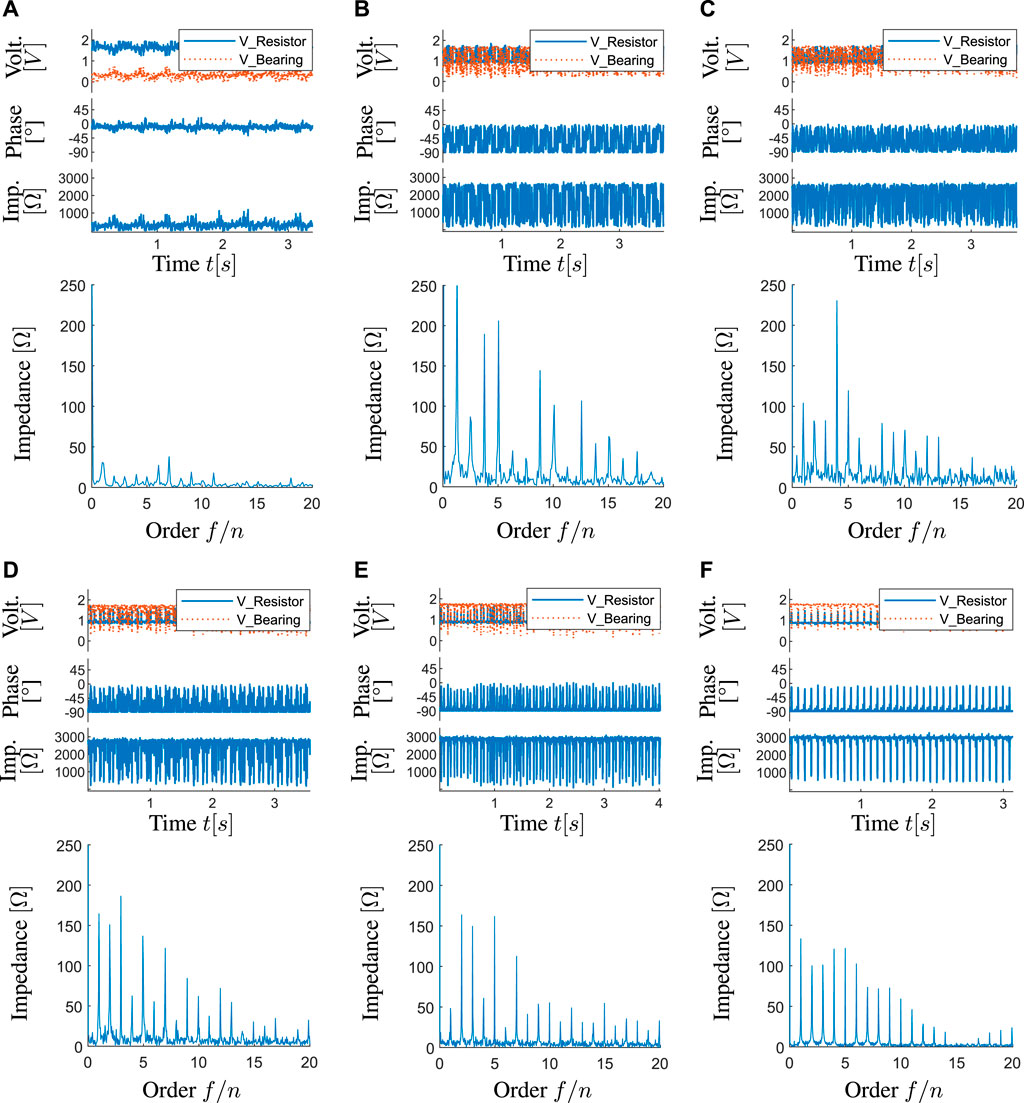
Figure 11. Measurement results for 1500 N vertical load, 30°C oil temperature and (A)
Some uncertainty lies with the phase angles of up to
An alternative hypothesis is the effect that the FFT has on the measurement results. In order to perform the FFT, the measured voltages are divided into time windows of
Another noteworthy difference between Figure 9A at
This general behaviour does not change with higher rpms at
The measurement with the highest rpm in mixed lubrication (Figure 11F) at
Due to the distinctly different electrical behaviour of the bearing depending on the relative speed, it may be possible to divide the mixed lubrication regime in multiple electrical regimes. In Figure 11A for example, the impedance signal shows a periodic behaviour. In this case the impedance is somewhere between the impedance during boundary lubrication and hydrodynamic lubrication. On the other hand, Figure 11B shows an oscillating behaviour with an increasing amount of hydrodynamic lubrication at higher relative speeds. A further increase in relative speed in Figure 11F shows a reduction in noise but a periodic disruption of the lubrication film. According to Figure 10 this result at
4 Discussion
In summary, this contribution aims to visualise and model the lubrication condition of hydrodynamic journal bearings based on the qWSM and impedance measurements. For this purpose, a journal bearing test bench was operated at constant load and varying speeds. The results show that the impedance measurement is suitable for visualising the lubrication regime of journal bearings. The expected capacitive behaviour of the bearing in hydrodynamic lubrication can be confirmed. The literature assessed in the introduction and state of research is limited to investigations of the electric behaviour of the bearing in hydrodynamic lubrication with the underlying assumptions that the capacitive modelling approach is not suitable for metal to metal contact. This can partially be confirmed as metal to metal contact produces resistive-capacitive behaviour instead of pure capacitive behaviour. However, important qualitative observations can be made even during periods of partial metal to metal contact. The impedance measurement in journal bearing is therefore further suited to observe the behaviour of the bearing in mixed lubrication and allows further interpretation (as discussed in section 3.3). As a result of the detailed analysis of the measurements taken in the mixed lubrication regime, the mixed lubrication regime can be classified into three distinctly different regimes with averaging behaviour (Figure 11A), noisy-oscillating behaviour (Figures 11B–E) and oscillating behaviour Figure 11F.
In order to explain the behaviour of the bearing, the quantitative working space model is used based on the phenomenological observations with the time varying model and based on the state of the art with the microstructural model. Each of these models can be equally valid for condition monitoring approaches as the microstructural model seems more suitable for the aforementioned averaging behaviour while the time-varying model seems more suitable for the oscillating behaviour. H1 can therefore be verified as the qWSM can describe and visualise the electrical behaviour of the journal bearing in all lubrication regimes. Whether the model will be able to support prediction or condition monitoring calculations for the electrical behaviour will have to be investigated in the future. It will also have to be investigated, whether influence factors on the electrical behaviour can be quantified using the qWSM modelling approach. One question that can’t be answered currently is whether the measured phase angles of, e.g.,
One solution to this problem could be a measurement with DC-voltages or offset AC-voltages in which the FFTs of the voltage signals are not required to detect metal to metal contact. This way the frequency resolution of the FFT is no longer a limitation and the time resolution is represented directly by the measurement frequency of the voltages. Alternatively, a curve fitting method could be used to fit a known sine signal to the measured signal which has the potential to improve the time resolution compared to an FFT analysis. In any case, the duration of a single contact within a time window will have to be investigated in future. The measurement results further prove that observing the electric behaviour of journal bearings continuously does not only allow a distinction between ohmic and capacitive behaviour, but it further allows the classification of the mixed lubrication regime. It also allows an assessment of whether the bearing displays averaging, noisy-oscillating or oscillating behaviour. The corresponding state according to the Stribeck curve could therefore be determined more closely using the electrical behaviour observed in this contribution. With this knowledge, small changes in the operating strategy can be made based on the behaviour observed to avoid unintended and unobserved abrasive wear or to improve the lubrication. H2 is therefore also proven right. Further developing descriptive models and the corresponding qWRM could improve the precision of the state determined according to the Stribeck curve.
The main problem with determining the lubrication regime is the difficulty to identify transition points. The results in Figures 9, 11 show different behaviours and measurement results of the bearing. However, the change between the different states is gradual and an individual point cannot be identified. The identification of an individual point, i.e., the detachment point, cannot be made at this time. The definition of a transition point can in theory be made using features like standard deviation according to Figure 10 but this definition is arbitrary and does not explain phenomenological differences between the different types of behaviours displayed by the bearing during measurements. But it may be sufficient depending on the monitoring application. As was pointed out in section 3.2, even analysis based on the friction coefficient according to Stribeck may not allow the identification of transition points between lubrication regimes.
Despite the difficulty to identify the transition point between lubrication regimes and different behaviours in mixed lubrication with the known Stribeck-model, the continuous observation of changes in the electrical behaviour of the journal bearing is possible. Lubrication conditions can deteriorate over time due to physical and chemical processes in the lubricant or due to physical wear of the bearing. Continuously monitoring the electrical behaviour would allow the detection of this deterioration before the detachment point is reached. When operating in mixed lubrication the deterioration could be observed by detecting an increase in the noise level of the oscillating behaviour or by detecting a change from oscillating behaviour to averaging behaviour in the bearing. Which features are suitable to detect changes in the electrical behaviour over time will have to be investigated in the future.
Further research in future will also include quantifying the qWSM models presented in this contribution which can help to describe the electrical behaviour of this bearing. The expansion of these models may allow even prediction of the state of operation of the bearing and assessments about expected bearing behaviour in different operating conditions. It may further allow the developing of wear models and predictive maintenance concepts if the behaviour of the bearing can be predicted to a sufficient accuracy. In this context it is also necessary to answer the question, whether impedance measurement can be beneficial compared to other monitoring solutions (i.e., temperature monitoring) in terms of building space, cost and observation accuracy. Especially cost is an unknown factor in impedance measurement as the electrical insulation required to refit a system with impedance measurement equipment may be negligible or extremely costly and complex depending on the machine and application.
An additional observation of this contribution is that the oscillating behaviour observed indicates roundness deviations as described by Ebermann Prase, (2019). This detection of the suspected roundness deviation or potential damage could further improve the knowledge provided by the measurement of the electrical impedance. This knowledge could improve condition monitoring approaches. It is already possible to mark individual contacts by observing the electrical behaviour. It could also be possible to develop a theory of damage accumulation based on the number of contacts observed under a certain load, speed and temperature for predictive maintenance. However, this possibility will have to be investigated in the future.
Data availability statement
The raw data supporting the conclusions of this article will be made available by the authors, without undue reservation.
Author contributions
FK: Conceptualization, Data curation, Investigation, Software, Validation, Visualization, Writing–original draft, Writing–review and editing. FS: Conceptualization, Investigation, Methodology, Project administration, Supervision, Writing–original draft, Writing–review and editing. EK: Funding acquisition, Resources, Writing–original draft, Writing–review and editing. EZ: Writing–original draft, Writing–review and editing.
Funding
The author(s) declare that financial support was received for the research, authorship, and/or publication of this article. The authors thank the Deutsche Forschungsgemeinschaft (DFG, German Research Foundation), which funded the presented research within the project “Opportunities and limits of the working space model in product development” Funded by the Deutsche Forschungsgemeinschaft (DFG, German Research Foundation) – 443578519.
Conflict of interest
The authors declare that the research was conducted in the absence of any commercial or financial relationships that could be construed as a potential conflict of interest.
Publisher’s note
All claims expressed in this article are solely those of the authors and do not necessarily represent those of their affiliated organizations, or those of the publisher, the editors and the reviewers. Any product that may be evaluated in this article, or claim that may be made by its manufacturer, is not guaranteed or endorsed by the publisher.
References
AlShorman, O., Alkahatni, F., Masadeh, M., Irfan, M., Glowacz, A., Althobiani, F., et al. (2021). Sounds and acoustic emission-based early fault diagnosis of induction motor: a review study. Adv. Mech. Eng. 13, 168781402199691. doi:10.1177/1687814021996915
Amirat, Y., Benbouzid, M., Al-Ahmar, E., Bensaker, B., and Turri, S. (2009). A brief status on condition monitoring and fault diagnosis in wind energy conversion systems. Renew. Sustain. Energy Rev. 13, 2629–2636. doi:10.1016/j.rser.2009.06.031
S. aus der Wiesche, and F. Joos (2018). Handbuch dampfturbinen: grundlagen, konstruktion, betrieb (Cham: Springer Fachmedien Wiesbaden and Springer International Publishing AG). doi:10.1007/978-3-658-20630-7
Baszenski, T., Kauth, K., Kratz, K.-H., Gutiérrez Guzmán, F., Jacobs, G., and Gemmeke, T. (2023). Sensor integrating plain bearings: design of an energy-autonomous, temperature-based condition monitoring system. Forsch. Im. Ingenieurwes. 87, 441–452. doi:10.1007/s10010-023-00642-1
Bittner, H., and Kluth, T. (1995). Gleitlagerdiagnose mittels magnetfeldmessungen. Tm. - Tech. Mess. 62, 346–351. doi:10.1524/teme.1995.62.jg.346
Bouzidane, A., and Thomas, M. (2008). An electrorheological hydrostatic journal bearing for controlling rotor vibration. Comput. and Struct. 86, 463–472. doi:10.1016/j.compstruc.2007.02.006
Branagan, L. (2015). Survey of damage investigation of babbitted industrial bearings. Lubricants 3, 91–112. doi:10.3390/lubricants3020091
Cabrera, F. (2024). Electric vehicle tribology: challenges and opportunities for a sustainable transportation future. auflage edn (Amsterdam: Elsevier Series on Tribology and Surface Engineering Series), 1.
Childs, P. R. N. (2019). Mechanical design engineering handbook. second edition edn (Oxford and Cambridge, MA: Butterworth-Heinemann).
Cho, M.-R., Han, D.-C., and Choi, J.-K. (1999). Oil film thickness in engine connecting-rod bearing with consideration of thermal effects: comparison between theory and experiment. J. Tribol. 121, 901–907. doi:10.1115/1.2834153
Cui, Z., Yang, C., Sun, B., and Wang, H. (2014). Liquid film thickness estimation using electrical capacitance tomography. Meas. Sci. Rev. 14, 8–15. doi:10.2478/msr-2014-0002
Dąbrowski, D., and Natarajan, A. (2015). Assessment of gearbox operational loads and reliability under high mean wind speeds. Energy Procedia 80, 38–46. doi:10.1016/j.egypro.2015.11.404
Dai, J., Hagen, S. S., and Ludois, D. C. (2017). Linear motion system cable elimination via multiphase capacitive power transfer through sliding journal bearings, 2157–2164. doi:10.1109/APEC.2017.7930998
Dai, J., Hagen, S. S., and Ludois, D. C. (2019). High-efficiency multiphase capacitive power transfer in sliding carriages with closed-loop burst-mode current control. IEEE J. Emerg. Sel. Top. POWER Electron. 7, 1388–1398. doi:10.1109/JESTPE.2018.2845385
Dobrica, M. B., and Fillon, M. (2013). “Mixed lubrication,” in Encyclopedia of tribology (Boston, MA: Springer), 2284–2291. doi:10.1007/978-0-387-92897-5_27
Ebermann, B. H. A., and Prase, M. (2019). Influence of geometric shape defects on operating parameters in cylindrical journal bearings. doi:10.1115/IMECE2019-10179
Fett, M., Puchtler, S., Zaiat, A., Bock, M., Melzer, C., Hoffmann, F., et al. (2024). Practical implementation of the sensory utilization of rolling bearing impedance measurement: a case study in machine tool spindle bearings. Tribol. Trans., 1–11doi. doi:10.1080/10402004.2024.2370902
Fritz, M., Burger, W., and Albers, A. (2001). Schadensfrüherkennung an geschmierten Kontakten mittels schallemissionsanalyse (karlsruhe). doi:10.5445/IR/5782002
Furtmann, A. (2017) “Elektrisches Verhalten von Maschinenelementen im Antriebsstrang,”. Hannover: Gottfried Wilhelm Leibniz Universität Hannover. Ph.D. thesis. doi:10.15488/8972
Golkhandan, R. K., and Torkaman, H. (2021). Reduction of induced shaft voltage and bearing current in turbo generators: modeling, compensation, and practical test. IEEE Trans. Industrial Electron. 68, 4362–4372. doi:10.1109/TIE.2020.2985006
Goyal, D. V., Pabla, B. S., and Dhami, S. S. (2019). Non-contact sensor placement strategy for condition monitoring of rotating machine-elements. Eng. Sci. Technol. Int. J. 22, 489–501. doi:10.1016/j.jestch.2018.12.006
Greenwood, J., and J, B. P. W. (1966). Contact of nominally flat surfaces. Proc. R. Soc. Lond. Ser. A. Math. Phys. Sci. 295, 300–319. doi:10.1098/rspa.1966.0242
Guo, H., Bao, J., Zhang, S., and Shi, M. (2022). Experimental and numerical study on mixed lubrication performance of journal bearing considering misalignment and thermal effect. Lubricants 10, 262. doi:10.3390/lubricants10100262
Heemskerk, R. S., Vermeiren, K. N., and Dolfsma, H. (1982). Measurement of lubrication condition in rolling element bearings. A S L E Trans. 25, 519–527. doi:10.1080/05698198208983121
Jablonka, K., Glovnea, R., and Bongaerts, J. (2018). Quantitative measurements of film thickness in a radially loaded deep-groove ball bearing. Tribol. Int. 119, 239–249. doi:10.1016/j.triboint.2017.11.001
Jaspert, D., Ebel, M., Eckhardt, A., and Poeppelbuss, J. (2021). Smart retrofitting in manufacturing: a systematic review. J. Clean. Prod. 312, 127555. doi:10.1016/j.jclepro.2021.127555
Kandeva, M., Karastoyanov, D., Assenova, E., Jakimovska, K., Simeonov, S., and Vencl, A. (2016). The influence of the valena metal-plating additive on tribotechnical characteristics of the steel–bronze tribological system. J. Frict. Wear 37, 187–190. doi:10.3103/S1068366616020082
Kardoudi, M., Nabhani, M., and El Khlifi, M. (2022). Non-Newtonian couple stress lubrication of mhd elastic journal bearings. Industrial Lubr. Tribol. 74, 753–762. doi:10.1108/ILT-11-2021-0456
Kataoka, T., Kikuchi, T., and Ashihara, K. (2012). Measurement of oil film thickness in the main bearings of an operating engine using thin-film electrode. SAE Int. J. Fuels Lubr. 5, 425–433. doi:10.4271/2011-01-2117
Kraus, B., Schmitt, F., Steffan, K.-E., and Kirchner, E. (2021). A valve closing body as a central sensory-utilizable component. Procedia CIRP 100, 109–114. doi:10.1016/j.procir.2021.05.018
Lee, Y.-B. (2015). Behavior analysis of controllable electrorheology fluid plain journal bearings. J. Dyn. Syst. Meas. CONTROL-TRANSACTIONS ASME 137. doi:10.1115/1.4029369
Manjunath, M., Hausner, S., Heine, A., de Baets, P., and Fauconnier, D. (2024). Electrical impedance spectroscopy for precise film thickness assessment in line contacts. Lubricants 12, 51. doi:10.3390/lubricants12020051
Maruyama, T., Maeda, M., and Nakano, K. (2019). Lubrication condition monitoring of practical ball bearings by electrical impedance method. Tribol. Online 14, 327–338. doi:10.2474/trol.14.327
Mokhtari Molk Abadi, N. (2020) “Überwachung hydrodynamischer Gleitlager basierend auf der Körperschallanalyse,”. Technische Universität Berlin. Dissertation.
Mourtzis, D., Angelopoulos, J., and Panopoulos, N. (2020). Recycling and retrofitting for industrial equipment based on augmented reality. Procedia CIRP 90, 606–610. doi:10.1016/j.procir.2020.02.134
Muetze, A. (2010). On a new type of inverter-induced bearing current in large drives with one journal bearing. IEEE Trans. Industry Appl. 46, 240–248. doi:10.1109/TIA.2009.2036672
Muzakkir, S. M., Lijesh, K. P., and Hirani, H. (2015). Failure mode and effect analysis of journal bearing. Int. J. Appl. Eng. Res. 10, 37752–37759.
Nikolakopoulos, P. G., and Papadopoulos, C. A. (1997). Controllable misaligned journal bearings, lubricated with smart fluids. J. Intelligent Material Syst. Struct. 8, 125–137. doi:10.1177/1045389X9700800203
Okoh, C., Roy, R., Mehnen, J., and Redding, L. (2014). Overview of remaining useful life prediction techniques in through-life engineering services. Procedia CIRP 16, 158–163. doi:10.1016/j.procir.2014.02.006
Paranjpe, R. S., Tseregounis, S. I., and Viola, M. B. (2000). Comparison between theoretical calculations and oil film thickness measurements using the total capacitance method for crankshaft bearings in a firing engine. Tribol. Trans. 43, 345–356. doi:10.1080/10402000008982350
Pastukhov, A., and Timashov, E. (2023). Procedure for simulation of stable thermal conductivity of bearing assemblies. Adv. Eng. Lett. 2, 58–63. doi:10.46793/adeletters.2023.2.2.3
Patel, R., Khan, Z. A., Saeed, A., and Bakolas, V. (2022). A review of mixed lubrication modelling and simulation. Tribol. Industry 44, 150–168. doi:10.24874/ti.1186.09.21.11
Patir, N., and Cheng, H. S. (1979). Application of average flow model to lubrication between rough sliding surfaces. J. Lubr. Technol. 101, 220–229. doi:10.1115/1.3453329
Peng, H., Zhang, H., Fan, Y., Shangguan, L., and Yang, Y. (2023). A review of research on wind turbine bearings’ failure analysis and fault diagnosis. Lubricants 11, 14. doi:10.3390/lubricants11010014
Peng, J., and Zhu, K.-Q. (2006). Effects of electric field on hydrodynamic characteristics of finite-length er journal bearings. Tribol. Int. 39, 533–540. doi:10.1016/j.triboint.2005.03.017
Pennacchi, P. (2022). “16 - advanced technologies for steam turbine bearings,” in Advances in steam turbines for modern power plants. Editor T. Tanuma (Oxford and Amsterdam: Woodhead Publishing and Elsevier ScienceDirect), 383–448. doi:10.1016/B978-0-12-824359-6.00009-3
Prashad, H. (1991). Theoretical evaluation of capacitance, capacitive reactance, resistance and their effects on performance of hydrodynamic journal bearings. J. Tribol. 113, 762–767. doi:10.1115/1.2920690
Prashad, H. (1996). Evaluation of dynamic coefficients of a two-lobe journal bearing using an electrical analogy approach. J. Tribol. 118, 657–662. doi:10.1115/1.2831588
Prashad, H. (2006) “Tribology in electrical environments,” in Tribology and interface engineering series, 49. Amsterdam: Elsevier.
Prashad, H., and Rao, K. N. (1994). Analysis of capacitive effect and life estimation of hydrodynamic journal bearings on repeated starts and stops of a machine operating under the influence of shaft voltages. Tribol. Trans. 37, 641–645. doi:10.1080/10402009408983341
Puchtler, S., van der Kuip, J., and Kirchner, E. (2023). Analyzing ball bearing capacitance using single steel ball bearings. Tribol. Lett. 71, 38–10. doi:10.1007/s11249-023-01706-7
Rolink, A., Jacobs, G., Müller, M., Jakobs, T., and Bosse, D. (2022). Investigation of manufacturing-related deviations of the bearing clearance on the performance of a conical plain bearing for the application as main bearing in a wind turbine. J. Phys. Conf. Ser. 2257, 012006. doi:10.1088/1742-6596/2257/1/012006
Rolink, A., Schröder, T., Jacobs, G., Bosse, D., Hölzl, J., and Bergmann, P. (2020). Feasibility study for the use of hydrodynamic plain bearings with balancing support characteristics as main bearing in wind turbines. J. Phys. Conf. Ser. 1618, 052002. doi:10.1088/1742-6596/1618/5/052002
Sanchez-Londono, D., Barbieri, G., and Fumagalli, L. (2023). Smart retrofitting in maintenance: a systematic literature review. J. Intelligent Manuf. 34, 1–19. doi:10.1007/s10845-022-02002-2
Saruhan, H., Sandemir, S., Çiçek, A., and Uygur, I. (2014). Vibration analysis of rolling element bearings defects. J. Appl. Res. Technol. JART 12, 384–395. doi:10.1016/S1665-6423(14)71620-7
Schirra, T., Martin, G., Neu, M., and Kirchner, E. (2019). Feasibility study of impedance analysis for measuring rolling bearing loads. STLE, 2019.
Schmitt, F., and Kirchner, E. (2022). Caenorhabditis elegans as a model for the effects of phytochemicals on mitochondria and aging. How Prod. Manuf. Des. enable Sustain. Co. Soc. (The Design Society) 12, 1550. doi:10.3390/biom12111550
Schmitt, F., Sallehsari, K., and Kirchner, E. (2023). “How to control the surface qualities in am channels?,” in Proceedings 34th DFX 2023, 153–162. doi:10.35199/dfx2023.16
Schneider, V., Bader, N., Liu, H., and Poll, G. (2022). Method for in situ film thickness measurement of ball bearings under combined loading using capacitance measurements. Tribology International 171, 107524. doi:10.1016/j.triboint.2022.107524
Schösser, F., Schmitt, F., Kirchner, E., and Breimann, R. (2022). Influence of the operation strategy on the energy consumption of an autonomous sensor node. Journal of Sensors and Sensor Systems 11, 263–275. doi:10.5194/jsss-11-263-2022
Singh, A. K., Kumar, V., Singh, S. J., and Sharma, S. C. (2023). Performance of hybrid thrust bearing textured surface operating with electro-rheological lubricant. Proceedings of the Institution of Mechanical Engineers, Part J Journal of Engineering Tribology 237, 911–925. doi:10.1177/13506501221128110
Surucu, O., Gadsden, S. A., and Yawney, J. (2023). Condition monitoring using machine learning: a review of theory, applications, and recent advances. Expert Systems with Applications 221, 119738. doi:10.1016/j.eswa.2023.119738
Tang, D., Xiang, G., Guo, J., Cai, J., Yang, T., Wang, J., et al. (2023). On the optimal design of staved water-lubricated bearings driven by tribo-dynamic mechanism. Physics of Fluids 35, 093611. doi:10.1063/5.0165807
Tavner, P. J., Ran, L., Penman, J., and Sedding, H. (2008) “Condition monitoring of rotating electrical machines,” in vol. 56 of IET power and energy series. London: Inst. of Engineering and Technology. doi:10.1049/PBPO056E
Vinaricky, E. (2016). Elektrische Kontakte, Werkstoffe und Anwendungen. Berlin, Heidelberg: Springer Berlin Heidelberg. doi:10.1007/978-3-642-45427-1
Vorwerk-Handing, G. (2021). Erfassung systemspezifischer Zustandsgrößen – Physikalische Effektkataloge zur systematischen Identifikation potentieller Messgrößen (Darmstadt). Gunnar.
Vorwerk-Handing, G., Gwosch, T., Schork, S., Kirchner, E., and Matthiesen, S. (2020). Classification and examples of next generation machine elements. Forschung im Ingenieurwesen 84, 21–32. doi:10.1007/s10010-019-00382-1
Vorwerk-Handing, G., Martin, G., and Kirchner, E. (2018). “Integration of measurement functions in existing systems – retrofitting as basis for digitalization,” in Design in the era of digitalization (scotland: the design society).
Weychardt, J. H., Thiemke, M., and Christiansen, K. (2008). Beschreibung der Interaktion maschinen-und schiffbaulicher Komponenten in allen für Beschreibung der Interaktion maschinen-und schiffbaulicher Komponenten in allen für Ausrichtrechnung relevanten Bau-und Betriebszuständen.
Xiao, Z., Shi, X., Wang, X., Ma, X., and Han, Y. (2021). Lubrication analysis and wear mechanism of heavily loaded herringbone gears with profile modifications in full film and mixed lubrication point contacts. Wear 477, 203790. doi:10.1016/j.wear.2021.203790
Zapoměl, J., and Ferfecki, P. (2021), Vibration control of rotors mounted in hydrodynamic bearings lubricated with magnetically sensitive oil by changing their load capacity. Bulletin of the Polish Academy of Sciences Technical Sciences. e137988. 1–8. doi:10.24425/bpasts.2021.137988
Keywords: journal bearing, condition monitoring, in-situ measurement, impedance measurement, quantitative working space model
Citation: Koetz F, Schmitt F, Kirchner E and Zancul E (2024) Visualising the lubrication condition in hydrodynamic journal bearings using impedance measurement. Front. Mech. Eng. 10:1456575. doi: 10.3389/fmech.2024.1456575
Received: 28 June 2024; Accepted: 06 August 2024;
Published: 21 August 2024.
Edited by:
Satoru Maegawa, Nagoya Institute of Technology, JapanReviewed by:
Fengshou Gu, University of Huddersfield, United KingdomMilan Bukvic, Faculty of Engineering, University of Kragujevac, Serbia
Copyright © 2024 Koetz, Schmitt, Kirchner and Zancul. This is an open-access article distributed under the terms of the Creative Commons Attribution License (CC BY). The use, distribution or reproduction in other forums is permitted, provided the original author(s) and the copyright owner(s) are credited and that the original publication in this journal is cited, in accordance with accepted academic practice. No use, distribution or reproduction is permitted which does not comply with these terms.
*Correspondence: Florian Koetz, florian.koetz@tu-darmstadt.de