- 1MCI Management Center Innsbruck, Innsbruck, Austria
- 2Vienna University of Technology, Vienna, Austria
Diesel engines, in combination with modern turbocharging and common rail injection systems, feature comparably high efficiencies coupled with favorable torque characteristics. Therefore, besides the utilization in commercial vehicles, diesel engines gained large market shares within the passenger car sector. The European Union regulates the deployment of biofuels and has issued several targets throughout the years; focusing on the urge to reduce carbon dioxide emissions, as well as on the growing demand for independence of fossil energy sources. This contribution presents investigations on fuel blends containing different shares of biomass to liquid, hydrotreated vegetable oils, and fatty acid methyl ester applied in a state of the art light-duty diesel engine. In general, the reviewed fuel blends exhibit good applicability for the utilization in the test engine with default settings of injection timing and exhaust gas recirculation; adjustments on the just mentioned parameters unveil further potential in terms of emissions and efficiency. Particularly the potential of biomass to liquid blends to partly mitigate the tradeoff between particulate matter and nitrogen oxides deserves distinct attention.
Introduction
Until 2050, an almost CO2-neutral traffic sector is required. This target is directly based on compliance with the Paris Climate Conference of 2015. These ambitious goals cannot be achieved by using conventional raw materials, namely crude oil or natural gas, for fuel production. Rather, an increasing variety of technically meaningful alternative fuels can be expected, which are tailored to respective applications' special necessities.
Biofuels as representatives of alternative fuels, such as fatty acid methyl ester (FAME), hydrotreated vegetable oils (HVO), biomass to liquid (BtL) or bioethanol, are based on biomass and can be blended with conventional fuels derived from crude oil. These biofuels provide a renewable alternative to fossil fuels in the transport sector, in order to reduce greenhouse gas emissions and mitigate fossil oil dependency. First generation biofuels are produced from sugar, starch, and vegetable oils, whereas advanced biofuels include a broader range of feedstock, for instance, wood, straw, and algae (Ballerini, 2012; Moeltner, 2018).
Public support has been the main factor for the development of biofuels, since they are still not intrinsically profitable in most countries, and the key instruments are blending target mechanisms, which have been adopted in more than 50 countries. In the EU, the Biofuels Directive (2003/30/EC) targeted a 5.75% share (energy content) of fuels by 2010. Diesel could contain up to 7% biofuel, fatty acid methyl ester (FAME), and gasoline could contain up to 10% ethanol (related to its volume) (Moeltner, 2018).
The already-established and widely available alternative fuels of biogenous origin, ethanol and FAME, currently play the most important role. As such, Figure 1 presents the progress of the global consumption of bioethanol, biodiesel, and, additionally, hydrotreated vegetable oils (HVO). With a worldwide consumption of 135 billion liters in 2016, these three bio-based fuels deserve particular attention (Nicolas, 2017). Ethanol and FAME can be purely applied with some technical restrictions, but, in most cases, these two biofuels act as a biogenous admixture to conventional fossil fuel.
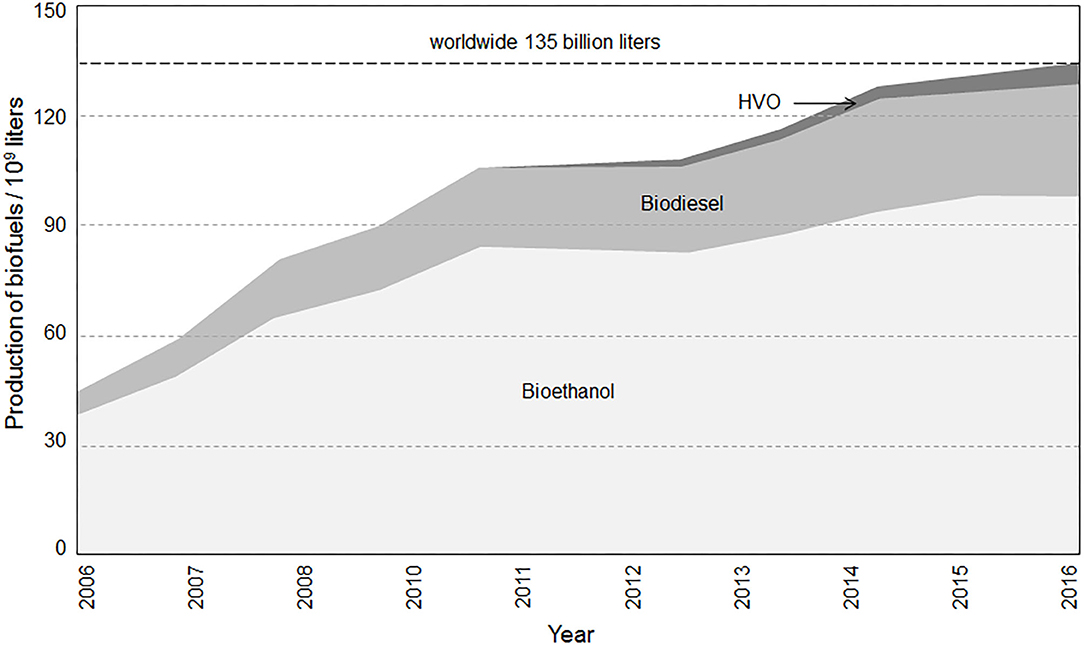
Figure 1. Global trends in bioethanol fuel (light gray), biodiesel (gray), and hydrotreated vegetable oil (dark gray) production from 2006–2016 (author's illustration based on Moeltner, 2018; REN21, 2018).
In addition to the reduction of CO2-emissions and the independence of fossil energy carriers, the application of alternative diesel fuels or blends provides many advantages. Particularly, the use of fuel blends containing hydrotreated vegetable oils (HVO) and/or biomass to liquid (BtL) are known to emit fewer regulated emissions, such as carbon monoxide (CO), hydrocarbons (HC), and particulate matter (PM) (United States Environmental Protection Agency and Department of Transportation, 2010a,b; Moeltner et al., 2017).
The presented study investigates different compositions of HVO, BtL, and FAME fuel blends and their behavior during internal combustion including fuel efficiency as well as their impact on engine-out emissions. In addition to already available studies, this work includes investigations of ternary admixtures into its scope.
Alternative Fuels for Diesel Engines
FAME produced from vegetable oils is the most commonly applied biodiesel in diesel-engines and has been predominant for many years. In addition, HVO gained increased attention during recent years. BtL fuel is theoretically produced from all kinds of biomass through gasification, followed by Fischer-Tropsch synthesis. However, the production of BtL is still on a pilot-scale but might see further commercialization in the future. The following section provides an overview of the individual production steps as well as feedstocks of FAME, HVO, and BtL. Furthermore, detailed information on fuel properties, combustion characteristics, and influence on emissions of the respective biodiesel fuels are presented (Millo et al., 2015; List, 2018; Moeltner, 2018).
Fatty Acid Methyl Esters
Currently, the most common substituents for diesel fuel are FAME. The substitution of FAME supports the reduction of greenhouse gas emissions in the transportation sector.
FAME are usually produced in a transesterification process from vegetable oils, such as soy, rapeseed, sunflower or even purified waste oil. A major component of natural fats and oils is the glycerin molecule, which can bond three fatty acids in varying sequences. During the transesterification process, fossil methanol is typically used as an alcohol to substitute for glycerin and to split the initial triglyceride. Fatty acids, which occur in fats and oils, can be distinguished as saturated or mono- or polyunsaturated compounds. The distinctive feature of these two types is the existence and quantity of carbon double bonds within the fatty acid molecule, which is generally preset by the genetics of the used plant oil or oil seed and has a great impact on its properties (Sugiyama et al., 2012; Moeltner, 2018).
A potential of FAME to reduce carbon monoxide (CO), unburned hydrocarbons (HC), and particulate matter (PM) during combustion has been detected, due to its bonded oxygen. However, different overlapping effects can cause a rise in nitrogen oxides (NOx) emissions (United States Environmental Protection Agency and Department of Transportation, 2010a; European commission, 2012). In contrast to these beneficial aspects, alterations of the fuel's viscosity and/or the formation of precipitates can cause major problems and, in extreme cases, even a complete failure of the injection system. Moreover, difficulties with FAME during the cold-start phase have repeatedly been described in the literature, which can be explained by the cold filter plugging point (CFPP) of FAME (Moeltner et al., 2017). In addition, problems during the cold-start are caused by the comparable low vapor pressure of the fuel, which leads to an increased input of FAME into the engine oil, particularly during regeneration phases of the diesel particulate filter (DPF), where post injections occur. The relatively high-boiling components of FAME cannot evaporate from the engine oil and, thus, effect a dilution and subsequently a decrease of the engine oil's lubricity.
Hydrotreated Vegetable Oils and Biomass to Liquid
The fuel properties of the renewable HVO diesel fuel are similar to those of BtL fuels. The production can occur from a wide spectrum of raw materials, for instance, vegetable and waste oils or animal fats, without considerable effects on the final fuel properties. The individual production steps of HVO can be divided as follows: pretreatment of the feedstock, hydrotreatment including hydro-cracking of molecules, and isomerization. HVO solely contains straight-chain paraffinic compounds. In contrast to other fuels, no aromatics, olefins, and naphthenes are included and no traces of sulfur can be detected. Therefore, an increase in its cetane number and calorific value is evident, as well as a decrease in its density, compared to fossil diesel (Tilli et al., 2010; Hartikka and Kiiski, 2012; Sugiyama et al., 2012; Liu et al., 2013; Moeltner, 2018).
The compounds of BtL also consist only of straight-chain alkanes. The production occurs by Fischer-Tropsch synthesis, where CO and hydrogen (H2) react to hydrocarbons in the presence of a catalyst containing cobalt. The employed synthesis gas of CO and H2 is produced in a pre-connected step by (waste) biomass gasification or from other non-food cellulosic raw materials (Ng et al., 2005; Heuser et al., 2013; Mizushima et al., 2014). However, the BtL production by the Fischer-Tropsch process occurs only on a pilot-scale until now (Bhardwaj et al., 2013).
The already mentioned hydrocarbons are from the family of alkanes (formerly paraffins), which includes all saturated hydrocarbons and is described by the universal molecular formula CnH2n+2 (e.g., heptane C7H16). Starting from C4, the backbone of alkanes can be classified into unbranched (linear) or branched carbon chains. The unbranched compounds are referred as n-alkanes, form a homologous series, and are of particular interest for diesel engines.
The inherent drawbacks of ester-based fuels, for example, rise in NOx, deposit formation, decreased oxidation stability, increased engine oil aging, or insufficient properties at low temperatures, are not evident for BtL and HVO (Mandpe et al., 2005; Rantanen et al., 2005; Zhang et al., 2009). Quite the contrary, BtL and HVO reduce exhaust gas emissions, particularly soot emissions, and reveal an increase in engine performance, primarily in combination with adapted engine controlling (Oguma et al., 2002; Sugiyama et al., 2012; Jaroonjitsathian et al., 2014).
Bio-Alcohols
In recent years, fuel blends consisting of (bio-)alcohols gained in importance and exhibit promising potential in achieving low emission diesel engines (De Poures et al., 2017). Even if (low carbon) alcohols like methanol or ethanol are mostly connoted with the utilization in gasoline engines, carbon-rich alcohols like butanol, pentanol, or hexanol are promising candidates for diesel engines (Kumar and Saravanan, 2015, 2016; Kumar et al., 2016).
Even longer alcohols are under investigation. De Poures et al. describes n-octanol to be a promising biofuel with several properties which are closer to regular diesel than the properties of n-butanol and with the potential to suppress emission formation (Kaliyaperumal et al., 2018; De Poures et al., 2019).
Methodology
Over the course of the perennial scientific work, issuing FAME, HVO, and BtL, the applied experimental setup was previously presented and published in Moeltner et al. (2017).
Engine and Test Bench
The experimental investigations included the characterization of the fuels and their emissions on an engine test bench. A state-of-the-art two-liter diesel engine, commonly used in passenger cars, featuring inline-four cylinders and EURO 6 emission calibration, was employed as a test engine in combination with a water-cooled eddy current brake. Furthermore, National Instruments LabView was applied for recording data of all thermocouples and pressure transducers and thus the investigation of engine performance and emission formation. In addition, AVL INDICOM with an AVL GU13G–14 piezoelectric pressure sensor and a Kistler 5011 amplifier was implemented for the evaluation of combustion. ETAS INCA ES 590.1 provided the engine control unit (ECU) communication via CAN, the intake airflow was determined by a thermal mass flow meter, and the fuel consumption was identified by a system based on the Coriolis principle.
The measurement position for the exhaust gas emissions was 0.3 m downstream of the exhaust gas turbine exit. The analyzing methods included non-dispersive infrared spectroscopy for CO and CO2, heated flame ionization detection for total hydrocarbons (THC), and chemiluminescence detection for NOx. For the goal of investigating trends in PM emissions the principle of a smokemeter was chosen, a further analysis of PM, e.g., determination of the soluble organic fraction, which would allow deeper insights into the chemical composition, was not carried out.
The primary intention of this study is to elaborate the potential of the fuel blends focusing on combustion and emission behavior independently from the engine's application and the configuration of an exhaust gas after treatment system to achieve emission standards. Therefore, the consequence of this approach is the analysis of engine-out emissions.
Due to the reason that the raw emissions were in the focus of this research, no exhaust gas after-treatment came into action, while an adjustable flap generated the backpressure in the exhaust gas system. Figure 2 displays a schematic illustration of the experimental setup including a test engine, a variable nozzle turbine-turbocharger, an intercooler and an exhaust gas recirculation (EGR) cooler.
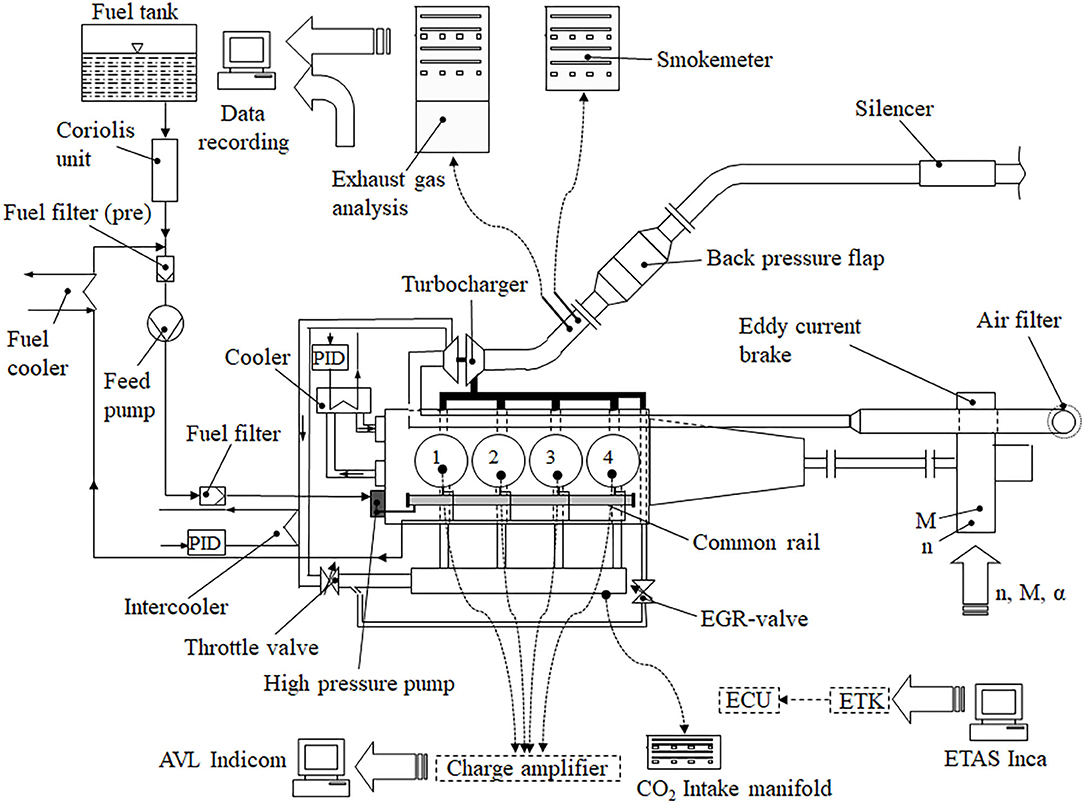
Figure 2. Experimental set up of the engine test bench (Moeltner et al., 2017).
Operation Points and Varied Parameters
The experimental procedure on the engine test bench was pursued by investigations of four different fuel blends compared to a fossil reference at different stationary operation points. With respect to the extent of this study, three operation points were selected for presentation and discussion within this contribution. Two operation points represent low load and medium load conditions and in one point, the engine operates at full load. For the low load operation point the investigations were conducted by varying the EGR rate and for medium load conditions the start of the main injection (SOI) was altered.
Figure 3 illustrates the presented operation points within the engine's overall operating map and Table 1 provides an overview of the variations and their respective ranges.
The variation of EGR was conducted by adjusting the intake air flow rate, whereby the total amount of combustion air was held constant. Moreover, the injected fuel quantity was modified for the torque to remain constant. Therefore, the boost pressure including the EGR valve position was adapted (Moeltner et al., 2017).
For operation points at comparable higher loads, a variation of SOI occurred, while other injection parameters, for instance, pilot injection, timing, or quantity, remained constant. The modification of fuel quantity during the main injection ensured the compensation for reoccurring fluctuations in torque (Moeltner et al., 2017).
For full-load operation of the engine, standard settings without variations were applied to identify the maximum power output and fuel consumption, which allows the determination of efficiency.
Fuels and Fuel Blends
The choice of fuel blends can be explained by the EU's targeted bio-quota. Furthermore, the compositions were chosen for a detailed investigation of the potential of increased BtL and HVO admixtures to fossil diesel. Instead of regular diesel (EN 590) CEC-reference diesel (European Committee for Coordination) was used to suppress any effects of already premixed (biogenous) substitutes. As a representative for the family of FAME, rapeseed methyl ester (RME) came into action, which is widely used as a biogenous substitute in Europe. The volumetric compositions of the investigated four fuel blends consisting of CEC, BtL, HVO, and FAME, are itemized below. Moreover, regular fossil diesel was tested as a reference.
• 50 vol-% CEC and 50 vol-% BtL,
• 80 vol-% CEC and 20 vol-% BtL,
• 90 vol-% CEC, 7 vol-% FAME, and 3 vol-% BtL,
• 90 vol-% CEC, 7 vol-% FAME, and 3 vol-% HVO,
• 100 vol-% CEC (fossil reference).
Discussion of Results
The section discussion of results includes a preliminary physicochemical analysis of the tested fuel blends and consecutively the results gathered on the engine test bench.
Physicochemical Analysis of Fuel Properties
In a preliminary step, physical and chemical properties of the pure test fuels were determined. This procedure later enables a coherent interpretation of emissions, combustion, and performance. The considered properties are:
• Composition of fuels (C/H/O),
• Share of aromatic compounds,
• Calorific value,
• Density,
• Viscosity number,
• Cetane number,
• Distillation curve.
Table 2 presents the results of the preliminary physicochemical analysis of the basic fuels and the respective test methods. Gravimetric composition were determined by an elementary analysis, total aromatics by gas chromatography, lower heating value (LHV) with a bomb calorimeter and the viscosity number by a rheometer.
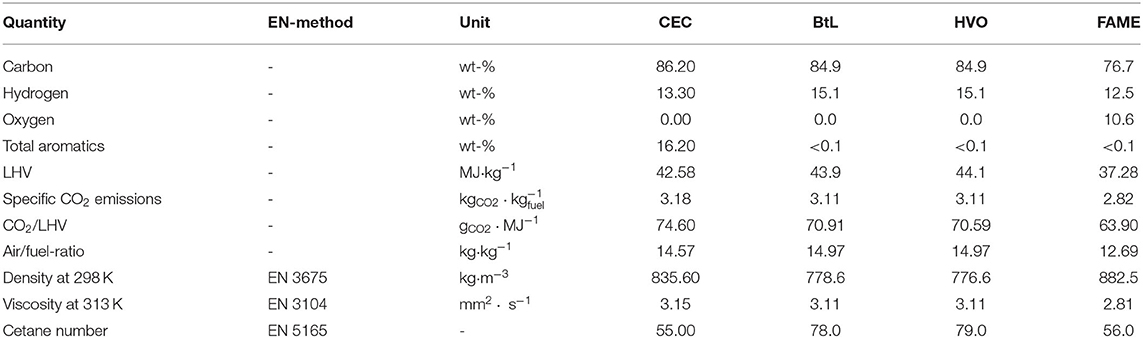
Table 2. Properties of pure test fuels (Moeltner et al., 2017).
The comparison of fuel composition delivers that BtL and HVO possess higher shares of hydrogen in comparison to CEC. This is traceable to the molecular structure of the dominating species, unbranched alkanes (CnH2n+2). In contrast to BtL and HVO, the CEC reference diesel consists also of alkenes (CnH2n) and aromatic compounds, and as a result, the hydrogen share is comparably lower than in paraffinic hydrocarbons. A particular role regarding the chemical composition play FAME. Due to their chemical structure containing a fatty acid bonded via an ester to a methyl-group, a FAME molecule consists of two atoms of oxygen. The composition of fuels, in particular the portion of hydrogen, strongly influences the LHV. The comparison of densities presents approximately 10% lowered density for both paraffinic fuels, BtL and HVO, and about 5% higher for FAME related to CEC. Viscosities of CEC, BtL, and HVO are in a similar range, the viscosity of FAME is slightly below. Generally, the viscosity of FAME depends on the raw material and is pre-set by the genetics of the seed.
Because the quality of mixture preparation greatly depends on the vapor pressures, and thus the boiling temperature of the fuel components, their distillation curves were determined in accordance to EN 3405 as shown in Figure 4. In order to additionally support the interpretation, several boiling temperatures (at 101325 Pa) for selected unilateral FAME (palmitic, oleic, and linoleic acid methyl ester) as well as alkanes (decane, dodecane, tetradecane, hexadecane, and octadecane) are highlighted in the diagram (Moeltner, 2018). A detailed consideration reveals information about possible compositions of the test fuels. The investigated FAME possess an initial boiling point (IBP) similar to palmitic acid, has a pronounced horizontal progress at the boiling temperature of oleic acid, which means that this species dominates in this FAME, and the final boiling point (FBP) seems to be set by linoleic acid methyl ester.
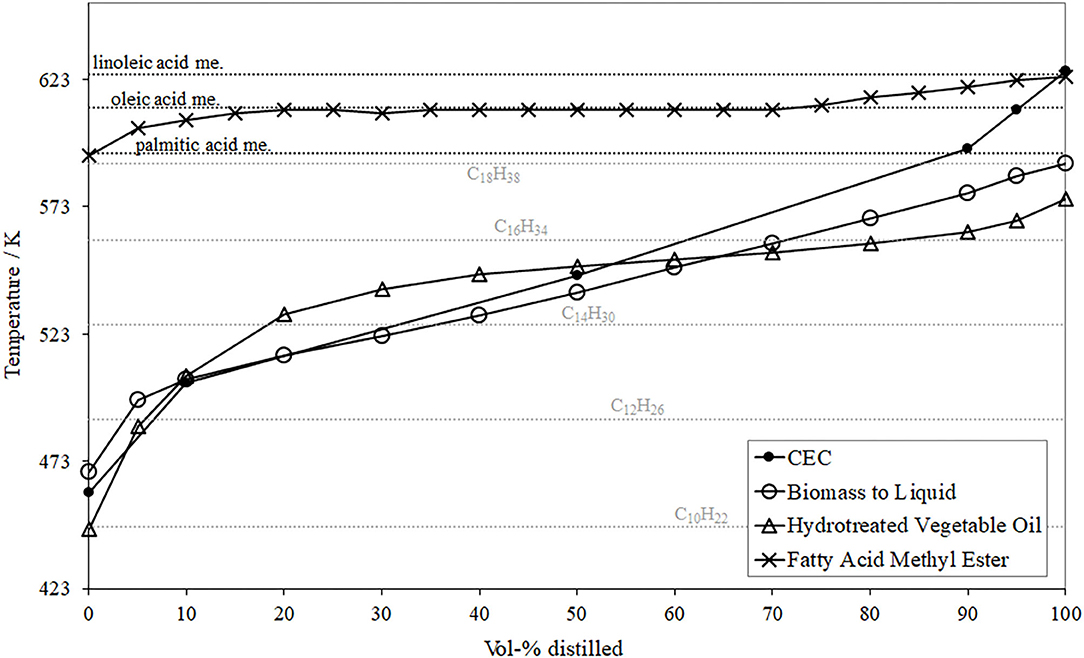
Figure 4. Distillation curves of the basic fuels prior blending (Moeltner et al., 2017).
A comparative consideration of both paraffinic test fuels, BtL and HVO, delivers that HVO consists of alkanes in a range from C10H22 to C17H36, while the investigated BtL exhibits a slightly higher boiling temperature, in a range between C11H24 and C18H38.
The results of this physicochemical analysis are supported by results of previous studies, published by Erkkilä et al. (2011); Jaroonjitsathian et al. (2014), and Hartikka and Kiiski (2012).
Engine Tests
The discussion of gained results during engine tests is subdivided into three sections; the first deals with an EGR-variation at low load operation, the second describes the variation of SOI at medium load and the last part is dedicated to the comparison of performance and efficiency at full load operation.
Low Load Operation
This operation point generates a power output of 12.5 kW at a speed of 1,500 min−1 and a BMEP of 5 bar.
Figure 5 presents the results of NOx- and PM-emissions for the conducted EGR-variation for all fuel blends as well as for the fossil reference. Firstly, the NOx-PM trade-off can clearly be attested to all test fuels, meaning that a decrease of NOx caused by higher EGR rates provokes an increase in PM.
The two test fuels containing solely shares of BtL present a mitigation of this trade-off. Particularly, the blend CEC (50 vol-%) and BtL (50 vol-%) features the potential to diminish both, PM and NOx, at high EGR. These results regarding admixtures containing BtL confirm previous surveys, e.g., Schaberg et al. (2002) and Larsson and Denbratt (2007).
The reductions in PM-emissions for both FAME blends, particularly at higher EGR-rates, can be explained by the bonded oxygen in FAME compounds as well as decreased content of total aromatics compared to fossil diesel. One possible explanation for the slight contrast between the two FAME blends can be found in the distinction of the third blend-component, HVO or BtL, and their already discussed difference in composition. In the case of pure CEC, the formation of particulate matter preferably occurs from unburnt aromatic hydrocarbons in a temperature range of 1,500–2,000 K. The increase of new soot particles can be explained by the following mechanisms, partial oxidation and/or thermal and oxidative pyrolysis of aromatics during rich conditions (Larsson and Denbratt, 2007; Moeltner et al., 2017). In the case of lower aromatics contents, insufficient mixture preparation after injection as well as diffusion-limited combustion can cause PM formation (Moeltner et al., 2017).
The two test fuels, containing shares of FAME, tend to increase NOx-emissions, which is commonly known as the “biodiesel NOx-effect”. This effect comprises several single factors such as ignition timing, ignition delay, adiabatic flame temperature, radiative heat loss, and other combustion phenomena (Zhang and Boehman, 2007; Moeltner et al., 2017).
Figure 6 presents the specific emissions of CO (upper segment in diagram) and THC (lower segment) and reveals a considerable increase in emissions with rising EGR rates. The explanation for this trend envelopes two aspects, the mixture preparation and the combustion itself. A co-consideration of the progress of CO- and THC-emissions reveals that even small shares of BtL, HVO, or FAME improve the mixture preparation caused by the lowered boiling temperatures (Figure 4) and thus contribute to decreased CO- and THC-emissions. Although, the FBP of FAME is increased in comparison to BtL and HVO, FAME can add to a reduction of CO- and THC-emissions particularly due to the bonded oxygen in esters of fatty acids. Incorporated oxygen promotes hydrocarbon oxidation and decreases local rich zones in the combustion chamber. Focusing on combustion, it can generally be argued that the available amount of oxygen strongly influences the post-oxidation of both, hydrocarbons and CO. Increased EGR reduces the availability of oxygen and thus supports the formation of THC and CO.
In terms of combustion, two parameters should be discussed, the ignition delay (IGD, lower segment in Figure 7) and the mass fraction burned, with 50% defining the center of combustion (AQ50, upper segment in Figure 7). Both parameters present a positive gradient as a function of increasing EGR rate, which implicates an overall decrease in the combustion chamber temperature and thus suppressed reaction rates.
A comparison between the investigated fuel blends reveals that for BtL blends this behavior diminishes, which is arguably due to the absence of aromatic compounds and their inherent reduced ignitibility; thus, higher cetane numbers can be achieved for BtL, as previously presented in Table 2. The FAME blends show similar tendencies as CEC diesel without any distinct differences.
The last issue to be discussed for the low load operation point includes the brake specific fuel consumption (BSFC), Figure 8. From a theoretic point of view, EGR can cause several effects, which influence the BSFC. The presence of exhaust gas in the combustion chamber, with its shares of CO2 and water, considerably decreases the reaction rates due to the comparably high heat capacities of CO2 and H2O. These lowered combustion kinetics can be traced by a delayed energy conversion and thus later crank angle positions of AQ50 with increased EGR, Figure 7. The slower combustion with high EGR causes higher fuel consumption along with decreased efficiency. Another contrary effect of an increased EGR is the reduction of wall heat dissipation due to decreased combustion temperatures. A further notable consequence of advanced EGR is the reduction in charge cycle losses, which results in a reduced fuel consumption and thus increased efficiency.
For the low load operation point and all test fuels, it can be stated that an increase in EGR effects higher BSFC. An EGR rate of ~20% causes a progressively worsen combustion, inducing a rise in THC- and CO-emissions, see also Figure 6.
A comparative consideration of the tested fuel blends and the CEC diesel reveals a lower BSFC for the binary fuel blends containing 20 or 50 vol-% of BtL. This behavior can be related to the improved ignitibility, which results in a shorter IGD as well as a faster combustion and thus in higher thermal efficiency, see Figure 7.
Both FAME blends effect a higher BSFC in comparison to its fossil pendant or the BtL blends. Due to the incorporated oxygen in the fatty acid methyl ester molecules, the calorific value is lower, see also Table 2.
Subsuming the results of the low load operation point, the investigated BtL blends exhibit an advantageous behavior in emissions as well as small improvements in BSFC. The ternary FAME blends partly show a slight reduction in PM but an increase in NOx. Emissions of THC and CO are mostly below the fossil reference and the combustion characteristics do not show any distinct differences to CEC diesel. The BSFC of the FAME blends is inherently higher due to the bonded oxygen in the ester. The presented results are in accordance with the outcome of a previously published study (Moeltner et al., 2017).
Medium Load Operation
The following section represents and discusses the results of a medium load operation point at an engine's speed of 3,000 min−1, 8 bar of BPME and a resulting power output of ~40 kW, also indicated in the operation map, Figure 3.
The varied parameter for this operation point is the start of the main injection in a range from 14° CA BTDC to TDC in steps of 2° CA. The variation of SOI leads to an expectable shift of combustion with all its implications on mixture preparation and furthermore, on emissions. Figure 9 impressively demonstrates the NOx-PM trade-off caused by the SOI variation. An early SOI positively effects the mixture preparation and thus induces a reduction in PM. The connoted negative aspect is a faster combustion, with higher temperatures, increased peak pressures, and an intensified combustion noise. These increased combustion temperatures promote the undesired formation of NOx via the thermal path introduced by Zeldovich.
A late SOI acts contrary, implicates a reduction of local temperature peaks, and thus leads to reduced NOx-emissions. However, lower combustion temperatures suppress the post-oxidation of already formed soot. A desirable decrease in PM compared to CEC diesel can be attested to all investigated fuel blends. Both BtL blends present a distinct reduction potential of PM-emissions, whereby an increased admixture of BtL enhances this potential. The ternary FAME blends also reduce PM-emissions because of their oxygen content.
Figure 10 displays the progress of CO (upper segment) and THC-emissions (lower segment). For the SOI variation at this medium load operation point, it can be expressed that the injection timing provokes only minor changes to CO and THC without any distinct tendencies.
However, differences between the test fuels are apparent. In particular, for BtL, both blends diminish the formation of CO and THC, which can be explained by an improved evaporation of the injected fuel due to the lower boiling point temperatures, see also Figure 4.
The results of the conducted combustion analysis are discussed by comparing—once again—the ignition delay and the center of combustion, Figure 11. As expected, the AQ50 strongly depends on the SOI, while the influence of the injection timing to the IGD only plays a minor role, with a slight tendency toward decreased IGD at earlier SOI. For both of these considered combustion parameters, the investigated fuel blends do not significantly affect the combustion.
A low BSFC in turn indicates a high efficiency, which can be achieved by a fast combustion and related high temperatures inside the combustion chamber including all negative side effects, e.g., pronounced thermal NOx formation or running noise, Figure 12. With the goal of high efficiency, the SOI should be set early, to ensure firstly an adequate mixture preparation and furthermore, to emphasize the premixed combustion phase rather than the consecutive relatively slow diffusion-controlled combustion. Considering this aspect, the decreasing efficiency caused by a late injection timing appears plausible.
However, comparing the test fuels reveals that the BtL blends feature the best fuel economy under these conditions. The reason for this effect can be found once again in the comparably high ignitibility underlined by the high cetane number of BtL.
Summarizing, beside of EGR, the SOI plays a major role for the combustion, for the formation of emissions, and for the efficiency. The binary fuel blends containing BtL present an attractive potential for simultaneously diminishing emissions and increasing engine's efficiency.
Fuels and Fuel Blends
The last operation point to be discussed in the consecutive section represents full load operation at medium speed. At a speed of 2,000 min−1 and a BMEP of 23.2 bar the test engine supplies a power output of 77 kW. During full load operation, the engine operates without EGR; this implicates that no variation was conducted. Furthermore, the evaluation of emissions is hardly expedient when considering the maximal possible power output and will thus not be discussed.
Figure 13 presents the percentage in maximum power output and the achieved efficiencies related to CEC diesel for all investigated fuel blends. Alternations in power output strongly correlate to the energy introduced to the combustion chamber, respectively to the amount of injected fuel. In a simple approximation, the mass flow of fuel throughout a constant free cross-intersection should follow a trend expressed by the square root of the pressure difference within the nozzle multiplied by the density of the fuel. Following this basic consideration the injected mass of fuel increases with higher density. That means, the relatively higher density of FAME blends results in an increased mass of fuel in the combustion chamber while the lower density of BtL and HVO inverts this effect. The low densities of BtL and HVO explain possible disadvantages without any adaptions on injection timing.
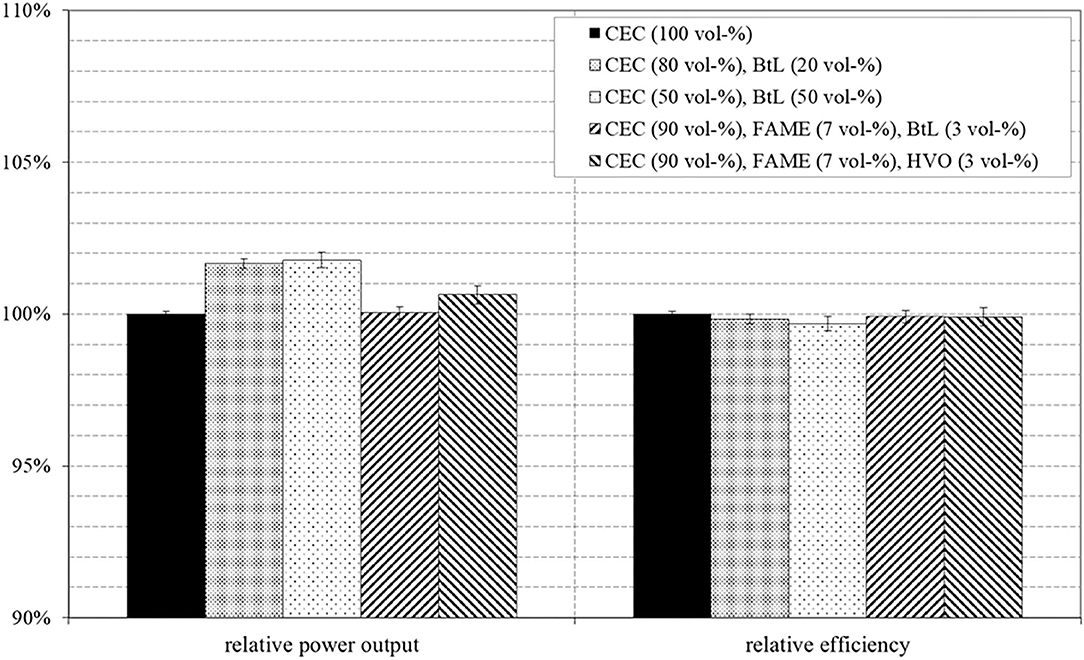
Figure 13. Relative power output and relative efficiency related to the results of pure CEC diesel, at 2,000 min−1 and 23.2 bar BMEP.
The co-consideration of the mass-related LHV possess the potential to turn these results, because BtL and HVO exhibit higher LHVs than CEC and FAME. The power output in Figure 13 widely correlates with the LHVs of the test fuels and overcompensate the effect of decreased mass of injected fuel by higher LHVs.
Furthermore, Millo et al., Wang et al., and Postrioti et al. describes a reduction in the injected volume to be a consequence of higher viscosities of RME compared to regular diesel (Postrioti et al., 2003; Wang et al., 2010; Millo et al., 2015). This argumentation is supported by the results presented in Figure 13, were the investigated binary fuel blends, in particular, the blend containing 50 vol-% BtL, increase the maximum power output. This behavior can be explained by the lower viscosity of BtL compared to CEC. The lower viscosity positively influences the flow through the injection nozzle holes, which in turn results in a larger injection quantity for an equal opening period. This effect was previously described by Pflaum et al. (2010).
The achieved efficiencies of the tested fuel blends are related to the efficiency of pure CEC diesel. The results reveal no clear differences among the determined efficiencies, only minor fluctuations, which might be evoked by measuring inaccuracies.
In summary, for the experiments at full load operation it can be attested that the maximum power output is slightly increased for both binary BtL blends. However, the comparison of efficiencies does not reveal distinct differences.
Conclusion
In the course of this study, four different fuels with biogenous shares were comparatively investigated with a particular focus on emissions and efficiency. The test fuels consisted of two blends containing BtL and CEC (20/80 and 50/50), another blend consisting of BtL/FAME/CEC (3/7/90) and one test fuel composed of HVO/FAME/CEC (3/7/90).
In order to allow a stringent interpretation of results gained during engine tests; in a preliminary step the most important physicochemical properties were determined for every pure component, e.g., distillation curves, LHV, cetane numbers, etc. BtL, as well as HVO, consists almost exclusively of n-paraffinic hydrocarbons, which feature chain lengths between C10 and C17 for HVO and from C12 to C18 for BtL. The investigated FAME was a rapeseed methyl ester; the distillation curve identifies oleic acid methyl ester as the dominating compound and minor shares of palmitic and linoleic acid methyl esters.
Engine tests were consecutively conducted by using a state-of-the-art, two-liter, inline-four, diesel engine mounted on an engine test bench equipped with systems for exhaust gas analysis and combustion diagnostics. The focus of the experiments was set to combustion and emission characteristics, therefore, no exhaust gas after-treatment system was utilized and simply engine-out emissions were considered.
The results, which were gathered during EGR-variation at low load operation, allow the conclusion that in particular, both BtL/CEC blends exhibit an advantageous behavior in emissions as well as slight improvements in efficiency. The two FAME blends with BtL and HVO partly provoke on the one hand a small reduction in PM but on the other hand an increase in NOx. The efficiency of the FAME blends is inherently higher due to the bonded oxygen in the ester and emissions of THC and CO mostly remain below CEC diesel. The combustion analysis does not present any clear tendencies for the ternary FAME blends, but a small trend to a slightly earlier AQ50 and hence faster combustion for binary BtL blends can be identified.
The variation of the SOI in a medium load operation point present for both binary BtL blends an attractive potential for reducing emissions and increasing engine's efficiency at the same time. The PM/NOx-trade off could be diminished and CO and HC emissions were successfully reduced. The FAME blends partly present also a reduction for CO and HC but drawbacks in BSFC.
For the experiments conducted at full load operation, it can be subsumed that the maximum power output is slightly increased for both binary BtL blends. This can be argued by an interplay of several factors, namely higher LHV, lower viscosity, and lower density compared to CEC diesel.
In general, the investigated fuel blends exhibit at least the technical potential to contribute to a reduction of dependency on fossil energy carriers, as wells as to comply with more and more stringent emission standards. Nevertheless, besides the mentioned technical potential other questions must be clarified, such as the creation of general frameworks for the production and distribution of raw materials or the final fuel components.
Data Availability Statement
The raw data supporting the conclusions of this article will be made available by the authors, without undue reservation.
Author's Note
Modern Diesel engines feature comparably high efficiencies coupled with favorable drivability; thus, also gained large market shares for passenger cars aside from the application in commercial vehicles. Due to the overarching goal to reduce carbon dioxide emissions escorted by the growing demand for independence from crude oil, blending with biofuels constitutes a promising path. Generally, biofuels are representatives of alternative fuels whereby fatty acid methyl esters (aka “biodiesel”) currently play the most important role as a mixing partner for fossil diesel. Although fatty acid methyl esters do not appear overly progressive besides more sophisticated advanced biofuels, e.g., biomass to liquid, hydrotreated vegetable oils, or polyoxymethylene dimethyl ethers, they still represent the backbone of biogenous substitution. This background aroused the intention of this study. In contrast to other publications, this manuscript does not exclusively describe the effects of pure biofuels or the role of one biofuel as a single mixing partner to fossil diesel. This work successfully evaluates how an admixture consisting of fatty acid methyl esters and small shares of advanced biofuels, namely biomass to liquid and hydrotreated vegetable oils, possess the potential to overcompensate or at least to mitigate inherent disadvantages of individual biofuels.
Author Contributions
All authors listed have made a substantial, direct and intellectual contribution to the work, and approved it for publication.
Conflict of Interest
The authors declare that the research was conducted in the absence of any commercial or financial relationships that could be construed as a potential conflict of interest.
Abbreviations
AQ50, center of combustion; BMEP, brake mean effective pressure; BSFC, brake specific fuel consumption; BTDC, before top dead center; BtL, biomass to liquid; CEC, Comité Européen de Coordination; CA, crank angle; CN, cetane number; CO, carbon monoxide; CO2, carbon dioxide; ECU, engine control unit; EGR, exhaust gas recirculation; FAME, fatty acid methyl ester; FBP, final boiling point; HC, hydrocarbons; HVO, hydrotreated vegetable oils, IBP, initial boiling point; IGD, ignition delay; NOx, nitrogen oxides; SOI, start of injection; TDC, top dead center; THC, total hydrocarbons; λ, air-fuel equivalence ratio.
References
Ballerini, D. (2012). Biofuels: Meeting the Energy and Environmental Challenges of the Transportation Sector. Paris: Editions Technip.
Bhardwaj, O., Kolbeck, A., Kkoerfer, T., and Honkanen, M. (2013). Potential of hydrogenated vegetable oil (HVO) in future high efficiency combustion system. SAE Int. J. Fuels Lubr. 6:13. doi: 10.4271/2013-01-1677
De Poures, M. V., Sathiyagnanam, A. P., Rana, D., Kumar, B. J., and Saravanan, S. (2017). 1-Hexanol as a sustainable biofuel in DI diesel engines and its effect on combustion and emissions under the influence of injection timing and exhaust gas recirculation (EGR). Appl. Thermal Eng. 113, 1505–1513. doi: 10.1016/j.applthermaleng.2016.11.164
De Poures, M. V., Sathiyagnanam, A. P., Rana, D., Kumar, B. J., Saravanan, S., and Sethuramasamyraja, B. (2019). Using renewable n-Octanol in a non-road diesel engine with some modifications. Energy Sources 41, 1194-1208 doi: 10.1080/15567036.2018.1544997
Erkkilä, K., Hulkkonen, T., Nylund, N. O., Tilli, A., Mikkonen, S., Saikkonen, P., et al. (2011). Emission Performance of Parrafinic HVO Diesel Fuel in Heavy Duty Vehicles. SAE Technical Paper Series 2011-01-1966. doi: 10.4271/2011-01-1966
European commission (2012). European commission Proposal to Amending Directive 98/70/EC Relating to the Quality of Petrol and Diesel Fuels and Amending Directive 200/28/EC on Promotion of the Use of Energy From Renewable Sources.
Hartikka, T., and Kiiski, U. (2012). Technical Performance of HVO (Hydrotreated Vegetable Oil) in Diesel Engines. SAE Technical Paper Series 2012-01-1585. doi: 10.4271/2012-01-1585
Heuser, B., Vauhkonen, V., Mannonen, S., Rohs, H., and Kolbeck, A. (2013). Crude tall oil-based renewable diesel as a blending component in passenger car diesel engines. SAE Int. J. Fuels Lubr. 6, 817–825. doi: 10.4271/2013-01-2685
Jaroonjitsathian, S., Saisirirat, P., Sivara, K., and Tongroon, M. (2014). Effects of GTL and HVO Blended Fuels on Combustion and Exhaust Emissions of a Common-Rail di Diesel Technology. SAE Technical Paper 2014-01-2763. doi: 10.4271/2014-01-2763
Kaliyaperumal, G., Sathiyagnanam, A. P., Kumar, B. J., Saravanan, S., Rana, D., and Sethuramasamyraja, B. (2018). Prediction of emissions and performance of a diesel engine fueled with n-octanol/diesel blends using response surface methodology. J. Clean. Produc. 184, 423–439. doi: 10.1016/j.jclepro.2018.02.204
Kumar, B., and Saravanan, S. (2015). Effect of iso-butanol addition to diesel fuel on performance and emissions of a DI diesel engine with exhaust gas recirculation. Proc. Inst. Mech. Eng. Part A. 230, 112–125. doi: 10.1177/0957650915617107
Kumar, B., and Saravanan, S. (2016). Use of higher alcohol biofuels in diesel engines: a review. Renew. Sust. Energy Rev. 60, 84–115. doi: 10.1016/j.rser.2016.01.085
Kumar, B. R., Saravanan, S., Rana, D., and Nagendran, A. (2016). A comparative analysis on combustion and emissions of some next generation higher-alcohol/diesel blends in a direct-injection diesel engine. Energy Convers. Manage. 119, 246–256. doi: 10.1016/j.enconman.2016.04.053
Larsson, M., and Denbratt, I. (2007). Combustion of Fischer-Tropsch, RME and Conventional Fuels in a Heavy-Duty Diesel Engine. SAE Technical Paper 2007-01-4009. doi: 10.4271/2007-01-4009
List, H. (2018). “Antriebssysteme im Wandel 39,” in Internationales Wiener Motorensymposium (Reihe: VDI Fortschritt-Berichte), 12.
Liu, D., Xu, H., Tian, J., Tan, C., and Li, Y. (2013). Cold and warm start characteristics using HVO and RME blends in a V6 diesel engine. SAE Int. J. Fuels Lubr. 6, 478–485. doi: 10.4271/2013-01-1306
Mandpe, S., Kadlaskar, S., Degen, W., and Keppeler, S. (2005). On Road Testing of Advanced Common Rail Diesel Vehicles With Biodiesel From the Jatropha Curcas Plant. SAE Technical Paper 2005-26-356. doi: 10.4271/2005-26-356
Millo, F., Debnath, B. K., Vlachos, T., Ciaravino, C., Postrioti, L., and Buitoni, G. (2015). Effects of different biofuels blends on performance and emissions of an automotive diesel engine. Fuel 159, 614–627. doi: 10.1016/j.fuel.2015.06.096
Mizushima, N., Kawano, D., Ishii, H., Takada, Y., and Sato, S. (2014). Evaluation of Real- World Emissions from Heavy-Duty Diesel Vehicle Fueled with FAME, HVO and BTL using PEMS. SAE Technical Paper 2014-01-2823. doi: 10.4271/2014-01-2823
Moeltner, L. (2018). Renewable Fuels, Advanced Combustion and Emission Control - Approaches to Fulfill Prospective Demands on Internal Combustion Engines. Habilitation Thesis at the Vienna University of Technology.
Moeltner, L., Konstantinoff, L., and Schallhart, V. (2017). Hydrotreated vegetable oils, biomass to liquid and fatty acid methyl ester as biogen admixtures for diesel engines in passenger cars. SAE Int. J. Fuels Lubr. 10, 340–345. doi: 10.4271/2017-01-9375
Ng, H., Biruduganti, M., and Stork, K. (2005). Comparing the Performance of SunDiesel™ and Conventional Diesel in a Light-Duty Vehicle and Heavy-Duty Engine. SAE Technical Paper 2005-01-3776. doi: 10.4271/2005-01-3776
Nicolas, R. (2017). Biofuels 2016 overview and general outlook report by IFPEN,” http://www.car-engineer.com/biofuels-2016-overview-general-outlook-ifpen/ (accessed July 13, 2018).
Oguma, M., Goto, S., Konno, M., Sugiyama, K., and Mori, M. (2002). Experimental Study of Direct Injection Diesel Engine Fueled with Two Types of Gas To Liquid (GTL). SAE Technical paper 2002-01-2691. doi: 10.4271/2002-01-2691
Pflaum, H., Hofmann, P., Geringer, B., and Weissel, W. (2010). Potential of Hydrogenated Vegetable Oil (HVO) in a Modern Diesel Engine. SAE Technical Paper 2010-32-0081.
Postrioti, L., Battistoni, M., Grimaldi, C. N., and Millo, F. (2003). Injection strategies tuning for the use of bio-derived fuels in a common rail HSDI diesel engine. SAE Transactions 112, 420–30. doi: 10.4271/2003-01-0768
Rantanen, R., Linnaila, R., Aakko, P., and Harju, T. (2005). NExBTL–Biodiesel fuel of the second generation. SAE Technical Paper Series 2005-01-3771. doi: 10.4271/2005-01-3771
Schaberg, P., Zarling, D., Waytulonis, R., and Kittelson, D. (2002). Exhaust Particle Number and Size Distributions With Conventional and Fischer-Tropsch Diesel Fuels. SAE Technical Paper 2002-01-2727.
Sugiyama„, K, Goto, I., Kitano, K., Mogi, K., and Honkanen, M. (2012). Effects of hydrotreated vegetable oil (HVO) as renewable diesel fuel on combustion and exhaust emissions in diesel engine. SAE Int. J. Fuels Lubr. 5, 205–217. doi: 10.4271/2011-01-1954
Tilli, A., Imperato, M., Aakko-Saksa, P., Larmi, M., Sarjovaara, T., and Honkanen, M. (2010). High Cetane Number Paraffinic Diesel Fuels and Emission Reduction in Engine Combustion. Cimac technical paper No. 26.
United States Environmental Protection Agency and Department of Transportation (2010a). Light-Duty Vehicle Greenhouse Gas Emission Standards and Corporate Average Fuel Economy Standards—Final Rule.
United States Environmental Protection Agency and Department of Transportation. (2010b). Renewable Fuel Standard Program (RFS2) Regulatory Impact Analysis.
Wang, X., Huang, Z., Kuti, O. A., Zhang, W., and Nishida, K. (2010). Experimental and analytical study on biodiesel and diesel spray characteristics under ultra-high injection pressure. Int. J. Heat Fluid Flow. 31, 659–666. doi: 10.1016/j.ijheatfluidflow.2010.03.006
Zhang, X., Li, L., Wu, Z., Hu, Z., and Zhou, Y. (2009). Material Compatibilities of Biodiesels with Elastomers, Metals and Plastics in a Diesel Engine. SAE Technical Paper 2009-01-2799.
Keywords: biodiesel, biofuels, biomass-to-liquid, hydrotreated vegetable oils, FAME
Citation: Moeltner L and Schallhart V (2020) Potential of Biomass to Liquid-, Hydrotreated Vegetable Oils-, and Fatty Acid Methyl Esters-Blends for Diesel Engines in Passenger Cars. Front. Mech. Eng. 6:576155. doi: 10.3389/fmech.2020.576155
Received: 25 June 2020; Accepted: 02 September 2020;
Published: 14 October 2020.
Edited by:
Tiegang Fang, North Carolina State University, United StatesCopyright © 2020 Moeltner and Schallhart. This is an open-access article distributed under the terms of the Creative Commons Attribution License (CC BY). The use, distribution or reproduction in other forums is permitted, provided the original author(s) and the copyright owner(s) are credited and that the original publication in this journal is cited, in accordance with accepted academic practice. No use, distribution or reproduction is permitted which does not comply with these terms.
*Correspondence: Lukas Moeltner, bHVrYXMubW9lbHRuZXJAbWNpLmVkdQ==