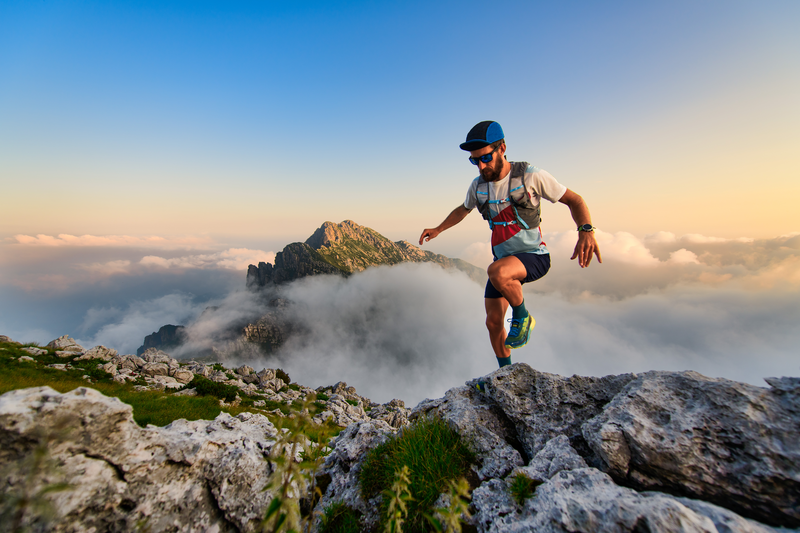
94% of researchers rate our articles as excellent or good
Learn more about the work of our research integrity team to safeguard the quality of each article we publish.
Find out more
MINI REVIEW article
Front. Mech. Eng. , 13 September 2019
Sec. Tribology
Volume 5 - 2019 | https://doi.org/10.3389/fmech.2019.00057
This article is part of the Research Topic Biotribology and Biotribocorrosion Properties of Implantable Biomaterials View all 5 articles
Osteoarthritis (OA) is a leading cause of disability in elderly individuals. As a common chronic degenerative joint disease, OA is typically characterized by articular cartilage degeneration, subchondral bone sclerosis, and concomitant synovium inflammation. As such, the structural and functional alterations in the articular cartilage and subchondral bone become the focus of research during progression of OA. Similarly, the molecular mechanism regulating articular cartilage lubrication and the cellular communication controlling metabolic status of subchondral bone cells promote innovative strategies for prevention and treatment of early stage OA. The current therapeutic options for OA are aimed at keeping the associated pain, inflammation, and degeneration of synovial joint tissues manageable in order to minimize the structural and symptomatic progression. These include, but are not limited to, synergetic therapy combining lubrication and drug intervention, regulatory balance between bone resorption and formation, and exercise therapy. In this mini review, we focus on the up-to-date research progress on these novel strategies for OA treatment.
Osteoarthritis (OA) is the most common chronic degenerative joint disease, which is typically characterized by articular cartilage degeneration, subchondral bone sclerosis, and synovium inflammation, and is the leading cause of disability in elderly individuals (Goldring and Goldring, 2010; Li et al., 2013). While all three synovial joint tissues are central components of OA development, there are other modifiable risk factors involved in the progression of this disease, including obesity, comorbidity, and injury (Georgiev and Angelov, 2019). However, despite the multifactorial disease origins, insights into its pathogenesis have been gained via the remarkable improvements in characterizing the structural and functional alterations in articular cartilage and subchondral bone during the progression of OA (Raman et al., 2018; Stewart and Kawcak, 2018). More importantly, there have also been significant achievements in elucidating the molecular mechanism regulating articular cartilage lubrication and the cellular communication controlling subchondral bone remodeling. These advances in understanding of disease pathogenesis make the prevention and treatment strategies for early stage OA (Luyten et al., 2012) possible to effectively minimize structural and symptomatic progression. In this mini review, we begin with a brief discussion of the pathogenesis and functional impairment of OA. Subsequently, we provide an overview of mechanisms of cartilage lubrication and bone remodeling, together with current progress of strategies for the treatment of early stage OA. Lastly, we give a perspective of future development of prevention and treatment strategies.
For the past few years, a better understanding of OA has progressed from the definition of primarily a non-inflammatory disease of articular cartilage to a broader concept of a low-grade inflammatory disease affecting all synovial joint tissues, such as articular cartilage, synovium as well as the underlying subchondral bone (Gupta et al., 2012; Barry and Murphy, 2013; Brown et al., 2019). As such, OA is considered as a degenerative whole-joint disease and all synovial joint tissues actively participate in the progression of this disease. Specifically, early stage OA is commonly associated with the alterations in the composition and organization of the cartilage matrix, which link to articular cartilage degeneration with concomitant lubrication deficiency (Goldring and Otero, 2011) and synovium inflammation (Scanzello and Goldring, 2012). For example, the abnormal synthesis of lubricin leads to an impaired cartilage lubrication while the degradation of collagen activates an inflammatory cascade within articular cartilage, causing low-grade synovium inflammation (Desrochers et al., 2013; Goldring and Berenbaum, 2015). In turn, synovium inflammation further contributes to articular cartilage disruption (Egloff et al., 2012). Meanwhile, subchondral bone deterioration in early stage OA due to an altered osteoblast metabolism occurs primarily in the region of articular cartilage degeneration and actively accelerates its destruction (Hayami et al., 2004). In fact, these synovial joint tissues afflicted with OA act synergistically, initiating a vicious circle to aggravate the disease. Additionally, recent studies have revealed OA as a multifactorial disease based on the fact that a cascade of mechanical and biological responses, which occur with age, obesity, and chronic injury, can destabilize the balance of degradation and synthesis within joint tissues and therefore also accelerate the development of this disease (Georgiev and Angelov, 2019).
As mentioned above, as a multifactorial whole-joint disease, OA is a major contributor to the pain and loss of functional capacity in elderly individuals. Its prevalence and incidence increase with age, for example, a majority of individuals over the age of 65 are afflicted with OA (Xia et al., 2014). Importantly, the tendency is progressively rising due to an increase in life expectancy and population aging together with obesity in the coming decades. The functional impairment of OA mainly involves activities of daily living related with the weight-bearing joints (hip, knee), such as walking and climbing stairs (Guccione et al., 1994). Individuals suffering from OA of weight-bearing joints have to live with joint pain and a limited range of motion for most of their lifetime (Corti and Rigon, 2003). However, pain and activity limitation may be intermediate steps over the course of OA, and disability in walking is the ultimate step necessitating total joint replacement. Accordingly, an insight into the OA pathology will facilitate the development of prevention and treatment strategies amenable to minimize the structural and symptomatic progression.
Insights into the molecular and cellular mechanisms separately regulating the function of articular cartilage and subchondral bone are essential to determine what therapeutic options can be applied for treatment of OA and to highlight tendency of future research. Consequently, in the following parts the mechanisms of articular cartilage lubrication and subchondral bone remodeling are briefly reviewed.
Articular cartilage is a remarkable self-lubricating system, which can actively maintain super-lubrication between two opposing sliding cartilage surfaces while bearing local high pressures over a wide range of shear rates (Zhu et al., 2017). Such remarkable lubrication has been systematically investigated for decades and theories relevant with lubrication of articular cartilage have been proposed based on fluid-film (McCutchen, 1959; Ateshian, 2009) or boundary lubrication (Schmidt et al., 2007; Sivan et al., 2010; Goldberg et al., 2011; Sorkin et al., 2014). Previously, a more detailed description of articular cartilage lubrication has been provided in a review by Jahn et al. (2016). For the purpose of this mini review, we focus on the recent findings on hydration lubrication mechanism in boundary lubrication of articular cartilage and its implication for therapeutics of OA. The hydration lubrication mechanism provides a framework for designing very efficient boundary lubrication systems, which relate to articular cartilage as the target in OA treatment. Hydration lubrication occurs in aqueous and biological media, where the hydration layers are tenaciously held by either ions or zwitterions they surround and thus avoid being squeezed out under compression. At the same time, they remain very rapidly relaxing and behave in a fluidlike response to shear (Gaisinskaya et al., 2012; Klein, 2013; Ma et al., 2015). Afterwards, more studies have been performed on cartilage lubrication in light of hydration lubrication mechanism (Sorkin et al., 2013, 2014). Amongst them, supramolecular synergy in boundary lubrication is considered to provide a realistic model for cartilage lubrication (Seror et al., 2015). Three key components of articular cartilage including hyaluronan, lubricin, and phosphatidylcholine lipid synergize in combination to achieve hydration lubrication at the exposed phosphocholine groups of the phosphatidylcholine lipid. As a consequence, a remarkable lubrication emerges with shear of such hydration layers surrounding the phosphocholine groups.
Under physiological conditions, bone remodeling continuously maintains the metabolic homeostasis and structural integrity of subchondral bone via a precise coordination of bone resorption and subsequent bone formation (Kwan Tat et al., 2010). Disturbances of this process gives rise to subchondral bone abnormalities, including bone loss and sclerosis, osteophyte formation in the initiation and progression of OA (Zaidi, 2007; Burr and Gallant, 2012; Castaneda et al., 2012). Accordingly, an understanding of the mechanism behind subchondral bone remodeling is essential for the inhibition of OA progression. Previous research finding has demonstrated that cellular communication at the level of progenitors through receptor activator of nuclear factor (NF)-kB (RANK) and RANK ligand (RANKL) functions to couple bone resorption and formation in the remodeling of subchondral bone (Suda et al., 1999). Subsequently, identifications of matricellular communication through active transforming growth factor-β1 (TGF-β1) or probably insulin-like growth factor-1 (IGF-1) (Tang et al., 2009; Qiu et al., 2010; Crane and Cao, 2014), and osteoclast-osteoblast communication through semaphoring 4D (Sema4D) and Plexin-B1 have also added new levels to cellular communication coupling bone resorption and formation (Negishi-Koga et al., 2011). Those different levels of cellular communication regulate bone remodeling and adapt subchondral bone to the alterations in local environmental and systemic factors during the initiation and progression of OA. Particularly, they provide an implication for drug intervention with coupling mechanism of action to repair subchondral bone abnormalities. In line with this idea, factors capable of influencing bone remodeling can be targeted as therapeutics for treatment of subchondral bone in individuals with OA.
Current strategies for prevention and treatment of OA strive toward decreasing joint pain while simultaneously restoring joint function, therefore delaying surgery therapy as long as possible. In view of this goal, novel strategies for OA treatment have yielded a multitude of options, such as synergetic therapy combining both lubrication and drug intervention, regulatory balance between bone resorption and formation, and exercise therapy (Figure 1). In this section, we will focus on the recent achievement of the above-mentioned three therapeutic options in OA treatment.
Figure 1. Current strategies for OA treatment. The strategies mainly include synergetic therapy combining both lubrication and drug intervention, regulatory balance between bone resorption and formation, and exercise therapy (Roos and Arden, 2016), Copyright 2015, Macmillan Publishers Limited. In the case of synergetic therapy, the dual-functional drug-loaded nanoparticles achieve simultaneously both enhanced lubrication on the outer hydration layer and sustained drug release in the inner core. Regarding regulatory balance between bone resorption and formation, coupling bone remodeling functions via three different levels of cellular communication, including (a) osteoclast progenitor-mesenchymal stem cell (MSC)/osteocyte communication through RANK–RANKL, (b) matricellular communication through TGF-β1 or IGF-1, and (c) osteoclast-osteoclast communication through Sema4D–Plexin-B1. Exercise therapy is intended for modifiable OA risk factors and mainly includes neuromuscular exercise, strength training, and aerobic exercise.
Synergetic therapy represents an innovative and optimized strategy, which can achieve simultaneously enhanced lubrication and sustained local drug release in OA treatment (Liu et al., 2014a,b; Sun et al., 2017). Compared with current clinical interventions including for example intra-articular injection of viscous hyaluronan and oral administration of anti-inflammatory or chondroprotective drugs, the synergetic therapy is likely to provide long-term joint lubrication without shear-thinning nature of hyaluronan in response to shear, and at the same time limit systemic toxicity associated with oral drug delivery. The focus of synergetic therapy is the intra-articularly injectable lubricating drug-loaded nanoparticles, which can be constructed by either modifying the drug nanocarriers with efficient lubricating materials such as polymer brushes and phosphocholine lipids or directly constructing self-lubricating polymer/liposome-based nanocarriers, further encapsulating target drugs of interest (Figure 2). Currently, the therapeutic drugs for OA treatment are predominantly small molecules (<10 kDa) that can provide symptomatic improvement or disease-modifying therapy. Small molecules available for symptomatic pain relief have been developed, including analgesics (e.g., paracetamol) (Ong et al., 2010), nonsteroidal anti-inflammatory drugs (NSAIDs) (e.g., diclofenac) (da Costa et al., 2017), and specific cyclooxygenase (COX)-2 inhibitors (e.g., celecoxib) (Laine et al., 2008). However, most of the small molecules available for disease-modifying therapy, that is, disease-modifying OA drugs (DMOADs) that can inhibit symptomatic and structural OA progression and ideally also improve joint function, are being investigated in ongoing clinical trials (Maudens et al., 2018). Based on these clinical trials, some DMOADs targeting articular cartilage have preliminarily demonstrated efficacy in stimulating chondrogenesis, such as transcription factor CBFβ (kartogenin) (Johnson et al., 2012) and Wnt/β-catenin inhibitor (SM04690) (Deshmukh et al., 2018). As a consequence, current synergetic therapy intervention has been limited to targeting pain relief, synovium inflammation and/or articular cartilage degeneration besides lubrication improvement. When intra-articularly injected into joints afflicted with OA, it is anticipated that aside from local sustained drug release to stabilize or revert disease progression, the dual-functional lubricating drug-loaded nanoparticles resembling phosphatidylcholine lipid at the articular cartilage surface can also provide enhanced lubrication via shear of hydration layers, which surround the grafted polymer brushes or exposed phosphocholine groups on the outer surface of the nanoparticles. In addition, the approaches for constructing lubricating drug-loaded nanoparticles do not involve tedious synthesis steps and toxic catalysts during the synthetic process, thereby allowing for easy popularization. For example, a previous study performed by Zhang et al. has revealed that phospholipids are readily coated onto the surface of mesoporous silica nanoparticles (MSNs) to produce the lubricating drug nanocarriers (MSNs@lip), which can greatly reduce the friction coefficient of the Ti6Al4V-polyethylene contact pair and release drugs over a prolonged period (Sun et al., 2018). Furthermore, more recent research findings have shown that, apart from enhanced lubrication, the sustained drug release of dual-functional lubricating drug-loaded nanoparticles accelerated the viability and proliferation of chondrocytes while providing the stable anti-inflammatory and chondroprotective effects through in vitro and in vivo experiments (Fan et al., 2018; Ji et al., 2019; Yan et al., 2019). These studies demonstrate the therapeutic efficacy of the intra-articularly injectable lubricating drug-loaded nanoparticles in the preclinical research, directing more attention to the investigation and development of various dual-functional nanoparticles. Accordingly, despite the voids in the current clinical trials as to lubricating drug-loaded nanoparticles, synergetic therapy integrating both enhanced lubrication and drug intervention will likely remain a central tenet of OA treatment.
Figure 2. Construction of typical intra-articularly injectable lubricating drug-loaded nanoparticles for OA treatment. Such dual-functional nanoparticles include (A) polymer brush-grafted MSNs with encapsulation of drugs (Yan et al., 2019), Copyright 2018, Wiley-Vch Verlag GmbH and Co. KGaA, Weinheim. (B) Self-lubricating polymer-based nanospheres encapsulated with drugs, and (C) drug-loaded liposomes (Ji et al., 2019), Copyright 2019, the Royal Society of Chemistry.
The therapeutic strategies based on regulatory balance between bone resorption and formation refer to novel approaches targeting bone diseases associated with increased resorption (Castaneda et al., 2012). Over the past decades, therapeutics available for the treatment of subchondral bone in individuals with OA are mainly concentrated on either inhibition of osteoclast activity or stimulation of osteoblast activity. Correspondingly, various agents capable of influencing the activities of osteoclasts or osteoblasts have been extensively developed. These agents include anti-resorptive agents (e.g., estrogens, calcitonin and bisphosphonates; Ham et al., 2002; Drake et al., 2008; Nielsen et al., 2011) as well as bone-forming agents (e.g., parathyroid hormone; Bellido et al., 2011). However, a growing number of studies have now demonstrated that their therapeutic efficacy is compromised due to the concomitant side effects. For example, inhibition of osteoclastic bone resorption with anti-resorptive agents can cause “frozen bone,” which greatly decreases bone quality and increases skeletal fragility (Reid, 2009). Under the circumstance, a novel therapeutic strategy in light of the recently emerging coupling mechanism of bone resorption and formation (Cao, 2011; Wan et al., 2012; Zhen and Cao, 2014; Sims and Martin, 2015; Xie et al., 2016) would be an ideal approach to treat subchondral bone as a target in OA. Many research findings have shown that TGF-β1 and IGF-1 in the bone matrix function to couple bone resorption and formation, and modulation of their activities can be an effective prevention and treatment for OA (Zhen et al., 2013; Xu et al., 2015; Cui et al., 2016; Zheng et al., 2018). Likewise, activity modulations of RANKL and Sema4D in other two cellular communications can also regulate bone resorption and formation. For example, the anti-RANKL antibody can be targeted to the pro-resorptive effect of RANKL, which stimulates osteoclastogenesis by binding its cell membrane receptor RANK on osteoclast progenitors (Bi et al., 2017). Particularly, Sema4D as a potential target for the development of new therapeutics for OA treatment has recently been highlighted due to the fact that osteoclast-osteoblast communication mediated by Sema4D and Plexin-B1 can integrate with matricellular signaling of TGF-β1 and IGF-1 to spatiotemporally couple bone resorption and bone formation (Cao, 2011). As a consequence, regulatory balance between bone resorption and formation can inhibit OA progression by altering the activity of coupling factors and thus serve as therapeutic approaches for the management of subchondral bone disease.
Exercise therapy, which is defined as a regimen or plan of physical activities designed and prescribed for specific therapeutic goals, has currently been recommended as first-line treatment of OA (Roos and Juhl, 2012; Roos and Arden, 2016; Dadabo et al., 2019). Broadly, the goals of exercise therapy program are pain reduction and restoration of musculoskeletal function by strengthening muscle, improving joint stability, increasing range of motion, and improving aerobic fitness. Within this framework, we primarily discuss three activities described as exercise therapy program, including neuromuscular exercise, strength training, and aerobic exercise. These activities have strong evidence supporting their use in the treatment of OA (Escalante et al., 2011; Fransen et al., 2015; Roos and Arden, 2016).
Neuromuscular exercise therapy, which is based on biomechanical and neuromuscular principles, has been developed specifically to target the sensorimotor deficiencies and functional instability associated with knee injury (Ageberg and Roos, 2015; Roos and Arden, 2016; Skou and Thorlund, 2018). Because similar deficiencies in sensorimotor function and perceptive instability have also been observed in individuals with OA, neuromuscular exercise therapy can be transferable to OA treatment (Svege et al., 2011; Stensrud et al., 2012). A typical evidence obtained from a study in 2010 has shown that neuromuscular exercise therapy is feasible in older individuals with OA (Ageberg et al., 2010). Importantly, it is the first neuromuscular exercise therapy developed for use in individuals with OA. Additionally, neuromuscular exercise has also been reported to improve cartilage matrix content in the middle-aged individuals with partial medial meniscectomy (Roos and Dahlberg, 2005). This finding suggests that neuromuscular exercise may be targeted to the prevention of OA in the knee joint.
Strength training is a local exercise therapy, which mainly targets to muscle weakness by increasing muscle strength and mass (Lange et al., 2008). As muscle weakness of the lower extremity is very common in individuals with knee OA, strength training has become the cornerstone of exercise therapy in the treatment of OA (Roos et al., 2011; Thorlund et al., 2012). Strong supporting evidence has shown that strength training can benefit individuals with OA in terms of reduced pain and improved physical function (Latham and Liu, 2010).
Aerobic exercise is a safe and effective exercise therapy intervention, characterized by sustained physical activity that requires oxidative metabolism for energy generation (Escalante et al., 2011; Juhl et al., 2014). There are various forms of activities that fall within the category of aerobic exercise, such as jogging, cycling, and swimming. Among them, walking and swimming are two accessible forms of land-based and aquatic-based aerobic exercise (Wyatt et al., 2001; Roddy et al., 2005). Moreover, research findings have demonstrated that aquatic aerobics are more effective in pain relief and function improvement for individuals with OA, which is due to the fact that the buoyancy of water can reduce weight-bearing stress on the joints (Fransen et al., 2015).
As described above, while three different types of exercises have similar pain-relieving effects, they differ in function improvement for individuals with OA. As a consequence, exercise therapy intervention should target to individuals with specific exercise, rather than enforcing homogeneous strategies across the whole population.
An increasing number of research findings have identified the therapeutic efficacy of novel strategies for the prevention and treatment of OA. These therapeutic approaches preliminarily accomplish decreasing joint pain while simultaneously improving joint function, thus limiting functional impairment. However, in order to obtain the optimal therapeutic efficacy, more intensive studies are required to optimize synergetic therapy intervention and to demonstrate acceptable safety for transformation into the clinic. In particular, synergetic therapy can be considered to integrate with therapeutic strategy in light of coupling of bone resorption and formation, so that abnormalities in articular cartilage and subchondral bone are repaired concurrently. Beyond that, exercise therapy program should be tailored to individuals with OA.
HZ outlined the structure of this review. XJ and HZ completed this review and drafted the manuscript.
This study was financially supported by National Natural Science Foundation of China (grant no. 51675296), Ng Teng Fong Charitable Foundation (grant no. 202-276-132-13), and Research Fund of State Key Laboratory of Tribology, Tsinghua University, China (grant no. SKLT2018B08).
The authors declare that the research was conducted in the absence of any commercial or financial relationships that could be construed as a potential conflict of interest.
Ageberg, E., Link, A., and Roos, E. M. (2010). Feasibility of neuromuscular training in patients with severe hip or knee OA: the individualized goal-based NEMEX-TJR training program. BMC Musculoskelet. Disord. 11:126. doi: 10.1186/1471-2474-11-126
Ageberg, E., and Roos, E. M. (2015). Neuromuscular exercise as treatment of degenerative knee disease. Exerc. Sport Sci. Rev. 43, 14–22. doi: 10.1249/JES.0000000000000030
Ateshian, G. A. (2009). The role of interstitial fluid pressurization in articular cartilage lubrication. J. Biomech. 42, 1163–1176. doi: 10.1016/j.jbiomech.2009.04.040
Barry, F., and Murphy, M. (2013). Mesenchymal stem cells in joint disease and repair. Nat. Rev. Rheumatol. 9, 584–594. doi: 10.1038/nrrheum.2013.109
Bellido, M., Lugo, L., Roman-Blas, J. A., Castaneda, S., Calvo, E., Largo, R., et al. (2011). Improving subchondral bone integrity reduces progression of cartilage damage in experimental osteoarthritis preceded by osteoporosis. Osteoarthr. Cartilage 19, 1228–1236. doi: 10.1016/j.joca.2011.07.003
Bi, H., Chen, X., Gao, S., Yu, X., Xiao, J., Zhang, B., et al. (2017). Key triggers of osteoclast-related diseases and available strategies for targeted therapies: a review. Front. Med. 4:234. doi: 10.3389/fmed.2017.00234
Brown, S., Kumar, S., and Sharma, B. (2019). Intra-articular targeting of nanomaterials for the treatment of osteoarthritis. Acta Biomater. 93, 239–257. doi: 10.1016/j.actbio.2019.03.010
Burr, D. B., and Gallant, M. A. (2012). Bone remodelling in osteoarthritis. Nat. Rev. Rheumatol. 8, 665–673. doi: 10.1038/nrrheum.2012.130
Cao, X. (2011). Targeting osteoclast-osteoblast communication. Nat. Med. 17, 1344–1346. doi: 10.1038/nm.2499
Castaneda, S., Roman-Blas, J. A., Largo, R., and Herrero-Beaumont, G. (2012). Subchondral bone as a key target for osteoarthritis treatment. Biochem. Pharmacol. 83, 315–323. doi: 10.1016/j.bcp.2011.09.018
Corti, M. C., and Rigon, C. (2003). Epidemiology of osteoarthritis prevalence, risk factors and functional impact. Aging Clin. Exp. Res. 15, 359–363. doi: 10.1007/BF03327356
Crane, J. L., and Cao, X. (2014). Function of matrix IGF-1 in coupling bone resorption and formation. J. Mol. Med. 92, 107–115. doi: 10.1007/s00109-013-1084-3
Cui, Z., Crane, J., Xie, H., Jin, X., Zhen, G., Li, C., et al. (2016). Halofuginone attenuates osteoarthritis by inhibition of TGF-β activity and H-type vessel formation in subchondral bone. Ann. Rheum. Dis. 75, 1714–1721. doi: 10.1136/annrheumdis-2015-207923
da Costa, B. R., Reichenbach, S., Keller, N., Nartey, L., Wandel, S., Jüni, P., et al. (2017). Effectiveness of non-steroidal anti-inflammatory drugs for the treatment of pain in knee and hip osteoarthritis: a network meta-analysis. Lancet 390, e21–e33. doi: 10.1016/S0140-6736(17)31744-0
Dadabo, J., Fram, J., and Jayabalan, P. (2019). Noninterventional therapies for the management of knee osteoarthritis. J. Knee Surg. 32, 46–54. doi: 10.1055/s-0038-1676107
Deshmukh, V., Hu, H., Barroga, C., Bossard, C., Kc, S., Dellamary, L., et al. (2018). A small-molecule inhibitor of the Wnt pathway (SM04690) as a potential disease modifying agent for the treatment of osteoarthritis of the knee. Osteoarthr. Cartilage 26, 18–27. doi: 10.1016/j.joca.2017.08.015
Desrochers, J., Amrein, M. W., and Matyas, J. R. (2013). Microscale surface friction of articular cartilage in early osteoarthritis. J. Mech. Behav. Biomed. Mater. 25, 11–22. doi: 10.1016/j.jmbbm.2013.03.019
Drake, M. T., Clarke, B. L., and Khosla, S. (2008). Bisphosphonates: mechanism of action and role in clinical practice. Mayo Clin. Proc. 83, 1032–1045. doi: 10.4065/83.9.1032
Egloff, C., Hugle, T., and Valderrabano, V. (2012). Biomechanics and pathomechanisms of osteoarthritis. Swiss Med. Wkly. 142:w13583. doi: 10.4414/smw.2012.13583
Escalante, Y., Garcia-Hermoso, A., and Saavedra, J. M. (2011). Effects of exercise on functional aerobic capacity in lower limb osteoarthritis: a systematic review. J. Sci. Med. Sport 14, 190–198. doi: 10.1016/j.jsams.2010.10.004
Fan, Z., Li, J., Liu, J., Jiao, H., and Liu, B. (2018). Anti-inflammation and joint lubrication dual effects of a novel hyaluronic acid/curcumin nanomicelle improve the efficacy of rheumatoid arthritis therapy. ACS Appl. Mater. Interfaces 10, 23595–23604. doi: 10.1021/acsami.8b06236
Fransen, M., McConnell, S., Harmer, A. R., Van der Esch, M., Simic, M., and Bennell, K. L. (2015). Exercise for osteoarthritis of the knee: a Cochrane systematic review. Br. J. Sports Med. 49, 1554–1557. doi: 10.1136/bjsports-2015-095424
Gaisinskaya, A., Ma, L., Silbert, G., Sorkin, R., Tairy, O., Goldberg, R., et al. (2012). Hydration lubrication: exploring a new paradigm. Faraday Discuss. 156, 217–233. doi: 10.1039/c2fd00127f
Georgiev, T., and Angelov, A. K. (2019). Modifiable risk factors in knee osteoarthritis: treatment implications. Rheumatol. Int. 39, 1145–1157. doi: 10.1007/s00296-019-04290-z
Goldberg, R., Schroeder, A., Silbert, G., Turjeman, K., Barenholz, Y., and Klein, J. (2011). Boundary lubricants with exceptionally low friction coefficients based on 2D close-packed phosphatidylcholine liposomes. Adv. Mater. 23, 3517–3521. doi: 10.1002/adma.201101053
Goldring, M. B., and Berenbaum, F. (2015). Emerging targets in osteoarthritis therapy. Curr. Opin. Pharmacol. 22, 51–63. doi: 10.1016/j.coph.2015.03.004
Goldring, M. B., and Goldring, S. R. (2010). Articular cartilage and subchondral bone in the pathogenesis of osteoarthritis. Ann. N.Y. Acad. Sci. 1192, 230–237. doi: 10.1111/j.1749-6632.2009.05240.x
Goldring, M. B., and Otero, M. (2011). Inflammation in osteoarthritis. Curr. Opin. Rheumatol. 23, 471–478. doi: 10.1097/BOR.0b013e328349c2b1
Guccione, A. A., Felson, D. T., Anderson, J. J., Anthony, J. M., Zhang, Y., Wilson, P. W. F., et al. (1994). The effects of specific medical conditions on functional limitations of elders in the Framingham Study. Am. J. Public Health 84, 354–358. doi: 10.2105/AJPH.84.3.351
Gupta, P. K., Das, A. K., Chullikana, A., and Majumdar, A. S. (2012). Mesenchymal stem cells for cartilage repair in osteoarthritis. Stem Cell Res. Ther. 3:25. doi: 10.1186/scrt116
Ham, K. D., Loeser, R. F., Lindgren, B. R., and Carlson, C. S. (2002). Effects of long-term estrogen replacement therapy on osteoarthritis severity in cynomolgus monkeys. Arthritis Rheum. 46, 1956–1964. doi: 10.1002/art.10406
Hayami, T., Pickarski, M., Wesolowski, G. A., McLane, J., Bone, A., Destefano, J., et al. (2004). The role of subchondral bone remodeling in osteoarthritis: reduction of cartilage degeneration and prevention of osteophyte formation by alendronate in the rat anterior cruciate ligament transection model. Arthritis Rheum. 50, 1193–1206. doi: 10.1002/art.20124
Jahn, S., Seror, J., and Klein, J. (2016). Lubrication of articular cartilage. Annu. Rev. Biomed. Eng. 18, 235–258. doi: 10.1146/annurev-bioeng-081514-123305
Ji, X., Yan, Y., Sun, T., Zhang, Q., Wang, Y., Zhang, M., et al. (2019). Glucosamine sulphate-loaded distearoyl phosphocholine liposomes for osteoarthritis treatment: combination of sustained drug release and improved lubrication. Biomater. Sci. 7, 2716–2728. doi: 10.1039/C9BM00201D
Johnson, K., Zhu, S., Tremblay, M. S., Payette, J. N., Wang, J., Bouchez, L. C., et al. (2012). A stem cell-based approach to cartilage repair. Science 336, 717–721. doi: 10.1126/science.1215157
Juhl, C., Christensen, R., Roos, E. M., Zhang, W., and Lund, H. (2014). Impact of exercise type and dose on pain and disability in knee osteoarthritis: a systematic review and meta-regression analysis of randomized controlled trials. Arthritis Rheumatol. 66, 622–636. doi: 10.1002/art.38290
Kwan Tat, S., Lajeunesse, D., Pelletier, J. P., and Martel-Pelletier, J. (2010). Targeting subchondral bone for treating osteoarthritis: what is the evidence? Best Pract. Res. Clin. Rheumatol. 24, 51–70. doi: 10.1016/j.berh.2009.08.004
Laine, L., White, W. B., Rostom, A., and Hochberg, M. (2008). COX-2 selective inhibitors in the treatment of osteoarthritis. Semin. Arthritis Rheum. 38, 165–187. doi: 10.1016/j.semarthrit.2007.10.004
Lange, A. K., Vanwanseele, B., and Fiatarone Singh, M. A. (2008). Strength training for treatment of osteoarthritis of the knee: a systematic review. Arthritis Rheum. 59, 1488–1494. doi: 10.1002/art.24118
Latham, N., and Liu, C. J. (2010). Strength training in older adults: the benefits for osteoarthritis. Clin. Geriatr. Med. 26, 445–459. doi: 10.1016/j.cger.2010.03.006
Li, G., Yin, J., Gao, J., Cheng, T. S., Pavlos, N. J., Zhang, C., et al. (2013). Subchondral bone in osteoarthritis: insight into risk factors and microstructural changes. Arthritis Res. Ther. 15:223. doi: 10.1186/ar4405
Liu, G., Cai, M., Zhou, F., and Liu, W. (2014a). Charged polymer brushes-grafted hollow silica nanoparticles as a novel promising material for simultaneous joint lubrication and treatment. J. Phys. Chem. B 118, 4920–4931. doi: 10.1021/jp500074g
Liu, G., Liu, Z., Li, N., Wang, X., Zhou, F., and Liu, W. (2014b). Hairy polyelectrolyte brushes-grafted thermosensitive microgels as artificial synovial fluid for simultaneous biomimetic lubrication and arthritis treatment. ACS Appl. Mater. Interfaces 6, 20452–20463. doi: 10.1021/am506026e
Luyten, F. P., Denti, M., Filardo, G., Kon, E., and Engebretsen, L. (2012). Definition and classification of early osteoarthritis of the knee. Knee Surg. Sports Traumatol. Arthrosc. 20, 401–406. doi: 10.1007/s00167-011-1743-2
Ma, L., Gaisinskaya-Kipnis, A., Kampf, N., and Klein, J. (2015). Origins of hydration lubrication. Nat. Commun. 6:6060. doi: 10.1038/ncomms7060
Maudens, P., Jordan, O., and Allemann, E. (2018). Recent advances in intra-articular drug delivery systems for osteoarthritis therapy. Drug Discov. Today 23, 1761–1775. doi: 10.1016/j.drudis.2018.05.023
McCutchen, C. W. (1959). Mechanism of animal joints sponge-hydrostatic and weeping bearings. Nature 184, 1284–1285. doi: 10.1038/1841284a0
Negishi-Koga, T., Shinohara, M., Komatsu, N., Bito, H., Kodama, T., Friedel, R. H., et al. (2011). Suppression of bone formation by osteoclastic expression of semaphorin 4D. Nat. Med. 17, 1473–1480. doi: 10.1038/nm.2489
Nielsen, R. H., Bay-Jensen, A. C., Byrjalsen, I., and Karsdal, M. A. (2011). Oral salmon calcitonin reduces cartilage and bone pathology in an osteoarthritis rat model with increased subchondral bone turnover. Osteoarthr. Cartilage 19, 466–473. doi: 10.1016/j.joca.2011.01.008
Ong, C. K., Seymour, R. A., Lirk, P., and Merry, A. F. (2010). Combining paracetamol (acetaminophen) with nonsteroidal antiinflammatory drugs: a qualitative systematic review of analgesic efficacy for acute postoperative pain. Anesth. Analg. 110, 1170–1179. doi: 10.1213/ANE.0b013e3181cf9281
Qiu, T., Wu, X., Zhang, F., Clemens, T. L., Wan, M., and Cao, X. (2010). TGF-β type II receptor phosphorylates PTH receptor to integrate bone remodelling signalling. Nat. Cell Biol. 12, 224–234. doi: 10.1038/ncb2022
Raman, S., FitzGerald, U., and Murphy, J. M. (2018). Interplay of inflammatory mediators with epigenetics and cartilage modifications in osteoarthritis. Front. Bioeng. Biotechnol. 6:22. doi: 10.3389/fbioe.2018.00022
Reid, I. R. (2009). Osteonecrosis of the jaw: who gets it, and why? Bone 44, 4–10. doi: 10.1016/j.bone.2008.09.012
Roddy, E., Zhang, W., and Doherty, M. (2005). Aerobic walking or strengthening exercise for osteoarthritis of the knee? A systematic review. Ann. Rheum. Dis. 64, 544–548. doi: 10.1136/ard.2004.028746
Roos, E. M., and Arden, N. K. (2016). Strategies for the prevention of knee osteoarthritis. Nat. Rev. Rheumatol. 12, 92–101. doi: 10.1038/nrrheum.2015.135
Roos, E. M., and Dahlberg, L. (2005). Positive effects of moderate exercise on glycosaminoglycan content in knee cartilage: a four-month, randomized, controlled trial in patients at risk of osteoarthritis. Arthritis Rheum. 52, 3507–3514. doi: 10.1002/art.21415
Roos, E. M., Herzog, W., Block, J. A., and Bennell, K. L. (2011). Muscle weakness, afferent sensory dysfunction and exercise in knee osteoarthritis. Nat. Rev. Rheumatol. 7, 57–63. doi: 10.1038/nrrheum.2010.195
Roos, E. M., and Juhl, C. B. (2012). Osteoarthritis 2012 year in review: rehabilitation and outcomes. Osteoarthr. Cartilage 20, 1477–1483. doi: 10.1016/j.joca.2012.08.028
Scanzello, C. R., and Goldring, S. R. (2012). The role of synovitis in osteoarthritis pathogenesis. Bone 51, 249–257. doi: 10.1016/j.bone.2012.02.012
Schmidt, T. A., Gastelum, N. S., Nguyen, Q. T., Schumacher, B. L., and Sah, R. L. (2007). Boundary lubrication of articular cartilage: role of synovial fluid constituents. Arthritis Rheum. 56, 882–891. doi: 10.1002/art.22446
Seror, J., Zhu, L., Goldberg, R., Day, A. J., and Klein, J. (2015). Supramolecular synergy in the boundary lubrication of synovial joints. Nat. Commun. 6:6497. doi: 10.1038/ncomms7497
Sims, N. A., and Martin, T. J. (2015). Coupling signals between the osteoclast and osteoblast: how are messages transmitted between these temporary visitors to the bone surface? Front. Endocrinol. 6:41. doi: 10.3389/fendo.2015.00041
Sivan, S., Schroeder, A., Verberne, G., Merkher, Y., Diminsky, D., Priev, A., et al. (2010). Liposomes act as effective biolubricants for friction reduction in human synovial joints. Langmuir 26, 1107–1116. doi: 10.1021/la9024712
Skou, S. T., and Thorlund, J. B. (2018). A 12-week supervised exercise therapy program for young adults with a meniscal tear: program development and feasibility study. J. Bodyw. Mov. Ther. 22, 786–791. doi: 10.1016/j.jbmt.2017.07.010
Sorkin, R., Dror, Y., Kampf, N., and Klein, J. (2014). Mechanical stability and lubrication by phosphatidylcholine boundary layers in the vesicular and in the extended lamellar phases. Langmuir 30, 5005–5014. doi: 10.1021/la500420u
Sorkin, R., Kampf, N., Dror, Y., Shimoni, E., and Klein, J. (2013). Origins of extreme boundary lubrication by phosphatidylcholine liposomes. Biomaterials 34, 5465–5475. doi: 10.1016/j.biomaterials.2013.03.098
Stensrud, S., Roos, E. M., and Risberg, M. A. (2012). A 12-week exercise therapy program in middle-aged patients with degenerative meniscus tears: a case series with 1-year follow-up. J. Orthop. Sports Phys. Ther. 42, 919–931. doi: 10.2519/jospt.2012.4165
Stewart, H. L., and Kawcak, C. E. (2018). The importance of subchondral bone in the pathophysiology of osteoarthritis. Front. Vet. Sci. 5:178. doi: 10.3389/fvets.2018.00178
Suda, T., Takahashi, N., Udagawa, N., Jimi, E., Gillespie, M. T., and Martin, T. J. (1999). Modulation of osteoclast differentiation and function by the new members of the tumor necrosis factor receptor and ligand families. Endocr. Rev. 20, 345–357. doi: 10.1210/edrv.20.3.0367
Sun, T., Sun, Y., and Zhang, H. (2018). Phospholipid-coated mesoporous silica nanoparticles acting as lubricating drug nanocarriers. Polymers 10:513. doi: 10.3390/polym10050513
Sun, Y., Zhang, H., Wang, Y., and Wang, Y. (2017). Charged polymer brushes-coated mesoporous silica nanoparticles for osteoarthritis therapy: a combination between hydration lubrication and drug delivery. J. Control. Release 259, e45–e46. doi: 10.1016/j.jconrel.2017.03.114
Svege, I. C., Fernandes, L., Nordsletten, L., and Risberg, M. (2011). Time to total hip replacement surgery after supervised exercise and patient education in patients with hip osteoarthritis. a randomized intervention study with between 3.5 and 6 years follow up. Osteoarthr. Cartilage 19, S44–S45. doi: 10.1016/S1063-4584(11)60113-1
Tang, Y., Wu, X., Lei, W., Pang, L., Wan, C., Shi, Z., et al. (2009). TGF-β1-induced migration of bone mesenchymal stem cells couples bone resorption with formation. Nat. Med. 15, 757–765. doi: 10.1038/nm.1979
Thorlund, J. B., Aagaard, P., and Roos, E. M. (2012). Muscle strength and functional performance in patients at high risk of knee osteoarthritis: a follow-up study. Knee Surg. Sports Traumatol. Arthrosc. 20, 1110–1117. doi: 10.1007/s00167-011-1719-2
Wan, M., Li, C., Zhen, G., Jiao, K., He, W., Jia, X., et al. (2012). Injury-activated transforming growth factor β controls mobilization of mesenchymal stem cells for tissue remodeling. Stem Cells 30, 2498–2511. doi: 10.1002/stem.1208
Wyatt, F. B., Milam, S., Manske, R. C., and Deere, R. (2001). The effects of aquatic and traditional exercise programs on persons with knee osteoarthritis. J. Strength Cond. Res. 15, 337–340. doi: 10.1519/00124278-200108000-00013
Xia, B., Di, C., Zhang, J., Hu, S., Jin, H., and Tong, P. (2014). Osteoarthritis pathogenesis: a review of molecular mechanisms. Calcif. Tissue Int. 95, 495–505. doi: 10.1007/s00223-014-9917-9
Xie, L., Tintani, F., Wang, X., Li, F., Zhen, G., Qiu, T., et al. (2016). Systemic neutralization of TGF-β attenuates osteoarthritis. Ann. N.Y. Acad. Sci. 1376, 53–64. doi: 10.1111/nyas.13000
Xu, X., Zheng, L., Bian, Q., Xie, L., Liu, W., Zhen, G., et al. (2015). Aberrant activation of TGF-β in subchondral bone at the onset of rheumatoid arthritis joint destruction. J. Bone Miner. Res. 30, 2033–2043. doi: 10.1002/jbmr.2550
Yan, Y., Sun, T., Zhang, H., Ji, X., Sun, Y., Zhao, X., et al. (2019). Euryale ferox seed-inspired superlubricated nanoparticles for treatment of osteoarthritis. Adv. Funct. Mater. 29:1807559. doi: 10.1002/adfm.201807559
Zaidi, M. (2007). Skeletal remodeling in health and disease. Nat. Med. 13, 791–801. doi: 10.1038/nm1593
Zhen, G., and Cao, X. (2014). Targeting TGFβ signaling in subchondral bone and articular cartilage homeostasis. Trends Pharmacol. Sci. 35, 227–236. doi: 10.1016/j.tips.2014.03.005
Zhen, G., Wen, C., Jia, X., Li, Y., Crane, J. L., Mears, S. C., et al. (2013). Inhibition of TGF-β signaling in mesenchymal stem cells of subchondral bone attenuates osteoarthritis. Nat. Med. 19, 704–712. doi: 10.1038/nm.3143
Zheng, L., Pi, C., Zhang, J., Fan, Y., Cui, C., Zhou, Y., et al. (2018). Aberrant activation of latent transforming growth factor-β initiates the onset of temporomandibular joint osteoarthritis. Bone Res. 6:26. doi: 10.1038/s41413-018-0027-6
Keywords: osteoarthritis, articular cartilage, self-lubricated drug-loaded nanoparticles, bone remodeling, exercise therapy
Citation: Ji X and Zhang H (2019) Current Strategies for the Treatment of Early Stage Osteoarthritis. Front. Mech. Eng. 5:57. doi: 10.3389/fmech.2019.00057
Received: 22 June 2019; Accepted: 30 August 2019;
Published: 13 September 2019.
Edited by:
Stanislav N. Gorb, University of Kiel, GermanyReviewed by:
Yoshitaka Nakanishi, Kumamoto University, JapanCopyright © 2019 Ji and Zhang. This is an open-access article distributed under the terms of the Creative Commons Attribution License (CC BY). The use, distribution or reproduction in other forums is permitted, provided the original author(s) and the copyright owner(s) are credited and that the original publication in this journal is cited, in accordance with accepted academic practice. No use, distribution or reproduction is permitted which does not comply with these terms.
*Correspondence: Hongyu Zhang, emhhbmdoeXVAdHNpbmdodWEuZWR1LmNu
Disclaimer: All claims expressed in this article are solely those of the authors and do not necessarily represent those of their affiliated organizations, or those of the publisher, the editors and the reviewers. Any product that may be evaluated in this article or claim that may be made by its manufacturer is not guaranteed or endorsed by the publisher.
Research integrity at Frontiers
Learn more about the work of our research integrity team to safeguard the quality of each article we publish.