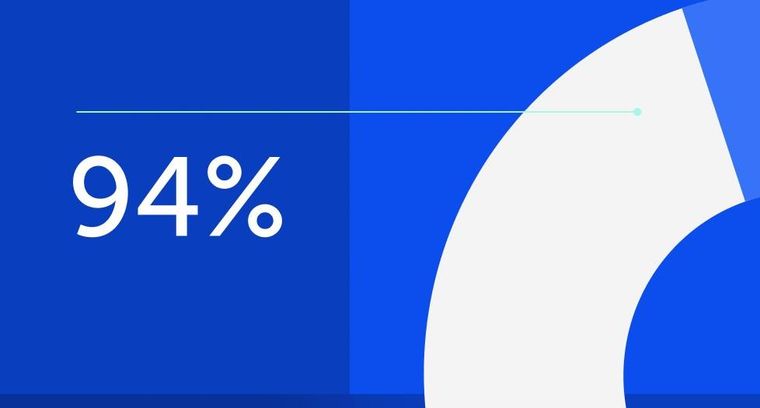
94% of researchers rate our articles as excellent or good
Learn more about the work of our research integrity team to safeguard the quality of each article we publish.
Find out more
EDITORIAL article
Front. Mater.
Sec. Energy Materials
Volume 12 - 2025 | doi: 10.3389/fmats.2025.1604024
This article is part of the Research TopicFunctionalization of Porous Materials for Sustainable Energy ApplicationsView all 5 articles
The final, formatted version of the article will be published soon.
Select one of your emails
You have multiple emails registered with Frontiers:
Notify me on publication
Please enter your email address:
If you already have an account, please login
You don't have a Frontiers account ? You can register here
This special topic explores recent advancements in energy and materials science through four scholarly papers, showcasing innovative solutions for sustainable energy technologies while providing valuable insights into the unique properties and structure of porous materials (Figure 1). Dr. Li and his group present their work on highly defective NiFeV layered triple hydroxides, highlighting enhanced electrocatalytic activity and stability for oxygen evolution reactions (OER). Dr. Kovalskii and the team contribute a mini-review on hydrogen storage using hexagonal boron nitride (h-BN) and BN-based materials, offering an insightful overview of these promising materials. Dr. Bashir and the team discuss their recent achievements in ceramic electrolytes used for improvement of performance of solid-state batteries. Lastly, Dr. Gao and Dr. Tie's team review the properties of porous materials with a focus on shrinkage behavior during the drying process, shedding light on key considerations for material design. Dr. Li and his collaborators have presented a compelling research article that marks an advance in electrocatalysis for the oxygen evolution reaction (OER). Their study focuses on a highly defective NiFeV layered triple hydroxide (LTH) catalyst, synthesized via a one-step hydrothermal process, demonstrating outstanding performance in OER. Compared to conventional catalysts, which often struggle with high overpotentials and stability issues, this innovative NiFeV LTH catalyst exhibits a low overpotential, a slight Tafel slope, and excellent long-term stability under high current densities. Their work represents a significant step forward in pursuing renewable energy technologies. It demonstrates that innovative material design can overcome existing limitations and pave the way for more sustainable and economically viable energy storage and conversion systems. The research reveals that incorporating vanadium plays a pivotal role in optimizing the catalyst's performance through structural and electrochemical characterizations, including X-ray diffraction, photoelectron spectroscopy, and in situ Raman spectroscopy. In the early stages of the OER, nickel species serve as the primary active sites, facilitating initial reaction steps. Iron and vanadium gradually join the catalytic process as overpotential increases, contributing to robust and efficient oxygen evolution. Notably, the presence of vanadium helps mitigate the dissolution of high-valence nickel species, thereby preventing catalyst degradation and ensuring sustained activity over prolonged operation. This research not only sheds light on the mechanisms that enhance ion transport and surface reconstruction during OER but also highlights the potential for targeted metal ion modifications to improve the performance of LDH-type catalysts. The study's findings suggest that precise doping strategies and structural optimizations can drive significant improvements in activity and durability. By addressing key challenges in catalyst design with a focus on the sluggish kinetics inherent in the OER's four-electron transfer process, Dr. Li's research offers promising avenues for developing next-generation, cost-effective, and high-performance electrocatalysts.Dr. Kovalskii's paper, Outstanding Features of Hexagonal Boron Nitride-Based Materials, presents a comprehensive overview of the promise and challenges associated with using h-BN nanomaterials for hydrogen storage. Highlighting a unique blend of high porosity, extended surface area, and exceptional thermal and chemical stability, even up to 1273 K in air, these h-BN materials emerge as attractive alternatives to traditional carbon-based materials. Its strong B-N covalent bonds and inherent structural rigidity suggest versatile applications, particularly in hydrogen storage technology, where chemisorption and physisorption mechanisms have been explored. The review underscores that h-BN-based materials offer reliable performance characteristics, including improved cycling and regeneration compared to conventional systems. However, one significant challenge remains: the lack of cost-effective, scalable production methods to fabricate these nanostructures at an industrial level, alongside efficient post-synthesis modifications to enhance their hydrogen absorption capacity. Dr. Kovalskii and his team emphasize that targeted strategies are key to optimizing performance. The approaches include increasing the effective specific surface area, engineering structural defects, tuning interplanar distances, and doping with elements like carbon and oxygen, or functionalizing surfaces with metal clusters. These modifications have shown potential to boost hydrogen uptake within a range of 0.1-9.67 wt%, making the material particularly promising for high-demand applications in automotive energy storage. Both experimental studies and first-principles and density functional theory (DFT) calculations consistently reveal the high potential of h-BN-based materials for effective hydrogen storage. Although theoretical predictions often suggest slightly higher capacities than those measured in laboratory settings, this divergence indicates the need for further experimental investigation to fine-tune material performance. Improved control over hydrogen release and material reuse will also be essential for transforming h-BN from a laboratory curiosity into a commercialization of hydrogen storage system. This work not only elucidates the underlying mechanisms that govern hydrogen sorption in h-BN but also charts a clear course for future research.Dr. Bashir and his co-workers have delivered a detailed research study on how the increasing demand for efficient and safe energy storage drives rapid advancements in solid-state battery (SSB) technology. These batteries offer higher capacity and improved safety compared to traditional liquid-electrolyte lithium-ion batteries, yet challenges such as lithium dendrite formation and poor solid-solid interfaces continue to hinder their widespread use. Their study addresses these obstacles by enhancing ceramic-based solid-state electrolytes through nanoscale doping with multicomponent halides. Guided by green chemistry principles, the team synthesized Li3InCl6-based electrolytes using an eco-friendly nanoemulsion method with water and natural aloe vera extract. The electrolytes were doped with fluorine (F), cerium (Ce), and molybdenum (Mo), with each dopant offering distinct benefits: The element F improved lattice stability and facilitated Li⁺ ion mobility; Ce bolstered structural integrity while reducing interfacial resistance; and Mo optimized ionic conductivity, achieving performance levels comparable to commercial liquid electrolytes. The structural and electrochemical analyses revealed that Mo-doped Li3InCl6 crystallizes in a triclinic structure, where modifications in electron density and reduced activation energy barriers lead to enhanced ion transport. Electrochemical impedance spectroscopy confirmed that Mo-doping markedly lowered the charge transfer resistance, indicating more efficient ionic pathways. These results underscore the pivotal role of Mo and other elements in enhancing the electrolyte's structural stability and overall ionic conductivity. The innovative synthesis approach advances the performance of solidstate electrolytes and aligns with sustainable practices. By employing green chemistry, the research team achieved a 40% reduction in energy consumption and a 75% decrease in hazardous waste generation compared to conventional methods, supporting broader environmental goals. Furthermore, their investigation illuminates the complex interactions among dopants, lattice modifications, ionic occupancy, and structural vacancies. The cyclic voltammetric and Nyquist plot analyses further validate the doped electrolyte's robust electrochemical performance and stability, confirming its potential for next-generation SSB applications. The study suggests that future research should explore additional doping strategies and integrate advanced computational modeling and machine-learning techniques to optimize electrolyte design further. These efforts are essential for developing sustainable, highperformance energy storage solutions that meet modern demands while addressing critical environmental and resource challenges.As outlined by Dr. Gao's and Dr. Tie's team, the shrinkage behavior of porous materials is a critical factor in the drying process, as it profoundly influences both the final product's texture and the efficiency of energy utilization. This phenomenon is governed by various factors, including the cellular structure, drying conditions, and the material's glass transition temperature. Advancing our understanding of the drying process necessitates the development of theoretical models that integrate simultaneous heat and mass transfer at the cellular level, as well as simulation tools to analyze morphological changes during drying. This paper examines the key factors influencing shrinkage in porous materials during drying, alongside discussions on modeling approaches, morphological simulations, and considerations for designing drying technologies. These insights aim to guide improvements in product quality and energy efficiency. Drying, a key material preservation process, uses heat to remove moisture, extending shelf life while reducing weight and storage costs. Porous materials undergo shrinkage and deformation during drying, influenced by temperature and moisture migration, impacting energy efficiency, product quality, and equipment design. Higher temperatures increase shrinkage effects. Their study identifies major factors affecting shrinkage behavior, summarizes theoretical and simulation approaches to understand material deformation during drying, and evaluates various industrial drying techniques. Understanding the interplay of these factors during drying is vital for optimizing drying equipment, reducing energy usage, and ensuring the quality and longevity of dried products. The major achievements made by the authors are four folds:1. Impact of Cellular Structure and Temperature: Shrinkage is strongly influenced by water migration within cellular structures and the mechanical properties of cell walls. Among various drying conditions, temperature plays the most significant role. When drying occurs below the glass transition temperature (Tg), the material assumes a highly viscous state, reducing shrinkage. 2. Multi-Scale Modeling Challenges: Multi-scale models, which span macro-scale and micro-scale perspectives, offer effective tools for analyzing drying processes. However, reducing computational complexity and modeling costs remains a significant challenge in meeting the diverse accuracy requirements of different applications. 3. Simulation Gaps: While computer graphics simulations have primarily focused on plant growth modeling, fewer studies have examined the mechanical deformation of porous materials during drying. Addressing these gaps will enhance our understanding of dryinginduced changes. 4. Assessment of Drying Techniques: Different drying methods have unique strengths and weaknesses. Hot air is cost-effective but may deform materials. The microwave is fast yet uneven. Freeze drying preserves structure but is slow. Infrared offers quality control, while vacuum suits heat-sensitive materials.The editorial team comprises three guest editors and one topic coordinator (Figure 2) with diverse expertise spanning academia, industry, and national laboratory. Their collective contributions advance materials design and evaluation, with several members renowned for pioneering applications of these materials in energy system development. Together, they bring a wealth of knowledge and innovation to the field. Dr. Vanadium Li (Figure 2a) has been serving as a Full Professor in the Department of Chemical Engineering at the Beijing University of Technology since 2018. His research expertise lies in the study of variable valence transition metal elements, focusing on their roles in material processes under high-temperature and wet chemical conditions. Dr. Li specializes in mixed valence oxides, particularly Magnéli phases, and their advanced applications in electro-catalysis and related fields. Dr. Li has an extensive publication record, authoring approximately 50 books and journal articles that have contributed significantly to the advancement of his field. His groundbreaking research has earned him key leadership roles, serving as Principal Investigator (PI) and Co-Principal Investigator (Co-PI) for numerous national and international research projects. Dr. Li's innovative work in mixed valence oxide systems and catalytic processes continues to push the boundaries of material science, offering practical solutions to challenges in energy conversion, catalysis, and sustainable materials development. His contributions demonstrate a commitment to advancing scientific knowledge and fostering innovation in chemical engineering.Dr. Ayrat Gizzatov (Figure 2b) is a distinguished leader in sustainable energy innovation and materials science, currently serving as Senior Manager of R&D
Keywords: Porous materials, energy materials, multi-scale models, Drying techniques, Emerging energy technologies
Received: 01 Apr 2025; Accepted: 07 Apr 2025.
Copyright: © 2025 Li, Gizzatov, Shi and Liu. This is an open-access article distributed under the terms of the Creative Commons Attribution License (CC BY). The use, distribution or reproduction in other forums is permitted, provided the original author(s) or licensor are credited and that the original publication in this journal is cited, in accordance with accepted academic practice. No use, distribution or reproduction is permitted which does not comply with these terms.
* Correspondence: Jingbo Louise Liu, Texas A&M University Kingsville, Kingsville, United States
Disclaimer: All claims expressed in this article are solely those of the authors and do not necessarily represent those of their affiliated organizations, or those of the publisher, the editors and the reviewers. Any product that may be evaluated in this article or claim that may be made by its manufacturer is not guaranteed or endorsed by the publisher.
Supplementary Material
Research integrity at Frontiers
Learn more about the work of our research integrity team to safeguard the quality of each article we publish.