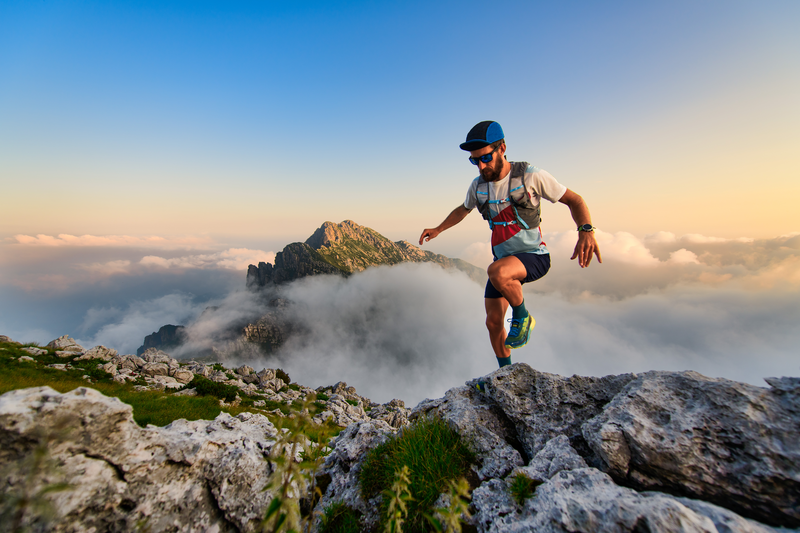
94% of researchers rate our articles as excellent or good
Learn more about the work of our research integrity team to safeguard the quality of each article we publish.
Find out more
ORIGINAL RESEARCH article
Front. Mater.
Sec. Semiconducting Materials and Devices
Volume 12 - 2025 | doi: 10.3389/fmats.2025.1567614
This article is part of the Research Topic Advancing Transition Metal Nitride Semiconductors: Overcoming Integration Challenges and Exploring Applications View all 3 articles
The final, formatted version of the article will be published soon.
You have multiple emails registered with Frontiers:
Please enter your email address:
If you already have an account, please login
You don't have a Frontiers account ? You can register here
This study reports on the characterization of a 45% scandium-doped Aluminum Nitride (AlScN) thin films co-doped with 10% Boron (B). AlScBN thin films were deposited on Si(100) 200 mm wafers using a pulsed DC reactive magnetron sputtering system. A 4" casted alloy target of Al 0.45 Sc 0.45 B 0.10 was used in the depositions. The influence of deposition parameters, including target-substrate distance, N 2 flow rate, and deposition temperature, on the films' crystallinity and surface characteristics was investigated on silicon substrates. The crystalline properties of the thin films were characterized using X-ray diffraction (XRD) method with 2θ and ω scans.Based on the characterization performed on Si, process conditions were selected to deliver the best crystallinity while maintaining a stable plasma. Parameters were set to a target-substrate distance of 65 mm, a flow rate of 30 sccm, and a deposition temperature of 300°C. Under these process conditions, a 564 nm-thick sample was fabricated for piezoelectric and ferroelectric characterization. Several capacitive structures of different areas were prepared using a tungsten (W) blanket bottom electrode and platinum (Pt) top electrode on a double-side polished Si substrate.The d 33,f coefficient was measured through Piezoelectric Measurement (PZM) technique using Double Beam Laser Interferometry (DBLI) system, yielding a value of ~25 pm/V . Ferroelectric characteristics were assessed through Dynamic Hysteresis Measurement (DHM) and Positive-Up, Negative-Down (PUND) measurement techniques using the same tool. The film exhibited a coercive field E + c = 1.5 M V /cm, E - c = 2.5 M V /cm and a remnant polarization (2P r ) of 280 µCcm -2 .
Keywords: piezoelectricity, Ferroelectricity, MEMS, thin films, Sputtering, AlScBN
Received: 27 Jan 2025; Accepted: 04 Mar 2025.
Copyright: © 2025 Saha, Simeoni, Colombo and Rinaldi. This is an open-access article distributed under the terms of the Creative Commons Attribution License (CC BY). The use, distribution or reproduction in other forums is permitted, provided the original author(s) or licensor are credited and that the original publication in this journal is cited, in accordance with accepted academic practice. No use, distribution or reproduction is permitted which does not comply with these terms.
* Correspondence:
Kapil Saha, Northeastern University, Boston, United States
Disclaimer: All claims expressed in this article are solely those of the authors and do not necessarily represent those of their affiliated organizations, or those of the publisher, the editors and the reviewers. Any product that may be evaluated in this article or claim that may be made by its manufacturer is not guaranteed or endorsed by the publisher.
Research integrity at Frontiers
Learn more about the work of our research integrity team to safeguard the quality of each article we publish.