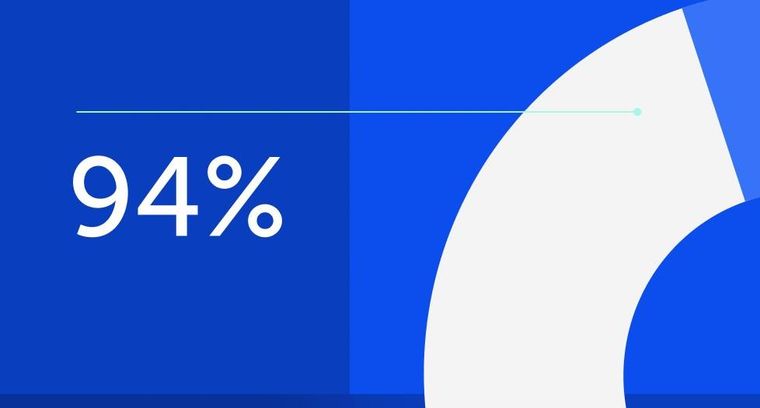
94% of researchers rate our articles as excellent or good
Learn more about the work of our research integrity team to safeguard the quality of each article we publish.
Find out more
EDITORIAL article
Front. Mater., 21 January 2025
Sec. Biomaterials and Bio-Inspired Materials
Volume 12 - 2025 | https://doi.org/10.3389/fmats.2025.1551590
This article is part of the Research TopicBiodegradable Polymers for Biomedical Applications , volume IIIView all 13 articles
Editorial on the Research Topic
Biodegradable polymers for biomedical applications -volume III
Biodegradable polymers have emerged as cornerstones in developing advanced biomedical applications, offering solutions for drug delivery (Soppimath et al., 2001), tissue engineering (Iqbal et al., 2019), and regenerative medicine (Arun et al., 2021). With their biocompatibility, tunable properties, and ability to degrade into non-toxic byproducts, biodegradable polymers present ideal solutions for modern medical challenges. This Research Topic, “Biodegradable Polymers for Biomedical Applications - Volume III,” brings together cutting-edge research and reviews, highlighting recent advances, innovative strategies, and future directions in this dynamic field.
This volume includes 12 articles, each contributing to the understanding and advancement of biodegradable polymers for biomedical applications. Among them, three articles focus on drug delivery systems (DDS), demonstrating their potential for enhanced therapeutic efficacy and reduced side effects. For instance, Qiu et al. delved into poly (lactic-co-glycolic acid) (PLGA)-based nanoparticles for bone tumor treatment, emphasizing their capability for sustained release and targeted drug delivery. Similarly, Wang et al. discussed PLGA nanoparticles, highlighting their versatility in cancer treatment and ability to improve drug bioavailability while reducing systemic toxicity. Wang et al. focused on recent advances in natural and synthetic polymer-based microsphere delivery systems for treating knee osteoarthritis.
Biodegradable polymers also play a crucial role in tissue engineering and regenerative medicine. Cao et al. summarized recent progress in addressing skin photoaging using hydrogels, exploring the challenges of utilizing hydrogels as therapeutic agents and outlining potential future developments. Similarly, Yang et al. provided a comprehensive overview of current biodegradable esophageal stent materials and their applications, highlighting current research limitations and innovations while offering insights into future development priorities and directions. Zhao et al. prepared a 3D printed composite scaffold of BMP-2/PLGA microspheres with polylactic acid glycolic acid copolymer/CaSO4, which was used to improve the osteogenic properties of the scaffold. This 3D printed scaffold was able to accelerate the repair of complex bone defects by promoting new bone formation, suggesting that it may prove to be a potential bone tissue engineering substitute. Anjum et al. demonstrated the promise of HA and ALN-loaded PVP/PVA-ALN-HA nanofiber composite scaffolds for bone regeneration applications. Wei et al. evaluated the potential of MSAH, prepared using OSA and CMC mixtures, for targeted vessel embolization in an internal iliac artery hemorrhage model. MSAH achieved aortic embolization without affecting peripheral arterial blood supply, enabling rapid short-term hemostasis and long-term degradation without target organ necrosis.
Furthermore, biodegradable polymers find extensive applications in other industries. Yarahmadi et al. outlined recent research on applying chitosan and gelatin polymers across various fields, including food packaging, antioxidant and antimicrobial properties, encapsulation of bioactive substances, tissue engineering, microencapsulation technology, water treatment, and drug delivery, showcasing the multiple uses of gelatin and chitosan polymers in addressing environmental issues and advancing different industries.
The development of novel biodegradable materials is a recurring theme in this volume. Anjum et al. prepared PVP/PVA nanofiber scaffolds through novel electrospinning techniques. Results showed that these porous PVP/PVA nanofibers demonstrate excellent biocompatibility with great potential in biomedical applications. Chen et al. introduced a novel smart elastomer, mPEG43-b-(PMBC-co-PCL)n, developed from polyester and polycarbonate blends with excellent shape memory and self-healing capabilities through physical crosslinking systems. This innovative elastomer provides a solid foundation and broad prospects for developing self-shrinking smart surgical sutures in the biomedical field. Additionally, Li et al. proposed a simple and effective strategy for adjusting PTMC degradation rates by blending photoactive PEGDA with PTMC, followed by UV photopolymerization of the mixture to prepare poly (trimethylene carbonate) (PTMC) and crosslinked poly (ethylene glycol) diacrylate (PEGDA) blends. The introduction of UV-crosslinked PEGDA significantly improved PTMC’s resistance to lipase erosion, expanding its applications in the medical field.
This volume of “Biodegradable Polymers for Biomedical Applications - Volume III” showcases significant progress in utilizing biodegradable polymers for drug delivery, tissue engineering, and other applications. In addition to the insightful contributions, this volume reflects remarkable geographic diversity among the authors, emphasizing the global collaboration within the field of biodegradable polymers for biomedical applications. The articles included in this volume are authored by researchers from a wide range of regions, including China, India, Russia, Saudi Arabi and Iran. This geographic diversity not only underscores the universal relevance of the topic but also highlights the collective effort of the global scientific community to address biomedical challenges. By uniting perspectives from various parts of the world, this volume serves as a bridge for cross-regional innovation and knowledge sharing, setting the foundation for future interdisciplinary collaborations. The authors’ contributions provide valuable insights into the design, application, and future potential of these materials. We extend our gratitude to all authors, reviewers, and editors who contributed to this Research Topic. Their efforts have greatly advanced our understanding of biodegradable polymers and their transformative impact on biomedicine.
As we conclude this volume on biodegradable polymers for biomedical applications, several crucial insights emerge that warrant careful consideration. The collected works demonstrate significant progress in developing biocompatible materials with controlled degradation profiles and enhanced functionality. However, we must acknowledge certain limitations and challenges that persist in this field. While biodegradable polymers show promising results in laboratory settings, concerns about their long-term stability, batch-to-batch consistency, and scalability in industrial production remain valid counterarguments to their immediate widespread adoption. Critics rightfully point out that the cost-effectiveness of these materials compared to traditional alternatives needs further evaluation, and the complete understanding of their degradation products’ long-term effects requires additional investigation. Nevertheless, these challenges should not overshadow the tremendous potential of biodegradable polymers in revolutionizing biomedical applications. The key take-home message for readers is that the field is rapidly evolving, with innovative solutions emerging to address current limitations. Future research directions should focus on developing standardized testing protocols, improving manufacturing processes for better consistency, and conducting more comprehensive in vivo studies. Additionally, the integration of artificial intelligence and machine learning approaches could accelerate material design and optimization, while enhanced collaboration between academic institutions and industry partners could bridge the gap between laboratory success and clinical application. We encourage readers to approach these developments with both enthusiasm and critical thinking. The challenges identified in this volume should serve as catalysts for innovation rather than barriers to progress. As we move forward, the focus should be on addressing unresolved questions about degradation mechanisms, biocompatibility, and scale-up processes while maintaining an open dialogue about both the possibilities and limitations of these materials. The future of biodegradable polymers in biomedical applications lies in our ability to balance optimism with pragmatic solutions to these remaining challenges.
LY: Conceptualization, Data curation, Supervision, Writing–original draft, Writing–review and editing. JH: Supervision, Writing–review and editing. SJ: Supervision, Writing–review and editing.
The author(s) declare that no financial support was received for the research, authorship, and/or publication of this article.
The authors declare that the research was conducted in the absence of any commercial or financial relationships that could be construed as a potential conflict of interest.
The author(s) declare that no Generative AI was used in the creation of this manuscript.
All claims expressed in this article are solely those of the authors and do not necessarily represent those of their affiliated organizations, or those of the publisher, the editors and the reviewers. Any product that may be evaluated in this article, or claim that may be made by its manufacturer, is not guaranteed or endorsed by the publisher.
Arun, Y., Ghosh, R., and Domb, A. J. (2021). Biodegradable hydrophobic injectable polymers for drug delivery and regenerative medicine. Adv. Funct. Mater. 31 (44), 2010284. doi:10.1002/adfm.202010284
Iqbal, N., Khan, A. S., Asif, A., Yar, M., Haycock, J. W., and Rehman, I. U. (2019). Recent concepts in biodegradable polymers for tissue engineering paradigms: a critical review. Int. Mater. Rev. 64 (2), 91–126. doi:10.1080/09506608.2018.1460943
Keywords: biodegradable polymers, drug delivery, tissue engineering, regenerative medicine, nanoparaticles, scaffold
Citation: Yang L, Hu J and Jiang S (2025) Editorial: Biodegradable polymers for biomedical applications - volume III. Front. Mater. 12:1551590. doi: 10.3389/fmats.2025.1551590
Received: 26 December 2024; Accepted: 07 January 2025;
Published: 21 January 2025.
Edited and reviewed by:
Hafiz M. N. Iqbal, Monterrey Institute of Technology and Higher Education (ITESM), MexicoCopyright © 2025 Yang, Hu and Jiang. This is an open-access article distributed under the terms of the Creative Commons Attribution License (CC BY). The use, distribution or reproduction in other forums is permitted, provided the original author(s) and the copyright owner(s) are credited and that the original publication in this journal is cited, in accordance with accepted academic practice. No use, distribution or reproduction is permitted which does not comply with these terms.
*Correspondence: Liqun Yang, eWFuZ2xpcXVuQHNqLWhvc3BpdGFsLm9yZw==
Disclaimer: All claims expressed in this article are solely those of the authors and do not necessarily represent those of their affiliated organizations, or those of the publisher, the editors and the reviewers. Any product that may be evaluated in this article or claim that may be made by its manufacturer is not guaranteed or endorsed by the publisher.
Research integrity at Frontiers
Learn more about the work of our research integrity team to safeguard the quality of each article we publish.