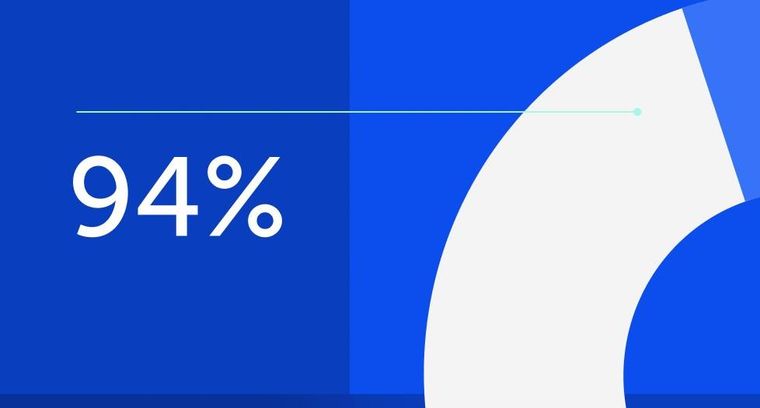
94% of researchers rate our articles as excellent or good
Learn more about the work of our research integrity team to safeguard the quality of each article we publish.
Find out more
REVIEW article
Front. Mater., 10 April 2025
Sec. Environmental Degradation of Materials
Volume 12 - 2025 | https://doi.org/10.3389/fmats.2025.1541204
This article is part of the Research TopicAdvances in Radiation-Resistant Composite Materials for Nuclear ApplicationsView all articles
Uranium contamination in water and soil poses serious risks to human health and ecosystems. Adsorption is a promising method for uranium removal due to its efficiency and simplicity. This review highlights recent advancements in uranium (VI) adsorption using Metal-Organic Frameworks (MOFs), Covalent Organic Frameworks (COFs), Graphene Oxide (GO), and MXenes. MOFs and COFs offer high adsorption capacities and tunable structures, while GO and MXenes exhibit excellent performance due to their large surface areas and unique chemical properties. However, challenges such as regeneration costs, material stability, and large-scale production remain significant barriers. This review discusses these challenges, compares material advantages and limitations, and outlines future directions to develop efficient adsorbents for radioactive waste management and environmental remediation.
Uranium is a radioactive element with significant importance and risks. It primarily exists as isotopes 235U, 238U, and 234U. 235U is fissile and used in nuclear reactors to produce energy. However, its presence in the environment, mainly due to nuclear activities and mining, poses severe health and environmental hazards. Uranium can contaminate water and soil, entering the food chain and causing toxic effects. It can accumulate in human organs, leading to kidney damage, cancer, and genetic mutations. Its long half-life means uranium persists in the environment, necessitating effective removal methods to minimize exposure. Traditional uranium removal methods, including chemical precipitation, ion exchange, adsorption, membrane separation, and electrochemical processes, have limitations such as secondary pollution, high costs, and durability issues (Kumar et al., 2022).
Recent advances in materials science offer promising solutions for uranium removal. Porous materials like covalent organic frameworks (COFs) and metal-organic frameworks (MOFs) show great potential due to their highly ordered pore structures and large surface areas, which enhance adsorption efficiency (Bi et al., 2024). Carbon-based materials, including graphene and its derivatives, along with activated carbon, provide abundant surface functional groups and high surface areas that are ideal for binding uranium ions (Liang et al., 2017). Additionally, two-dimensional materials such as MXenes possess unique electronic properties and structural characteristics that make them effective in uranium capture. Emerging composite materials, combining COFs, MOFs, or MXenes with other functional components, demonstrate synergistic effects, improving adsorption efficiency and selectivity. Functionalized adsorbents, such as GO modified with chelating agents or MOFs with tailored linkers, further enhance uranium capture.
This review focuses on recent research advancements in using MOFs, COFs, graphene oxide (GO), and MXenes for uranium removal. It explores their adsorption performance, mechanisms, and potential applications, highlighting how these advanced materials overcome the limitations of traditional methods (Figure 1). Furthermore, the review discusses future development directions and challenges, providing scientific guidance and insights into the application of new materials in environmental remediation. The goal is to foster the development of innovative, efficient, and sustainable technologies for managing uranium contamination and protecting environmental and human health.
Figure 1. Uranium adsorption mechanism and application of MOFs, COFs, GO and MXenes (Chen et al., 2013; Li et al., 2015; Verma and Dutta, 2015; Wang et al., 2016; Zhang L. et al., 2016; Chen et al., 2017; Li et al., 2017; Wang et al., 2017; Niu et al., 2018; Sun Y. et al., 2018; Wang et al., 2018; Yang A. L. et al., 2018; Yu et al., 2021; Liu et al., 2022; Li et al., 2023; Mei et al., 2023).
MOFs are crystalline porous materials consisting of metal ions or clusters coordinated with organic ligands, forming a three-dimensional network. Figure 2 presents a timeline of the application of MOFs for the adsorption, removal, and detection of uranium. First synthesized in 1989 by Professor Bernard F. Hoskins (Hoskins and Robson, 1989), MOFs have become a versatile platform for various applications, including gas storage, separation, catalysis, and environmental remediation. Their high surface area and porosity make them particularly effective for adsorbing radioactive nuclides like uranium. Liu et al. (2022) summary the developmental milestones of MOFs and MOF-based materials for adsorption and/or detection of U(VI) is illustrated in Figure 2. MOFs can be categorized into various types based on their structural characteristics. Notable MOFs include Zeolitic Imidazolate Frameworks (ZIFs), which feature a zeolite-like structure; UiO materials, which contain zirconium clusters connected with terephthalic acid; and Materials of Institute Lavoisier (MIL), incorporating metal ions such as Al3+ and Fe3+ coordinated with dicarboxylic acids. Among these, the ZIF, MIL, and UiO series, like ZIF-67, MIL-100(Al), and UiO-66, exhibit exceptional water stability, making them particularly effective for uranium adsorption (Yue et al., 2021). Their robust frameworks provide high surface areas and tunable pore sizes, essential for capturing uranium ions from aqueous solutions.
Figure 2. Chronological development of MOFs and MOF-based materials highlighting major milestones in Uranium (VI) adsorption and detection, including notable advances in structural design and practical applications (Liu et al., 2022).
MOFs have emerged as effective materials for uranium removal due to their unique structural and functional properties. The removal of uranium by MOFs involves a combination of physical and chemical adsorption mechanisms that leverage the material’s high surface area, porosity, and functional versatility (Adil et al., 2022). The adsorption mechanism of uranium by MOFs materials involves coordination, ion exchange, and electrostatic interactions. Figure 3 illustrates several mechanisms of uranium adsorption by MOFs through various case studies.
Figure 3. (A): Uranium (VI) adsorption mechanism in JXNU-4 MOF: Interaction with carboxylate oxygen sites (Liu et al., 2019); (B): Co-SLUG-35 structure and its adsorption behavior; GO–COOH/UiO-66 composites: FTIR, pH, and XPS spectroscopy before and after U(VI) adsorption (Li et al., 2017); (C): Graphical representation of MOFs and strategies to enhance their adsorption performance (Yang P. P et al., 2018).
Coordination is the dominant mechanism, where functional groups within MOFs, such as carboxyl and hydroxyl groups, form stable complexes with uranyl ions (UO22+). For instance, UiO-66-AO is functionalized with amidoxime groups to chelate uranium effectively (Chen et al., 2017). Similarly, Liu et al. (2019) used adenine and BPDC ligands to modify a zinc-MOF (JXNU-4) to capture U(VI). JXNU-4 uses adenine and BPDC ligands to provide binding sites through exposed carboxylate oxygen atoms (Figure 3A). MOFs also exhibit ion-exchange capabilities, where uranyl ions can displace exchangeable cations within the framework. This ion-exchange mechanism enhances the retention and immobilization of uranium, providing an additional layer of adsorption strength. Ion exchange involves the replacement of ions in the MOF with uranium ions. This is evident in Co-SLUG-35 (Li et al., 2017), where uranium ions exchange with carbonate ions, and in GO-COOH/UiO-66 composites (Vaddanam et al., 2023), where hydrogen ions in carboxyl groups are replaced by uranyl ions (Figure 3B).
In the process of uranium adsorption by MOF-based materials, electrostatic interactions play a crucial role. These interactions occur when the charged surfaces of MOFs attract oppositely charged uranium ions, a process that is significantly influenced by the pH of the solution. At specific pH levels, the surface charge of MOFs can either enhance or inhibit the adsorption of uranyl ions (UO22+), thereby affecting the overall efficiency of uranium removal. This mechanism is integral to optimizing MOF performance in various environmental conditions. In rGO/ZIF-67 aerogels, an increase in pH enhances the electrostatic attraction between negatively charged surfaces and uranyl ions, increasing removal efficiency (Zhao et al., 2020). Similarly, MOF-5’s adsorption of uranium is strongly dependent on pH, highlighting electrostatic interactions (Wu et al., 2019).
These mechanisms often work together, with the specific conditions and MOF types determining which interactions dominate. The combination of these mechanisms allows MOFs to efficiently capture uranium from aqueous solutions, making them highly effective for environmental remediation. This multifaceted approach is crucial for optimizing MOF design and improving uranium adsorption performance.
U(VI) is a typical Lewis acid that tends to bind with hard bases such as nitrogen, oxygen, and phosphorus-containing groups (e.g., amines, hydroxyl groups, and phosphorylurea groups) (Table 1). Studies have shown that the pore size of MOFs significantly affects their adsorption performance for U(VI), with larger pores facilitating the diffusion of U(VI) into the framework. Carboni et al. (2013) modified UiO-68 with amino and dihydroxy phosphorylurea groups to create MOF-2 and MOF-3, which were used to adsorb U(VI) from radioactive wastewater and simulated seawater. It was found that MOF-2 coordinated with UO22+ through two phosphorylurea ligands, resulting in a higher adsorption capacity (217 mg/g), whereas MOF-3, which coordinated with UO22+ through a single ligand, showed a lower capacity (109 mg/g). Additionally, ligand protonation under acidic conditions facilitated the formation of more stable coordination structures with UO22+, thereby improving the adsorption performance.
Studies have shown that post-synthetic modification can further enhance the adsorption capacity of MOFs for U(VI). For example Bai et al. (2015), introduced three different nitrogen-containing amine ligands into MIL-101(Cr) to create MIL-101-NH2, MIL-101-ED, and MIL-101-DETA, with MIL-101-DETA exhibiting the highest uranium adsorption capacity (350 mg/g). Zhang Y. J et al. (2016) developed MOF-74-Zn by grafting coumarin onto unsaturated Zn (II) centers, which enhanced U(VI) adsorption (360 mg/g) due to larger pore sizes and additional adsorption sites. Furthermore, defect engineering strategies (such as adding modulators during synthesis) can introduce more active sites and larger pores by adjusting the pore size, thereby enhancing adsorption capacity (Liu et al., 2017).
Combining MOFs with other functional materials to create composites can significantly enhance adsorption performance through synergistic effects. For example Yang A. L. et al. (2018), designed a GO-COOH/UiO-66 composite for U(VI) extraction from simulated seawater, which maintained efficient adsorption in solutions with pH 6-9 and achieved an adsorption capacity of up to 188.3 mg/g. Magnetic composites such as Fe3O4@ZIF-8 also exhibited ultra-high adsorption capacity (523.5 mg/g) and excellent selectivity, mainly due to the coordination between Zn atoms and U(VI) ions and hydrogen bonding (Min et al., 2017).
COFs were first synthesized and reported in 2005 by Professor Omar Yaghi and his team at the University of California, Los Angeles (UCLA) (Côté et al., 2005). This discovery marked a significant milestone in the field of novel porous materials, demonstrating the potential for synthesizing highly crystalline and highly ordered organic porous materials. COFs are porous crystal materials formed by the covalent bond of organic molecules, which have the characteristics of adjustable pore structure, excellent chemical stability, large specific surface area and so on (Chen et al., 2020). In recent years, research on using COFs for the adsorption of radioactive nuclides has gradually begun to emerge. Figure 4 shows some key milestones in this area of research. The initial COF materials synthesized by Yaghi’s group—COF-1 and COF-5—were created through a self-assembly process, showcasing the high order and porosity of COFs. Since then, the development of COF materials has rapidly advanced, becoming an important research direction in materials science (Figure 5).
Figure 4. Development of COFs and COF-Based materials highlighting major milestones in Uranium (VI) adsorption, including notable advances in structural design and practical applications. Adapted from Chen et al. (2021).
Figure 5. (A): The typical synthesis pathway of COFs by Schiff base reaction and irreversible tautomerism; (B–E): inside pore of COFs with quadrangular, rhombic and hexagon-shape, triangle-hexagon, respectively; (F–I): SEM images of COFs with sheet, fibrous, petal and spherical morphology, respectively (Zhang P. C et al., 2020).
COFs are crystalline porous polymers whose structures are determined by pre-designed molecular building units connected through covalent bonds. These materials exhibit high chemical stability and can be easily functionalized with organic units, making them excellent carriers for adsorbing radioactive nuclides, toxic metal ions, and organic pollutants. Compared to traditional adsorbents, COFs offer several distinct advantages: (1) ordered porous channels provide abundant adsorption sites; (2) high surface area and permanent porosity facilitate rapid mass transfer between pollutants and adsorbents; (3) high chemical and thermal stability; (4) customizable structures offer pre-designed chelation sites that enhance targeted interactions. Additionally, the rich functional groups, low density, reusability, and adjustable physicochemical properties of COFs confer high adsorption capacity for radioactive nuclides. However, COFs materials still have certain limitations. For instance, their synthesis process is relatively complex; the synthesis of COFs typically requires multiple steps and precise control of conditions, which limits their large-scale production and application. Moreover, the synthesis of COFs involves the use of some harmful solvents, such as aniline, which can pose significant risks to the environment and human health (Mei et al., 2023).
In uranium removal, COFs rely on a synergistic interaction of physical and chemical adsorption. In nature, uranium primarily exists as UO22+, which have a high positive charge density and are the main target of COF adsorption. Physically, COFs with their highly ordered porous structures effectively capture uranyl ions through van der Waals forces and electrostatic interactions. These structures provide abundant adsorption sites and facilitate the chemical bonding of uranium ions with functional groups on the COF surface. The high surface area of COFs enables rapid concentration of uranium ions during the initial adsorption phase, enhancing overall adsorption efficiency.
Chemically, the functional groups in COFs, such as carboxyl, amide, amino, and hydroxyl groups, form stable coordination bonds with uranyl ions, effectively immobilizing them. These groups engage in strong coordination interactions with uranyl ions, forming stable complexes and enhancing selective adsorption. Additionally, negatively charged functional groups on the COF surface can electrostatically attract the positively charged uranyl ions, further improving selectivity and efficiency. In some cases, uranyl ions may also displace cations such as H+ or Na+ in the COF material through ion exchange, achieving uranium fixation. Research indicates that the presence of hydroxypropoxy groups strengthens the affinity and adsorptive capacity for UO22+ at the hydrazino-carbonyl sites of COFs by means of hydrogen bonding and charge stabilization (Zhang et al., 2022). Mei and Yan (2023) employed a post-synthetic modification approach to synthesize two COFs with rich phosphine group modifications, connected by β-ketoenamine and benzoxazole, overcoming the disadvantage that the affinity of hydrazine groups for vanadium is stronger than that for uranium.
The synergy between physical and chemical adsorption significantly enhances the overall adsorption capacity and efficiency of COF materials. Physical adsorption provides favorable conditions for the concentration and rapid kinetics of uranium ions, while chemical adsorption ensures stable binding and thermodynamic stability. This synergistic effect allows COFs to perform exceptionally well in uranium removal, demonstrating effectiveness in both simulated laboratory solutions and complex real-world environments.
Recent studies have demonstrated the potential of COFs for the selective adsorption and extraction of UO22+ from various environments (Table 2). By modifying COF materials with specific functional groups or structures, researchers have achieved significant improvements in their adsorption capacity and selectivity for UO22+. The following cases highlight different approaches to enhance the performance of COFs in uranium removal.
Guo et al. (2022) synthesized two nitrogen-containing COF materials, TpBpy and TpPy, by reacting 2,4,6-triformylphloroglucinol (Tp) with 2,2′-bipyridine-5,5′-diamine (Bpy) or pyridine-2,5′-diamine (Py), respectively (Figure 6A). The study found that TpBpy exhibited better adsorption performance for UO22+than TpPy. This was mainly due to the pyridine nitrogen in TpBpy, which can form a bidentate coordination structure with UO22+, a structure that is absent in TpPy. Specifically, UO22+ forms a bidentate coordination with the N and N=O sites in TpBpy, resulting in an adsorption energy of up to 5.08 eV. In contrast, the lack of this chelating structure in TpPy reduces its extraction capacity for UO22+, with the adsorption energy significantly lowered to 2.61 eV. Therefore, this study demonstrates that the two nitrogen atoms in the pyridine moiety play a crucial role in the extraction of UO22+, highlighting that the adsorption selectivity can be significantly enhanced by tuning the material structure.
Figure 6. (A) shows the synthesis process of TpBpy and TpPy, along with the adsorption isotherms of U(VI) on these materials (Guo et al., 2022); (B) illustrates the synthesis of COF-TpDb and its transformation into COF-TpDb-AO, along with the structural views of COF-TpDb and COF-TpDb-AO, highlighting their stacking structures (Sun Q et al., 2018).
Yu et al. (2021) investigated the adsorption capacity of phosphate-functionalized two-dimensional COFs (COF-IHEP10 and COF-IHEP11) for U(VI) under strongly acidic conditions. The experimental results showed that COF-IHEP11 exhibited excellent adsorption performance for U(VI) due to the synergistic effect between the carbonyl functional groups on the COF backbone and the phosphate active sites. In comparison, COF-JLU4, which lacks phosphate groups as side chains, showed a significantly lower adsorption capacity of around 70 mg/g. Theoretical calculations revealed that this synergistic effect effectively enhances the adsorption performance of COF materials for UO22+, thereby improving their stability and efficiency in highly acidic environments.
Sun Q. et al. (2018) developed amidoxime-functionalized COFs (COF-TpDb-AO) for the extraction of UO22+from seawater (Figure 6B). The amidoxime groups can form various coordination modes with UO22⁺, including monodentate, bidentate, and η2-type binding, with η2-type binding being the most stable. This binding mode, facilitated by strong interactions between the N and O atoms on the COFs and UO22+, enables effective chelation of UO22+ over a wide pH range. The study demonstrated that the functionalization of COFs by introducing specific coordination sites significantly enhances their selectivity for UO22+ adsorption.
The research team led by Li et al. (2015) synthesized a COF material rich in carboxyl groups (COF-COOH) and further modified it with 2-(2,4-dihydroxyphenyl)-benzimidazole (HBI), producing COF-HBI. Experimental results showed that COF-HBI exhibited higher selective adsorption capacity for U(VI) and greater adsorption amounts. This enhanced performance is primarily attributed to the introduction of the HBI ligand, which increases the active sites on the material’s surface, thereby providing a higher selectivity for U(VI) adsorption.
In conclusion, COF materials demonstrate significant selectivity and efficiency in the removal of uranium. By incorporating specific functional groups, such as amidoxime, phosphate, and carboxyl groups, and optimizing the material structure, the adsorption capacity of COFs for UO22+ can be greatly enhanced. The ordered porous structures and adjustable chemical properties of COFs make them highly promising candidates for applications in radioactive wastewater treatment and environmental remediation. However, despite these advantages, challenges remain in enriching and extracting U(VI) from seawater and wastewater. Many COF composite materials tend to collapse or undergo hydrolysis due to their weak structural affinity and strength. To overcome these challenges and further enhance the adsorption capacity of COF materials for U(VI), the design of adsorbents should account for multiple factors and their synergistic effects, including the affinity of adsorption sites, the number and stability of chelation sites, and porosity. Future research should focus on optimizing the design of COF materials to improve their effectiveness in real-world environments and expand their broader application in environmental pollution control.
GO is a single-layer material derived from graphite through oxidation, typically using methods like the Hummers method (Marcano et al., 2018). This process oxidizes graphite with strong acids and oxidizing agents to form multilayered graphite oxide, which is then exfoliated by ultrasonic treatment to obtain single-layer GO. Its structure consists of a carbon-based honeycomb lattice decorated with oxygen-containing functional groups, such as hydroxyl, carboxyl, and epoxy groups. These groups give GO unique properties, including high hydrophilicity, excellent dispersibility in water, and rich chemical reactivity. In uranium adsorption, these functional groups facilitate strong interactions with UO22+, enhancing GO’s effectiveness in environmental remediation. Additionally, GO’s high specific surface area provides ample active sites for adsorption. Its excellent mechanical flexibility allows it to be incorporated into various composite materials for enhanced performance. Moreover, GO’s tunable functionalization enables improved selectivity for uranium ions in complex aqueous environments.
Reduced graphene oxide (rGO) is obtained by reducing GO to remove some of its oxygen-containing groups, which increases its electrical conductivity while retaining some functional groups for chemical interactions. The reduction process partially restores the electronic properties of graphene, making rGO more hydrophobic than GO but still capable of interacting with metal ions. The remaining functional groups in rGO allow for the adsorption of uranyl ions, and its high surface area contributes to its adsorption capacity. The combination of electrical conductivity and chemical reactivity makes rGO useful in applications requiring both adsorption and electronic sensing capabilities. Figure 7 shows the structure of graphene oxide and reduced graphene oxide.
Figure 7. (A) Structures of graphite, graphene oxide, and reduced graphene oxide; (B) Possible chemical interactions of graphite, graphene oxide and reduced graphene oxide (by amination) with uranium (Verma and Kim, 2022).
The adsorption of uranium on the surface of heterogeneous adsorbents, such as GO and rGO, is significantly influenced by the pH of the solution due to the varying forms of uranium species under different pH conditions. At pH levels below 4, uranium primarily exists as the cationic species UO22+, with a small fraction as UO2 (OH)+ and (UO2)2(OH)22+. Between pH 4 and 8, uranium species such as (UO2)3(OH)5+ and (UO2)4(OH) 7+ become dominant, while at pH levels above 8, anionic species like (UO2)3(OH)7− and UO2(OH)3− prevail.
GO, which bears oxygen-containing groups such as hydroxyl, carboxyl, and epoxy, carries a negative surface charge across a broad pH range (2–9) (Verma and Dutta, 2015). This negative charge makes GO and its composites effective adsorbents for cationic uranium species through mechanisms such as coordination, electrostatic interactions, hydrogen bonding, and π-π interactions. As the pH increases, the negative surface charge of GO becomes more pronounced, enhancing the adsorption of positively charged uranium species. At low pH levels, the adsorption efficiency is reduced due to competition between H+ ions and uranium species for active sites on the GO surface. For example, pure GO showed improved uranium removal efficiencies from 50% to 66% as the pH increased from 2 to 11 (Wang et al., 2020). Similarly, Cetyltrimethylammonium bromide-modified GO (MGO) demonstrated a marked increase in uranium removal efficiency from 50% at pH 2 to nearly 99% at neutral pH 7, due to favorable electrostatic interactions between the cationic uranium species and the negatively charged MGO surface (Wang et al., 2020). However, at pH levels above 7, the efficiency slightly decreases as anionic uranium species form, yet overall effectiveness remains above 90%. In the case of graphene oxide-activated carbon felt (GO-ACF) composites, maximum uranium adsorption was observed at pH 5.3, with reduced efficiency at lower pH levels due to competitive adsorption of H+ ions (Chen et al., 2013).
GO and rGO are effective in uranium removal because of their unique structures and functional groups (Figure 7B). GO, produced by oxidizing graphite, has abundant oxygen-containing groups that facilitate uranium adsorption. Meanwhile, rGO, formed by reducing GO, partially restores the electrical properties of graphene while retaining sufficient functional groups to bind uranium. The π-conjugated structure of rGO further aids in uranium capture, making both GO and rGO highly efficient for environmental remediation due to their strong interactions with uranium and structural versatility.
Graphene-based adsorbents, particularly those with abundant oxygen functional groups, play a key role in uranium adsorption. The effectiveness of these adsorbents depends heavily on synthesis conditions, influencing both the amount and type of functional groups present (Table 3). Few-layered GO prepared using a modified Hummer’s method shows high performance due to its large surface area and high oxygen content, achieving a high partition coefficient (PC) and removing nearly 99% of uranium at pH 5.0. In contrast, expanded graphite-derived GOs exhibit higher adsorption capacity but lower PC, indicating reduced efficiency due to the presence of more unadsorbed U species. The PC reflects the effectiveness of actual removal, while adsorption capacity more reflects the maximum adsorption ability under ideal conditions. Thus, both adsorption capacity and PC are critical for evaluating the performance of graphene-based adsorbents.
The adsorption capacity of GO for uranium can be enhanced by chemically modifying its oxygen-containing functional groups, particularly by incorporating nitrogen-containing groups such as amine, amide, imine, and oximes. For example, GO-polyethyleneimine (GO-PEI) macrostructures achieved a high PC of 513.40 mg·g−1·µM−1 and an adsorption capacity of 215.6 mg/g at pH 5 due to the strong coordination between uranium ions and amino and oxygen groups (Huang et al., 2018). In contrast, the capacity decreased at lower pH levels due to repulsion between cationic uranium ions and protonated sites. Additionally, oxime-functionalized adsorbents (RGO-PDA/oxime) showed excellent uranium adsorption with a capacity of 1,049 mg/g, attributed to the high affinity of oxime molecules for uranium ions and the stable molecular bridging provided by polydopamine (PDA) (Qian et al., 2018). Other factors, such as the porous 3D network and large surface area of RGO-PDA/oxime, further enhance U uptake (Gunathilake et al., 2015).
GO exhibits extreme hydrophilicity in aqueous solutions due to the presence of many oxygen-containing polar functional groups, making post-separation in wastewater treatment challenging. A solution is to load magnetic nanomaterials onto GO sheets, enabling easy separation with an external magnetic field and enhancing adsorption capacity. Magnetic GO-based adsorbents, such as mGO-PP, demonstrated over 90% uranium removal from simulated wastewater within 30 min, with an adsorption capacity of 200.4 mg/g and a high PC of 4,747.6 mg·g−1·µM−1 (Dai et al., 2019). In real mine wastewater, mGO-PP selectively adsorbed uranium with a removal rate of 93.68%, and the residual uranium concentration was well below the WHO limit of 30 μg/L (Verma and Dutta, 2015). This suggests that GO adsorbents with nitrogen functional groups are highly effective for the selective removal of uranium from both artificial and real wastewater.
MXene materials include layered transition metal carbides, carbonitrides, and nitrides, which have a two-dimensional hexagonal structure and the chemical formula Mn+1Xn. They are primarily obtained by etching away the weaker A layers from layered ternary metal ceramic materials (MAX phases, with the general formula Mn+1Xn. In these formulas, M represents early transition metals (such as Sc, Ti, Zr, Hf, V, Nb, Ta, Cr, and Mo), A is an element from groups 13 and 14 of the periodic table, and X is C or N, with n = 1–4 (Figure 8). In 2011, Naguib et al. (2011) discovered the first MXene compound Ti3C2Tx by selectively etching Al from Ti3AlC2 using hydrofluoric acid. Here, Tx represents surface functional groups such as -O, -F, and -OH introduced during etching. Since their first appearance in 2011, MXene materials have rapidly developed due to their excellent physicochemical properties and have been widely applied in various fields such as energy storage, seawater desalination, catalysis, electromagnetic interference shielding, transparent conductive films, and electrochemical detection. MXene has demonstrated its effectiveness as an adsorbent and catalyst for environmental adsorption and catalytic degradation.
Figure 8. Typical MXene structures and compositions (Naguib et al., 2021).
For the adsorption of radionuclides and heavy metal ions, MXene materials offer a large specific surface area, abundant active sites, high ion exchange capacity, controllable interlayer spacing, and good hydrophilicity. More importantly, they inherit the chemical and structural stability, excellent thermal conductivity, and radiation resistance of their parent MAX phases. This makes MXene materials not only effective for removing common environmental pollutants such as heavy metal ions but also valuable for disposing of nuclear waste in high-heat-release and strongly radioactive environments. Research on MXenes in pollutant purification has gradually matured over the years, as indicated by key events and milestones in the purification of heavy metal ions, radionuclides, and organic pollutants, depicted in Figure 9.
Figure 9. Developmental milestones of MXenes for the elimination of heavy metal ions, radionuclides, and organic pollutants (Yu et al., 2022).
Currently, there is no universally accepted mechanism for the adsorption of uranium on MXene materials. This is due to the diverse adsorption and reduction mechanisms exhibited by MXenes, which are influenced by various factors such as material type, surface modification, environmental conditions (e.g., pH), and specific application methods. Existing studies have proposed different mechanisms (Figure 10), but their dominant roles may vary under different conditions.
a. Chemisorption and Hydrogen Bonding: Studies have shown that uranyl ions (UO22+) can form chemical bonds and hydrogen bonds with the surface of MXene materials [such as Ti3C2(OH)2]. Depending on the pH conditions, the hydroxyl groups (OH) on the MXene surface can be deprotonated or protonated, allowing uranyl ions to be adsorbed on the surface through bidentate or tridentate coordination. Experiments have demonstrated that forming a bidentate coordination structure with two U-O bonds is more energetically favorable, enhancing the adsorption capacity of uranium (Zhang C. R. et al., 2016; Zhang et al., 2017).
b. Ion Exchange Mechanism: Experiments have also shown that the deprotonation of hydroxyl groups on the MXene surface allows uranyl ions to be adsorbed through an ion exchange mechanism. This mechanism is effective across various pH ranges, particularly under acidic conditions, where it exhibits stronger adsorption performance (Wang et al., 2016; Wang et al., 2017).
c. Reduction Mechanism: Certain MXene materials (such as Ti2CTx) have demonstrated the ability to reduce U(VI) to U(IV). Through photocatalysis or electro-adsorption, electrons are transferred by photoexcitation or electric fields, reducing highly mobile U(VI) to insoluble U(IV). This reduction mechanism improves the efficiency of uranium immobilization and removal (Wang et al., 2018).
d. Electro-adsorption Mechanism: Due to their excellent conductivity and large surface area, MXene materials are also used in electro-adsorption techniques. By applying an electric field, the formation of an electric double layer (EDL) accelerates the migration and adsorption of uranyl ions, enhancing the adsorption kinetics and capacity (Li et al., 2023).
Figure 10. Proposed mechanisms for uranium adsorption by MXenes. (A) Chemisorption and hydrogen bonding (Wang et al., 2017); (B) ion exchange mechanism (Zhang L. et al., 2016); (C) reduction mechanism (Wang et al., 2018); (D) electro-adsorption mechanism (Li et al., 2023).
Recent advancements have demonstrated the potential of MXene materials in the field of uranium remediation, leveraging their unique structural and chemical properties to achieve efficient removal of uranium from aqueous solutions (Table 4). The following examples highlight the different types of MXene materials, such as V2CTx, Ti2CTx, and Ti3C2Tx, and their mechanisms of action in effectively capturing and reducing uranium under various conditions (Yu et al., 2022).
In 2016, Wang et al. (2016) first reported the use of multilayer V2CTx MXene materials for capturing U(VI) from aqueous solutions, achieving a maximum adsorption capacity of 174 mg/g. The primary mechanisms for U(VI) removal by V2CTx MXene were identified as coordination and ion exchange. Density Functional Theory calculations and Extended X-ray Absorption Fine Structure analysis revealed that U(VI) forms bidentate inner-sphere complexes by binding to heterogeneous adsorption sites on the MXene nanosheets. Additionally, the deprotonation of hydroxyl groups on the surface of V2CTx upon binding with U(VI) suggests that the adsorption process follows an ion exchange mechanism (Wang et al., 2016). Building on this initial success, researchers explored the potential of other MXenes, such as Ti2CTx and Ti3C2Tx, for uranium removal. In 2018, Wang et al. (2020) demonstrated that highly reactive Ti2CTx MXene could effectively reduce U(VI) to U(IV) and remove uranium through an adsorption-reduction strategy. This study showed that Ti2CTx MXene exhibits good adsorption of U(IV) over a wide pH range: at low pH, U(VI) is reduced to mononuclear U(IV) and binds to the MXene substrate, while at near-neutral pH, UO2+x nanoparticles adsorb onto Ti2CTx MXene, with some Ti2CTx converting to amorphous TiO2. These findings highlighted the reduction-inducing effect of Ti2CTx MXene in the removal of U(VI), which is partially dependent on the solution’s pH.
Further advancing this field, Wang et al. (2017) utilized redox and adsorption processes to effectively remove U(VI) using Ti2CTx MXene, achieving a high adsorption capacity of 470 mg/g. Lin Wang et al. also conducted experiments using Ti3C2Tx MXene intercalated with dimethyl sulfoxide (DMSO), revealing that the DMSO-intercalated Ti3C2Tx had five times the adsorption capacity of non-intercalated Ti3C2Tx, indicating the critical role of interlayer spacing in U(VI) adsorption. In another advancement, Zhang C. R. et al. (2020) enhanced the application of MXenes by modifying them with carboxyl-terminated aryl diazonium salts. This modification improved the water stability and chelating capacity of MXenes for UO22+, achieving an adsorption capacity of up to 334.8 mg/g. The study aligned with the Langmuir and pseudo-second-order adsorption models and attributed the adsorption mechanisms to inner-sphere surface complexation and electrostatic interactions. Lee et al. (2018) proposed an innovative approach to extend the interlayer spacing of Ti3C2Tx MXene by preparing and storing pristine and intercalated MXenes under hydrated conditions. This strategy enhanced the sorption efficacy for radionuclide removal through ultrafast mild temperature post-treatment of adsorption calcination, confirming the potential for successful retention of U(VI) beneath the multi-pattern Ti3C2Tx structure.
Further research by Attia et al. (2021) using DFT and extensive X-ray absorption techniques identified heterogeneous sorption sites, such as -O, -F, and -OH, in MXene components as key factors for effective U(VI) capture. A bidentate adsorption mechanism for U(VI) in V2C(OH)2 was reported, achieving an uptake efficacy of approximately 535 mg/g. Meanwhile, another study found that uranyl ions tend to coordinate with hydroxyl groups on the V sites, forming a bidentate complex with an inner-sphere structure (Jun et al., 2020). The deprotonation of hydroxyl groups after binding with U(VI) facilitated an ion exchange mechanism. Sun Q. et al. (2018) further demonstrated that strong U-O linkages with hydroxyl groups and intense interactions between uranium and oxygen atoms at active adsorption sites, as well as strong hydrogen bonds, contributed to the maximum adsorptive energy.
These findings illustrate that MXene materials, particularly when modified or functionalized, offer significant promise for the removal of uranium from aqueous environments. Their high adsorption capacities, diverse adsorption mechanisms (such as coordination, ion exchange, and reduction), and tunable chemical properties make them highly effective for environmental remediation. Ongoing research to optimize MXene materials and refine their adsorption strategies could further expand their application potential in nuclear waste management and the removal of other toxic contaminants.
In summary, recent studies on MOFs, COFs, GOs, and MXenes materials have demonstrated their significant potential for uranium (VI) removal from contaminated water. Each material exhibits unique advantages and limitations in the adsorption of heavy metal contaminants. MOFs, with their highly ordered porous structures and tunable chemical functionalities, have shown remarkable performance in uranium adsorption through strategies such as using larger organic linkers, defect engineering, functionalization, and composite formation. However, their stability and reusability in complex environments need further enhancement. COFs, featuring stable covalent bonds and highly adjustable pore sizes, have also displayed good adsorption performance, especially when functional groups like amidoxime and phosphate are introduced. Nevertheless, challenges remain regarding their stability in aqueous environments and practical reusability. GOs, owing to its abundant oxygen-containing groups and large surface area, has proven effective in metal ion adsorption. Its adsorption capacity for uranium (VI) can be significantly improved through surface functionalization, yet its high hydrophilicity in aqueous solutions may lead to separation difficulties, necessitating the use of magnetic composites for easier recovery. MXenes, an emerging two-dimensional material, offers excellent electrical conductivity and unique surface chemistry, allowing efficient adsorption and reduction of uranium (VI), particularly under photocatalytic or electro-adsorption conditions. Despite its outstanding adsorption capabilities, the chemical stability and scalability of MXene remain areas that require further investigation.
Future research should focus on the development of multifunctional materials that combine the strengths of MOFs, COFs, GOs, and MXenes to achieve synergistic effects, thereby enhancing adsorption capacity, selectivity, and stability. Additionally, efforts should be made to optimize the structural and surface chemistry of these materials to improve their chemical stability and reusability in complex environmental conditions. Advanced characterization techniques and computational simulations are essential to gain a deeper understanding of the molecular mechanisms underlying uranium (VI) adsorption, which will provide theoretical support for further material optimization.
Moreover, the practical performance of these materials should be validated in real environmental samples, and their potential for industrial-scale applications, such as radioactive waste treatment and uranium extraction from seawater, should be explored. Addressing these areas will help develop more efficient, cost-effective, and environmentally friendly materials for broad applications in environmental remediation and radioactive waste management, contributing to global efforts to address pressing environmental pollution challenges.
DL: Conceptualization, Methodology, Writing–original draft. YW: Data curation, Software, Visualization, Writing–original draft. LZ: Investigation, Software, Writing–review and editing. MG: Data curation, Investigation, Writing–original draft. SL: Writing–review and editing.
The author(s) declare that no financial support was received for the research, authorship, and/or publication of this article.
The authors declare that the research was conducted in the absence of any commercial or financial relationships that could be construed as a potential conflict of interest.
The author(s) declare that no Generative AI was used in the creation of this manuscript.
All claims expressed in this article are solely those of the authors and do not necessarily represent those of their affiliated organizations, or those of the publisher, the editors and the reviewers. Any product that may be evaluated in this article, or claim that may be made by its manufacturer, is not guaranteed or endorsed by the publisher.
Adil, H. I., Thalji, M. R., Yasin, S. A., Saeed, I. A., Assiri, M. A., Chong, K. F., et al. (2022). Metal-organic frameworks (MOFs) based nanofiber architectures for the removal of heavy metal ions. RSC Adv. 12 (3), 1433–1450. doi:10.1039/D1RA07034G
Alqadami, A. A., Naushad, M., Alothman, Z. A., and Ghfar, A. A. (2017). Novel metal-organic framework (MOF) based composite material for the sequestration of U(VI) and Th(IV) metal ions from aqueous environment. ACS Appl. Mat. Interfaces 9 (41), 36026–36037. doi:10.1021/acsami.7b10768
Attia, L. A., Youssef, M. A., and Moamen, O. A. A. (2021). Feasibility of radioactive cesium and europium sorption using valorized punica granatum peel: kinetic and equilibrium aspects. Sep. Sci. Technol. 56 (2), 217–232. doi:10.1080/01496395.2019.1708111
Bai, Z. Q., Yuan, L. Y., Zhu, L., Liu, Z. R., Chu, S. Q., Zheng, L. R., et al. (2015). Introduction of amino groups into acid-resistant MOFs for enhanced U(VI) sorption. J. Mat. Chem. A 3 (2), 525–534. doi:10.1039/C4TA04878D
Bi, R.-X., Liu, X., Lei, L., Peng, Z.-H., Wang, X.-X., Zhang, L., et al. (2024). Core-shell MOF@COF photocatalysts for synergistic enhanced U(VI) and tetracycline cleanup through space and carrier separation. Chem. Eng. J. 485, 150026. doi:10.1016/j.cej.2024.150026
Carboni, M., Abney, C. W., Liu, S. B., and Lin, W. B. (2013). Highly porous and stable metal-organic frameworks for uranium extraction. Chem. Sci. 4 (6), 2396–2402. doi:10.1039/C3SC50230A
Chen, L., Bai, Z. L., Zhu, L., Zhang, L. J., Cai, Y. W., Li, Y. X., et al. (2017). Ultrafast and efficient extraction of uranium from seawater using an amidoxime appended metal-organic framework. ACS Appl. Mat. Interfaces 9 (38), 32446–32451. doi:10.1021/acsami.7b12396
Chen, Q., Wang, W. T., Zhang, Z. P., and Yan, T. H. (2021). Research progress in adsorption of radionuclides by covalent organic frameworks. Prog. Chem. Eng. 40 (S2), 241–255. doi:10.16085/j.issn.1000-6613.2021-0951
Chen, S., Hong, J., Yang, H., and Yang, J. (2013). Adsorption of uranium (VI) from aqueous solution using a novel graphene oxide-activated carbon felt composite. J. Environ. Radioact. 126, 253–258. doi:10.1016/j.jenvrad.2013.09.002
Chen, S., Yuan, B., Liu, G., and Zhang, D. (2020). Electrochemical sensors based on covalent organic frameworks: a critical review. Front. Chem. 8, 601044. doi:10.3389/fchem.2020.601044
Côté, A. P., Benin, A. I., Ockwig, N. W., O'Keeffe, M., Matzger, A. J., and Yaghi, O. M. (2005). Porous, crystalline, covalent organic frameworks. Science 310 (5751), 1166–1170. doi:10.1126/science.1120411
Cui, W. R., Chen, Y. R., Xu, W., Liu, K., Qiu, W. B., Li, Y. B., et al. (2023). A three-dimensional luminescent covalent organic framework for rapid, selective, and reversible uranium detection and extraction. Sep. Purif. Technol. 306, 122726. doi:10.1016/j.seppur.2022.122726
Dai, Z. R., Sun, Y. S., Zhang, H., Ding, D. X., and Li, L. (2019). Highly efficient removal of uranium(VI) from wastewater by polyamidoxime/polyethyleneimine magnetic graphene oxide. J. Chem. Eng. Data 64 (12), 5797–5805. doi:10.1021/acs.jced.9b00759
De Decker, J., Rochette, J., De Clercq, J., Florek, J., and Van Der Voort, P. (2017). Carbamoylmethylphosphine oxide-functionalized MIL-101(Cr) as highly selective uranium adsorbent. Anal. Chem. 89 (11), 5678–5682. doi:10.1021/acs.analchem.7b00821
Dutta, R. K., Shaida, M. A., Singla, K., and Das, D. (2019). Highly efficient adsorptive removal of uranyl ions by a novel graphene oxide reduced by adenosine 5-monophosphate (RGO-AMP). J. Mat. Chem. A 7 (2), 664–678. doi:10.1039/c8ta09746a
Gunathilake, C., Gorka, J., Dai, S., and Jaroniec, M. (2015). Amidoxime-modified mesoporous silica for uranium adsorption under seawater conditions. J. Mat. Chem. A 3, 11650–11659. doi:10.1039/C5TA02863A
Guo, R. X., Liu, Y., Huo, Y. Z., Zhang, A. R., Hong, J. H., and Ai, Y. J. (2022). Chelating effect between uranyl and pyridine N containing covalent organic frameworks: a combined experimental and DFT approach. J. Colloid Interface Sci. 606, 1617–1626. doi:10.1016/j.jcis.2021.08.118
Hoskins, B. F., and Robson, R. (1989). Infinite polymeric frameworks consisting of three dimensionally linked rod-like segments. J. Am. Chem. Soc. 111 (15), 5962–5964. doi:10.1021/ja00197a079
Huang, Z. W., Li, Z. J., Wu, Q. Y., Zheng, L. R., Zhou, L. M., Chai, Z. F., et al. (2018). Simultaneous elimination of cationic uranium(vi) and anionic rhenium(vii) by graphene oxide-poly(ethyleneimine) macrostructures: a batch, XPS, EXAFS, and DFT combined study. Environ. Sci-Nano 5 (9), 2077–2087. doi:10.1039/c8en00677f
Hwang, S. K., Kang, S. M., Rethinasabapathy, M., Roh, C., and Huh, Y. S. (2020). MXene: an emerging two-dimensional layered material for removal of radioactive pollutants. Chem. Eng. J. 397, 125428. doi:10.1016/j.cej.2020.125428
Jun, B. M., Park, C. M., Heo, J., and Yoon, Y. (2020). Adsorption of Ba2+ and Sr2+ on Ti3C2Tx MXene in model fracking wastewater. J. Environ. Manag. 256, 109940. doi:10.1016/j.jenvman.2019.109940
Kumar, J. A., Prakash, P., Krithiga, T., Amarnath, D. J., Premkumar, J., Rajamohan, N., et al. (2022). Methods of synthesis, characteristics, and environmental applications of MXene: a comprehensive review. Chemosphere 286, 131607. doi:10.1016/j.chemosphere.2021.131607
Lee, I., Kim, S. H., Rethinasabapath, M., Haldorai, Y., Lee, G. W., Choe, S. R., et al. (2018). Porous 3D Prussian blue/cellulose aerogel as a decorporation agent for removal of ingested cesium from the gastrointestinal tract. Sci. Rep. 8, 4540. doi:10.1038/s41598-018-22715-w
Li, F. F., Cui, W. R., Jiang, W., Zhang, C. R., Liang, R. P., and Qiu, J. D. (2020). Stable sp2 carbon-conjugated covalent organic framework for detection and efficient adsorption of uranium from radioactive wastewater. J. Hazard. Mat. 392, 122333. doi:10.1016/j.jhazmat.2020.122333
Li, J., Yang, X. D., Bai, C. Y., Tian, Y., Li, B., Zhang, S., et al. (2015). A novel benzimidazole-functionalized 2-D COF material: synthesis and application as a selective solid-phase extractant for separation of uranium. J. Colloid Interface Sci. 437, 211–218. doi:10.1016/j.jcis.2014.09.046
Li, J. Q., Gong, L. L., Feng, X. F., Zhang, L., Wu, H. Q., Yan, C. S., et al. (2017). Direct extraction of U(VI) from alkaline solution and seawater via anion exchange by metal-organic framework. Chem. Eng. J. 316, 154–159. doi:10.1016/j.cej.2017.01.046
Li, L., Wu, H., Chen, J. J., Xu, L. X., Sheng, G. D., Fang, P., et al. (2021). Anchoring nanoscale iron sulfide onto graphene oxide for the highly efficient immobilization of uranium (VI) from aqueous solutions. J. Mol. Liq. 332, 115910. doi:10.1016/j.molliq.2021.115910
Li, N., Yang, L., Su, R. D., Shi, N., Wu, J. K., Zhao, J., et al. (2023). Selective extraction of uranium from seawater on amidoximated MXene/metal-organic framework architecture under an electric field. Desalin 566, 116940. doi:10.1016/j.desal.2023.116940
Li, Z. D., Zhang, H. Q., Xiong, X. H., and Luo, F. (2019). U(VI) adsorption onto covalent organic frameworks-TpPa-1. J. Solid State Chem. 277, 484–492. doi:10.1016/j.jssc.2019.06.044
Liang, Y., Gu, P. C., Yao, W., Yu, S. J., Wang, J., and Wang, X. K. (2017). Adsorption of radionuclide uranium onto carbon-based nanomaterials from aqueous systems. Prog. Chem. 29 (9), 1062–1071. doi:10.7536/pc170427
Lin, X. B., Xin, W. W., Chen, S. S., Song, Y., Yang, L. S., Qian, Y. C., et al. (2023). Skeleton engineering of rigid covalent organic frameworks to alter the number of binding sites for improved radionuclide extraction. J. Hazard. Mat. 458, 131978. doi:10.1016/j.jhazmat.2023.131978
Lingamdinne, L. P., Choi, Y. L., Kim, I. S., Yang, J. K., Koduru, J. R., and Chang, Y. Y. (2017). Preparation and characterization of porous reduced graphene oxide based inverse spinel nickel ferrite nanocomposite for adsorption removal of radionuclides. J. Hazard. Mat. 326, 145–156. doi:10.1016/j.jhazmat.2016.12.035
Liu, C., Li, Y., Lei, M., Liu, D. X., Li, B. L., Fu, C. B., et al. (2024). Interlayer manipulation of bio-inspired Ti3C2Tx nanocontainer through intercalation of amino acid molecules to dramatically boosting uranyl hijacking capability from seawater. J. Hazard. Mat. 469, 134002. doi:10.1016/j.jhazmat.2024.134002
Liu, H. J., Fu, T. Y., and Mao, Y. B. (2022). Metal organic framework-based materials for adsorption and detection of uranium(VI) from aqueous solution. Acs Omega 7 (17), 14430–14456. doi:10.1021/acsomega.2c00597
Liu, R., Wang, Z. Q., Liu, Q. Y., Luo, F., and Wang, Y. L. (2019). A zinc MOF with carboxylate oxygen-functionalized pore channels for uranium(VI) sorption. Eur. J. Inorg. Chem. 2019 (5), 735–739. doi:10.1002/ejic.201801295
Liu, W., Dai, X., Bai, Z., Wang, Y., Yang, Z., Zhang, L., et al. (2017). Highly sensitive and selective uranium detection in natural water systems using a luminescent mesoporous metal-organic framework equipped with abundant Lewis basic sites: a combined batch, X-ray absorption spectroscopy, and first principles simulation investigation. Environ. Sci. Technol. 51 (7), 3911–3921. doi:10.1021/acs.est.6b06305
Liu, X., Li, J. X., Wang, X. X., Chen, C. L., and Wang, X. K. (2015). High performance of phosphate-functionalized graphene oxide for the selective adsorption of U(VI) from acidic solution. J. Nucl. Mat. 466, 56–64. doi:10.1016/j.jnucmat.2015.07.027
Luo, B. C., Yuan, L. Y., Chai, Z. F., Shi, W. Q., and Tang, Q. (2016). U(VI) capture from aqueous solution by highly porous and stable MOFs: UiO-66 and its amine derivative. J. Radioanal. Nucl. Chem. 307 (1), 269–276. doi:10.1007/s10967-015-4108-3
Marcano, D. C., Kosynkin, D. V., Berlin, J. M., Sinitskii, A., Sun, Z. Z., Slesarev, A. S., et al. (2018). Improved synthesis of graphene oxide. Acs Nano 12 (2), 2078. doi:10.1021/acsnano.8b00128
Mei, D., Liu, L., and Yan, B. (2023). Adsorption of uranium (VI) by metal-organic frameworks and covalent-organic frameworks from water. Coord. Chem. Rev. 475, 214917. doi:10.1016/j.ccr.2022.214917
Mei, D., and Yan, B. (2023). β-Ketoenamine and benzoxazole-linked covalent organic frameworks tailored with phosphorylation for efficient uranium removal from contaminated water systems. Adv. Funct. Mat. 34, 2313314. doi:10.1002/adfm.202313314
Meng, C., Gao, X., Zou, S., Na, B., Feng, W., Dai, Y., et al. (2022). Unraveling the adsorption behaviors of uranium and thorium on the hydroxylated titanium carbide MXene. Comput. Mat. Sci. 210, 111460. doi:10.1016/j.commatsci.2022.111460
Min, X., Yang, W. T., Hui, Y. F., Gao, C. Y., Dang, S., and Sun, Z. M. (2017). Fe3O4@ ZIF-8: a magnetic nanocomposite for highly efficient UO22+ adsorption and selective UO22+/Ln3+ separation. Chem. Commun. 53 (30), 4199–4202. doi:10.1039/c6cc10274c
Naguib, M., Barsoum, M. W., and Gogotsi, Y. (2021). Ten years of progress in the synthesis and development of MXenes. Adv. Mat. 33, 2103393. doi:10.1002/adma.202103393
Naguib, M., Kurtoglu, M., Presser, V., Lu, J., Niu, J., Heon, M., et al. (2011). Two-dimensional nanocrystals produced by exfoliation of Ti3AlC2. Adv. Mater 23 (37), 4248–4253. doi:10.1002/adma.201102306
Niu, J., Liu, M., Xu, F., Zhang, Z., Dou, M., and Wang, F. (2018). Synchronously boosting gravimetric and volumetric performance: biomass-derived ternary-doped microporous carbon nanosheet electrodes for supercapacitors. Carbon 140, 664–672. doi:10.1016/j.carbon.2018.08.036
Qian, Y. X., Yuan, Y. H., Wang, H. L., Liu, H., Zhang, J. X., Shi, S., et al. (2018). Highly efficient uranium adsorption by salicylaldoxime/polydopamine graphene oxide nanocomposites. J. Mat. Chem. A 6 (48), 24676–24685. doi:10.1039/c8ta09486a
Sun, Q., Aguila, B., Earl, L. D., Abney, C. W., Wojtas, L., Thallapally, P. K., et al. (2018). Covalent organic frameworks as a decorating platform for utilization and affinity enhancement of chelating sites for radionuclide sequestration. Adv. Mat. 30, 1705479. doi:10.1002/adma.201705479
Sun, Y. L., Jin, D., Sun, Y., Meng, X., Gao, Y., Dall'Agnese, Y., et al. (2018). g-C3N4/Ti3C2Tx (MXenes) composite with oxidized surface groups for efficient photocatalytic hydrogen evolution. J. Mat. Chem. A 6 (19), 9124–9131. doi:10.1039/c8ta02706d
Vaddanam, V. S., Pamarthi, A., Sengupta, S., Sahoo, M., Gupta, S. K., Balija, S., et al. (2023). Acid-Resistant luminescent Si/UiO-66-Amidoxime (AO) nanostructures for rapid and efficient recovery of U(VI): experimental and theoretical studies. ACS Appl. Nano Mat. 6 (10), 8222–8237. doi:10.1021/acsanm.3c00477
Verma, S., and Dutta, R. K. (2015). A facile method of synthesizing ammonia modified graphene oxide for efficient removal of uranyl ions from aqueous medium. RSC Adv. 5 (94), 77192–77203. doi:10.1039/c5ra10555b
Verma, S., and Kim, K. H. (2022). Graphene-based materials for the adsorptive removal of uranium in aqueous solutions. Environ. Int. 158, 106944. doi:10.1016/j.envint.2021.106944
Wang, C. L., Li, Y., and Liu, C. L. (2015). Sorption of uranium from aqueous solutions with graphene oxide. J. Radioanal. Nucl. Chem. 304 (3), 1017–1025. doi:10.1007/s10967-014-3855-x
Wang, J., Fang, F., Zhou, Y. C., Yin, M. L., Liu, J., Wang, J. W., et al. (2020). Facile modification of graphene oxide and its application for the aqueous uranyl ion sequestration: insights on the mechanism. Chemosphere 258, 127152. doi:10.1016/j.chemosphere.2020.127152
Wang, L., Song, H., Yuan, L., Li, Z., Zhang, Y., Gibson, J. K., et al. (2018). Efficient U(VI) reduction and sequestration by Ti2CTx MXene. Environ. Sci. Technol. 52 (18), 10748–10756. doi:10.1021/acs.est.8b03711
Wang, L., Tao, W. Q., Yuan, L. Y., Liu, Z. R., Huang, Q., Chai, Z. F., et al. (2017). Rational control of the interlayer space inside two-dimensional titanium carbides for highly efficient uranium removal and imprisonment. Chem. Commun. 53 (89), 12084–12087. doi:10.1039/c7cc06740b
Wang, L., Yuan, L. Y., Chen, K., Zhang, Y. J., Deng, Q. H., Du, S. Y., et al. (2016). Loading actinides in multilayered structures for nuclear waste treatment: the first case study of uranium capture with vanadium carbide MXene. ACS Appl. Mat. Interfaces 8 (25), 16396–16403. doi:10.1021/acsami.6b02989
Wang, M., Zhang, S., Li, Q., Li, Y. P., Duan, E. Z., Wen, C. M., et al. (2024). Insights into enhanced immobilization of uranyl carbonate from seawater by Fe-doped MXene. Sci. Total Environ. 920, 170850. doi:10.1016/j.scitotenv.2024.170850
Wang, S. Y., Wei, G., Xie, Y. H., Shang, H. L., Chen, Z. S., Wang, H. Q., et al. (2022). Constructing nanotraps in covalent organic framework for uranium sequestration. Sep. Purif. Technol. 303, 122256. doi:10.1016/j.seppur.2022.122256
Wang, Y. F., Lu, Z., Luo, M. D., Zhao, Z. Q., Wei, Y. Y., and Wang, H. H. (2024). Enhanced uranium adsorption performance of porous MXene nanosheets. Sep. Purif. Technol. 335, 126134. doi:10.1016/j.seppur.2023.126134
Wu, X. M., Huang, Q. X., Mao, Y., Wang, X. X., Wang, Y. Y., Hu, Q. H., et al. (2019). Sensors for determination of uranium: a review. Trac. Trends Anal. Chem. 118, 89–111. doi:10.1016/j.trac.2019.04.026
Xiong, X. H., Yu, Z. W., Gong, L., Tao, Y., Gao, Z., Wang, L., et al. (2019). Ammoniating covalent organic framework (COF) for high-performance and selective extraction of toxic and radioactive uranium ions. Adv. Sci. 6 (16), 1900547. doi:10.1002/advs.201900547
Yang, A. L., Zhu, Y. K., and Huang, C. P. (2018). Facile preparation and adsorption performance of graphene oxide-manganese oxide composite for uranium. Sci. Rep. 8, 9058. doi:10.1038/s41598-018-27111-y
Yang P. P, P. P., Liu, Q., Liu, J. Y., Zhang, H. S., Li, Z. S., Li, R. M., et al. (2018). Interfacial growth of a metal-organic framework (UiO-66) on functionalized graphene oxide (GO) as a suitable seawater adsorbent for extraction of uranium(VI). J. Mat. Chem. A 6 (17), 8121. doi:10.1039/c8ta90090f
Yang, T. T., Tian, C., Yan, X., Xiao, R. Y., and Lin, Z. (2021). Rational construction of covalent organic frameworks with multi-site functional groups for highly efficient removal of low-concentration U(vi) from water. Environ. Sci-Nano 8 (5), 1469–1480. doi:10.1039/d1en00059d
You, Z. X., Zhang, N., Guan, Q. L., Xing, Y. H., Bai, F. Y., and Sun, L. X. (2020). High sorption capacity of U(VI) by COF-based material doping hydroxyapatite microspheres: kinetic, equilibrium and mechanism investigation. J. Inorg. Organomet. Polym. Mat. 30 (6), 1966–1979. doi:10.1007/s10904-019-01420-9
Yu, J., Lan, J., Wang, S., Zhang, P., Liu, K., Yuan, L., et al. (2021). Robust covalent organic frameworks with tailor-made chelating sites for synergistic capture of U(vi) ions from highly acidic radioactive waste. Dalton Trans. 50 (11), 3792–3796. doi:10.1039/d1dt00186h
Yu, S. J., Tang, H., Zhang, D., Wang, S. Q., Qiu, M. Q., Song, G., et al. (2022). MXenes as emerging nanomaterials in water purification and environmental remediation. Sci. Total Environ. 811, 152280. doi:10.1016/j.scitotenv.2021.152280
Yue, C. Y., Wu, L., Lin, Y. Q., Lu, Y. Q., Shang, C., Ma, R., et al. (2021). Study on the stability, evolution of physicochemical properties, and postsynthesis of metal-organic frameworks in bubbled aqueous ozone solution. ACS Appl. Mat. Interfaces 13 (22), 26264–26277. doi:10.1021/acsami.1c02213
Zhang, C. R., Cui, W. R., Jiang, W., Li, F. F., Wu, Y. D., Liang, R. P., et al. (2020). Simultaneous sensitive detection and rapid adsorption of UO22+ based on a post-modified sp2 carbon-conjugated covalent organic framework. Environ. Sci-Nano 7 (3), 842–850. doi:10.1039/c9en01225g
Zhang, L., Wang, L. L., Gong, L. L., Feng, X. F., Luo, M. B., and Luo, F. (2016). Coumarin-modified microporous-mesoporous Zn-MOF-74 showing ultra-high uptake capacity and photo-switched storage/release of UVI ions. J. Hazard. Mat. 311, 30–36. doi:10.1016/j.jhazmat.2016.01.082
Zhang, L., Wang, S. L., Zhang, G. H., Shen, N., Chen, H., Tao, G. H., et al. (2022). Design principles based on intramolecular interactions for hydroxyl-functionalized covalent organic frameworks. Cell. Rep. Phys. Sci. 3 (11), 101114. doi:10.1016/j.xcrp.2022.101114
Zhang, N., Ishag, A., Li, Y., Wang, H. H., Guo, H., Mei, P., et al. (2020). Recent investigations and progress in environmental remediation by using covalent organic framework-based adsorption method: a review. J. Clean. Prod. 277, 123360. doi:10.1016/j.jclepro.2020.123360
Zhang, P. C., Wang, L., Du, K., Wang, S. Y., Huang, Z. W., Yuan, L. Y., et al. (2020). Effective removal of U(VI) and Eu(III) by carboxyl functionalized MXene nanosheets. J. Hazard. Mat. 396, 122731. doi:10.1016/j.jhazmat.2020.122731
Zhang, Y. J., Lan, J. H., Wang, L., Wu, Q. Y., Wang, C. Z., Bo, T., et al. (2016). Adsorption of uranyl species on hydroxylated titanium carbide nanosheet: a first-principles study. J. Hazard. Mat. 308, 402–410. doi:10.1016/j.jhazmat.2016.01.053
Zhang, Y. J., Zhou, Z. J., Lan, J. H., Ge, C. C., Chai, Z. F., Zhang, P. H., et al. (2017). Theoretical insights into the uranyl adsorption behavior on vanadium carbide MXene. Appl. Surf. Sci. 426, 572–578. doi:10.1016/j.apsusc.2017.07.227
Zhao, G. X., Wen, T., Yang, X., Yang, S. B., Liao, J. L., Hu, J., et al. (2019). Preconcentration of U(vi) ions on few-layered graphene oxide nanosheets from aqueous solutions. Dalton Trans. 48 (19), 6645. doi:10.1039/c9dt90096a
Keywords: uranium adsorption, metal-organic frameworks (MOFs), covalent organic frameworks (COFs), graphene oxide (GO), MXenes
Citation: Liu D, Wang Y, Zuo L, Guo M and Liu S (2025) Advanced materials for uranium adsorption: a mini review of recent developments. Front. Mater. 12:1541204. doi: 10.3389/fmats.2025.1541204
Received: 07 December 2024; Accepted: 13 January 2025;
Published: 10 April 2025.
Edited by:
Junqi Wang, Xi’an Jiaotong University, ChinaReviewed by:
Mohammad R. Thalji, Korea Institute of Energy Technology (KENTECH), Republic of KoreaCopyright © 2025 Liu, Wang, Zuo, Guo and Liu. This is an open-access article distributed under the terms of the Creative Commons Attribution License (CC BY). The use, distribution or reproduction in other forums is permitted, provided the original author(s) and the copyright owner(s) are credited and that the original publication in this journal is cited, in accordance with accepted academic practice. No use, distribution or reproduction is permitted which does not comply with these terms.
*Correspondence: Shun Liu, bGl1c2h1bkB3aGh3dGVjaC5jb20=
Disclaimer: All claims expressed in this article are solely those of the authors and do not necessarily represent those of their affiliated organizations, or those of the publisher, the editors and the reviewers. Any product that may be evaluated in this article or claim that may be made by its manufacturer is not guaranteed or endorsed by the publisher.
Research integrity at Frontiers
Learn more about the work of our research integrity team to safeguard the quality of each article we publish.