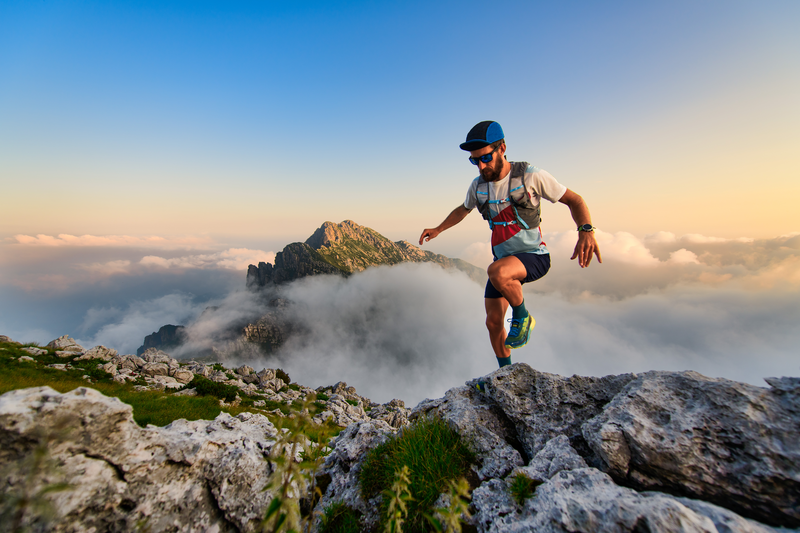
94% of researchers rate our articles as excellent or good
Learn more about the work of our research integrity team to safeguard the quality of each article we publish.
Find out more
ORIGINAL RESEARCH article
Front. Mater.
Sec. Polymeric and Composite Materials
Volume 12 - 2025 | doi: 10.3389/fmats.2025.1535825
The final, formatted version of the article will be published soon.
You have multiple emails registered with Frontiers:
Please enter your email address:
If you already have an account, please login
You don't have a Frontiers account ? You can register here
This study includes the synthesis of new hydrogel using pectin, chitosan, and Mo-MOF (pectin/chitosan Mo-MOF hydrogel). After confirming the structure of the synthetic hydrogel by Elemental Analysis (EA), Energy-Dispersive X-ray Spectroscopy (EDS), EDS mapping, Fouriertransform infrared spectroscopy (FT-IR), X-Ray Diffraction (XRD), Brunauer-Emmett-Teller (BET), Scanning Electron Microscopy (SEM), and Transmission Electron Microscopy (TEM), its application in wastewater treatment, including the absorption of Congo red and the inhibition of pathogenic bacterial strains in wastewater, was evaluated. The factors affecting the adsorption of Congo red, such as pH, temperature, and contact time, were studied. The highest adsorption rate was determined to be 93% using 0.06 g/L of pectin/chitosan Mo-MOF hydrogel under conditions including pH 8, temperature of 25°C, and contact time of 90 minutes. The microbiology evaluations of the pectin/chitosan Mo-MOF hydrogel, which were performed against the known strains of wastewater such as Campylobacter jejuni, Shigella dysenteriae, Vibrio cholerae, Yersinia enterocolitica, and Salmonella enterica, indicated its high antibacterial properties, so, MIC and MBC values were observed between 4-32 μg/mL and 8-64 μg/mL. The diverse characteristics of the pectin/chitosan Mo-MOF hydrogel can be attributed to its physical and chemical properties, such as its constituent compounds, specific surface area, and porosity. Finally, the pectin/chitosan Mo-MOF hydrogel can be introduced as a functional composition with unique capabilities in controlling pathogenic bacterial strains of wastewater and absorbing dangerous chemical compounds of wastewater for environmental purposes.
Keywords: wastewater treatment, Congo red absorption, Wastewater microbial strain, Metal-Organic Frameworks, Hydrogel, Molybdenum
Received: 27 Nov 2024; Accepted: 10 Mar 2025.
Copyright: © 2025 Ghnim, Adhab, Altalbawy, Salih Mahdi, Salah Mansoor, Radi, Saadoun Abd, Abdul- Reda Hussein, Hussain Hamza, MUZAMMIL and Alkhayyat. This is an open-access article distributed under the terms of the Creative Commons Attribution License (CC BY). The use, distribution or reproduction in other forums is permitted, provided the original author(s) or licensor are credited and that the original publication in this journal is cited, in accordance with accepted academic practice. No use, distribution or reproduction is permitted which does not comply with these terms.
* Correspondence:
Ahmad Alkhayyat, Department of computers Techniques engineering, College of technical engineering, The Islamic University, Najaf, Iraq, Najaf, Iraq
Disclaimer: All claims expressed in this article are solely those of the authors and do not necessarily represent those of their affiliated organizations, or those of the publisher, the editors and the reviewers. Any product that may be evaluated in this article or claim that may be made by its manufacturer is not guaranteed or endorsed by the publisher.
Research integrity at Frontiers
Learn more about the work of our research integrity team to safeguard the quality of each article we publish.