- School of Civil and Transportation Engineering, Beijing University of Civil Engineering and Architecture, Beijing, China
Natural zeolite is commonly used as an adsorbent for road dust suppression due to its excellent moisture absorption properties. However, during the formation process, natural zeolite tends to accumulate some zeolite water, organic impurities, and metal cations that occupy the pore channels and cavities, thereby blocking the passage of water molecules and hindering their adsorption. This study aims to improve the weak moisture absorption rate and capacity of dust suppression asphalt mixtures caused by clogged pores and impurities in natural zeolite. To achieve this different concentrations of salt solutions (NH4Cl, NaCl, MgCl2, and CaCl2) are used as modifiers for the treatment of natural zeolite. Scanning electron microscopy and Fourier transform infrared spectroscopy are used to study the interface and functional group changes between the modified zeolite substrate and asphalt mixture. The nitrogen adsorption/desorption curves of asphalt mixtures with different dosages were obtained using a nitrogen adsorption instrument. Based on this, parameters such as the specific surface area and adsorption pore volume of natural zeolite and modified zeolite asphalt mixtures were calculated using the BET and BJH methods. A macroscopic dust suppression test was further conducted to evaluate the dust suppression effect of the modified zeolite asphalt mixture on PM10. The results show that the moisture absorption rate and capacity of the modified zeolite are significantly improved. 20wt.%CaCl2 modification performs the best, and the moisture absorption capacity of the modified zeolite can reach up 10 times that of natural zeolite. Compared to the natural zeolite asphalt mixture, the specific surface area of the modified zeolite asphalt mixture is increased by 16.52%, and the adsorption pore volume is increased by 44.9%. The addition of modified zeolite powder significantly improves the dust suppression performance of the asphalt mixture. When the dosage of modified zeolite powder reaches 80%, the dust suppression effect reaches its peak, with an improvement of 16.34%.
1 Introduction
With the rapid urbanization process, road dust has become a new challenge in road maintenance and management (Bhaskar and Sharma, 2008; Edvardsson et al., 2012). Common measures to suppress road dust include sprinkling water and spraying dust suppressants (Wingenfelder et al., 2005). However, these methods not only require a significant amount of manpower and resources, but also have limitations such as high cost, easy erosion, and short dust suppression effectiveness, resulting in limited contributions to road dust suppression.
Currently, few scholars have explored the use of road material modification to incorporate dust suppression adsorbents into asphalt mixtures for road construction. This enables the road surface to have the “adsorption behavior” of autonomously absorbing moisture from the air to control road dust,as shown in Figure 1. Road material modification is a common method to meet the functional requirements of roads. In the development of road material modification technology, porous material modifiers play an important role, giving rise to various road materials with excellent performance or other urban adjustment functions (Shekarchi et al., 2023; Usmani et al., 2020; Martinez-Soto et al., 2023). These modified road materials can have long-lasting effects throughout the lifetime of the road. The selection of road dust suppressants is crucial for dust suppression asphalt concrete pavements. It is important to choose materials with excellent moisture absorption rate and capacity, such as porous adsorbents with ‘moisture absorption and retention’ functionality (Makarov et al., 2021; Rafique et al., 2016).
Natural zeolite is a mineral composed of hydrated aluminosilicate, with silicate and aluminate tetrahedra arranged in a framework structure (Ghasemi et al., 2023). The presence of the framework structure increases the internal space of zeolite, resulting in a large number of cavities and pore channels with a high specific surface area (Mohammad et al., 2023). Zeolite has a wide range of pore size distribution. When the diameter of the adsorbed molecule is smaller than the zeolite pore size, the molecule can enter the zeolite pore and be adsorbed under the influence of diffusion and other external forces, indicating its selective adsorption capacity (Ng and Mintova, 2008; Song et al., 2021). However, natural zeolite often accumulates organic impurities that fill the internal cavities and pore channels of zeolite during the formation process. Zeolite water in the zeolite lattice and metal cations that exist to balance charges also occupy the pore channels and cavities, blocking the pathway for water molecules and reducing their ability to adsorb moisture (Younes et al., 2007).
To enhance the adsorption performance of natural zeolite, many scholars have conducted modification treatments on natural zeolite. Lei (2014) improved the pore structure of zeolite using methods such as heat, acid, and alkali, and studied the effects of different treatment methods on the composition, surface morphology, and moisture adjustment performance of zeolite. Sodium polyacrylate resin was synthesized on the surface of the zeolite pores based on reverse suspension, and the moisture adsorption performance of the material was accurately fitted using diffusion kinetics adsorption models and chemical reaction kinetic adsorption models, obtaining the fitted equations for the modified zeolite material’s moisture adsorption and desorption. Ates and Akgül (2016) modified natural zeolite using NaOH (0.5–2.0 mol/dm3) solution at different humidities and stirring times, and studied the pore structure and specific surface area of the samples through N2 adsorption-desorption experiments. The results showed that suitable humidity can obtain zeolite materials with mesopores and micropores. Longer stirring times can result in higher crystallinity and specific surface area of the samples, but it may also lead to more amorphous content. Di et al. (2018) used acid leaching, alkali leaching, and alkali leaching-hydrothermal combined modification processes to adjust the pore structure of red zeolite. The modified pore structure characteristics, composition, microstructure, and moisture adjustment performance were analyzed. The results showed that acid leaching can enhance the micro-pores and the quantity of pores with sizes of 2–10 nm in zeolite, which helps enhance the moisture adsorption. Alkali leaching can increase the quantity of mesopores with sizes of 2–50 nm, which helps enhance moisture desorption. Velarde et al. (2023) analyzed the chemical modification of natural zeolites by acid/base/salt reagent, surfactants, and metallic reagents, compared, and described. Furthermore, the adsorption/desorption capacity, systems, operating parameters, isotherms, and kinetics for natural zeolites were described and compared. Dehmani et al. (2023) introduced the different parameters for studying the adsorption mechanism of organic and inorganic pollutants using zeolites and the main adsorption processes using zeolites as adsorbents include chelation, surface adsorption, natural processes, diffusion, electrostatic interactions and complexation. Deng (2014) prepared porous mineral-based composite humidity-regulating materials using inorganic salt modification and PAA composites for minerals such as sepiolite, diatomite, and zeolite. It was believed that the surface of multi-pore mineral-based humidity-regulating materials modified with inorganic salts is coated with uniform particles. Although it leads to a slight decrease in specific surface area and pore volume, the average pore diameter increases, which improves the functionality of the material. Zhou et al. (2018) modified zeolite using microwave-assisted NH4Cl solution and prepared humidity control materials with good thermal stability and acid resistance. The humidity migration process of the composite humidity control material based on dehydrated natural zeolite as a substrate was investigated. The micro-pore morphology and spectral distribution of zeolite before and after modification were characterized using scanning electron microscopy (SEM), Fourier-transform infrared spectroscopy (FTIR), and N2 adsorption testing.
This study employed different concentrations of salt solutions (NH4Cl, NaCl, MgCl2, and CaCl2) as modifiers for the modification treatment of natural zeolite, leading to the formation of modified zeolite asphalt mixtures. The zeolite powder modified with the salt solution exhibiting the strongest hygroscopicity was selected based on hygroscopicity experiments as the hygroscopic modifier. The modified zeolite-based materials and the interface between modified zeolite and asphalt were investigated through scanning electron microscopy and Fourier-transform infrared spectroscopy. Nitrogen adsorption experiments were conducted to obtain adsorption/desorption curves for asphalt mixtures with different modifier concentrations. Subsequently, the Brunauer–Emmett–Teller (BET) method and Barrett–Joyner–Halenda (BJH) method were employed to calculate parameters such as specific surface area and adsorption pore volume for both natural zeolite and modified zeolite asphalt mixtures. Integrated with macroscopic dust suppression tests, the dust suppression effect of the modified zeolite asphalt mixture on PM10 was quantitatively evaluated.
2 Materials and methods
2.1 Materials
2.1.1 SBS-modified asphalt
In order to enhance the high temperature stability and water stability of the asphalt mixture, this experiment selected SBS modified asphalt produced from a building materials factory in Beijing, model SBS-V205. Its basic performance indicators comply with the requirements of “standard test methods of bitumen and bituminous mixtures for highway engineering” (JTG E20-2011), as shown in Table 1.
2.1.2 Natural zeolite
The adsorbent used in this study is 200-mesh natural zeolite produced by a filter material manufacturer in Zhengzhou, Henan. The basic properties of the natural zeolite powder are shown in Table 2.
2.1.3 Aggregates
The aggregate used in this study is limestone, and the mineral powder is derived from grinding limestone, both sourced from a building materials factory in Beijing. According to the “Test Methods of Aggregates for Highway Engineering” (JTG E42-2005), the performance indicators of the aggregate and mineral powder were tested, and the test results meet the requirements specified in the regulations. The aggregate gradation is shown in Table 3.
2.1.4 Asphalt mixture proportioning design
The recommended AC-13 asphalt mixture with skid resistance is adopted in this study (Ministry of Transport of the People’s Republic of China, 2004). The ore powder is replaced with modified zeolite powder by equal volume replacement. The optimal asphalt content (OAC) ratio needs to be adjusted based on the proportion of added zeolite powder. The results are shown in Table 4.
2.2 Methods
2.2.1 Hygroscopicity test
This experiment investigates the influence of different types and concentrations of salt solutions on the moisture absorption rate and capacity of natural zeolite (Lei, 2014; Kuldeyev et al., 2023). To achieve this, natural zeolite with an appropriate particle size was selected, and the salt immersion method was used for its modification. The experimental procedure is as follows: Firstly, four inorganic salts (NH4Cl, NaCl, MgCl2 and CaCl2) were selected and the natural zeolite powders were soaked in salt solution for 72 h at three different concentrations (15wt%, 20wt% and 25wt%) with a liquid-solid ratio of 5:1. Next, the zeolite was removed and dried under low-temperature conditions, and then crushed and ground to 200 mesh using a grinder. Finally, the moisture absorption test was conducted at 80% relative humidity and 25°C.
2.2.2 SEM
The microstructure of natural zeolite and modified zeolite asphalt with different zeolite powder dosages was determined using a PHILIPS XL-30ESEM scanning electron microscope (SEM). Through SEM observation, the morphological changes of the “sea-island” structure between the adsorbent and asphalt phases were clearly identified, and the microstructure of the asphalt paste with added modified zeolite powder was analyzed.
2.2.3 FTIR
The Thermo Nicolet IS5 Fourier-transform infrared spectrometer (FTIR) was used for the experiment. In the FTIR testing, the wavenumber range was set from 4,000 to 300 cm-1, and the scanning is conducted for 16 times to observe the changes in functional groups after the addition of modified zeolite into the asphalt.
2.2.4 Nitrogen adsorption experiment for measuring specific surface area and adsorption pore volume
The nitrogen adsorption experiment enables nitrogen molecules to accumulate on the surface of the material and subsequently fill the voids within the material. Nitrogen adsorption-desorption curve was obtained during the nitrogen adsorption test, and the specific surface area and adsorption pore volume of the material were calculated using the volume method (Qi, 2023; Hu, 2021). In this experiment, the Micromeritics ASAP 2460 physical adsorption analyzer is used. Firstly, natural zeolite powder and modified zeolite powder were substituted for mineral powder at proportions of 40wt%, 60wt%, and 80wt%. Then, the asphalt mixture particles were obtained through abrasion experiments, and the particles of different sizes were separated into three intervals: 0.075–0.3, 0.3–1.18, and 1.18–2.36. Nitrogen adsorption experiments were conducted for each group of samples. Based on the specifications “Pore size distribution and porosity of solid materials by mercury porosimetry and gas adsorption-Part 2: Analysis of mesopores and macropores by gas adsorption” (GB/T 21650.1-2008, 2006) and “Determination of the specific surface area of solids by gas adsorption using the BET method” (GB/T 19587-2017, 2024), the specific surface area and adsorption pore volume of each sample are determined.
The experimental procedure is as follows:
2.2.4.1 Specific surface area calculation
According to “Determination of specific surface area of solid materials by gas adsorption BET method” (GBT19587-2017), the specific surface area is calculated using the BET method.
First, calculate the volume of gas adsorbed by zeolite powder in a monolayer. The calculation formula is shown in Equation 1.
Where:
The specific surface area
2.2.4.2 Adsorption pore volume calculation
According to “Pore size distribution and porosity of solid materials by mercury porosimetry and gas adsorption-Part 2: Analysis of mesopores and macropores by gas adsorption” (GBT21650.2–2008), the adsorbed pore volume is calculated according to the BJH (Barrett-Joyner-Halenda) method. The calculation is based on the assumption that capillary condensation occurs within the cylindrical pore, and the diameter of the pore is twice the sum of the Kelvin radius and the thickness of the adsorbed layer. The calculation formula is shown in Equations 3–4.
Where:
The calculation formula is shown in Equations 5–10.
Where,
2.2.5 Dust suppression experiment
Currently, there is little research both domestically and internationally on the functional absorption of road dust. Additionally, there is a lack of methods and equipment for simulating and testing small-scale dust pollution. This study developed a set of dust simulation equipment through research on dust simulation methods (Wang et al., 2014), indicator parameters, and impact laws. The equipment can display the real-time changes in PM10 concentration to characterize the dust suppression effect of asphalt mixtures.
3 Results and discussion
3.1 Hygroscopic effectiveness after optimizing pore size
Figure 2 shows that the modified zeolite, treated with salt solutions of different concentrations, exhibits varying moisture absorption rates and capacities.
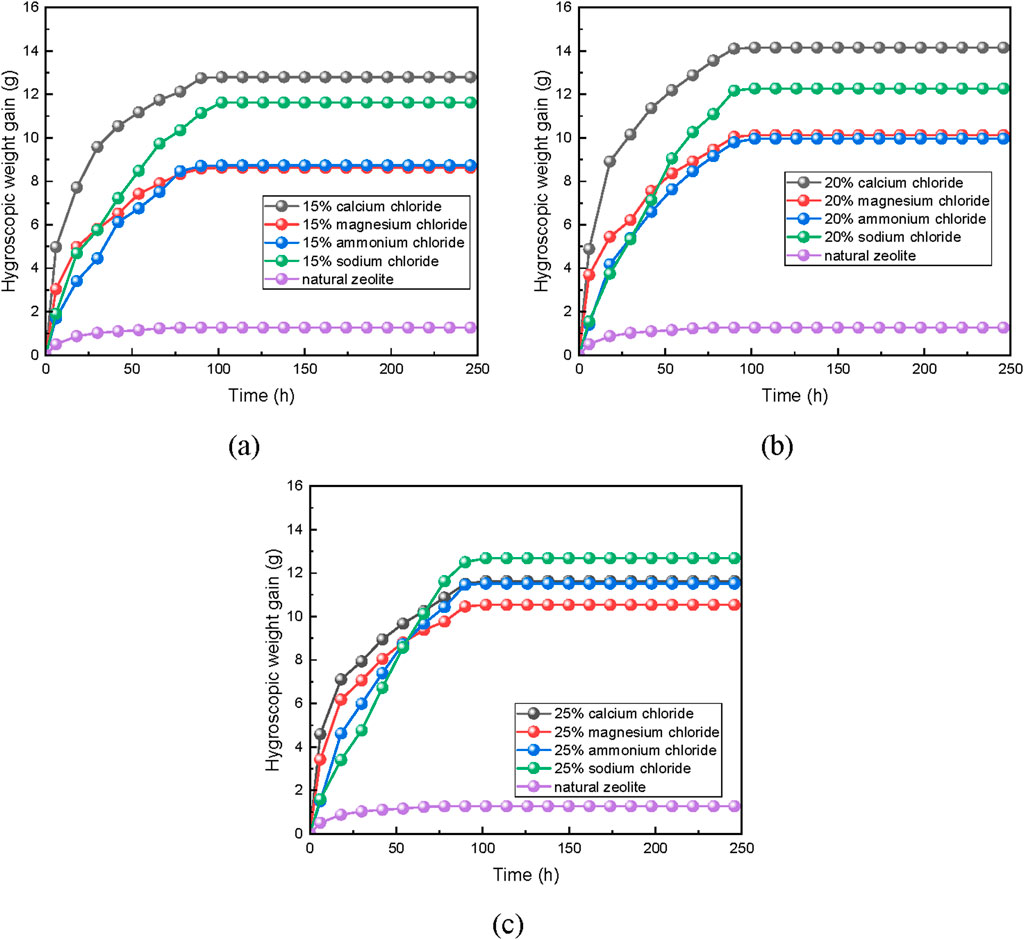
Figure 2. Hygroscopic weight gain graph of zeolite powder under different modification methods: (A) 15wt% concentration, (B) 20wt% concentration and (C) 25wt.%concentration.
Compared to natural zeolite, the modified zeolite has a higher moisture absorption capacity and rate. This is due to the accumulation of organic impurities in natural zeolite during its formation process, which fills the cavities and channels within the zeolite. Water molecules within the zeolite lattice and metal cations present for charge balance also occupy the pore and cavity spaces, obstructing the passage for water molecules and hindering their adsorption. It is important to note that this obstruction is caused by the presence of water molecules and metal cations, which occupy the pore and cavity spaces (Xie, 2018; Wang, 2019). By modifying with salt solution, zeolite’s ion-exchangeability enables the displacement of small-radius cations with larger-radius ones. This alters the activation energy of the internal surface, increases the effective pore size, reduces spatial resistance, and enhances the moisture absorption capacity and rate of the zeolite (Gordeeva and Aristov, 2012).
It can also be seen from Figure 3 that the maximum moisture absorption rate and capacity of the modified zeolite increase with the concentration of salt solution within a certain range. The modification with salt solution is beneficial for improving the moisture absorption rate and capacity of zeolite (Jänchen et al., 2004; Hu et al., 2018). For example, when natural zeolite powder is modified with 20wt% concentration of NH4Cl, NaCl, MgCl2, and CaCl2, the maximum moisture absorption capacities are 9.96%, 12.27%, 10.13%, and 14.15%, respectively. This represents an increase of 7.57 times, 7.69 times, 9.32 times, and 10.24 times compared to untreated natural zeolite.
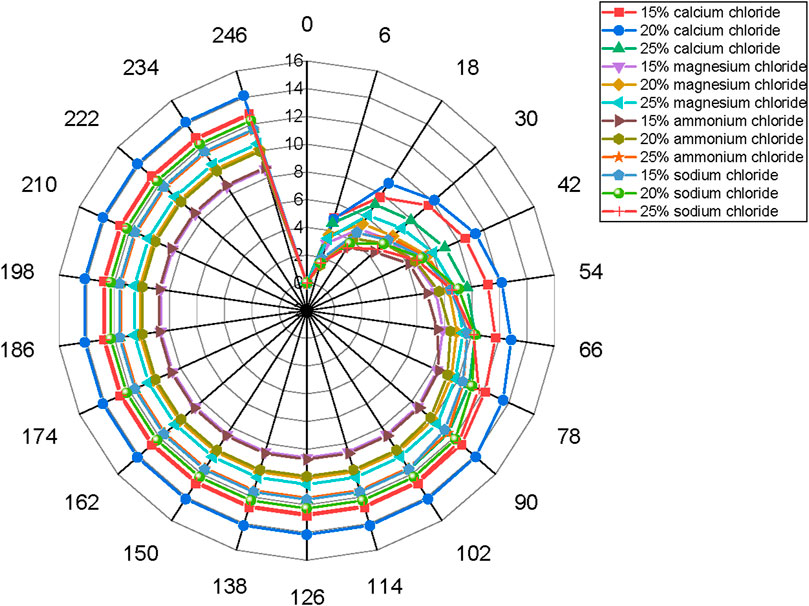
Figure 3. The hygroscopicity observed after treatment with salt solutions of varying concentrations.
The moisture absorption rates of zeolite powder treated with CaCl2 are as follows: 20%wt.CaCl2 > 15%wt.CaCl2 > 25%wt.CaCl2. The moisture absorption rate does not necessarily increase with the increase in concentration. This is because the salt solution treatment can dissolve the organic impurities and weakly crystalline aluminum within the zeolite. At lower concentrations of salt solution, the ability to remove impurities and dissolve metal cations is weak, leading to less significant adjustment of the pore structure and making it less conducive to the release of water molecules. On the other hand, at higher concentrations of salt solution, the ability to remove impurities and dissolve metal cations is excessive. This resulting in larger pore sizes and actually reducing the binding force between the zeolite and water molecules.
Figure 3 shows the moisture absorption rates 120 h after the start of the test. The ranking is as follows: 20wt% CaCl2 > 15wt% CaCl2 > 25wt% NaCl >20wt% NaCl >15wt% NaCl >25wt% CaCl2 > 25wt% NH4Cl > 25wt% MgCl2 > 20wt% MgCl2 > 20wt% NH4Cl > 15wt% NH4Cl > 15wt% MgCl2. The zeolite powder modified with a 20wt% CaCl2 solution consistently maintains a moisture absorption capacity that is at least 7 times higher than that of natural zeolite powder, and it can reach up to 10 times higher. Therefore, in this study, the zeolite powder modified with a 20wt% CaCl2 solution is chosen as the moisture absorption modifier.
3.2 Study on the Co-solubility interface between modified zeolite-based materials and asphalt
Microscopic morphology characterization was conducted on mineral powder asphalt mortar, natural zeolite asphalt mortar, and modified zeolite asphalt mortar. The results are shown in Figure 5.
Based on Figures 4A–C, it can be observed that the morphology of the modified zeolite asphalt mortar is similar to that of the natural zeolite asphalt mortar and the mineral powder asphalt mortar. The asphalt phase of the mineral powder asphalt mortar exhibits slight protrusions on the surface, but overall it is relatively flat. Compared to the other two types of asphalt mortars, the modified zeolite asphalt mortar has a rougher surface morphology, which is attributed to the larger specific surface area and adsorption pore volume of the modified zeolite powder (Zheng, 2017). At a magnification of 2000 times, as shown in Figure 4D, it can be seen that the interface between the adsorbent and the asphalt exhibits subtle undulations and small wrinkles. Due to capillary adsorption (Kuldeyev et al., 2023), some asphalt penetrates the surface gaps of the adsorbent, increasing the contact area between them and enhancing the adhesive force between the asphalt and the adsorbent. The fillers are uniformly distributed and completely enveloped by the asphalt, indicating good compatibility between the modified zeolite and the asphalt.
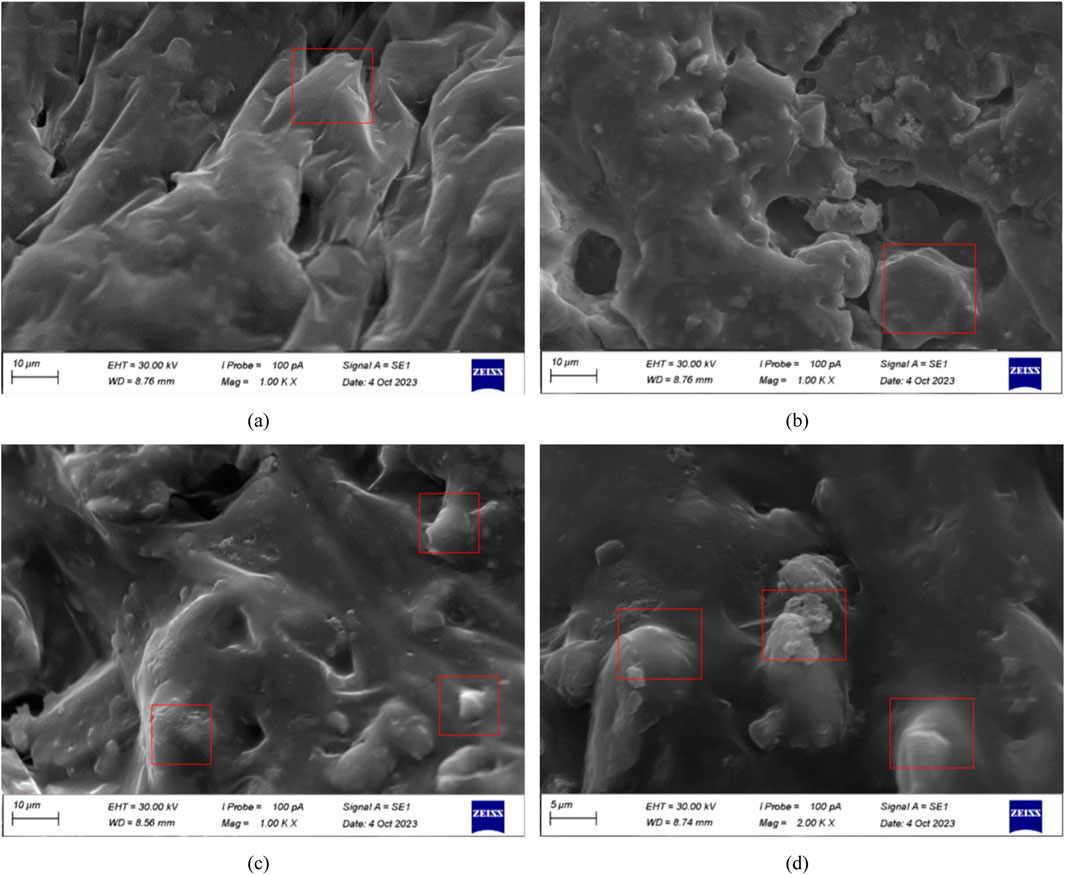
Figure 4. SEM images of asphalt binder with added modified zeolite: (A) Asphalt Blended with Mineral Powder; (B) Asphalt Blended with Natural Zeolite; (C) Asphalt Blended with Modified Zeolite; (D) Asphalt Blended with Modified Zeolite (2000 Times Dilution).
3.3 Influence of modified zeolite material on the functional groups of SBS-modified asphalt
Figure 5 shows the infrared spectra of asphalt mortar with modified zeolite-based materials and SBS asphalt mortar without any additives. The absorption peaks at 2,850.18 cm-1, 2,849.44 cm-1, 2,919.02 cm-1, and 2,917.43 cm-1 are the stretching vibration absorption peaks of -CH2-. The peaks at 966.44 cm-1 and 966.24 cm-1 are characteristic absorption peaks of (CH2CH = CHCH2)n (polybutadiene), and the peaks at 698.72 cm-1 and 698.74 cm-1 correspond to the characteristic absorption peaks of (C8H8)n (polystyrene). In the infrared spectrum, absorption peaks appear at specific wavenumbers (wave lengths) where the absorbed light is observed. The more light is absorbed at a certain wavenumber, the lower the transmittance and the stronger the absorption peak (Cheng et al., 2005). The SBS asphalt with the addition of modified zeolite-based material exhibits a new absorption peak only at 457.74 cm⁻1, which corresponds to the bending vibration peak of Si-O or Al-O in the zeolite molecules. Therefore, it can be inferred that there was no significant chemical reaction and the formation of new chemical groups between SBS asphalt and the modified zeolite-based materials during high-temperature mixing.
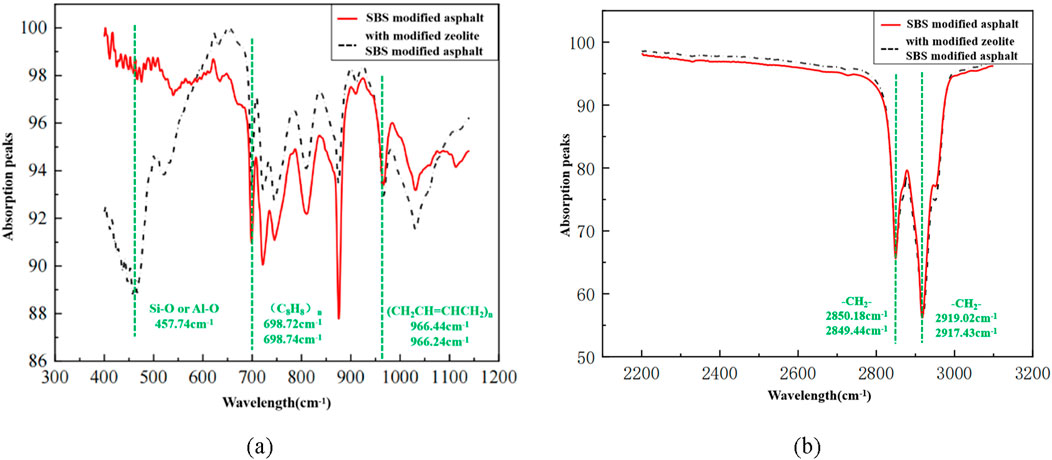
Figure 5. Infrared spectra comparison chart: (A) Wavenumber range of 300–1,200 cm-1; (B) Wavenumber range of 2,200–3,100 cm-1.
3.4 Analysis of specific surface area and porosity of asphalt mixtures with modified zeolite materials
Figures 6–8 show the nitrogen adsorption-desorption isotherms and corresponding pore structure distributions of asphalt mixtures with different contents of zeolite powder at different particle sizes. All samples exhibit typical Type IV adsorption-desorption isotherms, classified as the Brunauer classification (Ates and Akgül, 2016; Younes et al., 2017), named after Brunauer et al. The curve exhibits distinct characteristics: a significant increase in the low-pressure region, capillary condensation in the high-pressure region, and a clear hysteresis loop of non-overlapping adsorption and desorption.
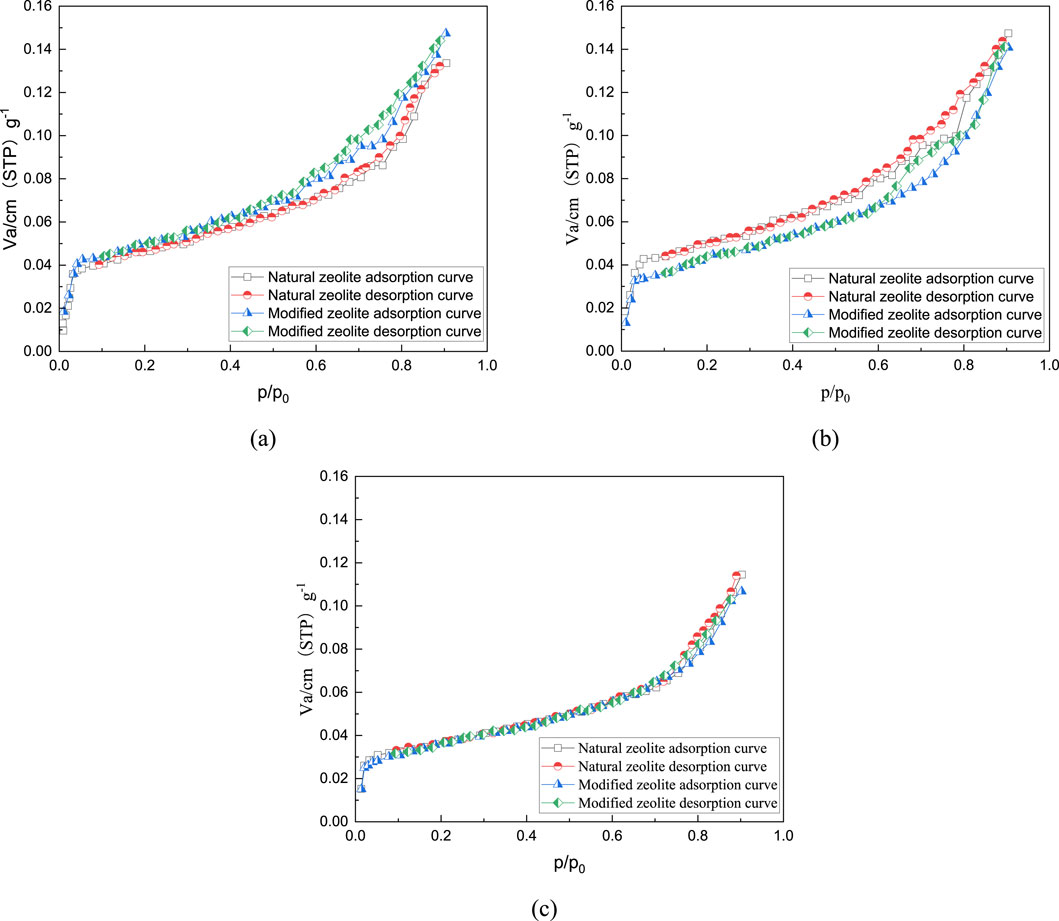
Figure 6. Adsorption-desorption curves for 40% natural zeolite and modified zeolite: (A) 0.075–0.3mm; (B) 0.3–1.18mm; (C) 1.18–2.36 mm.
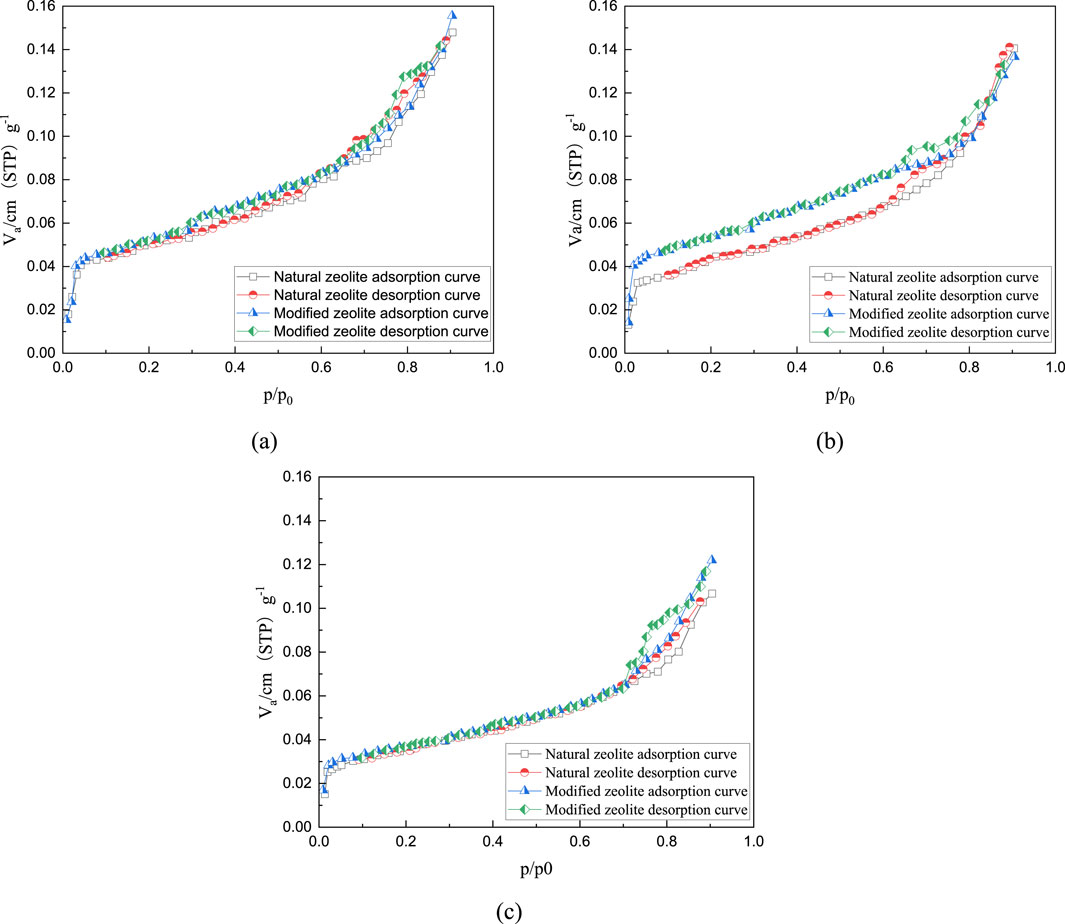
Figure 7. Adsorption-desorption curves for 60% natural zeolite and modified zeolite: (A) 0.075–0.3mm; (B) 0.3–1.18mm; (C) 1.18–2.36 mm.
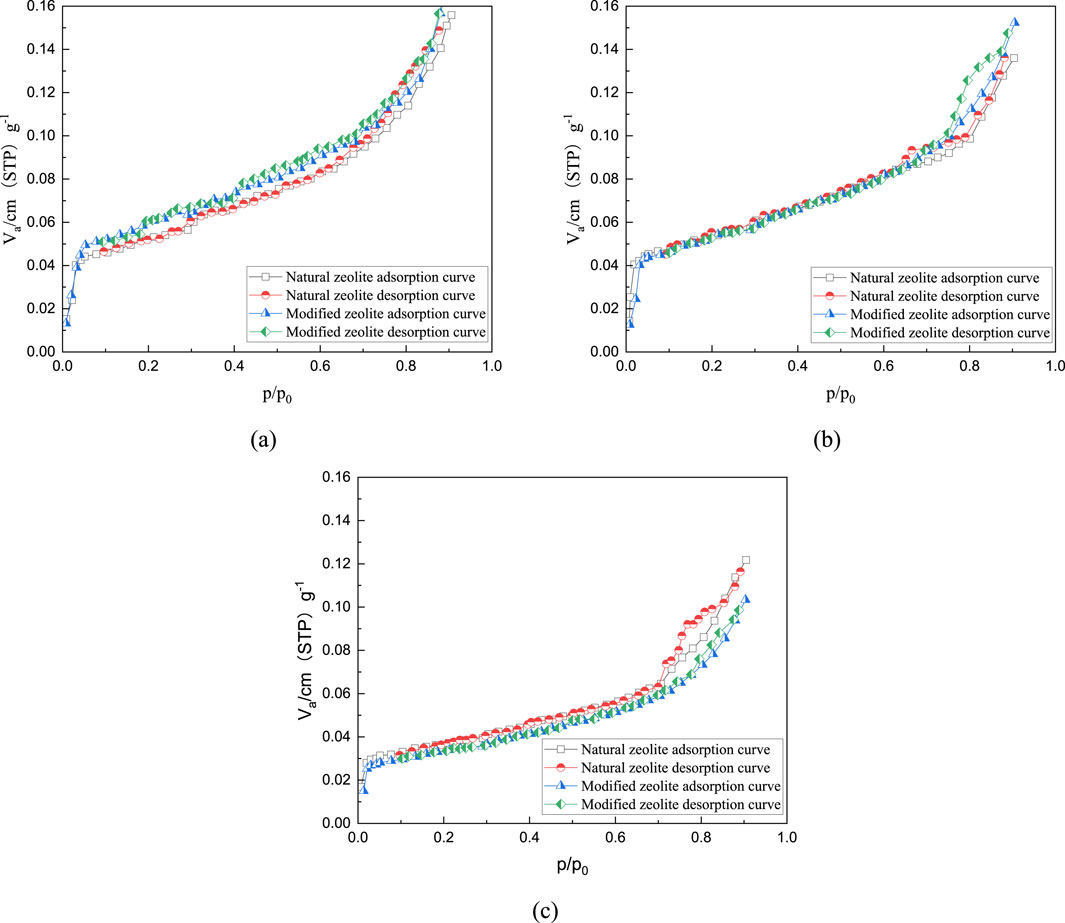
Figure 8. Adsorption-desorption curves for 80% natural zeolite and modified zeolite: (A) 0.075–0.3mm; (B) 0.3–1.18mm; (C) 1.18–2.36 mm.
The graphs demonstrate that N2 adsorption rapidly increases and approaches the saturation adsorption plateau at low relative pressures due to the strong interaction on the adsorbent surface. At this stage, the curve indicates mainly monolayer adsorption with a convex shape. Multilayer adsorption gradually forms as the relative pressure increases. In the intermediate relative pressure range, a distinct hysteresis loop appears, indicating the presence of a large number of micropores (<2 nm) and mesopores (2–50 nm) in both the modified zeolite and natural zeolite (Velarde et al., 2023; Naik et al., 2003). Compared to samples with only mineral powder and natural zeolite powder added, samples with modified zeolite powder show higher nitrogen adsorption capacity. Additionally, as the content of zeolite powder increases, the nitrogen adsorption capacity gradually increases, indicating the formation of more pores after the addition of modified zeolite.
Figures 9–11 and Table 5 demonstrate that the addition of both modified and natural zeolite powders significantly increases the specific surface area and total pore volume of asphalt mixtures. The adsorption pore volume curve of natural zeolite powder shows some degree of interweaving, indicating that the pore size distribution does not uniformly increase with the increase in dosage. At a dosage of 80%, the addition of natural zeolite powder resulted in a 46.25% increase in specific surface area and a 20.49% increase in adsorption pore volume compared to the mixture with only mineral powder. Similarly, the addition of modified zeolite powder at the same dosage resulted in a 62.77% increase in specific surface area and a 65.39% increase in adsorption pore volume. This indicates that adding modified zeolite powder can enhance the porosity characteristics of the asphalt mixture more effectively than adding natural zeolite powder, and the modification with salt solution has optimized the pore structure of the zeolite powder (Ren et al., 2020; Alsaman et al., 2022).
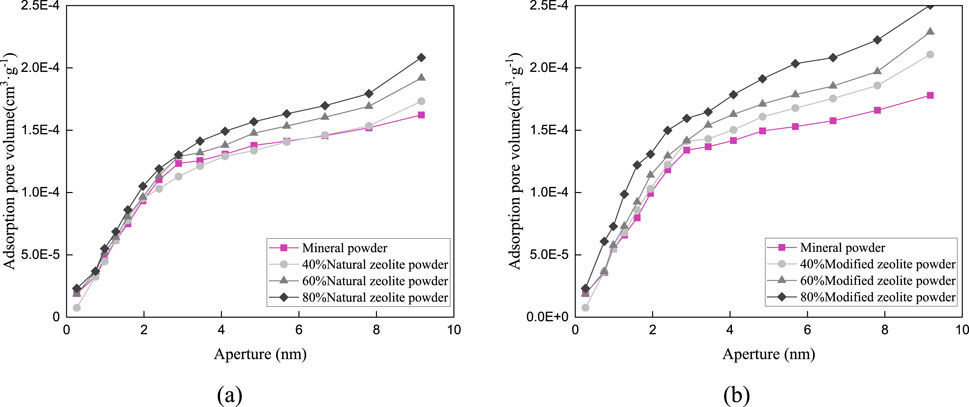
Figure 9. Pore distribution in the particle size range of 0.075–0.3 mm: (A) Pore size distribution of natural zeolite powder; (B) Pore size distribution of modified zeolite powder.
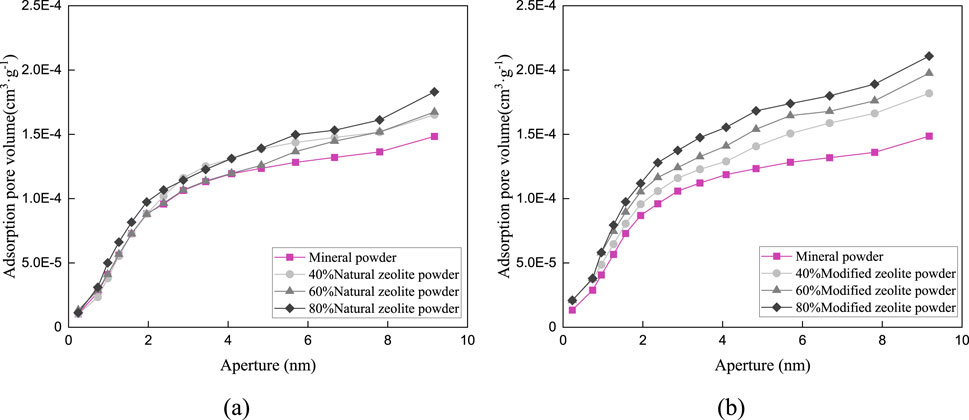
Figure 10. Pore distribution in the particle size range of 0.3–1.18 mm: (A) Pore size distribution of natural zeolite powder; (B) Pore size distribution of modified zeolite powder.
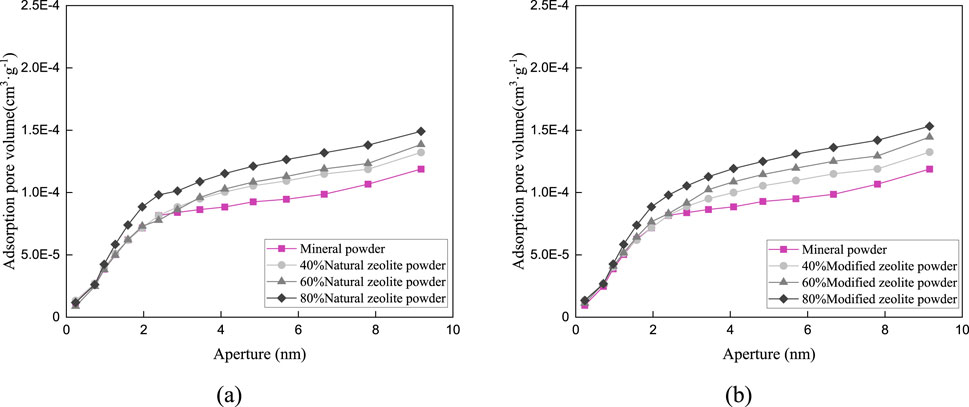
Figure 11. Pore distribution in the particle size range of 1.18–2.36 mm: (A) Pore size distribution of natural zeolite powder (B) Pore size distribution of modified zeolite powder.
3.5 Dust suppression effect test
To simulate the most severe surface dust pollution in Beijing during summer, a wind speed of 3 m/s was used, taking into account the weather conditions. Standard rutting board specimens, made of different replacement rates of natural zeolite powder and modified zeolite powder, were placed in a constant temperature and humidity chamber at 20°C and 60% humidity for 24 h of pre-treatment. Simulating the moisture absorption test on the road surface, rutting board specimens with only mineral powder added were used as the experimental control group to exclude the moisture condensation effect caused by the aggregates (Bhaskar and Sharma, 2008; Ren et al., 2020). The PM10 concentration variation curve is recorded in Figure 12.
From the observations in Figure 12, it can be concluded that:
(1) Adding only mineral powder to the asphalt mixture does not reduce the PM10 concentration in the container. However, the asphalt mixture containing natural or modified zeolite exhibits a significant reduction in PM10 concentration within the first 6 h, with concentrations reaching 200–400 μg/m3.
(2) At the 6-hour mark, the PM10 concentration in the container is ranked as follows: 80% modified zeolite asphalt mixture <60% modified zeolite asphalt mixture <40% modified zeolite asphalt mixture <80% natural zeolite asphalt mixture <60% natural zeolite asphalt mixture <40% natural zeolite asphalt mixture. The dust control ability of asphalt mixtures increases as the replacement rate of zeolite powder increases. Furthermore, the modified zeolite asphalt mixture exhibits a greater capacity for dust adsorption in comparison to the natural zeolite asphalt mixture, with a maximum improvement of 16.34%.
(3) In the presence of zeolite as an adsorbent, the water transfer and adsorption processes in the asphalt mixture occur in the following order: (i) Vapor is attracted to unsaturated surface sites of the adsorbent through van der Waals forces (intermolecular attraction). (ii) Vapor spreads from the outer phase boundary membrane into the pore space. (iii) Vapor transitions from the gas phase to the liquid phase, forming water molecules through internal diffusion within the pore space. Since water molecules are polar, they can interact with the surface of dust particles, achieving a dust suppression effect. In addition, modified zeolite asphalt mixtures have a larger specific surface area and adsorption pore volume. This attracts more water molecules and creates more water film interfaces, resulting in a better dust suppression effect (Aristov et al., 2002; Ng and Mintova, 2008).
4 Conclusion
In this study, natural zeolite was modified using four different salt solutions with different concentrations. Based on the moisture absorption rate and capacity, zeolite powder modified with a 20% CaCl2 solution was selected as the moisture absorption modifier for this study. The microscopic characteristics of the modified zeolite asphalt mixture were studied using techniques such as SEM and FTIR. Nitrogen adsorption experiments were designed to analyze the specific surface area and porosity of the modified zeolite asphalt mixture. Macroscopic dust suppression experiments were conducted to analyze the dust suppression ability of different blending ratios of asphalt mixtures. The conclusions of this study are as follows:
(1) Salt solutions can remove organic impurities and metal cations from natural zeolite. Modified zeolite treated with salt solutions shows significantly improved moisture absorption capacity and rate compared to natural zeolite powder. The zeolite powder modified with a 20wt% CaCl2 solution consistently maintains a moisture absorption capacity that is at least 7 times higher than that of natural zeolite powder, and it can reach up to 10 times higher;
(2) SEM images show that the asphalt phase of the modified zeolite asphalt mortar has a rougher surface morphology. The fillers are uniformly distributed and completely enveloped by the asphalt, indicating good compatibility between the modified zeolite and the asphalt. FTIR spectra show that there is no significant chemical reaction and the formation of new chemical groups between SBS asphalt and the modified zeolite materials during high-temperature mixing;
(3) The nitrogen adsorption results show that the modified zeolite asphalt mixture exhibits typical Type IV adsorption-desorption isotherms with distinct hysteresis loops, indicating the presence of a large number of micropores and a relatively high concentration of mesopores. Compared to natural zeolite in the mixture, the modified zeolite asphalt mixture has a higher specific surface area and adsorption pore volume. This indicates that adding modified zeolite powder can enhance the porosity characteristics of the asphalt mixture more effectively than adding natural zeolite powder, and the modification with salt solution has optimized the pore structure of the zeolite powder.
(4) The dust suppression experiments show that the modified zeolite mixture has a stronger ability to adsorb PM10 compared to the natural zeolite asphalt mixture. In the same time period, the dust suppression ability is improved by a maximum of 16.34%.
Although this research has achieved some initial results, there are still many shortcomings. For example, the retention rules of adsorbed water in asphalt mixtures are not revealed, and there is a lack of experiments to show the cyclic adsorption capacity of asphalt concrete pavements with porous adsorbents added under real conditions. Its quantification in the diffusion model requires further research.
Data availability statement
The original contributions presented in the study are included in the article/supplementary material, further inquiries can be directed to the corresponding author.
Author contributions
SZ: Investigation, Methodology, Writing–original draft, Writing–review and editing. HT: Investigation, Methodology, Writing–original draft, Writing–review and editing. ZZ: Investigation, Methodology, Supervision, Writing–review and editing. HJ: Investigation, Supervision, Writing–review and editing. WX: Formal Analysis, Investigation, Writing–review and editing. XS: Supervision, Validation, Writing–review and editing.
Funding
The author(s) declare that financial support was received for the research, authorship, and/or publication of this article. This study was funded by the National Key Research and Development Program of China (2021YFB2601204 and 2022YFC3803405), the National Natural Science Foundation of China (52078024) and the BUCEA Post Graduate Innovation Project (PG2024043).
Conflict of interest
The authors declare that the research was conducted in the absence of any commercial or financial relationships that could be construed as a potential conflict of interest.
Publisher’s note
All claims expressed in this article are solely those of the authors and do not necessarily represent those of their affiliated organizations, or those of the publisher, the editors and the reviewers. Any product that may be evaluated in this article, or claim that may be made by its manufacturer, is not guaranteed or endorsed by the publisher.
References
Alsaman, A. S., Askalany, A. A., Ibrahim, E. M. M., Farid, A. M., Ali, E. S., and Ahmed, M. S. (2022). Characterization and cost analysis of a modified silica gel-based adsorption desalination application. J. Clean. Prod. 379, 134614. doi:10.1016/j.jclepro.2022.134614
Aristov, Y. I., Restuccia, G., Cacciola, G., and Parmon, V. N. (2002). A family of new working materials for solid sorption air conditioning systems. Appl. Therm. Eng. 22 (2), 191–204. doi:10.1016/s1359-4311(01)00072-2
Ates, A., and Akgül, G. (2016). Modification of natural zeolite with NaOH for removal of manganese in drinking water. Powder Technol. 287, 285–291. doi:10.1016/j.powtec.2015.10.021
Bhaskar, V. S., and Sharma, M. (2008). Assessment of fugitive road dust emissions in Kanpur, India: a note. Transp. Res. Part D Transp. Environ. 13 (6), 400–403. doi:10.1016/j.trd.2008.06.003
Cheng, X. W., Zhong, Y., Wang, J., Guo, J., Huang, Q., and Long, Y. C. (2005). Studies on modification and structural ultra-stabilization of natural STI zeolite. Microporous Mesoporous Mater. 83 (1-3), 233–243. doi:10.1016/j.micromeso.2005.04.016
Dehmani, Y., Mohammed, B. B., Oukhrib, R., Dehbi, A., Lamhasni, T., Brahmi, Y., et al. (2023). Adsorption of various inorganic and organic pollutants by natural and synthetic zeolites: a critical review. Arabian J. Chem., 105474. doi:10.1016/j.arabjc.2023.105474
Deng, N. (2014). Preparation and performance of composite humidity-control materials based on porous minerals. Dissertation. Zhejiang University. Master.
Di, Y. H., Zheng, S. L., Li, C. Q., Sun, Z. M., and Zhang, X. C. (2021). Pore structure regulation and humidity-controlling effect of natural stellerite. Journal of the Chinese Ceramic Society (07), 1385–1394. doi:10.14062/j.issn.0454-5648.20200959
Edvardsson, K., Gustafsson, A., and Magnusson, R. (2012). Dust suppressants efficiency study: in situ measurements of dust generation on gravel roads. Int. J. Pavement Eng. 13 (1), 11–31. doi:10.1080/10298436.2011.561844
GB/T 19587-2017 (2024). Determination of the specific surface area of solids by gas adsorption using the BET method.
GB/T 21650.1-2008 (2006). Pore size distribution and porosity of solid materials by mercury porosimetry and gas adsorption- Part 2: Analysis of mesopores and macropores by gas adsorption.
Ghasemi, M. R., Nazemi, A. H., and Bozorgzadeh, H. R. (2023). Continuous adsorption process of CO2/N2/H2O from CH4 flow using type A zeolite adsorbents in the presence of ultrasonic waves. Results Eng. 20, 101490. doi:10.1016/j.rineng.2023.101490
Gordeeva, L. G., and Aristov, Y. I. (2012). Composites ‘salt inside porous matrix’for adsorption heat transformation: a current state-of-the-art and new trends. Int. J. Low-Carbon Technol. 7 (4), 288–302. doi:10.1093/ijlct/cts050
Hu, J. B. (2021). Study on moisture buffer capacity of building hygroscopic materials and its influencing factors. Dissertation. Xi’an University of Architecture and Technology. Master. doi:10.27393/d.cnki.gxazu.2021.000705
Hu, M. Y., Liu, Z. J., Ke, S. J., Fu, C., and Fan, C. J. (2018). Effect of inorganic modified admixture on performance of the zeolite humidity controlling materials. J. Build. Mater. (05), 791–796. doi:10.3969/j.issn.1007-9629.2018.05.015
Jänchen, J., Ackermann, D., Stach, H., and Brösicke, W. (2004). Studies of the water adsorption on zeolites and modified mesoporous materials for seasonal storage of solar heat. Sol. energy 76 (1-3), 339–344. doi:10.1016/j.solener.2003.07.036
Kuldeyev, E., Seitzhanova, M., Tanirbergenova, S., Tazhu, K., Doszhanov, E., Mansurov, Z., et al. (2023). Modifying natural zeolites to improve heavy metal adsorption. Water 15 (12), 2215. doi:10.3390/w15122215
Lei, P. P. (2014). Preparation and properties of zeolite-based humidity-controlling material. Dissertation. South China University of Technology. Master.
Makarov, D. V., Konina, O. T., and Goryachev, A. A. (2021). Dusting suppression at tailings storage facilities. J. Min. Sci. 57, 681–688. doi:10.1134/s1062739121040165
Martinez-Soto, A., Calabi-Floody, A., Valdes-Vidal, G., Hucke, A., and Martinez-Toledo, C. (2023). Life cycle assessment of natural zeolite-based warm mix asphalt and reclaimed asphalt pavement. Sustainability 15 (2), 1003. doi:10.3390/su15021003
Ministry of Transport of the People’s Republic of China (2004). JTG F40-2004; technical specifications for construction of Highway asphalt pavements. Beijing, China: China Communications Press.
Ministry of Transport of the People’s Republic of China (2011). JTG E20-2011; standard test methods of bitumen and bituminous mixtures for Highway engineering. Beijing, China: China Communications Press.
Mohammad, S., Babak, A., Fazel, A., Shafei, B., and Kioumarsi, M. (2023). Natural zeolite as a supplementary cementitious material – a holistic review of main properties and applications. Constr. Build. Mater. 409, 133766. doi:10.1016/j.conbuildmat.2023.133766
Naik, S. P., Chiang, A. S., and Thompson, R. W. (2003). Synthesis of zeolitic mesoporous materials by dry gel conversion under controlled humidity. J. Phys. Chem. B 107 (29), 7006–7014. doi:10.1021/jp034425u
Ng, E. P., and Mintova, S. (2008). Nanoporous materials with enhanced hydrophilicity and high water sorption capacity. Microporous Mesoporous Mater. 114 (1-3), 1–26. doi:10.1016/j.micromeso.2007.12.022
Qi, K. L. (2023). Research on the effect of modified zeolite based materials on the performance of dust suppressing asphalt mixture. Dissertation. Beijing University of Civil Engineering and Architecture. Master. doi:10.26943/d.cnki.gbjzc.2023.000367
Rafique, M. M., Gandhidasan, P., and Bahaidarah, H. M. (2016). Liquid desiccant materials and dehumidifiers–A review. Renew. Sustain. Energy Rev. 56, 179–195. doi:10.1016/j.rser.2015.11.061
Ren, W., Shi, J., Zhu, J., and Guo, Q. (2020). An innovative dust suppression device used in underground tunneling. Tunn. Undergr. Space Technol. 99, 103337. doi:10.1016/j.tust.2020.103337
Shekarchi, M., Ahmadi, B., Azarhomayun, F., Shafei, B., and Kioumarsi, M. (2023). Natural zeolite as a supplementary cementitious material–A holistic review of main properties and applications. Constr. Build. Mater. 409, 133766. doi:10.1016/j.conbuildmat.2023.133766
Song, C., Zhao, Y., Cheng, W., Hu, X., Zhu, S., Wu, M., et al. (2021). Preparation of microbial dust suppressant and its application in coal dust suppression. Adv. Powder Technol. 32 (12), 4509–4521. doi:10.1016/j.apt.2021.10.001
Usmani, R. S. A., Saeed, A., Abdullahi, A. M., Pillai, T. R., Jhanjhi, N. Z., and Hashem, I. A. T. (2020). Air pollution and its health impacts in Malaysia: a review. Air Qual. Atmos. and Health 13, 1093–1118. doi:10.1007/s11869-020-00867-x
Velarde, L., Nabavi, M. S., Escalera, E., Antti, M. L., and Akhtar, F. (2023). Adsorption of heavy metals on natural zeolites: a review. Chemosphere 328, 138508. doi:10.1016/j.chemosphere.2023.138508
Wang, X. M., Wang, W. J., Li, J., Wang, Y. E., and Li, Y. (2014). Urban dust pollution and its research of control method in China. Environ. Sci. and Technol. (S2), 588–592. doi:10.3969/i.issn.1003-6504.2014.120.121
Wang, Z. Y. (2019). Effect of indoor porous hygroscopie material on environmental humidity and energy consumption-taking wool insulation material as an example. Dissertation. Nanjing University. Master. doi:10.27235/d.cnki.gnjiu.2019.000809
Wingenfelder, U., Nowack, B., Furrer, G., and Schulin, R. (2005). Adsorption of Pb and Cd by amine-modified zeolite. Water Res. 39 (14), 3287–3297. doi:10.1016/j.watres.2005.05.017
Xie, H. H. (2018). Investigation on thermodynamic performance of composite hygroscopic material and the influence on indoor thermal and humidity environment. Dissertation. Hunan University. Ph.D. Thesis.
Younes, M. M., El-Sharkawy, I. I., Kabeel, A. E., and Saha, B. B. (2017). A review on adsorbent-adsorbate pairs for cooling applications. Appl. Therm. Eng. 114, 394–414. doi:10.1016/j.applthermaleng.2016.11.138
Zheng, J. Y. (2015). Study on heat and moisture transfer process of diatomite-based humidity control material and its application in buildings. Dissertation. Southeast University. Ph.D. Thesis.
Keywords: dust suppression pavement, natural zeolite, modified zeolite, nitrogen adsorption, asphalt mixture
Citation: Zhi S, Tao H, Zihao Z, Jiaheng H, Xinxin W and Shijie X (2024) Study on the humidification mechanism of asphalt mixtures by modified zeolite. Front. Mater. 11:1461129. doi: 10.3389/fmats.2024.1461129
Received: 07 July 2024; Accepted: 04 October 2024;
Published: 24 October 2024.
Edited by:
Jinyang Xu, Shanghai Jiao Tong University, ChinaReviewed by:
Yiqun Guo, South China University of Technology, ChinaKatarzyna Zarebska, AGH University of Science and Technology, Poland
Copyright © 2024 Zhi, Tao, Zihao, Jiaheng, Xinxin and Shijie. This is an open-access article distributed under the terms of the Creative Commons Attribution License (CC BY). The use, distribution or reproduction in other forums is permitted, provided the original author(s) and the copyright owner(s) are credited and that the original publication in this journal is cited, in accordance with accepted academic practice. No use, distribution or reproduction is permitted which does not comply with these terms.
*Correspondence: Suo Zhi, c3VvemhpQGJ1Y2VhLmVkdS5jbg==