- 1Linxia Highway Development Center of Gansu Province, Linxia, China
- 2Kanglin Expressway Toll Station of Gansu Province, Linxia, China
- 3Gansu Province Transportation Planning Survey & Design Institute Co., Ltd., Lanzhou, China
- 4School of Civil Engineering, Hebei University of Engineering, Handan, Hebei, China
The arch expansion damage of asphalt pavement is a typical disease in desert Gobi and saline-alkali areas, and the reasons for arch expansion are very complex. Exploring the impact of salt solution on the mechanical and drying shrinkage performances of cement-stabilized macadam helps to clarify the causes of the arch expansion damage. To this purpose, this paper designed a salt solution infiltration experiment, using salt solution infiltration to simulate the transmission and accumulation of salts in cement-stabilized macadam, and carried out the compressive and flexural tests of cement-stabilized mortar and cement-stabilized macadam, and measured the drying shrinkage performance of cement-stabilized mortar and macadam. The results show that the type of salt solution has a significant influence on the weight of the cement-stabilized mortar samples, sulfates will cause the samples to lose weight, while chlorides and mixed solutions cause the increase in weight. Chlorides and sulfates lead to the decrease in the strengths of cement-stabilized mortar and macadam. The salt crystallization will lead to the decline of the drying shrinkage strains of cement-stabilized mortar and macadam, which has a positive action for reducing the drying shrinkage deformation. However, under the combined action of chlorides and sulfates, cement-stabilized macadam expands with the moisture loss. This may be one of the important causes of the arch expansion of asphalt pavement in the Gobi area and saline-alkali area.
1 Introduction
The subbase layer is an important structure of asphalt pavement, which directly affects the stability, service life, and operational efficiency of the asphalt pavement. The subbase using cement-stabilized macadam has the advantages of high strength, large rigidity, strong integrity, and good stability and durability, therefore, it is a commonly used subbase form for asphalt pavement in many countries. However, salt erosion inside of cement or lime-modified materials can lead to the formation of expansive crystals, resulting in the arching up phenomenon of the road surface. After a period of construction, roads such as the U.S. Route 82, US 67, I-635 (Chen et al., 2005), the main road in Las Vegas (Mitchell, 1986), and the Bush Road in Georgia (Rollings et al., 1999) have all experienced arching up phenomenon, mainly due to the formation of ettringite crystals by sulfate erosion of cement-stabilized materials. Similarly, many roads in Inner Mongolia and Xinjiang, China (Song et al., 2019; Wang et al., 2020) have also exhibited similar diseases about arching up phenomenon. Arching up of the road surface gradually appears 1–2 years after construction, with the highest arching height reaching up to 10 cm. The most severe arching occurred in summer, the spacing of the most severe section is less than 50 m. The arching up phenomenon of the asphalt pavement is generally characterized by the overall uplift of the subbase or the appearance of bumps, which in turn causes the road surface to arch up. The severe bumps on the road surface greatly restricts the road travelling capacity and poses a threat to driving safety (Tang et al., 2020; Tang, 2022). Therefore, it is necessary to carry out research on the damage mechanism of arching up of cement-stabilized macadam.
In order to reveal the mechanism of arching up of asphalt pavement, many scholars have conducted many meaningful works. Huang et al. (2017) found that the pavement surface of the Lina section of the Beijing-Xinjiang Expressway in China appeared to arch up, and the arching up phenomenon occurred in the third year after the construction of the subbase. The longer the continuous construction length of the cement-stabilized subbase, the more the arching ups. Zhang et al. (Zhang, 2018) conducted a disease investigation on the roads in Inner Mongolia, China and found that the degree of pavement surface uplift varied, with a spacing of 10–30 m, and the direction of the uplift was mainly transverse. Wang et al. (Hong, 2019) and Xie et al. (2019) investigated the road sections of the Beijing-Xinjiang Expressway and S312, found that the arching up phenomenon mostly happens in summer. The maximum height of arching part on the Beijing-Xinjiang Expressway was about 20cm, and the maximum height of the arching section in S312 was about 10 cm. The higher the cement content, the more severe the subbase arching up, and the degree of salinization in the subbase at the arching site was high. Yao et al. (2021) pointed out that the arching up of asphalt pavement with cement-stabilized subbase in the desert and Gobi areas of Inner Mongolia were mainly caused by high-temperature expansion and uplift deformation. Temperature has a significant impact on the uplift and cracking of asphalt pavement with cement-stabilized macadam. Under the same thermal condition, the subbase is more prone to expansive than the surface layer. Yao et al. (2020) also designed a test to determine the coefficient of expansion of the cement-stabilized macadam, pointing out that the gradation, cement dosage and curing age have significant impacts on the coefficient of expansion, while the sulfate content has almost no effect on the expansion of cement-stabilized macadam. When the temperature rises from 10°C to 50°C, the coefficient of expansion reaches its maximum. Dai et al. (2021) pointed out that the arching disease of asphalt pavement has spatial regional attributes and is periodic in occurrence. The unconfined compressive strength of the subbase of the section without arching is greater than that of the arching section, and the content of sulfate in the arching section is higher than that of the non-arching section. The crystallization of sulfates has caused damage to the microstructure of the subbase at the arching up site. The combined action of heat and salt is the main cause of the arching up of the asphalt pavement with cement-stabilized subbase in the Gobi areas, and the pavement surface arching is caused by the combined action of temperature and salt expansion caused by sulfate.
Based on the above analysis, it can be seen that the arching of asphalt pavement mostly occurs in desert and Gobi areas, and the probability of arching in saline land areas is greater. The arching of the pavement layer mainly occurs in summer, and gradually stops after entering autumn. Besides, the material strength at the arching site of the road surface will be damaged. Teng et al. (Jidong et al., 2016) pointed out that in areas with very little rainfall and large evaporation, due to the large temperature difference between day and night, the migration of water vapor leads to a significant ‘lid effect’ at the bottom of the surface layer, that is, moisture will accumulate under the asphalt surface layer, which will lead to the continuous accumulation of salts in the subbase and ultimately cause the arching and destruction of the asphalt pavement. The crystallization and precipitation of salts directly related to the evaporation of water. Herein, exploring the impact of salts on the strength and shrinkage performance of cement-stabilized macadam can clarify the cause of the arching up.
In summary, this work determined the optimal moisture content of cement-stabilized mortar and macadam mixture through compaction tests, designed indoor salt erosion orthogonal experiments, measured the mechanical strength of cement-stabilized mortar and macadam mixture after salt erosion, and conducted drying shrinkage experiments, analyzed the drying shrinkage state of cement-stabilized macadam under different salt erosion conditions. The work in this paper confirms the adverse effect of salt erosion on the drying shrinkage performance of cement-stabilized macadam and has good reference significance for improving the design of cement-stabilized macadam in the Gobi area.
2 Materials and experimental
2.1 Raw materials
This work conducts the tests using P•O42.5 Portland cement. The properties of the cement are shown in Table 1. The aggregate used in this paper is limestone, and the performances of the macadam are listed in Table 2. The gradations of cement-stabilized mortar and cement-stabilized macadam mixture are shown in Figure 1.
This experiment uses analytically reagent produced by Tianjin Zhiyuan Chemical Reagent Co., Ltd. to simulate the salt environment in saline soil. The properties of sodium sulfate and sodium chloride are shown in Table 3.
2.2 Experimental
2.2.1 Salt erosion scheme
Under the influence of salt erosion, the crystallization not only alters the internal pore structure of the cement-stabilized macadam but also causes local expansion damage, thereby inducing changes in the strength of the cement-stabilized macadam. To clarify the changes in mechanical properties and volume stability of cement-stabilized macadam under the influence of salt, a salt solution migration and infiltration method is used to simulate the erosion effect. Since the migration process of salts in saline soil is complex and the salt solution immersion cannot simulate the salt migration phenomenon at road sites, this work uses the infiltration method to simulate the migration effect of salts with water, using a sponge containing salt solution to simulate the underlying road subgrade. The same wet sponge is placed above the specimen to cover it, and the infiltration treatment process of the specimen is shown in Figure 2. Each infiltration lasts for 12 h, followed by the drying at room temperature about 23°C for 12 h, completing one cycle every 24 h. After the infiltration treatment, mechanical property and volume stability tests are conducted.
This paper uses the orthogonal test to explore the effects of salt type, solution concentration, and erosion cycles on the mechanical properties and drying shrinkage of cement-stabilized macadam. According to existing results (Wang, 2023), the concentration of salt solution is generally between 3% and 10%, and this work adopts salt solution concentrations of 3wt%, 5wt%, and 10wt%. The types of salt solutions include sulfates, chlorides, and the mixed solution of sulfates and chlorides. The upper limit of erosion cycles is set to 5. Based on the design principle of L9(3³), the orthogonal groups can be determined, as shown in Table 4.
Based on the condition of the lowest mechanical strength of cement-stabilized mortar in the orthogonal experiment as the most unfavorable condition, in addition, most areas of saline-alkali land contain sulfates and chlorides, it is also necessary to investigate the impact of mixed salts on the stability of cement-stabilized macadam. Therefore, this work carries out salt erosion treatment for cement-stabilized macadam under the following conditions, as shown in Table 5.
2.2.2 Ion titration method
To evaluate the distribution of salts in the specimen after the migration of the salt solution, the silver nitrate titration method is used to determine the content of NaCl, and the ethylene diamine tetraacetic acid (EDTA) complexometric titration method is used to determine the concentration of Na2SO4, in accordance with the Chinese specification JTG 3430-2020 (Ministry of Transport’s Research Institute of Highway RIH, 2021). Samples are taken from different parts of the specimen, as shown in Figure 3. The preparing process of test solution is shown in Figure 4.
The silver nitrate titration method is used to determine the chloride ion content of the test solution. Methyl orange is used as an indicator, and 0.02 mol/L NaHCO3 is added until the solution turns pure yellow, ensuring a pH value of 7. Six drops of K2CrO4 with the 5% molar concentration are added as chemical indicator, and the silver nitrate standard solution is titrated until brick-red precipitate forms. The consumed volume of AgNO3 solution is recorded. The entire titration process is shown in Figure 5. The chloride ion content is calculated using the Equations 1, 2.
Where, c represents the concentration of the silver nitrate solution, mol/L; v represents the volume of the titrated solution, mL; m is the dry weight of the mixture taken from the volume of the leachate, g.
The EDTA indirect complexometric titration method is used to determine the sulfate ion content of the test solution. The specific procedure can refer to the standard JTG 3430-2020. After the titration, the content of sulfate ions is calculated according to Equations 3, 4.
Where, c is the concentration of the EDTA standard solution, mol/L;m represents the weight of dry mixture corresponding to the sodium sulfate content in the leachate, g. V1 represents the volume of EDTA solution consumed when the standard solution changes from a wine-red to a pure blue color during titration, ml; V2 represents the volume of EDTA solution consumed when the blank control group solution changes from a wine-red to a pure blue color during titration, ml; V3 represents the volume of EDTA solution consumed during titration of the test solution, ml.
2.2.3 Mechanical performances test
To evaluate the effect of salt erosion on the strength of cement-stabilized mortar and cement-stabilized macadam mixture, compressive and flexural tests were conducted. According to the Chinese standard GB/T 17,671-2021 (Standardization Administration of ChinaSAC, 2021), cement-stabilized mortar specimens with a dimension 40 mm × 40 mm × 160 mm are molded using a static compression method and cured in an environment of 20°C and 95% relative humidity. Tests are performed at the age days. The loading rate for the flexural test of cement-stabilized mortar is 50N/s, and each group of experiments is conducted with three replicates. Compressive tests are performed using the failure samples from the flexural test, with a loading rate of 2.4 kN/s for the compressive test. It should be noted that for the 7-day test, the specimens should be soaked in water on the last day of curing. The flexural and compressive strengths are determined using Equation 5.
Where, Rf is the flexural strength, MPa;Ff represents the failure load. N; L represents the support span of the beam specimen, mm; b represents the width of the specimen’s cross section, mm.
Where, Rc is the compressive strength, MPa;Fc represents the failure load, N; A is the area bearing the compressive load, mm2.
In addition, to evaluate the impact of salt erosion on the strength of cement-stabilized macadam, cylindrical specimens with dimensions of Φ150 mm × 150 mm were prepared using the compaction method according to JTG 3430-2020. After curing, the specimens were subjected to salt erosion treatment and their compressive strength was tested. The loading rate was 1 mm/min, and the compressive strength was calculated according to Equation 6.
2.2.4 Drying shrinkage test
The migrating of salt solution into cement-stabilized macadam not only affects its strength, but also, after the evaporation of water, salt crystallization occurs, generating crystallization pressure that causes volume expansion of the cement-stabilized macadam. In contrast, cement-stabilized macadam without salt will undergo drying shrinkage as water evaporating. For the mixture containing salt solution, the expansion effect caused by salt crystallization and the drying shrinkage are coupled. Therefore, this paper carries out drying shrinkage test on cement-stabilized mortar and cement-stabilized macadam to clarify the expansion of cement-stabilized macadam mixtures affected by salt erosion.
The specimens of cement-stabilized mortar with dimension of 40 mm × 40 mm × 160 mm were prepared in lab, and the specimens of cement-stabilized macadam with dimension of 100 mm × 100 mm × 400 mm also were prepared. After the compaction, they are cured in an environment with a temperature of 20°C ± 1°C and a relative humidity above 95% for 7 days, and then placed in an environment with a temperature of 20°C ± 2°C and a relative humidity of 50% ± 5% for shrinkage tests. Due to the rough surface of the beam specimen, if the dial indicator is directly in contact with the specimen surface, the dial indicator is very susceptible to disturbance. Therefore, organic glass plates are glued to both ends of the specimens to ensure good contact between the dial indicator and the glass plates.
The moisture loss and drying shrinkage coefficient can be determined using Equations 7–10.
Where, mi, mi+1 represent the specimen weight at the ith and (i+1)th weighing, respectively. mp refers to the weight of the specimen after drying. δi is the drying shrinkage deformation at the ith measurement, mm. Xi,j and Xi+1,j represent the dial reading value of the jth dial indicator at the ith and (i+1)th measurement, respectively, mm°εi is the shrinkage strain at the ith measurement. l represents the length of the specimen, mm;αdi represents the shrinkage coefficient of the ith test; %; ωi represents the moisture loss of the specimen at the ith measurement, %.
3 Result and discussions
3.1 Determination of the optimal moisture content
According to the specifications (Ministry of Transport’sResearch Institute of Highway RIH, 2021) and experience, the amount of cement used in this paper is 5%. Due to the abundance of fine aggregates in the cement-stabilized mortar, the moisture content of 6%–10% is selected for the compaction test; whereas the coarse aggregates in cement-stabilized macadam have a low specific surface area, hence the moisture content of 3%–7% is chosen for the compaction test. The diameter of the sample is 152 mm, and each layer of the mixture is compacted 98 times. The dry densities of the cement-stabilized mortar and the cement-stabilized macadam were measured, as shown in Figure 6.
From Figure 7, it can be seen that the dry density first increases and then decreases with the increase of moisture content. Based on the principle that the higher the dry density, the greater the load-bearing capacity of the mixture, the moisture content and density corresponding to the peak point are the optimal content and maximum dry density. Therefore, it can be determined that the optimal moisture content for cement-stabilized mortar is 7%, and the maximum dry density is 1.95 g/m³. The optimal moisture content for cement-stabilized macadam is 5%, and the maximum dry density is 2.504 g/cm³. Cement-stabilized mortar and cement-stabilized macadam mixtures are prepared according to the optimal moisture content. Flexural and compressive strengths are tested to determine whether they meet the strength requirements for the use in road engineering. The results are listed in Table 6. The data in the table show that the unconfined compressive strength of cement-stabilized macadam prepared with 5% cement after 7 days exceeds 5 MPa, meeting the strength requirements of the Chinese specification JTG D50-2017 (Ministry of Transport’s Research Institute of Highway RIH, 2017), meaning that the amount of cement selected in this paper is feasible.
3.2 Weight changing and strength of cement-stabilized mortar
During the salt solution infiltration process, due to the capillary action, cement-stabilized mortar and cement-stabilized macadam will absorb the salt solution as the time extends, thereby causing changes in the weight of the mixture. The results of the weight changing after salt solution treatment are listed in Table 7.
As shown in Table 7, the weight changing of cement-stabilized mortar to different solutions is not consistent. It should be noted that specimens containing sulfate solution experience a reduction in weight, while those infiltrated in chloride and mixed solutions both result in an increase in weight. The decrease of the specimen weight primarily due to the erosion of the specimen surface by the sulfate solution, which leads to the detachment of some materials. The detachment does not occur in the case of chloride and mixed solutions. Comparing the six groups where the weight changing is greater than zero, the highest moisture absorption is for the group #3, reaching 2.71%, while the lowest is for group #8, at only 0.68%, the maximum absorption is approximately four times that of the minimum. Thus, the concentration of the solution, the solution type, and the infiltration cycles can lead to significant differences in the weight changing. This further leads to variations of salt concentration inside the mixture, which in turn affects the mechanical properties of the cement-stabilized mixture.
Salt solutions can cause physico-chemical erosion effects on cement-stabilized mixture, leading to a reduction in strength. Therefore, this paper measured the compressive and flexural strengths of cement-stabilized mortar, the results is listed in Table 8. It should be clarified that after curing the specimens for 7 days, they are subjected to infiltration, and their strength is measured after the soaking treatment.
As listed in Table 8, it can be seen that salt solution erosion has a significant impact on the strength of cement-stabilized mortar, with compressive strength ranging from 2.29 to 9.0 MPa, and flexural strength ranges from 1.03 to 4.57 MPa. The maximum compressive strength is 3.9 times the minimum, while the difference in flexural strength is more pronounced, with the maximum flexural strength being 4.4 times the minimum. This indicates that the impact of salt solution erosion on flexural strength is significant, suggesting that after salt solution migration into cement-stabilized macadam, the flexural strength decays more rapidly, leading to premature cracking in the cement-stabilized subbase layer. Compared with the untreated cement-stabilized mortar, for groups #1 and #6, the compressive strength has already exceeded the 28-day compressive strength of the control group, indicating that the salt erosion has a positive role in the compressive strength of cement-stabilized mortar. Both groups of mixtures also have higher flexural strength than the 28-day flexural strength of the control group. It is found that all these groups contain sodium sulfate solution, and the solution concentration does not exceed 5%. Therefore, it is inferred that when the concentration of sulfate is low, it has a certain strengthening effect on cement-stabilized mortar, which can increase the compressive strength by up to 1.16 times. Zhang et al. (Zhang et al., 2022) indicated that the porosity of cement-stabilized macadam declined with the extension of drying period. It means that the few crystal particles just fill the micro-pores inside the mortar, making the mixture more compact, which is manifested as an increase in compressive strength. For groups #2-#5 and #7-#9, the compressive strengths all decrease after salt erosion. On the one hand, the corrosive effect of chloride salts affects the cement hydration reaction, leading to reduced hydration. On the other hand, the higher concentration of salt solution results in more salt crystal particles precipitating, and the massive crystal precipitation generates expansion and pressure, causing micro-cracks and damage inside the mortar, ultimately leading to a decrease in the compressive strength. The specific mechanism of strength decay is shown in Figure 7.
It can be found that the weight changes in the infiltration process, the compressive and flexural strengths also influenced by salt solution. But the significance what affects these properties is unknown. For this purpose, statistical analysis methods were used to evaluate the significance of solution concentration, solution type and infiltration cycles. The significance level (α) employed in this investigation was 0.05. The F-test, Levene’s test and Welch’s test were performed according to a confidence level of 95%. It should be noted that before conducting variance analysis, Levene’s test is first used to determine whether there is a significant difference in variance between the test data. If the p-value of Levene’s Test is less than 0.05, the variances among different data groups are significantly different, and in this case, Welch’s test should be conducted. Conversely, when the p-value of Levene’s Test is greater than 0.05, it is believed that there is no significant difference in variance, and an F-test can be performed. If the p-value of the F-test is less than 0.05, it is considered that the source of variance has a significant impact on the test indicator. The analysis results were listed in Table 9.
The statistical results from Table 9 indicate that the effect of salt solution concentration on the weight of the samples follows the F-test, while the effects of solution type and infiltration cycles on flexural strength also conform to the F-test. The influence of solution concentration, solution type, and infiltration cycles on compressive strength all follow the Welch test. Based on the p-value of the hypothesis test, the solution type has a significant impact on the change in the weight of the samples, and the solution concentration, solution type, and infiltration cycles all have significant impacts on the compressive and flexural strengths of cement-stabilized mortar.
3.3 Weight changing and strength of cement-stabilized macadam
The weight changing of the cement-stabilized macadam is shown in Figure 8.
As described in Figure 8, the weight changing of the cement-stabilized macadam mixture varies with the type of salt solution. Since the weight change is greater than zero, it indicates that the cement-stabilized macadam has absorbed moisture during the infiltration treatment process. In conjunction with the groups in Table 5, it can be seen that the mixture absorbs the most water when subjected to infiltration in a mixed solution. The moisture absorption of specimens infiltrated with a sodium chloride solution is higher than that of pure water. Since the internal pore structure of the cement-stabilized macadam mixture is essentially consistent under the standard compaction, the volume of water absorbed by the mixture also is consistent; the higher the concentration of the solution, the greater the specific gravity of the solution, thus leading to an apparent increase in the moisture absorption. Comparing the weight changing of cement-stabilized macadam and mortar reveals that the moisture absorption of the cement-stabilized macadam is lower than that of the cement-stabilized mortar. This is because the cement-stabilized mortar is composed of fine particles with a larger specific surface area and stronger capillary absorption within, resulting in a higher moisture absorption. This also indirectly proves that using a coarse gradation can reduce the moisture absorption of the cement-stabilized macadam, thereby decreasing the probability of drying shrinkage and cracking caused by moisture fluctuation in the subbase layer. The compressive strength of cement-stabilized macadam mixture after solution treatment is listed in Figure 9.
From Figure 9, it can be observed that the compressive strength of group #1(control) is the highest, reaching 5.1MPa, followed by group #2, with group #3 being the lowest. Compared with the samples that have not undergone salt solution treatment, the sodium chloride solution treatment (#2) resulted in a 19.6% reduction in the compressive strength, while the mixed solution caused a 29.4% decrease in the strength of the cement-stabilized macadam. So, the mixed solution has the most significant impact on the compressive strength of the cement-stabilized macadam. The reduction in compressive strength is mainly due to the dissolution of some calcium aluminate hydrates in the cement by the chloride salts, and the crystallization pressure induced by the crystallization of salts leading to the formation of micro-cracks. The salt crystallization phenomenon is evident on the surface of the samples.
3.4 Effect of salt erosion on drying shrinkage performance
From the analysis in Section 3.2, 3.3, it can be seen that the salt solutions will produce a corrosive effect, and the crystallization of salts will generate expansive pressure, both of which lead to a decrease in the strength of cement-stabilized mortar and cement-stabilized macadam. For cement-stabilized materials, shrinkage occurs with the moisture loss, and salt-containing cement-stabilized macadam will simultaneously undergo shrinkage and crystallization expansion. To clarify the impact of salt erosion on the drying shrinkage performance of cement-stabilized mortar and cement-stabilized macadam, the shrinkage performance of cement-stabilized mortar and cement-stabilized macadam was measured, and the test results are shown in Figure 10.
As described in Figure 10, it can be observed that compared to the control group, the shrinkage strains at 168 h of groups #1 to #9 have all decreased, indicating that the salt solution treatment reduced the degree of drying shrinkage of the cement-stabilized mortar. During the dehydration and shrinkage process, the crystallization of salts generated expansive pressure, causing the cement-stabilized mortar to expand. However, the expansion effect did not change the fundamental characteristic of the mortar undergoing shrinkage. By comparing the shrinkage strain of groups #1 to #9, it can be seen that the shrinkage strain of groups #7 to #9 is significantly smaller than that of the control group, about one-third of the strain of the control group. These three groups had a salt solution concentration of 10%, thus it can be seen that the higher the concentration of the salt solution, the stronger the crystallization expansion, leading to a significant reduction in drying shrinkage strain. If the minimum shrinkage strain is taken as the criterion, then salt erosion has a positive effect on reducing the shrinkage degree.
Considering the actual service state of subbase layer, salts gradually migrate day by day, and multiple salt erosions lead to a decline in the performance of cement-stabilized mortar, which involves complex physical and chemical processes. During the erosion processes, phenomena such as salt accumulation, crystal formation, dissolution of cement-based materials, and internal structure damage occur, which are the main reason for strength degradation. In this paper, when evaluating the shrinkage performance of cement-stabilized macadam, Infiltration cycles were set to 5. The drying shrinkage strain and moisture loss of cement-stabilized macadam are shown in Figure 11.
As shown in Figure 11, the drying shrinkage strain of the control group (#1) gradually increases with the extension of the drying time and approaches stable after reaching 60 days, with no further increase in shrinkage strain. This is consistent with the test results of Tran et al. (2021). Throughout the whole test process, the cement-stabilized macadam of the control group (#1) continues to shrinkage. The mixture treated by the solution of sodium chloride undergo shrinkage deformation in the early stage (not exceeding 20 days), and as the drying time extends, the shrinkage strain begins to gradually decrease, indicating that the mixture has generate expansion deformation, which is caused by the crystallization of salts due to the moisture loss. After the macadam was treated by the mixed salt solution (#3), the cement-stabilized macadam undergoes expansion strain, and this expansion strain gradually increases with the extension of the drying time. The expansion strain gradually stabilizes, reaching 147 × 10−6 after 60 days. Comparing the three groups, it can be determined that the effect of chloride components on the shrinkage of cement-stabilized macadam has a lag, and although expansion strain has occurred, the mixture still shows a general shrinkage characteristic. The coupled action of sulfates and chlorides will lead to a large expansion strain in the cement-stabilized macadam mixture, which is not conducive to maintaining the stability of the cement-stabilized subbase layer. When the cumulative expansion strain exceeds the limit of material, the subbase layer will arch up. Therefore, it is reasonable to infer that in saline areas, the arching up and bulging damage of asphalt pavement is closely related to the combined action of sulfates and chlorides.
From Figure 11, it can also be seen that the moisture losses of the specimens for three groups follow the same trend, with the maximum moisture loss at 90 days being about 3.6%. Thus, the moisture loss of the cement-stabilized macadam will not undergo significant changes under the same environmental condition. However, the salts that entering the mixture will gradually crystallize and precipitate as the increase of moisture loss, and different types of salts have significant impact on the drying shrinkage of the cement-stabilized macadam. To determine the shrinkage coefficient of the cement-stabilized macadam, a linear fitting was performed on the shrinkage strain, and the process is shown in Figure 12. Results are listed in Table 10.
From Figure 12, it can be observed that for the control group (#1), the shrinkage strain gradually increases with the increase of moisture loss, and it continues to show a trend of shrinkage throughout the test period. However, for group #2 treated by sodium chloride solution, an obvious shrinkage pattern is exhibited in the initial stage of moisture loss. When the moisture loss exceeds 2.5%, the shrinkage strain begins to decrease, indicating that the sample has transitioned from a shrinkage mode to an expansive mode. At this point, due to the significant moisture loss, the proportion of salt crystallization increases, leading to expansion strain, which offsets part of the shrinkage strain. For group #3, the expansion is evident from the initial stage of moisture loss. The results in Table 10 show that the R2 for the linear fitting is greater than 0.9, indicating that the expansion caused by salt crystallization and shrinkage present a linear relationship with the moisture loss, consistent with the shrinkage pattern of commonly cement-stabilized macadam (Tran et al., 2021). Comparing the shrinkage coefficients of fitting ① and ②, it can be seen that sodium chloride helps to reduce the shrinkage coefficient of cement-stabilized macadam, and chloride salts may have a positive effect in reducing the shrinkage cracks of the cement-stabilized macadam mixture. However, when the moisture loss reaches 2.5%, the amount of sodium chloride crystallization continuously increases, leading to a significant expansion in the cement-stabilized macadam (fitting ③). The expansion effect is greater than the shrinkage according to the absolute value of the shrinkage coefficient. The samples infiltrated with a mixed solution exhibit an expansion from the initial stage of moisture loss, once again proving that the coupling of sodium chloride and sodium sulfate will have an adverse influence on the volume stability of cement-stabilized macadam. Comparing the shrinkage coefficients in Table 10, the chloride salts will lead to a reduction in shrinkage, while the coupling action of sodium chloride and sodium sulfate will lead to expansion of cement-stabilized macadam. In saline areas, if the content of chloride and sulfate in the subbase layer continuously increases, it inevitably causes expansion due to salt crystallization and precipitation. When the expansion strain exceeds the limit of the subbase layer, the pavement will bulge, resulting in road arching up. Therefore, it can be inferred that the arching up of the asphalt pavement in saline areas is closely related to the salt type and concentration. The combined action of chloride and sulfate salts is the main reason of road arching up.
3.5 Ions concentration in cement-stabilized mortar
The content of SO42- and Cl− in the cement-stabilized mortar was determined by titration, as listed in Table 11.
From Table 11, it can be determined that both the sulfate ion content and the chloride ion content exhibit a characteristic of higher concentrations at the ends and lower concentrations in the middle of the specimen, which may be caused by the microstructure of the cement-stabilized mortar. During the sample molding process, due to the boundary effect of the mold, the compaction degree at both ends of the sample is lower than that of the central area, leading to an increase in micro-pores and a stronger capillary action, which is conducive to the rapid migration of the salt solution, manifesting as a higher ion content at the ends. Comparing the conditions of groups #1 to #9, the ion concentration increases with the increase of solution concentration and also gradually increases with the infiltration cycles. The relationship between ion content and the strength of cement-stabilized mortar is shown in Figure 13.
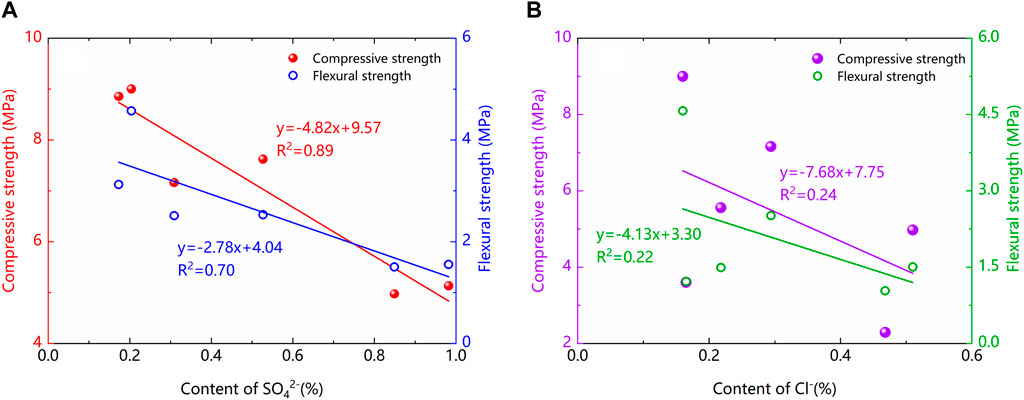
Figure 13. Correlation between the strength and ion content. (A) strength VS SO42-; (B) strength VS Cl-.
From Figure 13A, it can be seen that there is a good linear correlation between the compressive and flexural strengths of cement-stabilized mortar and the content of SO42-. The slope of linear fitting indicates that the decay rate of compressive strength is greater than that of flexural strength, suggesting that compressive strength is more sensitive to changes in SO42-. Figure 13B shows that chloride salts also lead to a decline in the strengths, but there is a general correlation between chloride ion content and strength, which may be due to the coupling effect caused by coexisting sulfates. Comparing Figures 13A, B, the variation in chloride ion content has significant impact on the compressive and flexural strengths of cement-stabilized mortar, as the decay rate of both strengths with the chloride ion is greater than that of sulfate ions.
4 Conclusion
This paper investigates the impact of salt erosion on the mechanical properties and shrinkage performance of cement-stabilized macadam, and determines the internal chloride and sulfate ion concentrations of cement-stabilized mortar through ion titration experiments, analyzing the salt erosion damage mechanism. The following conclusions can be drawn from this study.
• During the infiltration process, the type of salt solution has different effects on the weight change of cement-stabilized mortar, sulfate solution causes a reduction in sample weight, while chloride solution and mixed solutions lead to an increase in sample weight.
• Salt solution will lead to a decrease in the strength of cement-stabilized mortar and cement-stabilized macadam, with the strength of cement-stabilized mortar potentially dropping to 22.7% of the control group, and the strength of cement-stabilized macadam potentially dropping to 71% of the control group. In saline areas, the transport and accumulation of chloride and sulfate salts will have adverse effects on the strength of cement-stabilized subbase layer, leading to a significant decrease in strength.
• The migration of salt solution into the cement-stabilized macadam mixture changes the drying shrinkage properties, causing it to expand during the drying process. When the moisture loss is sufficiently high (exceeding 2.5%) or the salt solution concentration is high, the cement-stabilized macadam transitions from shrinkage to expansion. This can lead to the arching disease of the cement-stabilized subbase layer.
Data availability statement
The original contributions presented in the study are included in the article/supplementary material, further inquiries can be directed to the corresponding authors.
Author contributions
CW: Data curation, Investigation, Validation, Writing–original draft. YC: Data curation, Writing–review and editing. BA: Conceptualization, Methodology, Writing–review and editing. QG: Conceptualization, Formal Analysis, Funding acquisition, Writing–review and editing. YW: Data curation, Software, Visualization, Writing–review and editing.
Funding
The author(s) declare that financial support was received for the research, authorship, and/or publication of this article. The authors express their appreciation for the financial support of National Natural Science Foundation of China under Grant No. 51508150.
Conflict of interest
Author BA was employed by Gansu Province Transportation Planning Survey & Design Institute Co., Ltd.
The remaining authors declare that the research was conducted in the absence of any commercial or financial relationships that could be construed as a potential conflict of interest.
Publisher’s note
All claims expressed in this article are solely those of the authors and do not necessarily represent those of their affiliated organizations, or those of the publisher, the editors and the reviewers. Any product that may be evaluated in this article, or claim that may be made by its manufacturer, is not guaranteed or endorsed by the publisher.
References
Chen, D., Harris, P., Scullio, T., and Bilyeu, J. (2005). Forensic investigation of a sulfate-heaved project in Texas. J. Perform. Constr. Facil. 19 (4), 324–330. doi:10.1061/(asce)0887-3828(2005)19:4(324)
Dai, Z., Mao, X., Xu, W., Tang, K., Wu, Q., and Tang, W. (2021). Investigation and analysis of the causes of arch expansion of cement stabilized macadam base asphalt pavement in Gobi saline soil area. China Sci. Pap. 16 (4), 444–449.
Hong, W. (2019). Investigation of asphalt pavement blow-up on cement stabilized macadam base and preventive measures in Gobi desert. Highway 64 (03), 266–274.
Huang, X. (2017). Research on the bulging phenomenon of semi-rigid base course in road surfaces and strategies for elimination. Highway 62 (12), 59–61.
Irakoze, W., Prodjinoto, H., Nijimbere, S., Bizimana, J. B., Bigirimana, J., Rufyikiri, G., et al. (2021). NaCl- and Na2SO4-induced salinity differentially affect clay soil chemical properties and yield components of two rice cultivars (Oryza sativa L.) in Burundi. Agronomy 11, 571. doi:10.3390/agronomy11030571
Jidong, T., He, Z., Sheng, Z., and Daichao, S. (2016). Moisture transfer and phase change in unsaturated soils: physical mechanism and numerical model for two types of “canopy effect”. Chin. J. Geotechnical Eng. 38 (10), 1813–1821. doi:10.11779/CJGE201610010
Ministry of Transport's Research Institute of Highway (RIH) (2017). Specifications for design of highway asphalt pavement. JTG D50-2017, 9.
Ministry of Transport's Research Institute of Highway (RIH) (2021). Test methods of soils for Highway engineering, 1. JTG 3430-2020.
Mitchell, J. K. (1986). The twentieth terzaghi lecture. J. Geotechnical Engineering-Asce 112 (3), 255–289. doi:10.1061/(asce)0733-9410(1986)112:3(255)
Rollings, R. S., Burkes, J. P., and Rollings, M. P. (1999). Sulfate attack on cement-stabilized sand. J. Geotechnical Geo-environmental Eng. 125 (5), 364–372. doi:10.1061/(asce)1090-0241(1999)125:5(364)
Song, L., Song, Z., Wang, C., Wang, X., and Yu, G. (2019). Arch expansion characteristics of highway cement-stabilized macadam base in Xinjiang, China. Constr. Build. Mater. 215, 264–274. doi:10.1016/j.conbuildmat.2019.04.193
Standardization Administration of China(SAC) (2021) Test method of cement mortar strength. ISO method.GB/T 17671-2021.
Tang, Ke (2022). Study on arch expansion and damage mechanism of cement stabilized macadam base under the action of temperature and salt. Xi'an, China: Chang'an University. PhD dissertation.
Tang, Ke, Mao, X. S., Wu, Q., Zhang, J. X., and Huang, W. J. (2020). Influence of temperature and sodium sulfate content on the compaction characteristics of cement-stabilized macadam base materials. Materials 13 (16), 3610. doi:10.3390/ma13163610
Tran, N. P., Gunasekara, C., Law, D. W., Houshyar, S., Setunge, S., and Cwirzen, A. (2021). A critical review on drying shrinkage mitigation strategies in cement-based materials. J. Build. Eng. 38, 102210. doi:10.1016/j.jobe.2021.102210
Wang, P., Ye, Y., Zhang, Q., Liu, J., and Yao, J. (2020). Investigation on the sulfate attack-induced heave of a ballast less track railway subgrade. Transp. Geotech. 23 (C), 100316–100318. doi:10.1016/j.trgeo.2020.100316
Wang, Y. (2023). Effect of salt erosion on pavement performance of cement stabilized macadam and interaction mechanism. Master Disseration. Handan, China: Hebei University of Engineering.
Xie, H. (2019). Mechanism analysis and preventive measures of arch expansion of asphalt pavement on cement stabilized macadam base in Gobi desert. Highw. Eng. 44 (05), 180–187.
Yao, A., Han, F., Xu, M., Wang, J., and Yu, S. (2020). Swelling analysis of cement stabilized macadam mixture. Sci. Technol. Eng. 2020 (12), 4902–4908.
Yao, A., Wang, J., Xu, M., Yang, M., and Yang, T. (2021). Numerical analysis of the uplift of cement stabilized macadam base asphalt pavement. J. Chongqing Jiaot. Univ. Nat. Sci. 40 (6), 105–111.
Zhang, H. (2018). The cause and mechanical analysis on the transverse uplift of asphalt concrete pavement in desert are. Huhehot, China: Inner Mongolia University. Master Thesis.
Keywords: cement-stabilized macadam, arch expansion, salt erosion, mechanical property, drying shrinkage
Citation: Wang C, Chen Y, An B, Guo Q and Wang Y (2024) Impact of salt erosion on mechanical and drying shrinkage performance of cement stabilized macadam. Front. Mater. 11:1453768. doi: 10.3389/fmats.2024.1453768
Received: 24 June 2024; Accepted: 22 July 2024;
Published: 14 August 2024.
Edited by:
Leilei Chen, Southeast University, ChinaReviewed by:
Fang Liu, Taiyuan University of Technology, ChinaZhang Anshun, Changsha University of Science and Technology, China
Copyright © 2024 Wang, Chen, An, Guo and Wang. This is an open-access article distributed under the terms of the Creative Commons Attribution License (CC BY). The use, distribution or reproduction in other forums is permitted, provided the original author(s) and the copyright owner(s) are credited and that the original publication in this journal is cited, in accordance with accepted academic practice. No use, distribution or reproduction is permitted which does not comply with these terms.
*Correspondence: Yadi Chen, Y2hlbnlhZGkyMDIwQDE2My5jb20=; Qinglin Guo, Z3VvcWxAaGViZXUuZWR1LmNu