- Chongqing Industry Polytechnic College, Chongqing, China
Cementing, as an important part of the petroleum industry, plays a crucial role in ensuring the normal production and development of oil and gas resources. However, due to the brittle nature of Portland cement, it is prone to micro-cracks when subjected to downhole impact or vibration, which affects normal operations. Moreover, conventional elastic particles have a significant impact on the performance of cement paste, prompting people to continuously research new toughening admixtures. This article reviews the mechanism of toughening additives for oil well cement, the research status of physical toughening agents (fibers/whiskers, graphene) and polymer toughening agents (latex, epoxy resin, asphalt), aiming to provide reference and guidance for the development of new toughening additives.
Introduction
Cementing is the process of injecting cement slurry into the annular space formed by the formation and casing during drilling operations. As the cement slurry solidifies, it will eventually form a tightly sealed, complete, and effective cementing cement sheath (Shen et al., 2017; Zhang et al., 2022; Li et al., 2015a). Although the cement sheath has high compressive strength after hardening, it still retains the brittle characteristics of Portland cement. Its low tensile strength, poor resistance to cracking, low impact strength, poor coordination ability of denaturation, and high solidification shrinkage rate can easily lead to the failure of cement stone sealing (Figure 1), and directly affect and restrict the application and development of cement stone (Yang, 2011; Hui, 2000; Taylor et al., 1997). Therefore, it is necessary to introduce admixtures with certain toughening effects into the cement slurry formulation system. Up to now, there have been continuous researchers focusing on improving this issue to achieve the toughness transformation of cement stone. This article summarizes the current research status of physical toughening agents (fibers/whiskers, graphene) and polymer toughening agents (latex, epoxy resin, asphalt) that are at the forefront of research. The advantages and disadvantages of various toughening adA taxonomy/nomenclature query was posted in the proof. Please check. mixtures are discussed, aiming to provide guidance and reference for the development of ce + ment toughening materials.
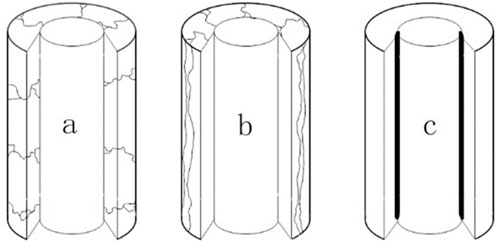
Figure 1. Several failure forms of cement ring. (A) Compressive stress failure; (B) Tensile stress failure; (C) Microannulus.
Toughening mechanism
Physical effects
The physical effect of oil well cement toughening admixture is mainly reflected in the improvement of the microstructure and fracture ductility of cement stone. In terms of microstructure, due to the presence of many pores inside Portland cement at a constant water-cement ratio, physical toughening agents such as carbon nanotubes and microspheres often use fillers with smaller particle sizes/diameters, which effectively enhance the compressive strength and durability of cement paste while improving the compactness of the cement matrix (Liu et al., 2023; Kanagaraj et al., 2023). However, these effects are relatively weak. Fiber/whisker toughening admixtures are mainly explained by micromechanical mechanisms such as bridging, fracture, crack deflection, and fiber/whisker pull-out (Liu et al., 2024; Paiva et al., 2019). Bridging is the core mechanism for the toughness of fiber-reinforced cement-based materials (Figure 2). Rough fibers can penetrate through the cross-section of cement stone. By improving the lateral ductility of cement stone, it can absorb the energy of crack propagation to a certain extent, or change the direction of crack propagation, thereby inhibiting the development of microcracks, reducing the brittleness of cement slurry, and improving the toughness of the matrix (Cao et al., 2016).
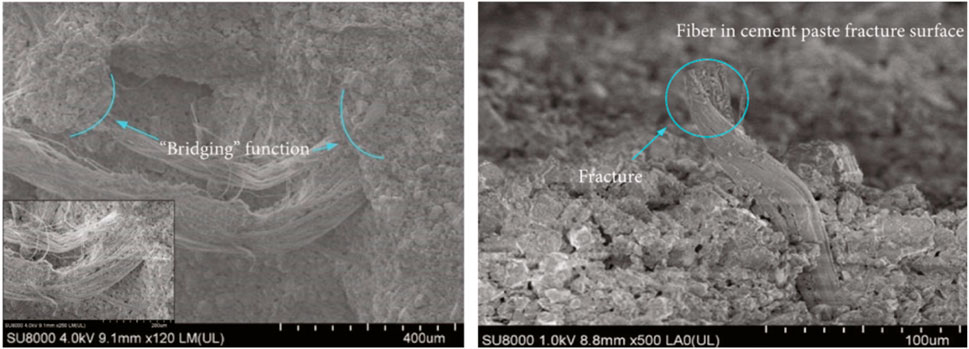
Figure 2. The bridging mechanism of fibers (Zhang et al., 2019)
The mechanism of action of physical filler-based toughening agents is simple. As they do not participate in hydration reactions, they have a relatively small impact on the mechanical properties of cement slurry systems. Moreover, most physical filler-based toughening agents have a low cost and are more suitable for practical projects with large amounts of cement. However, such toughening agents usually have significant effects at high dosages, and excessive dosages can seriously affect the fluidity of cement slurry.
Chemical action
The chemical effect of cementing cement toughening admixtures is reflected in the improvement of the microstructure, which is mainly composed of high molecular polymers. Different from the way fibers/whiskers, carbon nanotubes, and other materials change the cement matrix, the high molecular polymers, which are mainly composed of epoxy resin, adhesive lotion, etc., change the cement matrix significantly (Dominguez-Candela et al., 2023). By adjusting the high molecular polymers to water-based lotion, the problem of insufficient compatibility with cement slurry can be effectively solved. Such materials can be inserted into the internal micropores of the cement matrix to solidify and set, forming a cross-linked network structure, thus enhancing the cohesion and cohesion of materials. Unlike physical methods, the dosage of admixtures in chemical methods can usually reach 15%–45%, with little impact on the fluidity of cement slurry, and can reduce the permeability of cement paste to below 0.01mD. In addition, such admixtures can not only improve the toughness of cement stone but also effectively enhance its acid and alkali corrosion resistance (Kumar et al., 2022; Dai et al., 2022).
Factors affecting cement toughening additives
Environmental factors
In actual working conditions, toughening agents are often affected by factors such as freeze-thaw cycles and corrosion. Among them, fiber toughening agents are less affected by environmental factors. This is because fiber toughening agents inherently possess excellent temperature resistance, pressure resistance, and corrosion resistance, and are mostly interspersed in the cement matrix as physical fillers. As a result, their performance in freeze-thaw and corrosive environments mainly depends on the cement matrix itself (Tao et al., 2022). Resin toughening agents, due to their good wettability and acid/alkali resistance, can effectively penetrate the microchannels in the cement matrix, inhibiting the initiation, expansion, and nucleation of internal micro-defects, reducing the irregularity of crack structures, and playing a role in supplementing and refining internal micropores. Additionally, with the use of curing agents, resin can form a corrosion-resistant, high-toughness hardened layer on the surface of the cement stone. However, resin materials tend to soften to varying degrees at temperatures above 80°C, similar to asphalt toughening agents (Fernández-Ruiz et al., 2018; Zhang et al., 2023). Furthermore, resin needs to be adjusted to a water-based emulsion to maintain good compatibility with the cement paste. As for oxidized graphene oxide (GO), this material can effectively enhance the strength and relative dynamic modulus of elasticity of cement in the early and late stages of freeze-thaw cycles, delaying the apparent damage and corrosion processes. This is because oxidized graphene oxide refines the pore size distribution of the cement stone, inducing the growth of hydration products into petal-like and polyhedral crystal clusters, which better fill the internal pores of concrete and inhibit crack propagation (Zhang et al., 2024).
Cost factors
In the selection of cement toughening materials, fibers and asphalt additives are generally less expensive than graphene and resin. For instance, the most common polypropylene fiber costs approximately $1.1/kg, while graphene is $16.7/kg, epoxy resin is $1.9/kg, and asphalt is $0.08/kg (Ibrahim et al., 2024; Chen et al., 2023). Clearly, asphalt has the lowest cost, but as a thermoplastic material, its performance as a cement toughening agent is greatly affected by temperature and has poor fluidity. Therefore, considering both cost and toughening effectiveness, fibers are undoubtedly the best option. They have good physical properties and chemical stability, high strength, and abrasion resistance, while not affecting cement hydration. However, it is important to note that a high fiber dosage can significantly impact the fluidity of the cement paste, and the smooth surface of the fibers has insufficient bonding performance with the cement matrix. Typically, acid washing, wetting modifications, and other methods are necessary to enhance the “pull-out” performance with the cement matrix. Additionally, fiber toughening agents do not benefit the filling of micropores in the cement matrix. On the other hand, resin toughening agents are much more expensive than fibers, but they offer better performance in certain special applications (such as contact with metals or corrosive environments) (Ju et al., 2023). The resin content can even exceed 50%, owing to their good fluidity and ability to effectively fill micropores within the cement stone, similar to the action of graphene. However, the downside is that resins can inhibit cement hydration, leading to a significant reduction in strength.
Cement additive factors
Reinforcing agents such as fibers, graphene, resin, and asphalt in cement additives, especially their impact on water reducers and fluid loss additives, can be discussed from the following aspects: Fibers at relatively low dosages (exceeding 0.04%) can significantly affect the water-reducing effectiveness of cement. However, insufficient dispersion may lead to fiber agglomeration, increasing the fluid loss of the cement slurry. Modified fibers (such as modified cellulose), on the other hand, can effectively enhance the stability of the cement slurry system and reduce fluid loss. Graphene oxide, as a new nanomaterial, can improve the fluidity of cement slurry by reducing friction between cement particles, though it has little effect on the fluid loss rate (Wang et al., 2019). The role of resin in water reducers may not be directly reflected in the water reduction rate, but its high viscosity and network structure can enhance the stability of the cementitious structure, thus helping to reduce the fluid loss of the cement slurry (Wang et al., 2018). For asphalt, emulsified asphalt may cause competitive adsorption with the anions of water reducers, affecting the charge distribution and wetting state on the surface of cement particles. This competitive adsorption can influence the hydration process of cement and the demulsification behavior of emulsified asphalt, resulting in reduced stability of the cement slurry.
In summary, by analyzing the influencing factors of reinforcing materials, it is evident that from a cost perspective, materials such as fibers and asphalt have a clear price advantage. Fibers are notably effective in reinforcement, but their dosage is limited and they do not significantly improve the corrosion resistance of the cement matrix. Graphene and resins, although they can significantly enhance the properties of the cement matrix through micropore filling, are still limited to certain special operation environments due to their high cost. Therefore, in practical applications, comprehensive consideration and optimization adjustments should be made based on engineering requirements, material characteristics, and environmental conditions.
Research status of modified toughening admixtures
Fiber/whisker toughened cement stone
Fiber-toughened cementitious stone is composed of hydrated cement slurry as the matrix and discontinuous long and short fibers as reinforcing materials. Whiskers also belong to the category of fibers and are a type of fiber grown in single crystal form under artificial control. The biggest difference compared to fibers is their extremely small diameter (micrometer level), which avoids defects such as grain boundaries, dislocations, and holes in conventional materials (Khaloo et al., 1996; Elbadry et al., 2000). Their atomic arrangement is highly ordered, so their strength is close to the theoretical value of a complete crystal. Currently, there are several theories to explain and analyze the mechanism of fiber/whisker crack resistance and toughening:
Fiber spacing theory
The insufficient toughness of cement stone begins with the original defects (pores, cracks, etc.) existing inside, and the appropriate size and quantity of fibers can fill these original pores (Romualdi et al., 1964), effectively reducing internal cracks, preventing crack propagation, and playing a certain role in preventing cracks.
Composite material theory
There is a certain linear relationship between the strength and elastic modulus of cement stone and the matrix and fibers, and the strength of composite materials shows a linear growth trend with the increase of fiber content and strength (Swamy et al., 1980).
Due to being a physical admixture, fibers/whiskers often do not participate in any reaction during the cement solidification process in terms of cement mechanical performance debugging. After the cement stone is formed, the main methods to reduce bending fracture resistance are section bridging, fiber drawing, filling, crack deflection, peeling, and energy consumption by pulling out, to achieve the goal of strengthening and toughening (Das et al., 2015). Peng et al. (2023a) explored the impact of hydrothermal synthesis of xonotlite whiskers on the high-temperature performance of cement paste, and found that when cured at 290° C for 7 days, the strength reduction rate of cement mixed with xonotlite whiskers (the reduction rate of cement paste is 77.4%) was only 37.8%. This is because xonotlite whiskers can effectively promote the pozzolanic effect of SiO2, enhance the generation density of C-S-H gel, and the xonotlite whiskers still maintain needle shape at high temperature, effectively delaying the development of microcracks in cement paste. Geng Y et al. (2023) investigated the effect of SiO2-modified carbon fibers (CFs) on the mechanical properties of cement paste. After 14 days of curing, it was found that the toughness of the cement stone was significantly improved, with a compressive strength of 32.98 MPa and a tensile strength increase of 162%. This is because SiO2 effectively enhances the hydrophilicity of the CF surface and enhances the adhesion between the cement interface and fibers. Li et al. (2015b) investigated the effect of methyl cellulose and carboxymethyl cellulose on carbon fibers and found that a 0.2wt% CMC solution can effectively disperse carbon fibers. The addition of 0.3wt% carbon fibers increased the compressive strength, flexural strength, and splitting tensile strength of cement stone samples by 8.6%, 31.5%, and 52.4%, respectively. The elastic modulus decreased by 49.5% compared to pure cement samples.
Of course, the problem of toughening cement paste with fibers/whiskers is also quite obvious. If the amount used is too small, the toughening effect is not good. Even high-performance fiber-reinforced cementitious materials with very high fiber content are difficult to show quasi-strain hardening characteristics under direct tensile loads. At the same time, in the process of toughening composite cement paste with fibers/whiskers, to ensure that the composite cement paste has high toughness, the poor formulation flowability and increased cost caused by increasing fiber content are also urgent issues to consider.
Graphene-toughened cement stone
Graphene, as the material with the highest strength, best toughness, and largest specific surface area discovered by humans to date, has a theoretical Young’s modulus of 1.0 TPa and an inherent tensile strength of 130 GPa. Due to the presence of a large number of oxygen-containing functional groups, it exhibits excellent toughening effects on some polymer materials and inorganic non-metallic materials.
In terms of the mechanism analysis of graphene toughening cement paste, Guojian Jing et al. (2017) believe that graphene microsheets (GNPs) are mechanically dispersed in the cementitious matrix, mainly playing a physical toughening role. Graphene can, on the one hand, prevent crack propagation through crack deflection, bridging, and branching, and achieve toughening through interface detachment in a pull-out state. On the other hand, at the critical section, the pull-out of graphene flakes and interlayer sliding are also additional toughening methods (Baudín et al., 2019). Lv et al. (2014) believe that when GO participates in cement hydration, it will form hydration crystal growth points by adsorbing active ingredients (such as C3S, C2S, C3A, etc.) in the cement and reacting with oxygen-containing groups on its surface. If the reaction environment is compact and dense, it will form columnar or rod-shaped hydration crystals. When the hydration crystals grow to the pores and loose structures, they will form flower-shaped structures, which will fill the pores and loose areas for repair (Sun et al., 2015) (Figure 3). The hydration crystal products gradually form flower-shaped crystals and tend to form dense structures, thereby achieving the goal of toughening.
Huang et al. (2023) improved the Hummers method to prepare graphene oxide and investigated its effect on the toughness of alkali-activated slag cement. They found that when the content of graphene oxide was 0.03%, the flexural strength of alkali-activated slag cement after 60 days of curing increased by 51.9% compared to that of cement paste. Wang et al. (2017) prepared chemically functionalized graphene oxide (GOM) from polyether amine and graphene oxide (GO) and investigated the effect of GOM on cement. They found that when the content was 0.03wt%, the flexural strength of GOM cement paste was 10.53 MPa and 11.23 MPa, which was significantly better than that of neat cement paste.
Graphene and its oxides have a significant effect on improving the compressive/flexural strength of cement, but the market price of graphene is also an important challenge that limits its use as a cement additive.
Resin-toughened cement stone
Epoxy resin can react with different types of curing agents to prepare curing products with varying performance differences, and is widely used in aerospace, aircraft manufacturing, automotive, and other fields (Saba et al., 2016; Mohan et al., 2013). The advantages currently demonstrated in cement toughening and anti-corrosion, as well as improving resistance, are gradually being reflected.
In addition to strengthening the microstructure of cement paste, the resin enhances the attraction of cations such as Ca2+ to resin molecules during hydration (Du et al., 2018). Ca2+ ions in the C-S-H gel migrate to the interface area and form electrostatic bonds with the hydroxyl groups of the resin, which is also the main source of adhesion energy. Li et al. (2022) studied the effect of self-developed waterborne epoxy resin (WEP) and its curing agent on the mechanical properties of oil well cement stone. It was found that the toughness of cement paste is optimal when the curing condition is 70° C and the WEP dosage is 10%. The polymer film formed by curing WEP can interweave with the hydration products to form a stable network structure. Peng et al. (2023b) improved the dispersibility and heat resistance of epoxy resin (E−44) by hydrophilic modification with 2-aminoethanesulfonic acid (SEA) and evaluated the effect of WEY on the mechanical properties of Portland cement paste. When the amount of WEY added was 6%, the flexural strength of cement stone increased by 43.8%, the elastic modulus decreased by 38.9%, and the compressive strength increased by 26.6%. Li et al. (2017) prepared core-shell particles by grafting amino-terminated hyperbranched polymers onto the surface of silica nanoparticles. They found that adding 3wt% core-shell particles significantly improved the tensile and impact strength of epoxy/amino terminated hyperbranched polymer thermosolids. Mechanism analysis suggests that the excellent impact resistance of epoxy/amino terminated hyperbranched polymers/core-shell particles may be due to the synergistic effect of shear deformation and crack pinning/propagation.
Epoxy resin, with its outstanding toughening and strengthening effect, has a wide range of applications in construction, electronic sealing, and biological consumables. However, when used in cement construction, its poor initial fluidity and high cost are still urgent problems that need to be solved.
Asphalt-toughened cement stone
Asphalt is a mixture of hydrocarbons with different molecular weights and their non-metallic derivatives, belonging to high-viscosity organic liquids. Due to its insulation, waterproofing, anti-corrosion, moisture-proof, crack resistance, and toughening effects, it is widely used in many fields such as construction, highways, and coatings.
Zhang X. et al. (2024) investigated the effect of natural rock asphalt on the mechanical properties of oil well cement under high temperature and pressure (320 °C). It was found that high temperature and high pressure effectively promoted the pyrolysis reaction of rock asphalt. With the extension of solidification time, the particle pores caused by the pyrolysis of rock asphalt healed, improving the interface bonding between rock asphalt and cement matrix and exhibiting a good toughening effect. Zhang et al. (2017) selected sulfonated asphalt as an additive and tested the effect of different asphalt dosages on the mechanical properties of cement paste. Compared with the cement slurry without sulfonated asphalt, the cement slurry containing 3% sulfonated asphalt increased its tensile strength by 45% at 7 days and 17% at 28 days. In addition, a 2%–3% level of sulfonated asphalt can effectively improve the toughness of oil well cement through crack deflection, pore filling, and other methods. Song et al. (2016) used low-temperature plasma technology to hydrophilic asphalt particles to achieve toughness modification of cement stone. Mechanical performance tests showed that the toughness of cement stone was significantly improved.
However, in terms of usage, the poor fluidity of asphalt remains a major challenge when used with cement slurry, which to some extent affects the rheological properties of cement slurry and increases the difficulty of preparation.
Composite toughened cement stone
To explore better improvement strategies for the toughening and strengthening of cement stone, researchers have also adopted a combination of various toughening measures to further improve the mechanical properties of cement stone.
Zhang X. et al. (2024) prepared a novel flexible resin material (C-ER) using liquid nitrile rubber (CTBN) and nano SiO2-modified epoxy resin as materials. Through experiments on the application of C-ER in cement, it was found that C-ER has good hydrophilicity and dispersibility. The introduction of flexible chain segments results in a lower modulus and crosslinking density of C-ER, and its compressive elastic modulus is reduced by 47.4% compared to pure resin. In addition, compared with blank cement and unmodified resin cement, the flexural strength of cement samples containing 4% C-ER particles increased by 9.0% and 25.1%, respectively, and the elastic modulus of cement stone decreased by 31.2% and 25.0%, respectively. Marji et al. (2023) investigated the effect of the synergistic use of multi-walled carbon nanotubes (MWCNTs) and styrene butadiene styrene (SBS) on the properties of cement paste. It was found that incorporating SBS into the cement matrix improved ductility and crack resistance. The introduction of MWCNTs improved the cohesion, microscale crack resistance, and strength performance of the cement matrix, and reduced its elastic modulus by 41.67%. Li et al. (2024) investigated the effect of synergistic use of calcium carbonate whiskers (CWs), multi-walled carbon nanotubes (MWCMTs), and carboxylated styrene butadiene rubber latex (XSBRL) on cement, and determined the optimal mixing ratio to be 3% CWs content, 0.2% MWCNTs, and 10% XSBRL. Under this condition, the maximum improvement rate of mechanical strength of cement stone can reach 12.84%, and the pore structure has been significantly optimized.
The composite toughening method can more effectively integrate the advantages and disadvantages of various toughening methods, and adjust various toughening materials according to actual working conditions. Currently, toughening research mostly uses this type of method.
Conclusion
Through systematic research and classification of mainstream research results on toughening of cement stone both domestically and internationally, the following conclusions have been drawn:
1) For the use of fiber/whisker toughening, fiber materials need to address more issues such as poor dispersibility and flowability in the formulation; For whisker materials, research objectives need to focus more on the properties of the whiskers themselves and their interface properties, or consider selecting more excellent alternative materials.
2) The use of latex should focus on improving its stability and various types of resistance.
3) As the material with the highest strength and toughness on the market, it has a significant effect on toughening cement stone. However, if considering its widespread application, the primary issue is still the consideration of cost.
4) In terms of resin toughening, if resin-containing curing agents are considered for toughening, the first and foremost consideration is undoubtedly the issues of fluidity and compatibility. Therefore, selecting appropriate diluents and how to improve compatibility with cement are the directions that need to be studied in the future; If it is used in the form of resin lotion, it is necessary to consider achieving toughening without too much reduction of cement base properties.
5) Asphalt has a lower cost, but its compatibility and poor fluidity are the primary difficulties that affect its use as a toughening additive for cement paste.
6) From an application perspective, choosing to use the above-mentioned toughening agents in combination is more in line with the actual working conditions, and adjusting according to the actual situation can effectively reduce costs without losing the purpose of toughening/strengthening.
Author contributions
YZ: Writing–original draft, Supervision, Funding acquisition.
Funding
The author(s) declare that financial support was received for the research, authorship, and/or publication of this article. This work was supported by The Scientific and Technological Research Program of Chongqing Municipal Education Commission (KJQN202303222), the Doctoral Fund of Chongqing Industry Polytechnic College (2022G2YBS2K2-12).
Conflict of interest
The author declares that the research was conducted in the absence of any commercial or financial relationships that could be construed as a potential conflict of interest.
Publisher’s note
All claims expressed in this article are solely those of the authors and do not necessarily represent those of their affiliated organizations, or those of the publisher, the editors and the reviewers. Any product that may be evaluated in this article, or claim that may be made by its manufacturer, is not guaranteed or endorsed by the publisher.
References
Baudín, C., Benet, T., and Pena, P. (2019). Effect of graphene on setting and mechanical behaviour of tricalcium phosphate bioactive cements. J. Mech. Behav. Biomed. Mater. 89 (1), 33–47. doi:10.1016/j.jmbbm.2018.09.002
Cao, M., Xu, L., and Zhang, C. (2016). Rheology, fiber distribution and mechanical properties of calcium carbonate (CaCO3) whisker reinforced cement mortar. Compos. Part A Appl. Sci. Manuf. 90 (11), 662–669. doi:10.1016/j.compositesa.2016.08.033
Chen, H. (2023). Research on the blending modification of urea formaldehyde resin and isocyanate with new process. Shandong Agricultural University. (thesis).
Dai, D., Peng, J., Wei, R., Li, L., and Lin, H. (2022). Improvement in dynamic behaviors of cement-stabilized soil by super-absorbent-polymer under cyclic loading. Soil Dyn. Earthq. Eng. 163 (9), 107554. doi:10.1016/j.soildyn.2022.107554
Das, S., Hendrix, A., Stone, D., and Neithalath, N. (2015). Flexural fracture response of a novel iron carbonate matrix – glass fiber composite and its comparison to Portland cement-based composites. Constr. and Build. Mater. 93 (9), 360–370. doi:10.1016/j.conbuildmat.2015.06.011
Dominguez-Candela, I., Perez-Nakai, A., Torres-Roca, E., Lora-Garcia, J., and Fombuena, V. (2023). Development of a novel epoxy resin based on epoxidized chia oil as matrix and maleinized chia oil as bio-renewable crosslinker. J. Appl. Polym. Sci. 140 (10), e53574. doi:10.1002/app.53574
Du, J., Bu, Y., and Shen, Z. (2018). Interfacial properties and nanostructural characteristics of epoxy resin in cement matrix. Constr. and Build. Mater. 164 (3), 103–112. doi:10.1016/j.conbuildmat.2017.12.200
Elbadry, M., Abdalla, H., and Ghali, A. (2000). Effects of temperature on the behaviour of fiber reinforced polymer reinforced concrete members: experimental studies. Can. J. Civ. Eng. 27 (5), 993–1004. doi:10.1139/cjce-27-5-993
Fernández-Ruiz, M. A., Gil-Martín, L. M., Carbonell-Márquez, J. F., and Hernández-Montes, E. (2018). Epoxy resin and ground tyre rubber replacement for cement in concrete: compressive behaviour and durability properties. Constr. Build. Mater. 173 (6), 49–57. doi:10.1016/j.conbuildmat.2018.04.004
Geng, Y., Ren, Q., Yang, Z., He, D., Wu, Z., Cai, J., et al. (2023). Preparation of SiO2 coated carbon fibers and its interfacial properties with cement paste matrix. Front. Mater. 10 (3), 1128054. doi:10.3389/fmats.2023.1128054
Huang, N., Guo, Z., Yu, L., and Tian, Y. (2023). Study on the toughening effect of oxidized graphene on alkali slag cement. New Build. Mater. 50 (06), 42–45. doi:10.3390/ma14206107
Ibrahim, H., Alam, G., and Faheem, A. (2024). Eco-economic analysis of utilizing high volumes of recycled plastic and rubber waste for green pavements: a comparative life cycle analysis. Case Stud. Constr. Mater. 28 (8), e03690. doi:10.1016/j.cscm.2024.e03690
Jing, G., Ye, Z., Lu, X., and Hou, P. (2017). Effect of graphene nanoplatelets on hydration behaviour of Portland cement by thermal analysis. Adv. Cem. Res. 29 (2), 63–70. doi:10.1680/jadcr.16.00087
Jianxiao, Y., Xuanke, L., Jinshui, L., Hongbo, L., and Fuquan, Z. (2016). Research progress on low-cost high-performance carbon fibers. High tech Fibers Appli., 41 (06), 6. doi:10.3969/j.issn.1007-9815.2016.06.002
Kanagaraj, B., Lubloy, E., Anand, N., Hlavicka, V., and Kiran, T. (2023). Investigation of physical, chemical, mechanical, and microstructural properties of cement-less concrete-state-of-the-art review. Constr. Build. Mater. 4 (2), 130020. doi:10.1016/j.conbuildmat.2022.130020
Khaloo, A., and Kim, N. (1996). Mechanical properties of normal to high-strength steel fiber-reinforced concrete. Cem. Concr. Aggregates 18 (2), 92–97. doi:10.1520/CCA10156J
Kumar, S., Manimaran, R., and Anbukarasi, K. (2022). Mechanical, wear and fatigue behavior of silane-treated corncob biosilica toughened epoxy resin composite reinforced with prickly pear short fibers. Polym. Compos. 43 (10), 6998–7006. doi:10.1002/pc.26761
Li, M., Liu, M., Yang, Y., Li, Z., and Guo, X. (2015b). Mechanical properties of oil well cement stone reinforced with hybrid fiber of calcium carbonate whisker and carbon fiber. Petroleum Explor. Dev. 42 (1), 104–111. doi:10.1016/S1876-3804(15)60012-X
Li, M., Sun, K., and He, M. (2022). Effects of waterborne epoxy resin on the mechanical properties and microstructure of oil-well cement. J. Dispersion Sci. Technol. 43 (14), 2107–2114. doi:10.1080/01932691.2021.1915158
Li, M., Yang, Y., and Guo, X. (2015a). Mechanical properties of carbon fiber reinforced oil well cement stone. Acta Mater. Compos. Sin. 32 (03), 782–788. doi:10.13801/j.cnki.fhclxb.20141010.002
Li, S., Wu, Q., Zhu, H., Lin, Q., and Wang, C. (2017). Impact resistance enhancement by adding core-shell particle to epoxy resin modified with hyperbranched polymer. Polymers 9 (12), 684. doi:10.3390/polym9120684
Li, X., Wang, Z., Zhang, M., Liu, R., Zhang, C., and Yan, J. (2024). Properties of the cement containing hybrid micro-fibers and polymer latex using the orthogonal test: a comparative study of freshwater and seawater curing conditions. Constr. Build. Mater. 411 (12), 134661. doi:10.1016/j.conbuildmat.2023.134661
Liu, H., Sun, C., and Kou, G. (2024). The effect of hard calcium silicate whiskers on the strength of oil well cement at high temperatures. Synth. Chem. 41 (1), 1–7. doi:10.15952/j.cnki.cjsc.1005-1511.24007
Liu, Q., Ming, X., Wang, M., Li, Z., Sun, G., and Liang, R. (2023). Improved mechanical strength of cement paste by polyvinylpyrrolidone and in situ polymerized acrylic acid. J. Mater. Civ. Eng. 35 (5), 04023096. doi:10.1061/jmcee7.mteng-13954
Lv, S., Liu, J., Qiu, C., Ma, Y., and Zhou, Q. (2014). The microstructure and mechanism of nano graphene oxide reinforced and toughened cement-based composites. J. Funct. Mater. 45 (4), 84–89. doi:10.3969/j.issn.1001-9731.2014.04.018
Marji, M., Yousofian, H., Soltanian, H., Pourmazaheri, Y., and Abdollahipour, A. (2023). Superior crack propagation inhibitory effectiveness of MWCNT reinforced SBS toward improving oil/gas well cement integrity. Constr. Build. Mater. 403 (8), 133171. doi:10.1016/j.conbuildmat.2023.133171
Mohan, P. (2013). A critical review: the modification, properties, and applications of epoxy resins. Polymer-plastics Technol. Eng. 52 (2), 107–125. doi:10.1080/03602559.2012.727057
Paiva, L., Ferreira, I., Martinelli, A., Freitas, J. C. d. O., and Bezerra, U. T. (2019). Milled basalt fiber reinforced Portland slurries for oil well applications. J. Petroleum Sci. and Eng. 175 (4), 184–189. doi:10.1016/j.petrol.2018.11.068
Peng, Z., Chen, J., Feng, Q., Zheng, Y., and Zhang, B. (2023a). Study of xonotlite whisker used to inhibit the high-temperature strength decline of cementing cement stone. Silicon 15 (1), 425–436. doi:10.1007/s12633-022-02032-8
Peng, Z., Zeng, W., Feng, Q., and Zheng, Y. (2023b). Study on improving the toughness of silicate cement stone by modified epoxy resin. Silicon 15 (7), 3137–3148. doi:10.1007/s12633-022-02221-5
Romualdi, J., and Mandel, J. (1964). Tensile strength of concrete affected by uniformly distributed and closely spaced short lengths of wire reinforcement. J. Proc. 61 (6), 657–672. doi:10.14359/7801
Saba, N., Jawaid, M., Alothman, O. Y., Paridah, M., and Hassan, A. (2016). Recent advances in epoxy resin, natural fiber-reinforced epoxy composites and their applications. J. Reinf. Plastics Compos. 35 (6), 447–470. doi:10.1177/0731684415618459
Shen, J., Shi, L., Li, Y., Liu, S., and Jiang, L. (2017). Analysis and prospect of cement ring sealing integrity under high pressure difference conditions. Nat. Gas. Ind. 37 (04), 98–108. doi:10.3787/j.issn.1000-0976.2017.04.012
Song, Y., Zhang, C., Cheng, X., and Guo, X. (2016). Plasma modified rock asphalt for toughness modification of oil well cement stone. Bull. Chin. Ceram. Soc. 35 (12), 4082–4087. doi:10.16552/j.cnki.issn1001-1625.2016.12.030
Sun, T. (2015) The effect of graphene oxide on the structure and properties of cement-based materials. Shaanxi University of Science and Technology. (thesis). doi:10.7666/d.D648946
Swamy, R. (1980). Fracture mechanics applied to brittle materials. Int. J. Cem. Compos. and Lightweight Concr. 2 (3), 165–166. doi:10.1016/0262-5075(80)90019-6
Tao, Z., Zhang, Y., Chen, X., and Gu, X. (2022). Effects of freeze-thaw cycles on the mechanical properties of cement-fiber composite treated silty clay. Constr. Build. Mater. 316 (1), 125867. doi:10.1016/j.conbuildmat.2021.125867
Wang, B., and Pang, B. (2019). Mechanical property and toughening mechanism of water reducing agents modified graphene nanoplatelets reinforced cement composites. Constr. Build. Mater. 226 (11), 699–711. doi:10.1016/j.conbuildmat.2019.07.229
Wang, M., Yao, H., Wang, R., and Zheng, S. (2017). Chemically functionalized graphene oxide as the additive for cement–matrix composite with enhanced fluidity and toughness. Constr. Build. Mater. 150 (9), 150–156. doi:10.1016/j.conbuildmat.2017.05.217
Wang, Q., Li, S., Pan, S., and Guo, Z. w. (2018). Synthesis and properties of a silane and copolymer-modified graphene oxide for use as a water-reducing agent in cement pastes. New carbon Mater. 33 (2), 131–139. doi:10.1016/S1872-5805(18)60330-0
Yang, Z. (2011). A study on the factors influencing the toughness and strength of cementing cement stone. Chengdu University of Technology. 2011. (thesis).
Zhang, C., Song, Y., Wang, W., Guo, X., and Li, H. (2017). The influence of sulfonated asphalt on the mechanical properties and microstructure of oil well cement paste. Constr. and Build. Mater. 132 (2), 438–445. doi:10.1016/j.conbuildmat.2016.12.014
Zhang, C., Wang, J., Cai, J., Li, K., Hu, C., Mei, K., et al. (2024a). The effect of evolution of rock asphalt subjected to high temperature and high pressure on the mechanical properties of oil well cement. Constr. Build. Mater. 420 (3), 135323. doi:10.1016/j.conbuildmat.2024.135323
Zhang, R., Long, Z., Long, G., Wang, J., Wang, X., Zhang, X., et al. (2024). Mechanism of graphene oxide concrete macro-micro properties evolution under large temperature difference freeze-thaw action. Constr. Build. Mater. 415 (2), 135019. doi:10.1016/j.conbuildmat.2024.135019
Zhang, X., Peng, Z., Feng, Q., and Zheng, Y. (2024b). Effect of CTBN and Nano-SiO2 modified epoxy resin powder on the mechanical properties of cement-based composites. J. Build. Eng. 83 (1), 108456. doi:10.1016/j.jobe.2024.108456
Zhang, Y., He, M., Xu, M., and Xu, P. (2019). Effect of acid activation-hydrophilic-modified sepiolite on comprehensive properties of oil-well cement. J. Chem. 2019 (12), 1–11. doi:10.1155/2019/4289315
Zhang, Y., Xu, M., Song, J., Wang, C., Wang, X., and Hamad, B. A. (2022). Study on the corrosion change law and prediction model of cement stone in oil wells with CO2 corrosion in ultra-high-temperature acid gas wells. Constr. Build. Mater. 323, 125879. doi:10.1016/j.conbuildmat.2021.125879
Keywords: toughening, asphalt, resin, graphene, review
Citation: Zhang Y (2024) Application of modified and toughened admixtures in well cementing slurry: Progress and challenges. Front. Mater. 11:1451609. doi: 10.3389/fmats.2024.1451609
Received: 19 June 2024; Accepted: 09 September 2024;
Published: 23 September 2024.
Edited by:
Hamada Elsayed, University of Padua, ItalyReviewed by:
Nahla Hilal, University of Fallujah, IraqArnab Bose, Boehringer Ingelheim, United States
Copyright © 2024 Zhang. This is an open-access article distributed under the terms of the Creative Commons Attribution License (CC BY). The use, distribution or reproduction in other forums is permitted, provided the original author(s) and the copyright owner(s) are credited and that the original publication in this journal is cited, in accordance with accepted academic practice. No use, distribution or reproduction is permitted which does not comply with these terms.
*Correspondence: Yihang Zhang, bHVja3l6aGFuZ3loQGZveG1haWwuY29t