- 1Department of Plastic Surgery, Zhejiang Rongjun Hospital, Jiaxing, Zhejiang, China
- 2Department of Dermatology, Zhejiang Rongjun Hospital, Jiaxing, Zhejiang, China
- 3General Surgery Department, Jiaxing No. 1 Hospital, Jiaxing, Zhejiang, China
Skin photoaging, caused by prolonged exposure to ultraviolet (UV) radiation, manifests as intricate biological transformations, encompassing oxidative damage and structural alterations. Despite a growing need for effective interventions, the existing therapeutic repertoire for treating skin photoaging remains constrained. Recent investigations have shifted focus towards the application of hydrogels as a novel avenue for addressing this concern. Various active substances can be combined with hydrogels for transdermal delivery, including compounds from the ocean, plants, cell active substances, which can promote skin UV damage repair mainly through antioxidant, anti-inflammatory and promote collagen production. This review seeks to offer a thorough summary of recent progress in employing hydrogels for addressing skin photoaging. Topics covered include the mechanism of skin photoaging, and emerging trends in hydrogel-based therapies for skin rejuvenation. The discussion also explores challenges and outlines potential future directions in leveraging hydrogels as therapeutic agents to address skin photoaging.
1 Introduction
The skin, our body’s largest protective organ, is prone to injuries from genetic factors, lifestyle choices, diet, sunlight, and the environment (Hubbard et al., 2014; Belkaid and Segre, 2014). The consequences of skin aging and diseases go beyond physical implications, impacting individuals, families, and society at large in terms of mental, social, and financial aspects (Rittié and Fisher, 2015; Gu et al., 2020; Sreedhar et al., 2020). Notably, skin photoaging, a prominent sign of skin aging, mainly results from prolonged UV radiation exposure (Fisher et al., 2002; Battie et al., 2014). This type of aging is marked by fine lines, wrinkles, uneven pigmentation, and decreased skin elasticity (Cao et al., 2020; Zhang and Duan, 2018; Letsiou, 2021). Structural changes in photoaged skin involve collagen fiber breakdown, abnormal elastic fiber buildup, and disruption of the epidermal barrier (Papaccio et al., 2022; Schuch et al., 2017; Nakanishi et al., 2009). Skin photoaging, characterized by the premature aging of the skin due to chronic exposure to UV radiation, is a significant dermatological concern. Unlike intrinsic aging, which occurs naturally over time, photoaging is primarily induced by external environmental factors, particularly UV radiation from the sun. This process involves a complex interplay of molecular and cellular mechanisms, leading to visible changes such as wrinkles, loss of elasticity, and hyperpigmentation (Chaudhary et al., 2020; Zhao Y. et al., 2021a; Sales et al., 2022). The pathophysiology of skin photoaging is primarily driven by DNA damage and collagen degradation. UV radiation penetrates the skin and generates reactive oxygen species (ROS), which in turn cause oxidative stress (Kim et al., 2022). This oxidative stress results in direct damage to cellular DNA, leading to mutations and impaired cellular functions (Schuch et al., 2017; Poon et al., 2015). Moreover, UV radiation disrupts the extracellular matrix by accelerating the degradation of collagen, the protein responsible for maintaining skin structure and elasticity (Battie et al., 2014). The combination of these effects results in the characteristic signs of photoaged skin.
Current treatment methods for skin photoaging include topical antioxidants, laser therapy, and chemical peels (Poon et al., 2015). Topical antioxidants, such as vitamins C and E, aim to neutralize ROS and reduce oxidative stress (Tran et al., 2023). However, their efficacy is often limited by poor skin penetration and stability issues. Laser therapy and chemical peels, while effective in promoting skin renewal and collagen production, can be associated with side effects such as redness, swelling, and risk of infection (Hamilton and Kao, 2020; Sharad, 2013). Additionally, these treatments often require multiple sessions and maintenance, posing a challenge for long-term management of photoaging. Given these limitations, there is an urgent need for innovative and effective approaches to prevent and treat skin photoaging. Current research aims to address these gaps by exploring novel therapeutic strategies that target the underlying mechanisms of photoaging. By focusing on enhancing skin repair and protection at the molecular level, we hope to develop treatments that offer more sustained and comprehensive benefits for aging skin.
Recently, hydrogel materials, renowned for their exceptional water retention capabilities and biocompatibility, have emerged as versatile substances with vast applications (Cao H. et al., 2021a; Ho et al., 2022; Zhao et al., 2020). Their unique properties make them well-suited for creating conducive environments that promote various biological processes, particularly in the realms of wound healing and skin injury repair (Kim et al., 2023; Xiong et al., 2022; Wang et al., 2021). In the context of wound healing, hydrogel materials play a pivotal role in maintaining a moist and supportive milieu at the wound site (Cortes et al., 2020; Wang Y. et al., 2024a). This environment accelerates the healing process by facilitating cell migration, proliferation, and tissue regeneration (Mast et al., 1991). The inherent softness and permeability of hydrogels distinguish them from traditional dressings, providing a more favorable substrate for wound recovery (Francesko et al., 2018). The synergy of hydrogel materials with stem cells has garnered significant attention in advancing wound healing and skin injury repair (Fu et al., 2023). The combination capitalizes on the regenerative potential of stem cells, further enhancing tissue repair mechanisms. Studies have demonstrated promising outcomes in burn injuries, ulcers, and various skin lesions, showcasing the considerable potential of hydrogel-stem cell combinations as innovative therapeutic strategies (Li et al., 2021; Khayambashi et al., 2021). Skin photoaging is essentially a form of skin damage. Hydrogels can also serve as a drug delivery tool for treating skin photoaging damage, facilitating the administration of drugs to rejuvenate the skin, promoting a more youthful appearance (Zhao X. et al., 2021b; Li et al., 2023). Additionally, hydrogels can be combined with manufacturing technologies such as 3D printing to create more efficient transdermal drug delivery systems, including innovations like microneedle patch (Vijayavenkataraman et al., 2018; Huang et al., 2023; Cassano et al., 2023).
This review offers a detailed look at the recent advancements in using hydrogels as carriers for therapeutic agents or bioactive molecules to treat skin aging. Hydrogels, primarily composed of diverse polymeric materials, are employed to deliver a variety of substances, including natural products, stem cell extracts, extracellular vesicles, and growth factors, to address skin damage caused by UVA/UVB and facilitate skin rejuvenation. Additionally, we explore how hydrogels can enhance the loading efficiency of these bioactive substances and facilitate effective transdermal delivery for therapeutic interventions. In conclusion, we address the challenges and outline future directions for utilizing hydrogels in the treatment of skin photoaging.
2 Attributes of skin photoaging
Skin photoaging is a complex biological process marked by premature aging and damage due to extended exposure to sunlight’s ultraviolet (UV) radiation (Wang et al., 2019). This issue has worldwide ramifications for individuals’ looks, health, and general welfare. This section offers a thorough overview of the mechanisms behind skin photoaging, encompassing molecular and cellular changes induced by UV exposure (Battie et al., 2014). The exploration expands to cover the clinical signs of photoaged skin, including wrinkles, pigmentation changes, and decreased elasticity (Pourang et al., 2022; Fitsiou et al., 2021). Many studies have shown that skin photoaging mainly involves epidermal fibroblasts, keratinocytes and mesenchymal dermal cells.
UV radiation initiates a sequence of molecular and cellular processes that contribute to accelerated skin aging as depicted in Figure 1 (Kim et al., 2022). These processes result in the production of reactive oxygen species (ROS), leading to oxidative stress and DNA damage (Tan et al., 2022). Ferritin is an important marker of antioxidant activity, and its elevation proves that UV radiation induces oxidative stress. Activation of inflammatory pathways, such as NF-kB signaling, they increased expression of matrix metalloproteinases (MMPs), heme oxygenase-1, superoxide dismutase-2 and interference with collagen and elastin synthesis contribute to skin degradation and reduced elasticity (Kim et al., 2022). Additionally, UVA radiation also induced a significant increase in the expression of tenascin, a protein closely associated with skin cancer lesions, and UVA radiation induced significant lysozyme deposition. In the skin, lysozyme deposits are seen on elastic fibers in sunburned areas, and the number of lysozyme-containing elastin fibers appears to correlate with the degree of sunburn (Lim et al., 2008; Ortonne, 1990).
A variety of therapeutic strategies are employed to prevent and treat skin aging. Key preventive measures include the use of broad-spectrum sunscreens and other photoprotective practices such as minimizing sun exposure and wearing protective clothing (Poon et al., 2015). Topical antioxidants like vitamins C and E have shown potential in mitigating oxidative stress and protecting against UV-induced damage (Greul et al., 2002; Gaspar and Campos, 2007; Steenvoorden and Beijersbergen van Henegouwen, 1999). Retinoids, which are vitamin A derivatives, have been proven effective in enhancing skin texture, boosting collagen production, and reducing pigmentation (Mukherjee et al., 2006; Riahi et al., 2016). The TGF signaling pathway is the most common therapeutic target for skin photoaging because it is closely related to collagen degradation caused by metalloproteinases and skin inflammation caused by UV (Li et al., 2024). Advanced technologies, such as laser therapy, intense pulsed light (IPL), and photodynamic therapy (PDT) provide targeted methods to address specific signs of photoaging (Sales et al., 2022; Wang Y. et al., 2023a). Furthermore, new approaches such as regenerative medicine, which use stem cells, growth factors, and tissue engineering, offer potential for skin rejuvenation and repair (Yan et al., 2023; Zhang et al., 2022; Zhang et al., 2020). Hydrogels can be formulated for antioxidant properties or as carriers for drugs or cytokines with photoaging repair effects.
3 Hydrogel for skin photoaging damage repair
Hydrogels, composed of natural or synthetic polymers like proteins and polysaccharides, form three-dimensional networks with high water content (Ho et al., 2022; Francesko et al., 2018). Notably, these hydrogels find significant application in tissue damage repair, creating a conducive environment for cell proliferation and migration, preventing infections, and allowing controlled release of bioactive compounds (Hu et al., 2022; Chawla et al., 2011; Xia et al., 2022). Looking ahead, their versatile and customizable nature positions bio-based hydrogels for promising roles in drug delivery, tissue engineering, and various medical innovations, including wearable sensors (Du et al., 2023; Luo et al., 2023; Rahmani and Shojaei, 2021) and regenerative medicine (Brown and Anseth, 2017; Chyzy and Plonska-Brzezinska, 2020), marking a dynamic trajectory in biomedical research with implications for personalized medicine and diverse clinical applications (Xu et al., 2021; Burdick and Prestwich, 2011; Chen et al., 2023). Here we would like to introduce some of the latest research on the application of hydrogels to skin photoaging damage.
3.1 Hydrogels derived from marine organisms resist photoaging
The use of marine resources can reduce the reliance on chemically synthesized materials in medical applications. Currently, many marine-derived biomaterials, such as sodium alginate, fish collagen, and chitosan, are commonly found in everyday life. In recent years, these materials have been extensively researched and applied in the field of hydrogels (Lewicka et al., 2024; Amnuaykan et al., 2024; Rahman et al., 2024; Guo et al., 2024). Their unique biocompatibility, degradability, and processability make them ideal choices not only for biomedical and tissue engineering applications but also for anti-aging skincare products. This is due to their ability to provide moisturizing, anti-inflammatory, and antioxidant benefits. Additionally, through certain modifications, they can become solutions for drug delivery and tissue regeneration. Recently, A team utilized marine algae extract, including sulfated galactofucan polysaccharides and alginate oligosaccharides as active ingredients, with modified polyacrylonitrile κ-carrageenan serving as the substrate, to develop a multifunctional composite hydrogel (FACP5) (Wu et al., 2023). Animal experiments assessing anti-photoaging properties showed that after FACP5 treatment, there was an increase in skin water content, a reduction in epidermal and dermal thickness, an increase in the activities of catalase and superoxide dismutase, among other effects (Wu et al., 2023). These results suggest that FACP5 has skin barrier repair, antioxidant, anti-inflammatory, and collagen degradation inhibition activities, positioning it as a skincare product for protecting against photoaging (Wu et al., 2023). And extract from fish scales of marine fish was also used to prepare a natural composite hydrogel known as SE-gel (Figure 2B) (Liu et al., 2023). This hydrogel, rich in glycine and proline, exhibits excellent ultraviolet absorption, water absorption, moisturizing, and free radical scavenging abilities (Liu et al., 2023). SE glue can effectively increase the ability of antioxidant and reduce ROS damage in L929 cells under UV irradiation (Liu et al., 2023). Some materials like chitosan can also be processed into drug delivery carriers to enhance the transdermal delivery efficiency of certain anti-photoaging drugs. For instance, carboxymethyl-modified chitosan/hyaluronic acid (CMC/HA, CMH) thermosensitive hydrogel can be used for the sustained transdermal delivery of exosomes (Figures 2A–C) (Wu et al., 2024). Its thermosensitive property allows it to quickly gel on the skin surface, delaying the release of liposomes. The release rate of ascorbyl glucoside (AA2G) over 24 h ranges from 33.92% to 49.35% (Wu et al., 2024). This system achieves the long-term release of bioactive ingredients and promotes the anti-photoaging effects of AA2G on the skin, reducing epidermal thickness, melanin deposition, and lipid oxidative damage while increasing collagen density (Figure 2D) (Wu et al., 2024).
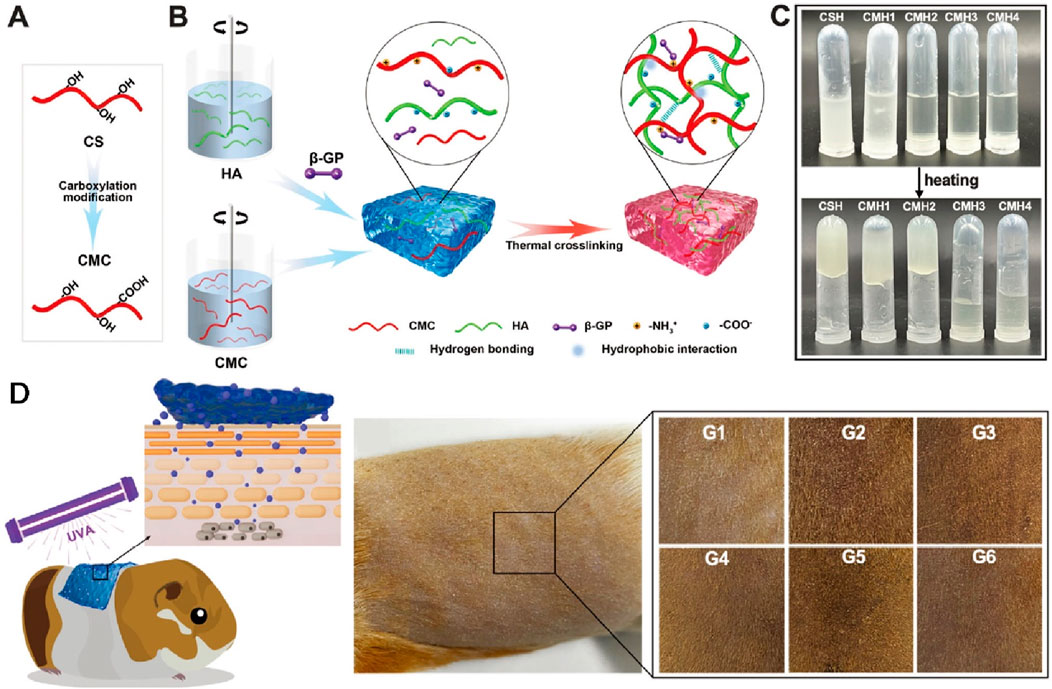
Figure 2. The preparation and skin photoaging therapy of thermosensitive hydrogels. (A) Chitosan carboxylation modification. (B) Schematic diagram of hydrogel synthesis. (C) Observation of the hydrogel. (D) Skin photoaging treatment experiment on Guinea pig. Reproduced with permission of Wu et al. (2024).
In addition to directly using marine extracts for the preparation of related hydrogels, some scholars have also engaged in biomimetic design, drawing inspiration from sea anemones. From the 1,262 adhesive-related proteins found in sea anemones, they identified the cysteine-rich thrombospondin-1 type I repeat-like (TSRL) protein, which is a biocompatible protein with excellent antioxidative properties (Wang et al., 2022). They prepared TSRL recombinant protein based on this protein sequence, and the produced TSRL recombinant protein can self-assemble into a gel (Figure 2C), exhibiting antioxidant effects in L929 cells, a kind of mouse fibroblast (Wang et al., 2022). Animal experiments have demonstrated that this hydrogel can effectively prevent photoaging of the skin (Wang et al., 2022). The above research demonstrates that the ocean is a rich resource treasure trove with many substances suitable for clinical therapeutic applications, addressing challenges in treating skin photoaging (Table 1). In practical life, these marine-derived biomaterials already have a wide range of applications, including clinical settings and skincare products. Therefore, some of their new application forms should also be quickly utilized in actual treatments. This is also due to the fact that their processing methods and side effects have been thoroughly explored and understood. However, within the plant kingdom on land, there are also numerous medicinal components worthy of exploration.
3.2 Hydrogels derived from plants resist photoaging
There are numerous natural active substances in plants, many of which possess antioxidant properties and even medicinal value. For instance, certain traditional Chinese medicinal herbs contain these properties. Due to the antioxidant effects found in plants, many plant-based ingredients are now incorporated into anti-aging skincare products. And there are plant extracts such as dried fruits of European blueberry (Vaccinium myrtillus) or blueberry (Vaccinium corymbosum). Extracts from these fruits, along with chitosan, are jointly used to prepare antioxidant hydrogels (Studzińska-Sroka et al., 2024). These hydrogels are employed for protecting the skin against UV damage and have been proven to exhibit excellent biocompatibility through relevant HaCaT cell experiments (Studzińska-Sroka et al., 2024). Additionally, other tests have confirmed their antioxidant, anti-hyaluronidase, and anti-tyrosinase activities (Studzińska-Sroka et al., 2024). Scientists have also achieved controlled release of anti-photoaging agents by preparing specialized hydrogels to enhance their efficacy against photodamage. They utilized hyaluronic acid to create a novel hydrogel crosslinked with boronate esters (de Oliveira et al., 2020). The release of the anti-photoaging agent dihydrocaffeic acid (DHCA) from this dynamic covalent hydrogel (HG) is controlled through the dynamic properties of complexes between phenylboronic acid and diol-containing molecules, as well as the pH dependence (de Oliveira et al., 2020). The hydrogel, formed through reversible drug complexation/decomplexation, extends the release of DHCA at pH 7.4, with the fastest release rate observed under acidic (skin) conditions (de Oliveira et al., 2020). Interestingly, incorporating DHCA into the network enhances its protective effect against UVB-induced L929 fibroblast death. Unlike extracting natural compounds from plants, experts have directly extracted exosome-like nanovesicles from Olea europaea leaves (OLELNVs) and enhanced them into a potent core biomaterial with high-dose effects that are skin-friendly and non-cytotoxic, inhibiting cell aging (Wang Z. et al., 2024b). These nanovesicles were incorporated into a cross-linked hyaluronic acid (HA) and tannic acid (TA) hydrogel with strong UV-absorbing properties, creating the OLELNVs@HA/TA hydrogel system as shown in Figure 3A. In vitro and in vivo experiments demonstrated that the OLELNVs@HA/TA hydrogel can effectively reduce UV-induced skin damage and promote skin repair and regeneration (Figure 3B) (Wang Z. et al., 2024b). Additionally, RNA-seq and clustering analysis of miR168a-5p predicted targets revealed significant downregulation of the NF-κB signaling pathway, mediating inflammatory aging responses (Wang Z. et al., 2024b). Overall, the OLELNVs@HA/TA hydrogel represents a novel dual-strategy approach for clinical application in treating photoaging.
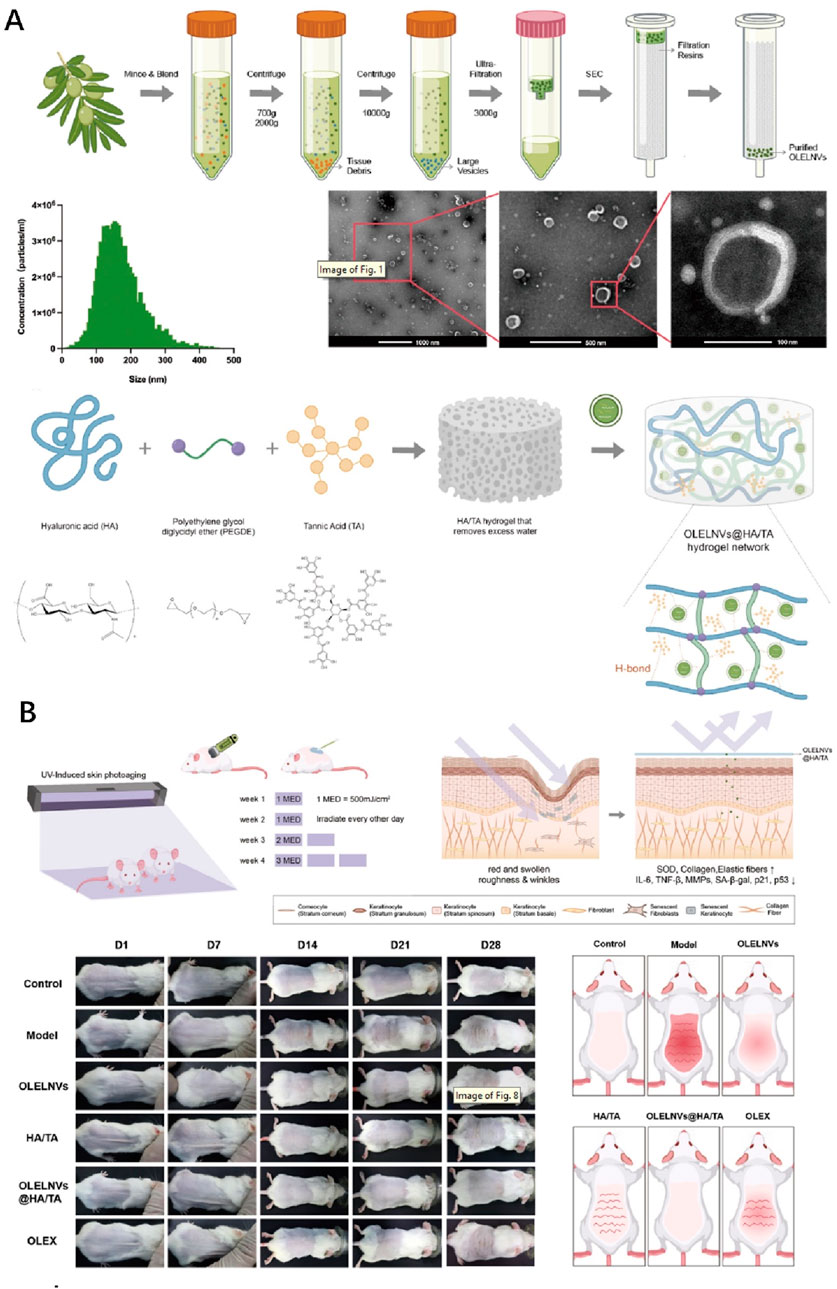
Figure 3. The preparation and skin photoaging therapy of OLELNVs@HA/TA hydrogel system. (A) Schematic diagram of exosome-like nanovesicles extraction and hydrogel synthesis. (B) Animal experiment on skin photoaging treatment using the OLELNVs@HA/TA hydrogel system. Reproduced with permission of Wang Z. et al. (2024).
In addition, there are other special plant extracts used in the preparation research of hydrogels for anti-photoaging, such as Lycium barbarum fruit polysaccharides, olive leaf extracts, and hesperetin. Researchers prepared a hydrogel named LBP from polysaccharides extracted from Lycium barbarum fruits (Neves et al., 2020). Their study revealed that this hydrogel, in combination with red light laser treatment, could inhibit UVR-induced skin thickening, decreasing the expression of c-Fos, c-Jun, MMP-1, -2, and -9, while simultaneously increasing the levels of collagen I, III, and FGF2. The combined treatment of PBM (Photobiomodulation) and LBP holds potential clinical applications in skin rejuvenation (Neves et al., 2020). Giglio et al. utilized olive leaf extracts (OLEs) combined with gellan gum and sodium alginate to prepare a hydrogel crosslinked with tartaric acid (Busto et al., 2023). This hydrogel demonstrated excellent antioxidant properties and effectively protected human dermal fibroblasts under UVA exposure (Busto et al., 2023). Furthermore, some researchers incorporated hesperetin into an AAMVPC hydrogel, achieving significant anti-UV effects by visibly reducing redness and skin aging caused by UV irradiation (de Araújo Andrade et al., 2022). The above hydrogels exhibit excellent anti-photoaging effects. However, directly incorporating relevant components into hydrogels may still face challenges such as uncontrolled release and poor transdermal efficacy.
Therefore, scientists have further explored combining other technologies, such as nanoparticles and liposomes, to enhance the controlled release of active ingredients within hydrogels and even improve the transdermal drug delivery effects. As early as 2015, a team undertook the nanocapsulation of rice bran oil, loading it into hydrogels to enhance its UV protective properties and more effectively reduce skin photoaging damage (Rigo et al., 2015). In this study, researchers initially prepared lipid-core nanocapsules containing rice bran oil with an average particle size of approximately 200 nm (Rigo et al., 2015). These nanocapsules were then incorporated into hydrogels made from acrylic acid polymers (Rigo et al., 2015). In animal experiments aimed at combating photoaging, the hydrogel containing nanocapsulated rice bran oil demonstrated a significant reduction in protein carbonylation levels (a biomarker of oxidative stress) and decrease in NF-κB nuclear translocation (a biomarker of pro-inflammatory and carcinogenic response). These in vivo experimental results substantiate the beneficial effects of nanocapsulation in enhancing the protective properties of rice bran oil against skin damage induced by UVB exposure (Rigo et al., 2015). As shown in Figure 4, another study discussed the preparation and evaluation of ligustrazine hydrochloride (TMPZ)-loaded liposome-hydrogel (TMPZ-LG) for its antioxidant properties and potential application in the treatment and prevention of ultraviolet (UV) radiation-induced skin damage (Liu et al., 2022). TMPZ-LG was prepared by the membrane dispersion method and natural swelling method using sodium carboxymethyl cellulose (2%, CMC-Na), achieving a transdermal release rate of 40% in vitro. The feasibility of TMPZ-LG in preventing and treating UV radiation-induced skin oxidation was demonstrated through DPPH and hydrogen peroxide (H2O2) scavenging experiments as well as malondialdehyde (MDA) and low-density lipoprotein (LDL) oxidation experiments (Liu et al., 2022). This indicates its potential as a novel antioxidant drug for transdermal delivery systems.
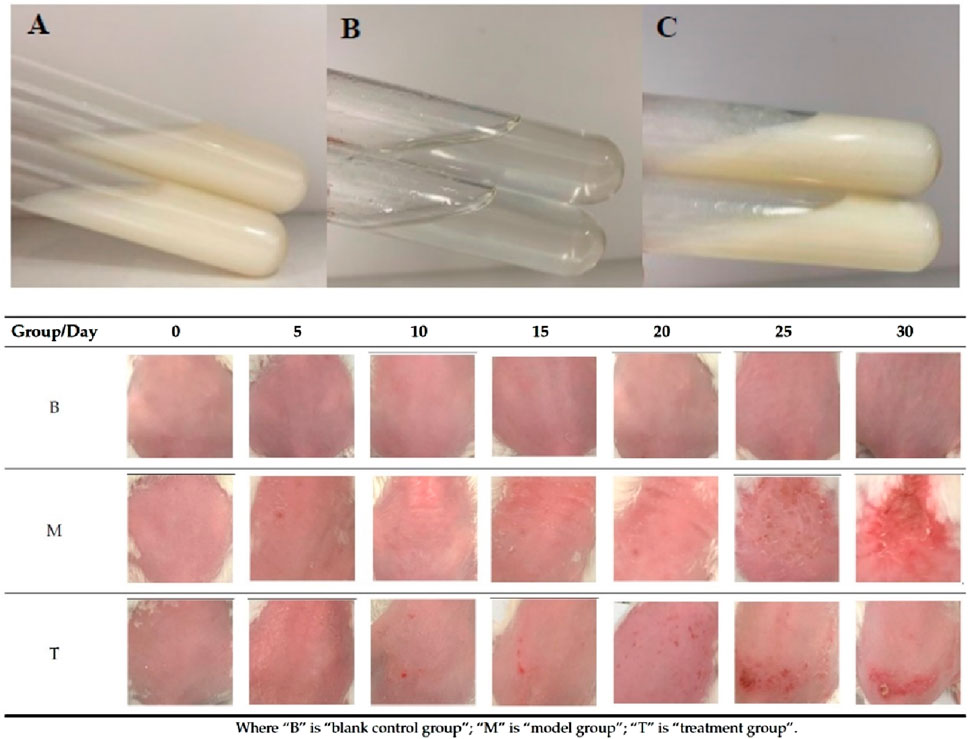
Figure 4. Hydrogel preparations (A) TMPZ-L; (B) TMPZ-G; (C) TMPZ-LG and the skin status of mice in each group after treatment 30 days. Reproduced with permission of Liu et al. (2022).
Oroxylin A (OA) is a flavonoid compound with excellent antioxidant activity and protective effects against photoaging induced by UV radiation. To enhance the transdermal delivery of OA, Nan Li and colleagues utilized nanostructured lipid carriers (NLC) to load OA, encapsulated it in a hydrogel composed of carbomer 940 as a gel matrix and hydroxypropyl methyl cellulose (HPMC) as a thickener (Zhu et al., 2022). In vitro studies revealed that OA-NLC exhibited superior therapeutic effects in a UVB radiation cell model compared to OA solution (OA-Sol) (Zhu et al., 2022). OA-Sol and OA-NLC were immobilized in a hydrogel matrix for topical application to the dorsal skin of mice. The results demonstrated that OA-NLC-gel, in comparison to OA-Sol-gel, exhibited significant antioxidant and anti-apoptotic activities, effectively protecting the skin from damage after UV radiation in mice (Zhu et al., 2022). The research findings indicate that OA-BLC-gel is a promising nanocarrier system for treating UV-induced skin oxidative stress damage (Zhu et al., 2022). However, further in-depth investigation is needed to study its long-term effects and potential side effects. To enhance the transdermal absorption of poorly soluble drugs for local use, researchers prepared nanocrystals of 18-β-glycyrrhetinic acid (NGAs) using high-pressure homogenization (Quan et al., 2023). These NGAs were combined with amphiphilic chitosan (ACS) to form ANGA composites through electrostatic adsorption (Quan et al., 2023). By incorporating glycerol, they prepared ANGA hydrogel. The ANGA hydrogel significantly improved the characteristics of photoaging in mouse skin under UV irradiation (Quan et al., 2023). It achieved this by inhibiting the abnormal expression of matrix metalloproteinase (MMP)-1 and MMP-3, thereby mitigating the damage caused by UV radiation to the collagen fiber structure (Quan et al., 2023). Therefore, with the advancement of nanotechnology, the transdermal delivery efficiency of certain ingredients has been enhanced, further promoting innovation in skincare product ingredients and the development of the industry.
From the above studies (Table 2), we can see that many plant ingredients have antioxidant and anti-inflammatory effects, which also makes them effective in treating skin damage caused by UV. And with the progress of nanotechnology, the transdermal effect of related drugs can also be further improved, the progress of these studies can also improve the progress of related skin care industry. Most plant-derived natural products are generally considered to have low physiological toxicity or side effects, making them relatively safe for use. However, extensive human trials are necessary to thoroughly investigate and validate their safety and efficacy for specific applications.
3.3 A hydrogel composed of a special natural product
A team from China conducted a study on skin photoaging therapy using the natural compound selenomethionine (Se-Met), which presents an innovative approach to address the challenges of skin aging by targeting epidermal stem cells (EpiSCs) and their role in wound healing (Sun et al., 2024). By integrating transcriptomics and untargeted metabolomics, the research identifies age-dependent changes in the Gpx gene family and arachidonic acid (AA) metabolic pathways, which contribute to increased susceptibility to ferroptosis—a form of cell death driven by lipid peroxidation. The study proposes Se-Met as a potential therapeutic agent to counteract these effects. Se-Met is shown to enhance the expression of GPX4, a key enzyme involved in protecting cells from lipid peroxidation and ferroptosis (Sun et al., 2024). Furthermore, Se-Met exhibits antioxidative properties and effective absorption of ultraviolet (UV) radiation, making it suitable for addressing multiple aspects of skin aging, including oxidative stress and UV damage.
To facilitate controlled release of Se-Met, the researchers develop a novel delivery system by covalently grafting Se-Met onto UV-responsive GelMA hydrogels using AC-PEG-NHS tethers. This Se-Met@GelMA hydrogel is demonstrated to accelerate wound healing in aging mice models by inhibiting lipid peroxidation and ferroptosis while enhancing GPX4 expression. Additionally, in a photoaging model, the hydrogel reduces inflammatory responses, promotes extracellular matrix remodeling, and mitigates ferroptosis induced by UV exposure (Sun et al., 2024).
The findings suggest that Se-Met@GelMA hydrogel holds promise for practical clinical applications in addressing various aspects of skin aging, including wound healing and protection against UV-induced damage (Sun et al., 2024). The controlled release mechanism provided by the hydrogel system ensures sustained and localized delivery of Se-Met, enhancing its therapeutic efficacy.
4 Hydrogels based on microneedle patches promote skin photoaging damage repair
Drug delivery systems have become a major focus of research in the biomedical field. Liposomes are a prominent area of study in drug delivery, and integrating liposomes into hydrogels enhances the development of more powerful multifunctional systems for achieving more efficient and sustained local drug delivery. Like the above-mentioned hydrogel system CMC/HA, CMH for the purpose of sustained transdermal delivery of AAG2 loaded liposomes (Wu et al., 2024), they serves as a multifunctional delivery system for sustained transdermal delivery. However, to achieve better delivery outcomes, some researchers have started using hydrogels to prepare more advanded delivery system—microneedle patches.
The research team led by Xiuli Wang investigated the utilization of roller microneedles (MN) to deliver adipose-derived stem cell-derived extracellular vesicles (ADSC-EVs) to the skin. ADSCs-EVs have been demonstrated to have immunomodulatory and anti-photoaging effects (Cao Z. et al., 2021b). The MN + EVs group exhibited the least wrinkles, the highest collagen density, and the most organized collagen fibers. Extensive research has confirmed the reparative effects of extracellular vesicles from adipose-derived stem cells (ADSCs-EVs) on skin photoaging. Some laboratories have focused on studying the extracellular matrix components of adipose tissue, such as purifying adipose collagen fragments (ACF) (Jin et al., 2022). In order to facilitate its clinical applications, researchers have designed a detachable ACF microneedle (ACF-MN) patch, where the needle tip and the patch backing can be separated (Jin et al., 2022). The research results indicate that ACF-MN exhibits excellent skin puncture performance and can release ACF components slowly. Moreover, this microneedle device carrying ACF shows therapeutic effects on skin photoaging in a mouse model (Jin et al., 2022). Its main anti-photoaging effect on the skin is that it prevents ROS accumulation and induces antioxidase production and induces neovascularization and reduction apoptosis (Jin et al., 2022).
Supplementing collagen in the skin is a reliable anti-aging method, but the effectiveness of some transdermal deliveries is hindered by the large molecular weight of collagen. Some researchers have attempted to address this issue through gene regulation, similar to the success of mRNA therapy, which largely depends on the safe, effective, and stable transformation of genetic material into functional proteins. Lee et al. combined extracellular vesicles (EVs) encapsulating mRNA encoding extracellular matrix α1 type-I collagen (COL1A1) with microneedle patches (You et al., 2023). Their EVs were primarily produced through cellular nanoporation of human dermal fibroblasts (You et al., 2023). Their results demonstrated that the microneedle patches could induce the formation of collagen-protein grafts and reduce wrinkle formation in the collagen-depleted dermal tissue of mice with photoaged skin (You et al., 2023). Injecting COL1A1 mRNA enclosed in extracellular vesicles (EVs) into the skin shows potential as a protein replacement therapy for treating photoaged skin (You et al., 2023). Currently, researchers are also incorporating certain cytokines into microneedle patch systems. For instance, Ke Cheng et al. loaded FGF-2 and FGF-21 into microneedle patches made from hyaluronic acid (HA) hydrogel for the treatment of skin photoaging. Significant improvement was observed after 4 weeks of treatment (Yang et al., 2023).
Microneedle patches, in addition to delivering common bioactive substances, can also be used to administer microalgae for treating skin photoaging (Wang Z. et al., 2023b). This is based on the principle previously demonstrated that dissolved oxygen could reverse photoaged skin (Shin et al., 2019). Researchers have developed microneedle patch systems carrying active microalgae (Figure 5), the patch use microalgae to generate reactive oxygen species under light, which helps reverse skin damage by reducing inflammation, promoting collagen regeneration, and diminishing wrinkles. This approach offers a novel method for skin oxygenation and photoaging reversal, laying a theoretical foundation for future clinical applications (Wang Z. et al., 2023b).
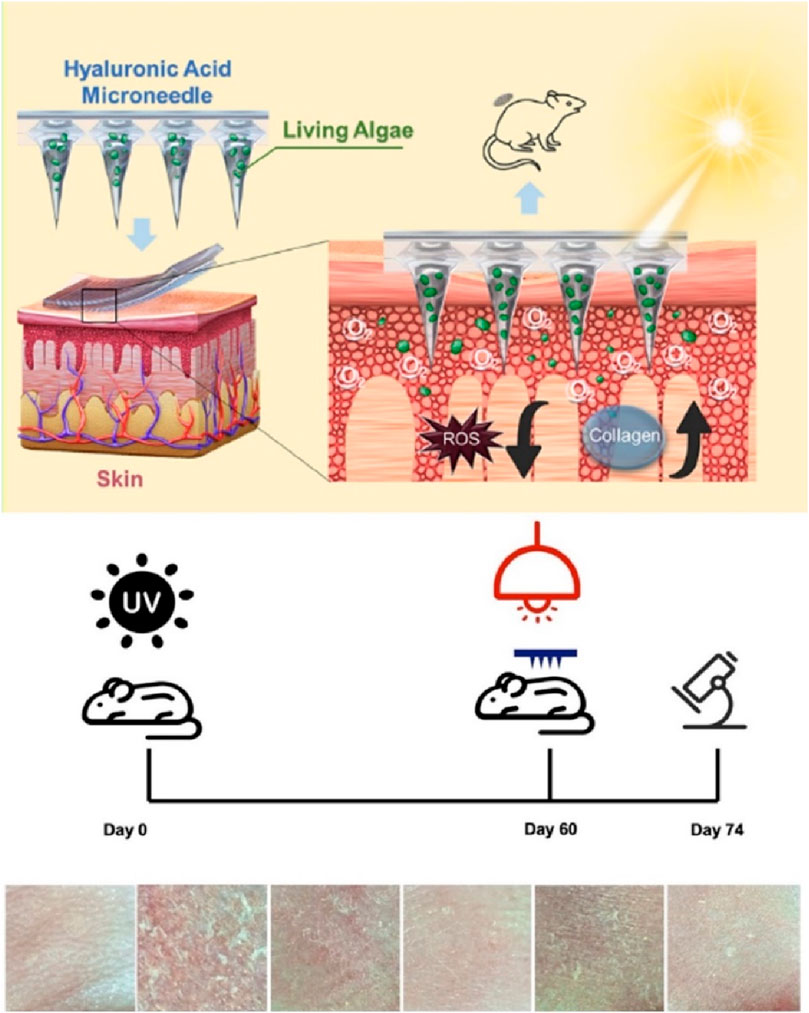
Figure 5. Living algae loading HA microneedle treat skin photoaging. Reproduced with permission of Wang Z. et al. (2023).
We have made a summary of the microneedle system that will be applied in skin photoaging treatment, as shown in Table 3. In addition to the different substances contained in each microneedle, their main solutions to the problems of skin photoaging are mainly aimed at solving the skin inflammation, oxidative stress and cell aging caused by UV. Moreover, HA is the most used polymer hydrogel material for MN production, which has been widely used in medical and cosmetic fields. Its biocompatibility has been extensively validated in clinical settings, proving to be very safe. Therefore, when considering the application of such microneedle patches, the primary focus should be on the safety and efficacy evaluation of the incorporated drugs.
5 TiO2-based materials for UV resistance
TiO2 serves as an inorganic sunscreen and photocatalyst to protect the human body from environmental pollutants. Researchers, led by Jia-You Fang, incorporated TiO2 into mesoporous silica, where this material effectively filters environmental pollutants, shielding the skin from environmental harm (Lin et al., 2021). Additionally, the material exhibits excellent UV-blocking properties, and the inclusion of avobenzone further enhances its UV-blocking effectiveness (Lin et al., 2021). The combination of avobenzone and TiO2 in SBA-15 (TiO2/SBA) significantly alleviated skin cell death and neutrophil recruitment in photoaged mouse skin compared to the application of SBA-15 alone (Lin et al., 2021). The researchers also formulated avobenzone-loaded TiO2/SBA into a Carbopol 940 hydrogel, creating a sunscreen gel applicable to the skin. avobenzone-loaded TiO2/SBA-S hydrogel demonstrated greater improvement in skin barrier recovery and proinflammatory mediator mitigation compared to SBA-S hydrogel (without TiO2) (Alalaiwe et al., 2023). After avobenzone-loaded TiO2/SBA-S treatment, cytokines/chemokines in photoaged skin were reduced by two to three times compared to the untreated control group (Alalaiwe et al., 2023). The data suggests that mesoporous formulations with low porosity and specific surface area exhibit effective adsorption of environmental pollutants and UVA protective properties (Alalaiwe et al., 2023). This study provides valuable insights into the development of versatile mesoporous silica for safeguarding the skin from environmental stress.
6 Discussion and the future perspectives
As the quality of life in developed regions continues to improve year by year, people’s expectations for their own lives are also increasing, especially among some young women who place great emphasis on anti-aging. Ultraviolet radiation is a major factor causing skin aging, and beauty-conscious women always take measures to protect themselves from UV exposure during the summer. Therefore, there is an urgent need for the development of an effective, skin-applicable anti-aging gel product. In addition to providing basic protection against ultraviolet rays, it is preferable for these products to have a certain effect in promoting skin regeneration and repair, aiming to reduce the occurrence of wrinkles and signs of aging.
We discussed various hydrogels for the treatment of skin photoaging, including those containing components derived from Marine organisms, including components derived from plants, and cytokines and nucleic acids derived from some human stem cells. These studies have shown that they can repair the damage caused by photoaging of the skin, but these effects need to be further demonstrated through clinical trials. When hydrogels are used for treating photoaged skin, biocompatibility and non-toxicity are crucial considerations. Although non-toxicity is a key requirement, it alone is not sufficient to ensure the safety and efficacy of hydrogels. It is also necessary to consider degradability, mechanical properties, absorption and release performance, and moisturizing effects. If the hydrogel is degradable, its degradation products must also be non-toxic and capable of being safely metabolized or excreted by the body. The hydrogel should have appropriate mechanical properties to ensure it remains on the skin surface for a sufficient period. Additionally, hydrogels should have good drug loading and release characteristics to ensure that their active ingredients can be continuously and stably released into the skin. Furthermore, hydrogels usually should have moisturizing effects to help maintain the skin’s moisture balance. However, most of the current research on hydrogels primarily demonstrates their efficacy in treating photoaged skin, while other aspects have been less frequently tested, with only a few studies addressing them. Among these hydrogels, individuals prefer hydrogels from bioactive substances containing human self-cells, which do not have to worry about xenograft rejection and should have better effects, and other sources of hydrogels can play a greater role in the field of cosmetics.
From the current research progress, the application of natural product extracts is extensive, encompassing substances from natural sources such as the ocean and plants. On one hand, they serve as high-quality bioactive substances with specific anti-photoaging effects; on the other hand, during the development and industrialization of related products, they can be rapidly deployed for practical applications, providing consumers with efficient anti-photoaging products. Therefore, efforts should be intensified in the development of relevant components, allocating more resources to related research, and driving the development of associated industries. In addition, the functional effectiveness and side effects of some new ingredients must undergo clinical trials. As for research outcomes involving stem cells, extracellular vesicles, or even genetic engineering approaches in anti-photoaging, there are currently challenges in industrialization due to ethical and technological maturity issues. Nevertheless, in the near future, they still hold significant market prospects, as related research continues to mature, leading to more outstanding anti-photoaging effects. In summary, in the field of combating skin photoaging, it is anticipated that numerous innovations will emerge, further transforming skincare approaches and market dynamics.
Author contributions
LC: Conceptualization, Writing–original draft, Writing–review and editing. XQ: Writing–review and editing. JM: Writing–review and editing. ZZ: Writing–review and editing. MY: Writing–review and editing. DY: Writing–review and editing.
Funding
The author(s) declare that no financial support was received for the research, authorship, and/or publication of this article.
Conflict of interest
The authors declare that the research was conducted in the absence of any commercial or financial relationships that could be construed as a potential conflict of interest.
Publisher’s note
All claims expressed in this article are solely those of the authors and do not necessarily represent those of their affiliated organizations, or those of the publisher, the editors and the reviewers. Any product that may be evaluated in this article, or claim that may be made by its manufacturer, is not guaranteed or endorsed by the publisher.
References
Alalaiwe, A., Lin, Y. C., Lin, C. F., Huang, C. C., Wang, P. W., and Fang, J. Y. (2023). TiO(2)-embedded mesoporous silica with lower porosity is beneficial to adsorb the pollutants and retard UV filter absorption: a possible application for outdoor skin protection. Eur. J. Pharm. Sci. 180, 106344. doi:10.1016/j.ejps.2022.106344
Amnuaykan, P., Juntrapirom, S., Kanjanakawinkul, W., and Chaiyana, W. (2024). Enhanced antioxidant, anti-aging, anti-tyrosinase, and anti-inflammatory properties of vanda coerulea griff. Ex lindl. Protocorm through elicitations with chitosan. Plants (Basel) 13 (13), 1770. doi:10.3390/plants13131770
Battie, C., Jitsukawa, S., Bernerd, F., Del Bino, S., Marionnet, C., and Verschoore, M. (2014). New insights in photoaging, UVA induced damage and skin types. Exp. Dermatol 23 (Suppl. 1), 7–12. doi:10.1111/exd.12388
Belkaid, Y., and Segre, J. A. (2014). Dialogue between skin microbiota and immunity. Science 346 (6212), 954–959. doi:10.1126/science.1260144
Brown, T. E., and Anseth, K. S. (2017). Spatiotemporal hydrogel biomaterials for regenerative medicine. Chem. Soc. Rev. 46 (21), 6532–6552. doi:10.1039/c7cs00445a
Burdick, J. A., and Prestwich, G. D. (2011). Hyaluronic acid hydrogels for biomedical applications. Adv. Mater 23 (12), H41–H56. doi:10.1002/adma.201003963
Busto, F., Licini, C., Luccarini, A., Damiani, E., Mattioli-Belmonte, M., Cometa, S., et al. (2023). Oleuropein-rich gellan gum/alginate films as innovative treatments against photo-induced skin aging. Molecules 28 (11), 4352. doi:10.3390/molecules28114352
Cao, C., Xiao, Z., Wu, Y., and Ge, C. (2020). Diet and skin aging-from the perspective of food nutrition. Nutrients 12 (3), 870. doi:10.3390/nu12030870
Cao, H., Duan, L., Zhang, Y., Cao, J., and Zhang, K. (2021a). Current hydrogel advances in physicochemical and biological response-driven biomedical application diversity. Signal Transduct. Target Ther. 6 (1), 426. doi:10.1038/s41392-021-00830-x
Cao, Z., Jin, S., Wang, P., He, Q., Yang, Y., Gao, Z., et al. (2021b). Microneedle based adipose derived stem cells-derived extracellular vesicles therapy ameliorates UV-induced photoaging in SKH-1 mice. J. Biomed. Mater Res. A 109 (10), 1849–1857. doi:10.1002/jbm.a.37177
Cassano, R., Curcio, F., Sole, R., and Trombino, S. (2023). Transdermal delivery of phloretin by gallic acid microparticles. Gels 9 (3), 226. doi:10.3390/gels9030226
Chaudhary, M., Khan, A., and Gupta, M. (2020). Skin ageing: pathophysiology and current market treatment approaches. Curr. Aging Sci. 13 (1), 22–30. doi:10.2174/1567205016666190809161115
Chawla, K., Yu, T., Liao, S. W., and Guan, Z. (2011). Biodegradable and biocompatible synthetic saccharide-Peptide hydrogels for three-dimensional stem cell culture. Biomacromolecules 12 (3), 560–567. doi:10.1021/bm100980w
Chen, Y., Wang, X., Tao, S., Wang, Q., Ma, P. Q., Li, Z. B., et al. (2023). Research advances in smart responsive-hydrogel dressings with potential clinical diabetic wound healing properties. Mil. Med. Res. 10 (1), 37. doi:10.1186/s40779-023-00473-9
Chyzy, A., and Plonska-Brzezinska, M. E. (2020). Hydrogel properties and their impact on regenerative medicine and tissue engineering. Molecules 25 (24), 5795. doi:10.3390/molecules25245795
Cortes, H., Caballero-Florán, I. H., Mendoza-Muñoz, N., Córdova-Villanueva, E. N., Escutia-Guadarrama, L., Figueroa-González, G., et al. (2020). Hyaluronic acid in wound dressings. Cell. Mol. Biol. (Noisy-le-grand) 66 (4), 191–198. doi:10.14715/cmb/2020.66.4.23
de Araújo Andrade, T., Heimfarth, L., dos Santos, D. M., dos Santos, M. R. V., de Albuquerque-Júnior, R. L. C., dos Santos-Neto, A. G., et al. (2022). Hesperetin-based hydrogels protect the skin against UV radiation-induced damage. AAPS PharmSciTech 23 (6), 170. doi:10.1208/s12249-022-02323-8
de Oliveira, M. M., Nakamura, C. V., and Auzély-Velty, R. (2020). Boronate-ester crosslinked hyaluronic acid hydrogels for dihydrocaffeic acid delivery and fibroblasts protection against UVB irradiation. Carbohydr. Polym. 247, 116845. doi:10.1016/j.carbpol.2020.116845
Du, J., Ma, Q., Wang, B., Sun, L., and Liu, L. (2023). Hydrogel fibers for wearable sensors and soft actuators. iScience 26 (6), 106796. doi:10.1016/j.isci.2023.106796
Fisher, G. J., Kang, S., Varani, J., Bata-Csorgo, Z., Wan, Y., Datta, S., et al. (2002). Mechanisms of photoaging and chronological skin aging. Arch. Dermatol 138 (11), 1462–1470. doi:10.1001/archderm.138.11.1462
Fitsiou, E., Pulido, T., Campisi, J., Alimirah, F., and Demaria, M. (2021). Cellular senescence and the senescence-associated secretory phenotype as drivers of skin photoaging. J. Investig. Dermatol 141 (4s), 1119–1126. doi:10.1016/j.jid.2020.09.031
Francesko, A., Petkova, P., and Tzanov, T. (2018). Hydrogel dressings for advanced wound management. Curr. Med. Chem. 25 (41), 5782–5797. doi:10.2174/0929867324666170920161246
Fu, H., Zhang, D., Zeng, J., Fu, Q., Chen, Z., Sun, X., et al. (2023). Application of 3D-printed tissue-engineered skin substitute using innovative biomaterial loaded with human adipose-derived stem cells in wound healing. Int. J. Bioprint 9 (2), 674. doi:10.18063/ijb.v9i2.674
Gaspar, L. R., and Campos, P. M. (2007). Photostability and efficacy studies of topical formulations containing UV-filters combination and vitamins A, C and E. Int. J. Pharm. 343 (1-2), 181–189. doi:10.1016/j.ijpharm.2007.05.048
Greul, A. K., Grundmann, J. U., Heinrich, F., Pfitzner, I., Bernhardt, J., Ambach, A., et al. (2002). Photoprotection of UV-irradiated human skin: an antioxidative combination of vitamins E and C, carotenoids, selenium and proanthocyanidins. Skin. Pharmacol. Appl. Skin. Physiol. 15 (5), 307–315. doi:10.1159/000064534
Gu, Y., Han, J., Jiang, C., and Zhang, Y. (2020). Biomarkers, oxidative stress and autophagy in skin aging. Ageing Res. Rev. 59, 101036. doi:10.1016/j.arr.2020.101036
Guo, K., Zheng, L., Zeng, X., Huang, G., Meng, L., and Yin, Y. (2024). Compound collagen peptide powder improves skin photoaging by reducing oxidative stress and activating TGF-β1/Smad pathway. Photochem Photobiol. doi:10.1111/php.13940
Hamilton, M. M., and Kao, R. (2020). Recognizing and managing complications in laser resurfacing, chemical peels, and dermabrasion. Facial Plast. Surg. Clin. North Am. 28 (4), 493–501. doi:10.1016/j.fsc.2020.06.008
Ho, T. C., Chang, C. C., Chan, H. P., Chung, T. W., Shu, C. W., Chuang, K. P., et al. (2022). Hydrogels: properties and applications in biomedicine. Molecules 27 (9), 2902. doi:10.3390/molecules27092902
Hu, X., Xia, Z., and Cai, K. (2022). Recent advances in 3D hydrogel culture systems for mesenchymal stem cell-based therapy and cell behavior regulation. J. Mater Chem. B 10 (10), 1486–1507. doi:10.1039/d1tb02537f
Huang, X., Chang, Q., Gao, J. h., and Lu, F. (2023). Sustained release microneedles: materials and applications in facial rejuvenation. Tissue Eng. Part B Rev. 29 (3), 190–202. doi:10.1089/ten.teb.2022.0131
Hubbard, B. A., Unger, J. G., and Rohrich, R. J. (2014). Reversal of skin aging with topical retinoids. Plast. Reconstr. Surg. 133 (4), 481e–490e. doi:10.1097/prs.0000000000000043
Jin, X., Zhang, X., Li, Y., Xu, M., Yao, Y., Wu, Z., et al. (2022). Long-acting microneedle patch loaded with adipose collagen fragment for preventing the skin photoaging in mice. Biomater. Adv. 135, 212744. doi:10.1016/j.bioadv.2022.212744
Khayambashi, P., Iyer, J., Pillai, S., Upadhyay, A., Zhang, Y., and Tran, S. (2021). Hydrogel encapsulation of mesenchymal stem cells and their derived exosomes for tissue engineering. Int. J. Mol. Sci. 22 (2), 684. doi:10.3390/ijms22020684
Kim, D. J., Iwasaki, A., Chien, A. L., and Kang, S. (2022). UVB-mediated DNA damage induces matrix metalloproteinases to promote photoaging in an AhR- and SP1-dependent manner. JCI Insight 7 (9). doi:10.1172/jci.insight.156344
Kim, N., Lee, H., Han, G., Kang, M., Park, S., Kim, D. E., et al. (2023). 3D-Printed functional hydrogel by DNA-induced biomineralization for accelerated diabetic wound healing. Adv. Sci. (Weinh) 10 (17), e2300816. doi:10.1002/advs.202300816
Letsiou, S. (2021). Tracing skin aging process: a mini-review of in vitro approaches. Biogerontology 22 (3), 261–272. doi:10.1007/s10522-021-09916-z
Lewicka, K., Smola-Dmochowska, A., Dobrzyński, P., Śmigiel-Gac, N., Jelonek, K., Musiał-Kulik, M., et al. (2024). Microspheres based on blends of chitosan derivatives with carrageenan as vitamin carriers in cosmeceuticals. Polym. (Basel) 16 (13), 1815. doi:10.3390/polym16131815
Li, P., Fu, L., Liao, Z., Peng, Y., Ning, C., Gao, C., et al. (2021). Chitosan hydrogel/3D-printed poly(ε-caprolactone) hybrid scaffold containing synovial mesenchymal stem cells for cartilage regeneration based on tetrahedral framework nucleic acid recruitment. Biomaterials 278, 121131. doi:10.1016/j.biomaterials.2021.121131
Li, S., Sun, J., Yang, J., Yang, Y., Ding, H., Yu, B., et al. (2023). Gelatin methacryloyl (GelMA) loaded with concentrated hypoxic pretreated adipose-derived mesenchymal stem cells(ADSCs) conditioned medium promotes wound healing and vascular regeneration in aged skin. Biomater. Res. 27 (1), 11. doi:10.1186/s40824-023-00352-3
Li, Y., Zhao, L., Li, S., Ruan, D., Xiong, L., Tang, J., et al. (2024). Skin-derived precursor conditioned medium alleviated photoaging via early activation of TGF-β/Smad signaling pathway by thrombospondin1: in vitro and in vivo studies. J. Photochem Photobiol. B 253, 112873. doi:10.1016/j.jphotobiol.2024.112873
Lim, S. H., Kim, S. M., Lee, Y. W., Ahn, K. J., and Choe, Y. B. (2008). Change of biophysical properties of the skin caused by ultraviolet radiation-induced photodamage in Koreans. Skin. Res. Technol. 14 (1), 93–102. doi:10.1111/j.1600-0846.2007.00272.x
Lin, Y. C., Fang, Y. P., Hung, C. F., Yu, H. P., Alalaiwe, A., Wu, Z. Y., et al. (2021). Multifunctional TiO(2)/SBA-15 mesoporous silica hybrids loaded with organic sunscreens for skin application: the role in photoprotection and pollutant adsorption with reduced sunscreen permeation. Colloids Surf. B Biointerfaces 202, 111658. doi:10.1016/j.colsurfb.2021.111658
Liu, C., Xia, Y., Li, Y., Cheng, Y., Xia, H., Wang, Y., et al. (2022). Ligustrazine as an extract from medicinal and edible plant chuanxiong encapsulated in liposome-hydrogel exerting antioxidant effect on preventing skin photoaging. Polym. (Basel) 14 (21), 4778. doi:10.3390/polym14214778
Liu, Y., Qin, D., Wang, H., Zhu, Y., Bi, S., et al. (2023). Effect and mechanism of fish scale extract natural hydrogel on skin protection and cell damage repair after UV irradiation. Colloids Surf. B Biointerfaces 225, 113281. doi:10.1016/j.colsurfb.2023.113281
Luo, Y., Li, J., Ding, Q., Wang, H., Liu, C., and Wu, J. (2023). Functionalized hydrogel-based wearable gas and humidity sensors. Nanomicro Lett. 15 (1), 136. doi:10.1007/s40820-023-01109-2
Mast, B. A., Flood, L. C., Haynes, J. H., Depalma, R. L., Cohen, I. K., Diegelmann, R. F., et al. (1991). Hyaluronic acid is a major component of the matrix of fetal rabbit skin and wounds: implications for healing by regeneration. Matrix 11 (1), 63–68. doi:10.1016/s0934-8832(11)80228-3
Mukherjee, S., Date, A., Patravale, V., Korting, H. C., Roeder, A., and Weindl, G. (2006). Retinoids in the treatment of skin aging: an overview of clinical efficacy and safety. Clin. Interv. Aging 1 (4), 327–348. doi:10.2147/ciia.2006.1.4.327
Nakanishi, M., Niida, H., Murakami, H., and Shimada, M. (2009). DNA damage responses in skin biology--implications in tumor prevention and aging acceleration. J. Dermatol Sci. 56 (2), 76–81. doi:10.1016/j.jdermsci.2009.09.001
Neves, L. M. G., Parizotto, N. A., Tim, C. R., Floriano, E. M., Lopez, R. F. V., Venâncio, T., et al. (2020). Polysaccharide-rich hydrogel formulation combined with photobiomodulation repairs UV-induced photodamage in mice skin. Wound Repair Regen. 28 (5), 645–655. doi:10.1111/wrr.12826
Ortonne, J. P. (1990). The effects of ultraviolet exposure on skin melanin pigmentation. J. Int. Med. Res. 18 (Suppl. 3), 8C–17c.
Papaccio, F., D′Arino, A., Caputo, S., and Bellei, B. (2022). Focus on the contribution of oxidative stress in skin aging. Antioxidants (Basel) 11 (6), 1121. doi:10.3390/antiox11061121
Poon, F., Kang, S., and Chien, A. L. (2015). Mechanisms and treatments of photoaging. Photodermatol. Photoimmunol. Photomed. 31 (2), 65–74. doi:10.1111/phpp.12145
Pourang, A., Tisack, A., Ezekwe, N., Torres, A. E., Kohli, I., Hamzavi, I. H., et al. (2022). Effects of visible light on mechanisms of skin photoaging. Photodermatol. Photoimmunol. Photomed. 38 (3), 191–196. doi:10.1111/phpp.12736
Quan, W., Kong, S., Li, S., Ouyang, Q., Lu, S., Guo, J., et al. (2023). Anti-photoaging effects of nanocomposites of amphiphilic chitosan/18β-glycyrrhetinic acid. Molecules 28 (11), 4362. doi:10.3390/molecules28114362
Rahman, A., Rehmani, R., Pirvu, D. G., Huang, S. M., Puri, S., and Arcos, M. (2024). Unlocking the therapeutic potential of marine collagen: a scientific exploration for delaying skin aging. Mar. Drugs 22 (4), 159. doi:10.3390/md22040159
Rahmani, P., and Shojaei, A. (2021). A review on the features, performance and potential applications of hydrogel-based wearable strain/pressure sensors. Adv. Colloid Interface Sci. 298, 102553. doi:10.1016/j.cis.2021.102553
Riahi, R. R., Bush, A. E., and Cohen, P. R. (2016). Topical retinoids: therapeutic mechanisms in the treatment of photodamaged skin. Am. J. Clin. Dermatol 17 (3), 265–276. doi:10.1007/s40257-016-0185-5
Rigo, L. A., da Silva, C. R., de Oliveira, S. M., Cabreira, T. N., de Bona da Silva, C., Ferreira, J., et al. (2015). Nanoencapsulation of rice bran oil increases its protective effects against UVB radiation-induced skin injury in mice. Eur. J. Pharm. Biopharm. 93, 11–17. doi:10.1016/j.ejpb.2015.03.020
Rittié, L., and Fisher, G. J. (2015). Natural and sun-induced aging of human skin. Cold Spring Harb. Perspect. Med. 5 (1), a015370. doi:10.1101/cshperspect.a015370
Sales, A. F. S., Pandolfo, I. L., de Almeida Cruz, M., Parisi, J. R., Garcia, L. A., Martignago, C. C. S., et al. (2022). Intense Pulsed Light on skin rejuvenation: a systematic review. Arch. Dermatol Res. 314 (9), 823–838. doi:10.1007/s00403-021-02283-2
Schuch, A. P., Moreno, N. C., Schuch, N. J., Menck, C. F. M., and Garcia, C. C. M. (2017). Sunlight damage to cellular DNA: focus on oxidatively generated lesions. Free Radic. Biol. Med. 107, 110–124. doi:10.1016/j.freeradbiomed.2017.01.029
Sharad, J. (2013). Glycolic acid peel therapy - a current review. Clin. Cosmet. Investig. Dermatol 6, 281–288. doi:10.2147/ccid.s34029
Shin, J. W., Kwon, S. H., Choi, J. Y., Na, J. I., Huh, C. H., Choi, H. R., et al. (2019). Molecular mechanisms of dermal aging and antiaging approaches. Int. J. Mol. Sci. 20 (9), 2126. doi:10.3390/ijms20092126
Sreedhar, A., Aguilera-Aguirre, L., and Singh, K. K. (2020). Mitochondria in skin health, aging, and disease. Cell. Death Dis. 11 (6), 444. doi:10.1038/s41419-020-2649-z
Steenvoorden, D. P., and Beijersbergen van Henegouwen, G. (1999). Protection against UV-induced systemic immunosuppression in mice by a single topical application of the antioxidant vitamins C and E. Int. J. Radiat. Biol. 75 (6), 747–755. doi:10.1080/095530099140096
Studzińska-Sroka, E., Paczkowska-Walendowska, M., Erdem, C., Paluszczak, J., Kleszcz, R., Hoszman-Kulisz, M., et al. (2024). Anti-aging properties of chitosan-based hydrogels rich in bilberry fruit extract. Antioxidants (Basel) 13 (1), 105. doi:10.3390/antiox13010105
Sun, J., Xie, X., Song, Y., Sun, T., Liu, X., Yuan, H., et al. (2024). Selenomethionine in gelatin methacryloyl hydrogels: modulating ferroptosis to attenuate skin aging. Bioact. Mater 35, 495–516. doi:10.1016/j.bioactmat.2024.02.013
Tan, C. Y. R., Chin, T., Morenc, M., Ho, C. Y., Rovito, H. A., et al. (2022). Nicotinamide prevents UVB- and oxidative stress‒induced photoaging in human primary keratinocytes. J. Investig. Dermatol 142 (6), 1670–1681.e12. doi:10.1016/j.jid.2021.10.021
Tran, J. T., Diaz, M. J., Rodriguez, D., Kleinberg, G., Aflatooni, S., Palreddy, S., et al. (2023). Evidence-based utility of adjunct antioxidant supplementation for the prevention and treatment of dermatologic diseases: a comprehensive systematic review. Antioxidants (Basel) 12 (8), 1503. doi:10.3390/antiox12081503
Vijayavenkataraman, S., Yan, W. C., Lu, W. F., Wang, C. H., and Fuh, J. Y. H. (2018). 3D bioprinting of tissues and organs for regenerative medicine. Adv. Drug Deliv. Rev. 132, 296–332. doi:10.1016/j.addr.2018.07.004
Wang, L., Zhang, X., Xu, P., Yan, J., Zhang, Y., Su, H., et al. (2022). Exploration of sea anemone-inspired high-performance biomaterials with enhanced antioxidant activity. Bioact. Mater 10, 504–514. doi:10.1016/j.bioactmat.2021.08.021
Wang, M., Charareh, P., Lei, X., and Zhong, J. L. (2019). Autophagy: multiple mechanisms to protect skin from ultraviolet radiation-driven photoaging. Oxid. Med. Cell. Longev. 2019, 1–14. doi:10.1155/2019/8135985
Wang, X., Song, R., Johnson, M., He, Z., Milne, C., et al. (2021). An injectable chitosan-based self-healable hydrogel system as an antibacterial wound dressing. Mater. (Basel) 14 (20), 5956. doi:10.3390/ma14205956
Wang, Y., Lu, Z., Huang, Y., Jia, W., Wang, W., Zhang, X., et al. (2023a). Smart nanostructures for targeted oxygen-producing photodynamic therapy of skin photoaging and potential mechanism. Nanomedicine (Lond) 18 (3), 217–231. doi:10.2217/nnm-2022-0170
Wang, Y., Zhang, Y., Yang, Y. P., Jin, M. Y., Huang, S., Zhuang, Z. M., et al. (2024a). Versatile dopamine-functionalized hyaluronic acid-recombinant human collagen hydrogel promoting diabetic wound healing via inflammation control and vascularization tissue regeneration. Bioact. Mater 35, 330–345. doi:10.1016/j.bioactmat.2024.02.010
Wang, Z., Kwong, C. H. T., Zhao, H., Ding, Y. F., Gao, C., Zhang, D., et al. (2023b). Microalgae microneedle supplies oxygen for antiphotoaging treatment. ACS Appl. Bio Mater 6 (9), 3463–3471. doi:10.1021/acsabm.3c00192
Wang, Z., Yuan, J., Xu, Y., Shi, N., Lin, L., Wang, R., et al. (2024b). Olea europaea leaf exosome-like nanovesicles encapsulated in a hyaluronic acid/tannic acid hydrogel dressing with dual “defense-repair” effects for treating skin photoaging. Mater Today Bio 26, 101103. doi:10.1016/j.mtbio.2024.101103
Wu, S., Liu, G., Shao, P., Lin, X., Yu, J., Chen, H., et al. (2024). Transdermal sustained release properties and anti-photoaging efficacy of liposome-thermosensitive hydrogel system. Adv. Healthc. Mater 13 (2), e2301933. doi:10.1002/adhm.202301933
Wu, Y., Geng, L., Zhang, J., Wu, N., Yang, Y., Zhang, Q., et al. (2023). Preparation of multifunctional seaweed polysaccharides derivatives composite hydrogel to protect ultraviolet B-induced photoaging in vitro and in vivo. Macromol. Biosci. 24, e2300292. doi:10.1002/mabi.202300292
Xia, J., Liu, Z. Y., Han, Z. Y., Yuan, Y., Shao, Y., Feng, X. Q., et al. (2022). Regulation of cell attachment, spreading, and migration by hydrogel substrates with independently tunable mesh size. Acta Biomater. 141, 178–189. doi:10.1016/j.actbio.2022.01.025
Xiong, Y., Chen, L., Liu, P., Yu, T., Lin, C., Yan, C., et al. (2022). All-in-One: multifunctional hydrogel accelerates oxidative diabetic wound healing through timed-release of exosome and fibroblast growth factor. Small 18 (1), e2104229. doi:10.1002/smll.202104229
Xu, Q., et al. (2021). Collagen- and hyaluronic acid-based hydrogels and their biomedical applications. Mater Sci. Eng. R. Rep., 146.
Yan, T., Huang, L., Yan, Y., Zhong, Y., Xie, H., and Wang, X. (2023). Bone marrow mesenchymal stem cell-derived exosome miR-29b-3p alleviates UV irradiation-induced photoaging in skin fibroblast. Photodermatol. Photoimmunol. Photomed. 39 (3), 235–245. doi:10.1111/phpp.12827
Yang, G., Hu, S., Jiang, H., and Cheng, K. (2023). Peelable microneedle patches deliver fibroblast growth factors to repair skin photoaging damage. Nanotheranostics 7 (4), 380–392. doi:10.7150/ntno.79187
You, Y., Tian, Y., Yang, Z., Shi, J., Kwak, K. J., Tong, Y., et al. (2023). Intradermally delivered mRNA-encapsulating extracellular vesicles for collagen-replacement therapy. Nat. Biomed. Eng. 7, 887–900. doi:10.1038/s41551-022-00989-w
Zhang, K., Yu, L., Li, F. R., Li, X., Wang, Z., Zou, X., et al. (2020). Topical application of exosomes derived from human umbilical cord mesenchymal stem cells in combination with sponge spicules for treatment of photoaging. Int. J. Nanomedicine 15, 2859–2872. doi:10.2147/ijn.s249751
Zhang, S., and Duan, E. (2018). Fighting against skin aging: the way from bench to bedside. Cell. Transpl. 27 (5), 729–738. doi:10.1177/0963689717725755
Zhang, Y., Zhang, M., Yao, A., Xie, Y., Lin, J., Sharifullah, F., et al. (2022). Circ_0011129 encapsulated by the small extracellular vesicles derived from human stem cells ameliorate skin photoaging. Int. J. Mol. Sci. 23 (23), 15390. doi:10.3390/ijms232315390
Zhao, C., Zhou, L., Chiao, M., and Yang, W. (2020). Antibacterial hydrogel coating: strategies in surface chemistry. Adv. Colloid Interface Sci. 285, 102280. doi:10.1016/j.cis.2020.102280
Zhao, X., Liu, Y., Jia, P., Cheng, H., Wang, C., Chen, S., et al. (2021b). Chitosan hydrogel-loaded MSC-derived extracellular vesicles promote skin rejuvenation by ameliorating the senescence of dermal fibroblasts. Stem Cell. Res. Ther. 12 (1), 196. doi:10.1186/s13287-021-02262-4
Zhao, Y., Dai, Q., and Xiao, R. (2021a). Photoaged skin therapy with adipose-derived stem cells. Plast. Reconstr. Surg. 148 (3), 494e. doi:10.1097/prs.0000000000008242
Keywords: hydrogel, stem cells, photoaging, collagen, rejuvenation
Citation: Cao L, Qian X, Min J, Zhang Z, Yu M and Yuan D (2024) Cutting-edge developments in the application of hydrogels for treating skin photoaging. Front. Mater. 11:1443514. doi: 10.3389/fmats.2024.1443514
Received: 04 June 2024; Accepted: 17 September 2024;
Published: 30 September 2024.
Edited by:
Jianshe Hu, Northeastern University, ChinaReviewed by:
Yiming Zhang, Xinqiao Hospital, ChinaVincenza De Gregorio, University of Naples Federico II, Italy
Copyright © 2024 Cao, Qian, Min, Zhang, Yu and Yuan. This is an open-access article distributed under the terms of the Creative Commons Attribution License (CC BY). The use, distribution or reproduction in other forums is permitted, provided the original author(s) and the copyright owner(s) are credited and that the original publication in this journal is cited, in accordance with accepted academic practice. No use, distribution or reproduction is permitted which does not comply with these terms.
*Correspondence: Lili Cao, Y2FvbGwyMTVAMTYzLmNvbQ==