Erratum: Prospects for 3D-printing of clear aligners—a narrative review
- 1Stomatology Collage of Jiamusi University, Jiamusi, Heilongjiang, China
- 2Key Laboratory of Oral Biomedical Materials and Clinical Application, Jiamusi, Heilongjiang, China
Clear aligner therapy is a rapidly developing orthodontic treatment. 3D-printing technology, which enables the creation of complex geometric structures with high precision, has been used in dentistry. This article aims to summarize the various aspects of 3D-printing clear aligners and give an outlook on their future development. The traditional thermoforming technology is introduced and the principle and application of 3D-printed clear aligners and materials are introduced, as well as the application prospects of 3D-printed clear aligners. According to PRISMA statement, the relevant literature of 3D-printing clear aligner was searched in PubMed, Web of Science, Embase and other databases. We searched the related words in the MESH database and then carried out advanced searches. We read systematic review and conference papers to find the articles related to the subject and manually added and excluded articles by reading the title and abstract. The production of clear aligners combines computer-aided 3D analysis, personalized design and digital molding technology. The thickness and edges of the 3D-printed clear aligner can be digitally controlled, which allows appliance more efficiently fitted. Presently, the array of clear resins suitable for 3D-printing include photo polymeric clear methacrylate-based resin (Dental LT) (Form Labs, Somerville, Mass), aliphatic vinyl ester-polyurethane polymer (Tera Harz TC-85) (Graphy, Seoul, South Korea). They all have good biocompatibility. But no such material is currently approved on the market. Developing biocompatible resins and further improving the material’s mechanical properties will be critical for the combination of 3D-printing and clear aligners. However, the literature on 3D-printed clear aligners is limited and lacks clinical application. Further in vivo and in vitro tests, as well as additional exploration in conjunction with corresponding cytological tests, are required for the research on available materials and machinery for 3D-printing clear aligners.
1 Introduction
Clear aligners are extensively used orthodontic devices (Kaklamanos, Makrygiannakis and Athanasiou, 2023; Park, 2022). The force mode of the clear aligner is different from that of the conventional fixed appliances. It transfers the force to the periodontal tissue through the shape change, thereby causing the tooth movement and alveolar bone reconstruction (Quinzi et al., 2022). Its three-dimensional wrapping mode enables full control of teeth (Caruso et al., 2024; Kaklamanos et al., 2023). Ideal clear aligners should have good resilience, energy storage and biocompatibility. Figure 1 shows the transition from conventional clear aligners production to 3D printing clear aligner. Harold D. Kesling (Kesling, 1946) invented a tooth positioning device in 1945, with the goal of creating a device that could move teeth without using brackets and archwires. Sankes first demonstrated clear retainers and the equipment capable of producing them in 1963. Ponitz utilized Kesling’s concept of moving and repositioning teeth in a pink base plate wax on the model in 1971 (Ponitz, 1971). Sheridan introduced a clear aligner technique in 1993 that combined clear aligners with interproximal reduction utilizing 0.030″ thermoplastic copolyester sheets. Later in the early 1990s, Sheridan and others created Essix retainers for continuous tooth movement (Sheridan, LeDoux and McMinn, 1993). The Invisalign® system was pioneered by two graduates of Stanford University, namely, Zia Chishti and Kelsey Wirth. It is an invisible orthodontic device designed and manufactured in America by Align Technology (Santa Clara, CA) and a complete clear aligner therapy system (Bichu et al., 2023). Over the years, the clear aligner has undergone numerous changes. Computer-aided design/computer-aided manufacturing (CAD/CAM) uses computer to process various digital information and graphic information to assist in the design and manufacture of aligner. Over the past few decades, the widespread utilization of CAD/CAM techniques in the dental field have facilitated large-scale manufacturing of clear aligners, significantly advancing the use of clear aligners for orthodontic purposes (Bauer et al., 2022; Suganna et al., 2022). 3D-printing, also known as additive manufacturing, boasts exceptionally high production efficiency and is capable of rapidly constructing objects with intricate shapes (G. M. Tartaglia et al., 2021). It has extremely high flexibility and can meet personalized and some special needs. In traditional manufacturing methods, materials often need to be processed by cutting, turning and carving, resulting in a large amount of waste. Additive manufacturing technology directly processes materials in the required area, which results in less material waste and saves material resources. Due to its capacity for personalized treatment, digital dentistry has expanded rapidly over the past decade (Subramanian and Harikrishnan, 2023). Table 1 shows the 3D-printing techniques commonly used in dentistry. The ability of 3D-printing technology to create precise products and reduce production time is appealing to both scientists and orthodontists. In the past 10 years. As 3D-printing technology continues to advance, it is increasingly utilized in the medical field, particularly for orthodontic treatment (Khorsandi et al., 2021; Tartaglia et al., 2021). Nasef first tried to use 3D-printing technology to manufacture retainers, which brought inspiration for the printing of clear aligners (Nasef et al., 2014). Using 3D-printing technology to directly manufacture the clear aligners can not only avoid many disadvantages of the existing production process, but also use digital auxiliary design to make the course of treatment more simplified (Tian et al., 2021).
Currently, Dental Long Term (LT) resin (Form Labs, Somerville, Mass), Tera Harz TC-85 (Graphy, Seoul, South Korea) and Accura 60 SLA (3D Systems, Rockhill, SC) are among the clear resins available for photopolymerization 3D-printing (Jindal et al., 2019; Kumar, 2019; Lee et al., 2022). Currently used materials for 3D-printing do not meet biocompatibility standards, with the exception of Tera Harz TC-85 (Graphy, Seoul, South Korea), which has received approval from the Food and Drug Administration (FDA), the European Commission (EC) and the Korean Food and Drug Administration (KFDA) (Lee et al., 2022). But final approval from the Food and Drug Administration for commercial use of Tera Harz TC-85 aligner material is still pending (Lee et al., 2022).
At present, there are few studies on the production of clear aligners by 3D-printing and the appearance and surface quality of the clear aligner made directly using 3D-printing technology do not meet the clinical requirements (Rajput et al., 2023). These problems may be caused by the improper printing and post-processing processes (Bangalore et al., 2023). This article will introduce the procedure, technology and materials of 3D-printing clear aligners and look forward to the future.
2 Traditional thermoforming technology
The great majority of manufacturing processes used in aligner orthodontic treatment are still thermoforming techniques, notwithstanding advancements in materials and auxiliary designs (Cousley, 2020; Grassia et al., 2023). The most popular polymers used in the production of orthodontic aligners made of clear plastic that are sold commercially include polyester, polyurethane or co-polyester, polypropylene, polycarbonate, ethylene vinyl acetate and polyvinyl chloride among others (Tamburrino et al., 2020). Table 2 shows the types and properties of resins for fabricating thermoplastic aligners. Depending on the kind of malocclusion and the quantity of aligner replacements needed, creating a set of clear aligners from dental models can be expensive, time-consuming and labor-intensive (Hallmann and Gerngross, 2021; Peter et al., 2022; Venezia et al., 2022). Properties of a material such as transparency, surface hardness, water solubility, water absorption, bending modulus and elastic modulus can all be altered by thermoforming (Zheng et al., 2017). Kwon et al. (2008), discovered that the thermoforming process and mechanical loading substantially altered the mechanical properties of the clear aligner material, resulting in a reduction in force transfer. Ryu et al. (2018), found that thermoforming increases their water absorption, solubility and surface hardness while reducing the transparency of materials. Temperature, humidity, pressure, radiation and salivary enzymes can result in chemical reactions that alter the physical and mechanical properties of the aligner. Dalaie et al. (2021) discovered that thermoforming reduced flexural modulus, hardness, glass transition temperature, elastic, while the viscosity modulus and the loss factor were not affected (Dalaie et al., 2021). The material is stretched unevenly during thermoforming, leading to inaccurate sizing, patient discomfort and a prolonged treatment time. Although clinically acceptable, all clear aligner materials are mildly cytotoxic and the thermoforming procedure amplifies it (Jindal et al., 2020a). Gracco et al. (2009) detected microcracks, erosion and delamination, localized calcified biofilm deposits and loss of transparency after 14 days of wearing clear aligners. But the thermoplastic aligners suspended in artificial saliva did not discharge any monomers or byproducts and the material was biocompatible (Gracco et al., 2009). Thermoforming and intraoral environment alter the properties of material, which may influence treatment outcomes (Doomen et al., 2018). In contrast to the two commercially available plastic foils that were tested, which are frequently used to produce thermoplastic aligners, the printed aligners demonstrated superior fitting accuracy, according to Koenig et al. (2022). Jindal et al. (2020a), reported that LT clear resin is a good clinical substitute since it functions similarly to thermoplastic materials under mechanical stress of non-linear compressive forces equivalent to human bite cycles. Because of its exceptional break resistance, dental LT resin is the perfect material to use for creating printed orthodontic products like orthodontic retainers and gnathological splints (Alharbi and Osman, 2024).
The emission of cyanide and bisphenol-A (BPA) as a result of thermal degradation enhances the strength and transparency of clear aligners (Freitas, 2022). Plastics have an effect on marine life, global warming and human health (Peter et al., 2022). To prevent cross-infection and environmental contamination, their disposal must be environmentally friendly (Freitas, 2022). Thus, to increase production efficiency and reduce costs, it is necessary to personalize the fabrication process by enhancing existing technologies. 3D-printing can be viewed as a more convenient alternative to thermoforming because it does not require altering the material’s properties (Pratsinis et al., 2022). As 3D-printing of clear aligners is a direct production method, there is no need for printed models and vacuum prototypes, which saves time and money (Lo et al., 2022; Wu, 2022). The popularity of employing orthotics in the office, which saves time and money, is a result of the recent decrease in the cost of 3D printers, which has made them more accessible in dentistry and orthodontic clinics (Khorsandi et al., 2021; Shannon and Groth, 2021). Furthermore, ongoing improvements in procedures and technology are actively lowering the material waste related to thermoplastic appliances. This is accomplished by using a minimal number of models and attachments during the printing process (Tartaglia et al., 2021). The fabrication of 3D-printed aligners will increasingly shift from conventional techniques to an entirely digital operation. The next orthodontic revolution is the 3D-printing of clear aligners utilizing specified polymers. Table 3 shows advantages and disadvantages of thermoplastic and 3D printed aligners.
3 3D-printing of clear aligners
For the 3D-printing of aligners, various 3D-printing processes, including fused filament fabrication, selective laser sintering, selective laser melting and stereolithography can be utilized (Bichu et al., 2023; Jindal et al., 2020a; Nakano et al., 2019). The utilization of photopolymerization of transparent resins for 3D-printing shows potential as a promising alternative (Bichu et al., 2023). However, the literature and clinical data on printing technology for clear aligners are limited. Printing clear aligners requires refinement and improvement in people’s perception of digital orthodontic treatment (Behrents, 2016; Chandran et al., 2022; Sycińska-Dziarnowska et al., 2022), as well as a continued effort to integrate 3D-printing and orthodontic treatment, in order to attain widespread acceptance. Figure 2 shows the production process of 3D printing clear aligners.
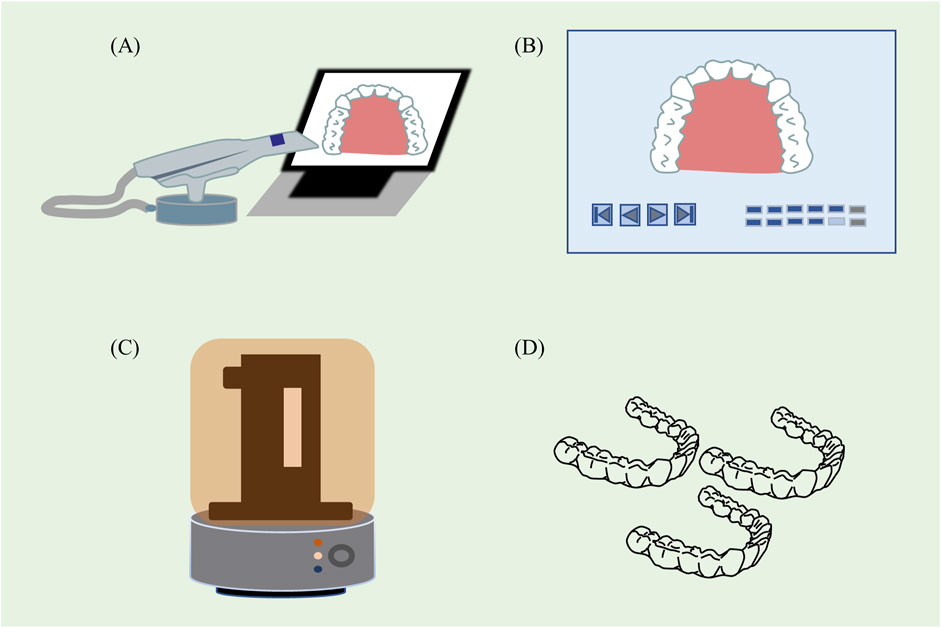
Figure 2. The production process of 3D printing clear aligners. (A) Obtain data through the intraoral scan. (B) The medical design expert carries out the tooth correction design on the digital model through the computer-assisted medical diagnosis and design system. (C) 3D printing by resin and printer. (D) The clear aligners of each step are produced by 3D printing and post-curing.
3.1 Principle
The intraoral scanning eliminates many of the variables associated with traditional impressions, allowing dental technicians to make designs based on more accurate data. A wide variety of intraoral scanners with different light source combinations and working principles are available for clinicians to choose from. Common digital intraoral scanners are iTero (Align Technology, Inc. San Jose, Calif., United States) and Trios (3Shape, Copenhagen, Denmark) (Kuo and Miller, 2003). Using computer aided design to simulate tooth arrangement, the effect of correction can be predicted. However, at this stage, the combination of digital technology and clear aligner therapy needs to be improved. In clinical practice, sometimes the teeth may not move in the direction designed by the virtual tooth arrangement software, resulting in deviations between the actual moving position of the teeth and the target position and re-oral scanning and design should be performed if necessary. The ideal clear aligners should have transparency, low rigidity, resilience, elasticity, resistance to mechanical stress and durability, as well as biocompatibility (Francisco et al., 2022; Khan et al., 2022; Rajasekaran and Chaudhari, 2023). 3D-printing of clear aligners primarily employs photo-polymerization, which is a technology that regulates the light-curing of photosensitive resin to form target parts under computer-aided design direction. Light-curing 3D-printing has the advantages of high precision and rapid printing pace when compared to other 3D-printing technologies (Jheon et al., 2017). Since approximately 30 years ago, it has been widely utilized in industry, design, engineering and manufacturing (Tian et al., 2021). The photopolymerization process yields finished products with a high level of surface accuracy, which allows for a clearer display of product details (Cousley, 2022). Compared to standard reference files, retainers manufactured using stereolithography, digital light processing, continuous liquid interface production and polyjet photopolymer technologies were clinically acceptable and accurate (Tartaglia et al., 2021). The stereolithography and polyjet photopolymer printers produced the most precise prints due to their greater precision and authenticity (Naeem et al., 2022). The photosensitive resin material utilized for 3D-printing is comprised of four primary components: photoinitiator, oligomer, monomer diluent and infill or additive. Photosensitive resins have excellent flowability and a rapid light curing rate (Panayi, 2023). They are the optimal material for 3D-printing of clear aligners and the material of choice for printing high-precision parts. Jindal et al. (2019) demonstrated the successful fabrication of 0.75 mm thick clear aligners using. Dental LT resin on a Formlabs 3D-printer and compared their mechanical and geometric properties to those of conventionally fabricated aligners. The combination of 3D-printing and Dental LT clear resin to produce clear aligners is more suitable for patients due to their higher geometric accuracy and reducing processing time while ensuring that 3D-printing of clear aligners are mechanically stronger and more resilient than thermoforming-based clear aligners (Jindal et al., 2019). Post-curing facilitates the polymer’s ability to undergo further cross-linking, resulting in an ultimate improvement of the mechanical properties of the printed material and a reduction in random residual stresses (Jindal et al., 2020a; Lambart et al., 2022). The uncured aligners underwent greater plastic deformation under higher compressive loading conditions, whereas the aligners were cured between 400°C and 800°C for 15–20 min before breaking after being subjected to higher compressive. The compression modulus ratio between the cured and uncured aligner is 4.46–5.90 (Jindal et al., 2020b).
The printed aligner material must undergo post-processing to remove supports, clean any remaining uncured resin and undergo curing after production. It is suggested that the Tera Harz TC-85 aligners be cleaned by centrifugation for about 3–5 min or that any residue be scraped off with a soft scraper in order to improve the physical characteristics of the resin material. This will also help to prevent the formation of an oxygen inhibition layer (Lee et al., 2022; Pratsinis et al., 2022; Zinelis et al., 2022). The Dental LT-clear resin aligners undergo post-production curing with the Form Cure machine (Formlabs, Somerville, Mass, United States) (24, 34, 38) and cleaning by ultrasonication with isopropyl alcohol (McCarty et al., 2020; Rajasekaran and Chaudhari, 2023).
The research on clear aligners is progressively shifting from thermoformed and vacuum-formed retainer production to 3D-printed clear aligner production. Additionally, 3D-printing will progressively transition from the production of retainers to clear aligners.
3.2 Materials
The aligner material should be resistant to deterioration in the oral environment in order to withstand chewing forces (Hartshorne and Wertheimer, 2022; Panayi, 2023). The literature on the materials and their properties is insufficient and there is a lack of relevant printing materials despite developments in 3D-printing technologies. Table 4 shows mechanical properties and cytotoxicity of different resins used for 3D-printing.
Dental LT Clear resin is composed of 7,7,9-(or 7,9,9)-trimethyl-4,13-dioxo-3, 14-dioxa-5, 12-diazahexadecane-1,16-diyl bismethacrylate, 2-hydroxyethyl methacrylate, a reaction mass of Bis(1,2,2,6,6-pentamethyl4-piperidyl) sebacate and methyl 1,2,2,6,6-pentamethyl-4-piperidyl sebacate, diphenyl(2,4,6trimethylbenzoyl)phosphine oxide, acrylic acid, monoester with propane-1,2-diol, ethylene dimethacrylate, 2-hydroxyethyl acrylate, mequinol, 4-methoxyphenol and hydroquinone monomethyl ether (Paradowska-Stolarz et al., 2022). Dental LT resin (Form Labs, Somerville, Massachusetts) is FDA approved, photo polymeric clear methacrylate-based (methacrylate oligomer and glycol methacrylate) resin made available for appliance fabrication considered to have long-term biocompatibility (Cole et al., 2019; Edelmann et al., 2020; Jindal et al., 2019). And it is a photosensitive resin material suitable for 3D-printing of medical devices that have direct contact with the human oral environment (Jindal et al., 2020b). Dental LT complies with the fundamental rules and specifications of the Medical Device Directive 2007/47/EC and Council Directive 93/42/EEC. The material utilized also complies with a number of specific standards, such as EN-ISO 10993–3:2009, EN-ISO 10993–5:2009, EEN ISO 1641:20092 and EN 908:2008. These standards are all related to dentistry equipment, medical device materials and biological testing of medical devices (Jindal et al., 2020b).
Tera Harz TC-85 is a photopolymer material introduced by Graphy, which can be printed directly by a 3D printer to overcome the limitations of existing thermoformed clear aligners. It has excellent optical properties, including a low refractive index and a high degree of transparency. In addition, it has high-temperature dimension stability and shape memory properties. Tera Harz TC-85 is an aliphatic vinyl ester-polyurethane polymer functionalized with methacrylate (Can et al., 2022). It is a shape memory polymers (SMPs) (Atta et al., 2024). The essential components of SMPs’ shape memory mechanism are the existence of a stable polymer network that establishes the material’s initial shape and a reversible polymer network that permits the material to change into a transient shape. SMPs can reach greater strain levels and can take on a variety of transient forms. The material’s various phases, each of which has a unique form recovery temperature, are thought to be responsible for this unique property (Lendlein and Kelch, 2002; Meng and Li, 2013; Xia et al., 2021).
The TC-85 DAC is transparent and the TC-85 DAW is white for durability and aesthetic purposes (Bichu et al., 2023). The product can be utilized in orthodontics, particularly following tooth extraction. In addition, Graphy announced that it is color-customizable to satisfy the needs of patients and clinical specifications (Bichu et al., 2023). Comparing the thermomechanical properties of PETG and the light-curing resin TC-85, it was discovered that TC-85 is flexible and viscoelastic, allowing for the consistent application of lower forces on the teeth when used in 3D-printed clear aligners (Lee et al., 2022). In addition, it is anticipated that force decay induced by repeated application of clear aligners will be mitigated and a constant orthodontic force will be maintained. Clinical applications can benefit from its geometric stability at high temperatures and shape-memory properties. Due to the increased flexibility and range of elasticity of clear aligners made with TC-85, more tooth movement per step can be accomplished without permanent deformation. The tension relaxation and creep behavior of TC-85 gives these clear aligners greater flexibility and a better fit when worn in the mouth. The creep behavior of TC-85 provides a progressive increase in static force under cyclic loading, which may improve clinical performance by reducing force decay and preserving the aligner’s orthodontic force. TC-85’s cross-linked structure is extremely stable, thus able to maintain a constant stiffness as well as static force and strain recovery patterns following repeated loading. Accura 60® SLA (3D systems, Rockhill, South Carolina), a polycarbonate-based SLA material is also available for aligner fabrication (Kumar, 2019).
In order to manufacture the acrylic-epoxy hybrid light-cured resin for 3D-printing using only water-soluble monomers, a Japanese team created an acrylic-epoxy hybrid photopolymerizable resin known as 3D:1M (Okamoto Chemicals) and undertook aligner fabrication with thicknesses of 0.7 mm and 1.5 mm (Nakano et al., 2019). They inventively mixed epoxy monomers to increase the sensitivity of their photoreactions because the photoreactions of epoxy monomers are slower than those of acrylic monomers and because the relatively high-sensitivity antimony photoinitiators were not used in this investigation. Additionally, the sensitivity and tensile strength of the mixture are higher than those of the individual components when epoxy and acrylic monomers are added along with photoinitiators. 3D: 1M does not respond to the skin, cannot cause cancer and is not harmful to reproduction. However, their intermediate components are fragile and these monomers are required to further enhance their mechanical properties (Nakano et al., 2019).
As an alternative to expensive and cumbersome metal bracket-based or plastic thermoforming techniques, it is necessary to develop a transparent resin for orthodontic use that satisfies multiple requirements and has outstanding properties (Tsoukala et al., 2023). The utilization of bioactive glass in the formulation of materials for 3D-printing, which possess the capability to release ions, presents a promising avenue for the fabrication of orthodontic aligners. Zbigniew and colleagues developed a novel 3D-printing material possessing bioactive characteristics, The material obtained released Ca2+ and PO43- ions when using glass Biomin C at pH 4. Which has promise for its application in the fabrication of transparent detachable orthodontic appliances (Raszewski et al., 2022). However, the mechanical qualities were also diminished by the inclusion of bioactive glass. This results from the glasses not undergoing the silanization process, thus they did not form a chemical bond between the resin and the filler (Raszewski et al., 2022).
Key questions about the effects of tunable printing parameters, such as build angle, post-print heat and duration of ultraviolet (UV) light exposure, on the dimensional accuracy of aligners directly fabricated using 3D-printing of clear materials marketed for long-term intraoral use remain, despite the emergence of technologies presenting the potential to directly 3D print aligner designs (McCarty et al., 2020; Boyer et al., 2021; Williams et al., 2022; Šimunović et al., 2023).
3.3 Thickness
Different clear aligner thicknesses were designed for various tooth surfaces by 3D-printing, which has the potential to improve the prescribed orthodontic motions while minimizing unwanted tooth movements, hence boosting tooth movement predictability (Grant et al., 2023). There are patents for 3D-printed clear aligners with flat occlusal surfaces that can simultaneously treat temporomandibular disorder (TMD), mandibular growth and obstructive sleep apnea-hypopnea syndrome, as well as position the teeth orthodontically (Tartaglia et al., 2021). Theoretically, it is possible to manufacture custom thickness clear aligners using 3D-printing and to spatially control the aligner’s thickness. But Edelmann et al. (2020) demonstrated through experimentation that the 3D-printed aligners were, on average, thicker than the corresponding design files. This may have negative effects on their clinical application (Edelmann et al., 2020).
The ability to print aligners with uniform thickness, which has the advantage of applying uniform forces to all teeth, is a significant benefit of printed aligners. A study by Koenig et al. (2022) found that the thermoformed aligner has a considerable decrease in thickness compared to the printed aligner, which thickened by 12%. According to another study, thermoformed aligners are thinner than the original plastic foil following the thermoforming process (Koenig et al., 2022). The differences in thickness can be attributed to the distinct processes of thermoforming and 3D-printing. Thermoforming typically results in material thinning, while printing presents the challenge of excess resin removal before the post-curing stage (Bucci et al., 2019; Palone et al., 2021). Incomplete removal of resin leads to an increase in ultimate layer thickness. This increase could also be due to water absorption or an increase in surface roughness of the printed aligner following intraoral usage (Koenig et al., 2022). Park et al. (2023) conducted a comparative analysis of the thickness, gap breadth and transparency of clear aligners produced using 3D-printing and thermoforming techniques. The researchers employed micro-computed tomography (micro-CT) as the imaging method for their investigation. Throughout the production process, it was discovered that the clear aligners produced via 3D-printing exhibited an increase in thickness of 0.2 mm. Furthermore, distinct variations in thickness were noted across various tooth positions. Cole et al. (2019) demonstrated that 3D-printed retainers deviated from the original reference model by no more than 0.50 mm, which has been deemed clinically acceptable. They suggested that PMMA resin is not the best material to employ for this purpose and attributed the largest deviations of the 3D-printed retainer group to its extreme rigidity (Cole et al., 2019).
When compared to thermoformed and uncured 3D printed aligners, cured aligners showed better compressive mechanical strength and dimensional precision. The type of 3D-printing technology used, the post-curing process and the anatomical features of the teeth can all have an impact on how thick the clear aligners are. Naeem also came to the conclusion that the accuracy of the aligners was impacted by the print orientation, the post-processing techniques, overexposures of some layers and mistakes from the CBCT scan (Naeem et al., 2022). According to Williams et al. (2022), when compared to the digital reference file, the incisal edges and cusp tips of the 3D-printed retainers were accurate to within 0.25 mm. However, the smooth facial surfaces fell short of clinical acceptability standards. The most time-saving and most economical printing angles are 15 and 45, respectively (Williams et al., 2022).
The biomechanical properties of the aligners are determined by the material properties, the thickness of the material and the precision of the fit between the aligners, the teeth and any accoutrements. The selection of the proper aligner thickness is a crucial aspect of the orthodontics (Hartshorne and Wertheimer, 2022). Additional research on stress distribution and deformation at various aligner thicknesses is required. Consideration should be given to 3D printed clear aligners with reduced step effects and build higher physical prototype accuracy, allowing for greater control over tooth movement. The clinical application of 3D-printing clear aligners should take into account the stress encountered in the patient’s mouth and the stress variations in the dental tissue. Due to the fact that various types of aligners have different mechanical properties, it is crucial for designers to consider the mechanical loads and stress distribution in the periodontal tissue under their action (Rossini et al., 2015).
3.4 Biotoxicity
Various photocuring resins have different biocompatibility due to their different material compositions. Kumar (2019) discovered that Polyurethane (Invisalign®) and methacrylate oligomer–glycol methacrylate (Dental LT®) are biocompatible and safe for intraoral orthodontic use. However, the use of Polycarbonate (Accura 60®) intraorally is questionable as it was found to be significantly more toxic than polyurethane and methacrylate oligomer–glycol methacrylate. Accura 60® releases Bisphenol-A, a recognized environmental endocrine disruptor with estrogen-like effects. The leaching of methacrylate from Dental LT resin was found to be highest on day 1, with decreasing levels observed as the days progressed. This decrease in leaching correlated with a reduction in cell viability. Kopperud et al. (2011) have suggested that the cytotoxic effects of methacrylate are attributed to its genotoxicity, whereby the monomer directly impacts DNA through the formation of reactive oxygen species. Andreas found that Tera Harz TC85A can be efficiently aggregated. The cytotoxicity was greatest on the first day and decreased over time. Although the leachate did not contain bisphenol-A, the large variation in the amount of UDMA monomer leached from the tested samples may pose potential health risks after intraoral environment use of the aligners (Willi et al., 2023).
Polymerization during 3D-printing only results in a partial reaction of the double bonds and a certain degree of conversion of the C = C double bonds in the methacrylic group. Therefore, for the material to be fully cured and more bio-compatible, it is necessary to subject the material to a post-curing process in a light furnace (Raszewski et al., 2022). Prior to 3D-printing, materials are exceedingly toxic, but their toxicity decreases after polymerization. Various post-processing procedures did not affect surface characteristics. The chemical ingredients of the post-rinsing solutions may have had a significant impact on the aligner’s flexural strength. Cytocompatibility was not altered by any of the post-rinsing procedures (Lambart et al., 2022). Pratsinis et al. (2022) discovered that any factors released from 3D-printed aligners during 14 days of aging in water were non-cytotoxic to human gingival fibroblasts and had no effect on their intracellular reactive oxygen levels. Based on the limited preliminary evidence provided by in vitro studies, cytotoxic effects or estrogen levels of clear aligners cannot be confirmed (Pratsinis et al., 2022).
3D-printing clear aligners lack existing biocompatible resins, but there are many experiments that simulate the intra-oral environment to test the biocompatibility (Kumar, 2019; Nakano et al., 2019; FayyazAhamed et al., 2020). In order to guarantee these materials’ safety and effectiveness for use by patients, the FDA’s approval is an essential first step. The physical and mechanical qualities of 3D-printed dental resins used in orthodontic devices must meet the requirements specified in ISO 20795-2 in order to guarantee their quality and safety (Nakano et al., 2019). Due primarily to trade secrets, one important barrier is the paucity of knowledge regarding the chemical makeup of current dental resins (Alharbi and Osman, 2024). Furthermore, few Food and Drug Administration (FDA)-approved resins are available for 3D-printed aligners, despite the fact that a number of businesses are actively working on advanced development stages of this technology (Wu, 2022).
While the existing body of literature on the topic of 3D-printing aligners suggests that this approach is theoretically viable, it is important to note that no materials have been officially authorized for commercial usage. Based on the existing body of research, it is imperative to conduct in vitro and in vivo experimentation to evaluate the efficacy and safety of 3D-printing technology and innovative materials. Further advancements should be made in the refinement of biocompatible resins possessing superior safety profiles and desirable physical characteristics, hence facilitating their application for long-term usage within the oral environment.
3.5 Effect
Using a series of computer-generated designs, clear aligners are manufactured to provide step-by-step directions for the correct movement of teeth (Jacox et al., 2022; Koenig et al., 2022). In theory, this new manufacturing technique enables the production of products of various sizes and configurations in a fraction of the time required by conventional methods and can be utilized anywhere and at any time (Tian et al., 2021). But the use of 3D-printing technology to produce clear aligners is still not mature. It is necessary to enhance the pace of printing and post-processing to comply with dental, technical and biocompatibility standards (Tian et al., 2021; Willi et al., 2023). Entry-level 3D printers may require frequent recalibration to resolve printing problems, potentially affecting the efficiency of CAD/CAM workflows in an office environment, particularly for treatments involving numerous aligners (Hartshorne and Wertheimer, 2022). In the future, we will consider openly adopting the 3D printing process for low-budget 3D printers, effectively complementing conventional methods (Lo Giudice et al., 2022; Venezia et al., 2022; Grassia et al., 2023). By utilizing 3D-printing, it is possible to produce aligners with novel geometries that apply torque, rotation and full 3D control forces to teeth in novel ways (Tsoukala et al., 2023). McKay et al. (2023) found that thermoformed aligners generated significantly higher mean forces and moments than 3D-printed aligners. Extrusion can be accomplished in the absence of attachments through the utilization of pressure columns within 3D-printed aligners employing TC-85. Nevertheless, it is important to note that these strategies may also result in unintended forces (McKay et al., 2023).
The contentious issue surrounding the qualities of 3D-printed clear aligners pertains to the influence of print orientation and post-curing. McCarty and others (McCarty et al., 2020) suggest that print orientation and post-curing time have little effect on the overall precision of 3D-printed aligner designs. Future research should consider the potential impact of position-specific bias on the clinical implementation of 3D-printed clear aligners. But Boyer et al. (2021) concluded that the orientation of printing significantly affects the dimensional accuracy of directly 3D-printed clear aligners and suggested that printing at a 90-degree angle produces the least deviation. Additionally, it has been suggested that the printing angle has a substantial impact on the color stability and surface texture of orthodontic appliances. Bangalore et al. (2023) analyzed the impact of printing angle, coffee staining, simulated bristle-induced color variation and surface texture on 3D-printed aligners and compared them to conventional thermoplastic aligners. Among the various angles used to produce the 3D-printed retainers, the 15° angle was found to be the most advantageous in terms of color stability and surface roughness (Bangalore et al., 2023). Centrifugal treatment in post-processing significantly improved the 3D-printed aligners’ transparency. However, because centrifugal cleansing is a physical rather than chemical process, there is a risk of aligner distortion (Bichu et al., 2023).
The alignment between aligners and teeth is subject to various factors, including the printing orientation and the duration of post-print curing. The study conducted by Jindal et al. examined the impact of post-curing conditions on the mechanical characteristics of clear aligners produced using printing techniques. The findings of the study revealed that both time and temperature play a critical role in determining the compressive strength of the printed resin (Prashant Jindal et al., 2020b). The researchers reached the conclusion that applying pressure during the post-curing process can effectively reduce deformation and enhance the tensile characteristics of the material. Adherence to a correct post-curing protocol is crucial for achieving adequate mechanical strength, biocompatibility and other desirable characteristics in the final product resulting from 3D-printing (Prashant Jindal et al., 2020b). The monomer of the Dental LT resin is based on acrylic ester, which limits the chain mobility of the cross-linked polymer network and increases flux during curing, leading to a large number of radicals. The mobility of unreacted double bonds becomes a limiting factor in the overall conversion of double bonds during post-curing. The kinetic chain generated by propagating radical leads to extra cross-linking of the resin during printing, ultimately enhancing the mechanical properties of the printed parts (Loza-Herrero et al., 1998; Hague et al., 2004).
Zinelis et al. (2022) conducted a comparative analysis of the mechanical qualities exhibited by aligners produced using different commercially available 3D printers. Their findings revealed a significant correlation between the mechanical characteristics of printed orthodontic appliances and the specific 3D printer utilized in their production (Zinelis et al., 2022). It was discovered that the relaxation index of 3D printed clear aligners was nearly ten times greater than that of Invisalign®, which is cause for concern regarding the degree of relaxation of 3D printed clear aligners (Uzunov et al., 2023). And evidence revealed that the exposure and use of aligners within the oral cavity resulted in notable and significant alterations in the roughness characteristics of aligners produced by 3D-printing, at all levels (Koletsi et al., 2023).
Shirey et al. (2023) conducted a comparative analysis of the mechanical characteristics exhibited by thermoformed aligners that are commercially available and 3D printed aligners. This investigation was carried out in controlled laboratory settings, as well as in a simulated oral environment. The results of their research revealed notable disparities in terms of elastic modulus, ultimate tensile strength and stress relaxation between the two types of aligners. The mechanical properties of 3D printed clear aligners were found to be significantly affected by the humidity of the simulated oral environment, as compared to thermoformed aligners (Shirey et al., 2023). This may impact the ability of 3D-printed aligners to generate and maintain adequate levels of tooth movement forces. However, in vivo studies have shown that the mechanical properties of 3D-printed aligners are unaffected throughout a 1-week period of utilization (Can et al., 2022). Aleksa Milovanovi’c (Milovanović et al., 2021) examined the effect of time on the mechanical properties of biocompatible photopolymer resin to determine the optimal time for placing 3D printed aligners. Tensile, compression, and three-point bending experiments on biocompatible dental LT Clear V1 (Formlabs) material revealed an increase in tensile, compression, and bending load resistance over time. However, elongation at failure decreased, and after 7 days, the aligner can achieve optimal performance.
Customizing the coarseness of the intra-aligner reduces the need for attachments, which can make clear aligners less transparent. There is experimental evidence that the transparency of thermoformed materials is better than 3D printed materials. Rajput et al. (2023) developed chemical vapor smoothing to enhance the surface quality of the clear aligners (Rajput et al., 2023). Šimunović et al. (2023) discovered that polyurethane materials produced through 3D-printing may display a greater tendency to become stained in comparison to those made through thermoforming. This disparity can be attributed to variations in surface features and material properties resulting from the distinct production methods employed. 3D-printing commonly leads to parts with increased surface roughness and porosity, making them more susceptible to trapping staining agents (Šimunović et al., 2023). In contrast, thermoforming typically produces parts with smoother and denser structures that are less likely to stain (Park et al., 2023). The polyurethane formulations utilized in 3D-printing may vary from those used in thermoforming, as additives in 3D-printing materials could potentially impact the ability to resist stains. Moreover, the temperature history and microstructure resulting from the manufacturing procedures may impact the material’s ability to resist stains. In the case of 3D-printed materials, their quick cooling and layer-by-layer assembly could potentially create more reactive spots that are prone to staining. Moreover, exposure to chemicals during 3D printing has the potential to alter the surface characteristics, which might subsequently affect how stains interact with them (Bangalore et al., 2023). The post-curing process for 3D-printed aligners was carried out in a nitrogen environment to avoid the creation of an oxygen inhibition layer, which facilitated the polymerization of the specimen’s surface. To enhance the mechanical characteristics and surface smoothness of the 3D-printed aligners, it is beneficial to introduce inert nitrogen gas to eliminate oxygen (Reymus et al., 2019; Gojzewski et al., 2020). The effect of polishing on the characteristics of Dental LT resin is determined to be moderate, although it significantly improves the durability of the specimens against simulated aging. Hence, it is advisable to perform post-printing polishing on appliances fabricated using Dental LT Clear resin in order to augment their endurance and strengthen their resistance to wear during usage (Paradowska-Stolarz, Wezgowiec et al., 2023). Further in vivo and in vitro tests, as well as additional exploration in conjunction with corresponding cytological tests, are required to research available materials and machinery for 3D-printed clear aligners.
4 Conclusion
The combination of 3D-printing and clear aligners can lead to innovation in the material and production of clear aligners. In theory, 3D-printing can simplify the production process, overcome the shortcomings of thermoforming technology and can produce clear aligners that meet the requirements of clinical use. The resin’s particle size, shape, distribution of the granules and the polymer’s density, flow, viscosity, as well as printing process and post-curing direct impact on the mechanical properties of the final product. Because no commercially approved material exists, clinical studies of printed aligners have not been published in the current literature. Further standardized testing is needed to evaluate these new technologies and materials. Particular attention must be paid to the cytotoxicity of printable resins and the clinical performance of 3D printed aligners must be evaluated to compare the obtained data with that of thermoformed aligners. Advances in technology and materials will drive the development of clear aligners to better meet doctors’ requirements, thereby enhancing patient care.
Author contributions
CN: Writing–review and editing, Writing–original draft. DL: Writing–original draft, Writing–review and editing. YZ: Writing–review and editing. YW: Writing–review and editing. SN: Writing–review and editing. GZ: Writing–review and editing. ZY: Writing–review and editing. YK: Writing–review and editing. DY: Writing–review and editing.
Funding
The author(s) declare financial support was received for the research, authorship, and/or publication of this article. This work was financially supported by the Research Operating Expenses Project Fund of Education Department of Heilongjiang Province (No. 2023-KYYWF-0612) and Fund of Medical And Health Research Project of Heilongjiang Province (No. 2020-318).
Conflict of interest
The authors declare that the research was conducted in the absence of any commercial or financial relationships that could be construed as a potential conflict of interest.
Publisher’s note
All claims expressed in this article are solely those of the authors and do not necessarily represent those of their affiliated organizations, or those of the publisher, the editors and the reviewers. Any product that may be evaluated in this article, or claim that may be made by its manufacturer, is not guaranteed or endorsed by the publisher.
References
Alharbi, N., and Osman, R. B. (2024). Additive manufacturing technologies: where did we start and where have we landed? A narrative review. Int. J. Prosthodont 37 (7), 243–252. doi:10.11607/ijp.8828
Atta, I., Bourauel, C., Alkabani, Y., Mohamed, N., Kimbe, H., Alhotan, A., et al. (2024). Physiochemical and mechanical characterisation of orthodontic 3D printed aligner material made of shape memory polymers (4D aligner material). J. Mech. Behav. Biomed. Mater 150, 106337. doi:10.1016/j.jmbbm.2023.106337
Bangalore, D., Alshehri, A. M., Alsadon, O., Alaqeel, S. M., Alageel, O., Alsarani, M. M., et al. (2023). Coffee staining and simulated brushing induced color changes and surface roughness of 3D-printed orthodontic retainer material. Polym. (Basel) 15 (9), 2164. doi:10.3390/polym15092164
Bauer, C. A. J., Lux, C. J., and Hodecker, L. D. (2022). Orthodontic extrusion in the digital age: a technical note. Quintessence Int. 53 (5), 394–402. doi:10.3290/j.qi.b2644869
Behrents, R. G. (2016). Do-it-yourself impressions and clear retainers: a fairy tale. Am. J. Orthod. Dentofac. Orthop. 150 (2), 205–207. doi:10.1016/j.ajodo.2016.06.010
Bichu, Y. M., Alwafi, A., Liu, X., Andrews, J., Ludwig, B., Bichu, A. Y., et al. (2023). Advances in orthodontic clear aligner materials. Bioact. Mater 22, 384–403. doi:10.1016/j.bioactmat.2022.10.006
Boyer, R. A., Kasper, F. K., English, J. D., and Jacob, H. B. (2021). Effect of print orientation on the dimensional accuracy of orthodontic aligners printed 3-dimensionally. Am. J. Orthod. Dentofac. Orthop. 160 (5), 732–742.e1. doi:10.1016/j.ajodo.2021.01.018
Bucci, R., Rongo, R., Levatè, C., Michelotti, A., Barone, S., Razionale, A. V., et al. (2019). Thickness of orthodontic clear aligners after thermoforming and after 10 days of intraoral exposure: a prospective clinical study. Prog. Orthod. 20 (1), 36. doi:10.1186/s40510-019-0289-6
Can, E., Panayi, N., Polychronis, G., Papageorgiou, S. N., Zinelis, S., Eliades, G., et al. (2022). In-house 3D-printed aligners: effect of in vivo ageing on mechanical properties. Eur. J. Orthod. 44 (1), 51–55. doi:10.1093/ejo/cjab022
Caruso, S., De Felice, M. E., Valenti, C., Pagano, S., Caruso, S., Gatto, R., et al. (2024). An evaluation of the Invisalign® Aligner Technique and consideration of the force system: a systematic review. Syst. Rev. 13 (1), 43. doi:10.1186/s13643-023-02437-5
Chandran, J., Balakrishnan, N., and Sreenivasagan, S. (2022). Awareness on three-dimensional printing of orthodontic appliances among dental students. J. Adv. Pharm. Technol. Res. 13 (Suppl. 2), S563–S567. doi:10.4103/japtr.japtr_157_22
Cole, D., Bencharit, S., Carrico, C. K., Arias, A., and Tüfekçi, E. (2019). Evaluation of fit for 3D-printed retainers compared with thermoform retainers. Am. J. Orthod. Dentofac. Orthop. 155 (4), 592–599. doi:10.1016/j.ajodo.2018.09.011
Cousley, R. R. (2020). Introducing 3D printing in your orthodontic practice. J. Orthod. 47 (3), 265–272. doi:10.1177/1465312520936704
Cousley, R. R. J. (2022). In-house three-dimensional printing within the digital orthodontic workflow. J. World Fed. Orthod. 11 (6), 182–189. doi:10.1016/j.ejwf.2022.10.001
Dalaie, K., Fatemi, S. M., and Ghaffari, S. (2021). Dynamic mechanical and thermal properties of clear aligners after thermoforming and aging. Prog. Orthod. 22 (1), 15. doi:10.1186/s40510-021-00362-8
Doomen, R. A., Aydin, B., and Kuitert, R. (2018). Possibilities and limitations of treatment with clear aligners. An orientation. Ned. Tijdschr. Tandheelkd. 125 (10), 533–540. doi:10.5177/ntvt.2018.10.18131
Edelmann, A., English, J. D., Chen, S. J., and Kasper, F. K. (2020). Analysis of the thickness of 3-dimensional-printed orthodontic aligners. Am. J. Orthod. Dentofac. Orthop. 158 (5), e91–e98. doi:10.1016/j.ajodo.2020.07.029
FayyazAhamed, S., Kumar, S. M., Vijayakumar, R. K. S., AprosKanna, A. S., and Indrapriyadharshini, K. (2020). Cytotoxic evaluation of directly 3D printed aligners and Invisalign. Eur. J. Mol. Clin. Med. 7 (5), 1129-40.
Francisco, I., Paula, A. B., Ribeiro, M., Marques, F., Travassos, R., Nunes, C., et al. (2022). The Biological effects of 3D resins used in orthodontics: a systematic review. Bioeng. (Basel) 9 (1), 15. doi:10.3390/bioengineering9010015
Freitas, M. P. M. (2022). Aligners, environmental contamination, and the role of orthodontics. Angle Orthod. 92 (1), 148–149. doi:10.2319/1945-7103-92.1.148
Gojzewski, H., Guo, Z., Grzelachowska, W., Ridwan, M. G., Hempenius, M. A., Grijpma, D. W., et al. (2020). Layer-by-layer printing of photopolymers in 3D: how weak is the interface? ACS Appl. Mater Interfaces 12 (7), 8908–8914. doi:10.1021/acsami.9b22272
Gracco, A., Mazzoli, A., Favoni, O., Conti, C., Ferraris, P., Tosi, G., et al. (2009). Short-term chemical and physical changes in invisalign appliances. Aust. Orthod. J. 25 (1), 34–40. doi:10.2478/aoj-2009-0007
Grant, J., Foley, P., Bankhead, B., Miranda, G., Adel, S. M., and Kim, K. B. (2023). Forces and moments generated by 3D direct printed clear aligners of varying labial and lingual thicknesses during lingual movement of maxillary central incisor: an in vitro study. Prog. Orthod. 24 (1), 23. doi:10.1186/s40510-023-00475-2
Grassia, V., Ronsivalle, V., Isola, G., Nucci, L., Leonardi, R., and Lo Giudice, A. (2023). Accuracy (trueness and precision) of 3D printed orthodontic models finalized to clear aligners production, testing crowded and spaced dentition. BMC Oral Health 23 (1), 352. doi:10.1186/s12903-023-03025-8
Hague, R., Mansour, S., Saleh, N., and Harris, R. (2004). Materials analysis of stereolithography resins for use in Rapid Manufacturing. J. Mater. Sci. 39 (7), 2457–2464. doi:10.1023/B:JMSC.0000020010.73768.4a
Hallmann, L., and Gerngross, M.-D. (2021). Effect of dental thermoplastic materials on the clinical effectiveness of clear aligner. Austin J. Dent. 8. doi:10.26420/austinjdent.2021.1151
Hartshorne, J., and Wertheimer, M. B. (2022). Emerging insights and new developments in clear aligner therapy: a review of the literature. AJO-DO Clin. Companion 2 (4), 311–324. doi:10.1016/j.xaor.2022.04.009
Jacox, L. A., Bocklage, C., Edwards, T., Mihas, P., Lin, F. C., and Ko, C. C. (2022). Understanding technology adoption by orthodontists: a quantitative study. Am. J. Orthod. Dentofac. Orthop. 161 (3), 364–374. doi:10.1016/j.ajodo.2020.08.024
Jheon, A. H., Oberoi, S., Solem, R. C., and Kapila, S. (2017). Moving towards precision orthodontics: an evolving paradigm shift in the planning and delivery of customized orthodontic therapy. Orthod. Craniofac Res. 20 (1), 106–113. doi:10.1111/ocr.12171
Jindal, P., Juneja, M., Bajaj, D., Siena, F. L., and Breedon, P. (2020a). Effects of post-curing conditions on mechanical properties of 3D printed clear dental aligners. Rapid Prototyp. J. 26, 1337–1344. doi:10.1108/rpj-04-2019-0118
Jindal, P., Juneja, M., Siena, F. L., Bajaj, D., and Breedon, P. (2019). Mechanical and geometric properties of thermoformed and 3D printed clear dental aligners. Am. J. Orthod. Dentofac. Orthop. 156 (5), 694–701. doi:10.1016/j.ajodo.2019.05.012
Jindal, P., Worcester, F., Siena, F. L., Forbes, C., Juneja, M., and Breedon, P. (2020b). Mechanical behaviour of 3D printed vs thermoformed clear dental aligner materials under non-linear compressive loading using FEM. J. Mech. Behav. Biomed. Mater 112, 104045. doi:10.1016/j.jmbbm.2020.104045
Kaklamanos, E. G., Makrygiannakis, M. A., and Athanasiou, A. E. (2023). Oral health-related quality of life throughout treatment with clear aligners in comparison to conventional metal fixed orthodontic appliances: a systematic review. Int. J. Environ. Res. Public Health 20 (4), 3537. doi:10.3390/ijerph20043537
Kesling, H. D. (1946). Coordinating the predetermined pattern and tooth positioner with conventional treatment. Am. J. Orthod. Oral Surg. 32, 285–293. doi:10.1016/0096-6347(46)90053-1
Khan, M. I., Sm, L., Gopal, T., and Neela, P. K. (2022). Artificial intelligence and 3D printing technology in orthodontics: future and scope. AIMS Biophys. 9, 182–197. doi:10.3934/biophy.2022016
Khorsandi, D., Fahimipour, A., Abasian, P., Saber, S. S., Seyedi, M., Ghanavati, S., et al. (2021). 3D and 4D printing in dentistry and maxillofacial surgery: printing techniques, materials, and applications. Acta Biomater. 122, 26–49. doi:10.1016/j.actbio.2020.12.044
Koenig, N., Choi, J. Y., McCray, J., Hayes, A., Schneider, P., and Kim, K. B. (2022). Comparison of dimensional accuracy between direct-printed and thermoformed aligners. Korean J. Orthod. 52 (4), 249–257. doi:10.4041/kjod21.269
Koletsi, D., Panayi, N., Laspos, C., Athanasiou, A. E., Zinelis, S., and Eliades, T. (2023). In vivo aging-induced surface roughness alterations of Invisalign® and 3D-printed aligners. J. Orthod. 50 (4), 352–360. doi:10.1177/14653125221145948
Kopperud, H. M., Kleven, I. S., and Wellendorf, H. (2011). Identification and quantification of leachable substances from polymer-based orthodontic base-plate materials. Eur. J. Orthod. 33 (1), 26–31. doi:10.1093/ejo/cjq020
Kuo, E., and Miller, R. J. (2003). Automated custom-manufacturing technology in orthodontics. Am. J. Orthod. Dentofac. Orthop. 123 (5), 578–581. doi:10.1067/mod.2003.S0889540603000519
Kwon, J. S., Lee, Y. K., Lim, B. S., and Lim, Y. K. (2008). Force delivery properties of thermoplastic orthodontic materials. Am. J. Orthod. Dentofac. Orthop., 133(2), 228–234; quiz 328.e221. doi:10.1016/j.ajodo.2006.03.034
Lambart, A. L., Xepapadeas, A. B., Koos, B., Li, P., and Spintzyk, S. (2022). Rinsing postprocessing procedure of a 3D-printed orthodontic appliance material: impact of alternative post-rinsing solutions on the roughness, flexural strength and cytotoxicity. Dent. Mater 38 (8), 1344–1353. doi:10.1016/j.dental.2022.06.010
Lee, S. Y., Kim, H., Kim, H. J., Chung, C. J., Choi, Y. J., Kim, S. J., et al. (2022). Thermo-mechanical properties of 3D printed photocurable shape memory resin for clear aligners. Sci. Rep. 12 (1), 6246. doi:10.1038/s41598-022-09831-4
Lendlein, A., and Kelch, S. (2002). Shape-memory polymers. Angew. Chem. Int. Ed. Engl. 41 (12), 2034–2057. doi:10.1002/1521-3773(20020617)41:12<2034::aid-anie2034>3.0.co;2-m
Lo Giudice, A., Ronsivalle, V., Rustico, L., Aboulazm, K., Isola, G., and Palazzo, G. (2022). Evaluation of the accuracy of orthodontic models prototyped with entry-level LCD-based 3D printers: a study using surface-based superimposition and deviation analysis. Clin. Oral Investig. 26 (1), 303–312. doi:10.1007/s00784-021-03999-1
Loza-Herrero, M. A., Rueggeberg, F. A., Caughman, W. F., Schuster, G. S., Lefebvre, C. A., and Gardner, F. M. (1998). Effect of heating delay on conversion and strength of a post-cured resin composite. J. Dent. Res. 77 (2), 426–431. doi:10.1177/00220345980770021201
McCarty, M. C., Chen, S. J., English, J. D., and Kasper, F. (2020). Effect of print orientation and duration of ultraviolet curing on the dimensional accuracy of a 3-dimensionally printed orthodontic clear aligner design. Am. J. Orthod. Dentofac. Orthop. 158 (6), 889–897. doi:10.1016/j.ajodo.2020.03.023
McKay, A., McCray, J., Bankhead, B., Lee, M. M., Miranda, G., Adel, S. M., et al. (2023). Forces and moments generated during extrusion of a maxillary central incisor with clear aligners: an in vitro study. BMC Oral Health 23 (1), 495. doi:10.1186/s12903-023-03136-2
Meng, H., and Li, G. (2013). A review of stimuli-responsive shape memory polymer composites. Polymer 54 (9), 2199–2221. doi:10.1016/j.polymer.2013.02.023
Milovanović, A., Sedmak, A., Golubović, Z., Mihajlović, K. Z., Žurkić, A., Trajković, I., et al. (2021). The effect of time on mechanical properties of biocompatible photopolymer resins used for fabrication of clear dental aligners. J. Mech. Behav. Biomed. Mater 119, 104494. doi:10.1016/j.jmbbm.2021.104494
Naeem, O. A., Bencharit, S., Yang, I. H., Stilianoudakis, S. C., Carrico, C., and Tüfekçi, E. (2022). Comparison of 3-dimensional printing technologies on the precision, trueness, and accuracy of printed retainers. Am. J. Orthod. Dentofac. Orthop. 161 (4), 582–591. doi:10.1016/j.ajodo.2021.03.016
Nakano, H., Kato, R., Kakami, C., Okamoto, H., Katsuyoshi, M., and Maki, K. (2019). Development of biocompatible resins for 3D printing of direct aligners. J. Photopolym. Sci. Technol. 32, 209–216. doi:10.2494/photopolymer.32.209
Nasef, A. A., El-Beialy, A. R., and Mostafa, Y. A. (2014). Virtual techniques for designing and fabricating a retainer. Am. J. Orthod. Dentofac. Orthop. 146 (3), 394–398. doi:10.1016/j.ajodo.2014.01.025
Palone, M., Longo, M., Arveda, N., Nacucchi, M., Pascalis, F., Spedicato, G. A., et al. (2021). Micro-computed tomography evaluation of general trends in aligner thickness and gap width after thermoforming procedures involving six commercial clear aligners: an in vitro study. Korean J. Orthod. 51 (2), 135–141. doi:10.4041/kjod.2021.51.2.135
Panayi, N. C. (2023). Directly printed aligner: aligning with the future. Turk J. Orthod. 36 (1), 62–69. doi:10.4274/TurkJOrthod.2023.2023.20
Paradowska-Stolarz, A., Malysa, A., and Mikulewicz, M. (2022). Comparison of the compression and tensile modulus of two chosen resins used in dentistry for 3D printing. Mater. (Basel) 15 (24), 8956. doi:10.3390/ma15248956
Paradowska-Stolarz, A., Wezgowiec, J., Malysa, A., and Wieckiewicz, M. (2023). Effects of polishing and artificial aging on mechanical properties of dental LT clear® resin. J. Funct. Biomater. 14 (6), 295. doi:10.3390/jfb14060295
Park, J. H. (2022). Clear aligner therapy in contemporary orthodontics. AJO-DO Clin. Companion 2 (6), 511. doi:10.1016/j.xaor.2022.09.002
Park, S. Y., Choi, S. H., Yu, H. S., Kim, S. J., Kim, H., Kim, K. B., et al. (2023). Comparison of translucency, thickness, and gap width of thermoformed and 3D-printed clear aligners using micro-CT and spectrophotometer. Sci. Rep. 13 (1), 10921. doi:10.1038/s41598-023-36851-5
Peter, E., Monisha, J., and Ani George, S. (2022). Are clear aligners environment friendly? Am. J. Orthod. Dentofac. Orthop. 161 (5), 619–620. doi:10.1016/j.ajodo.2021.12.012
Ponitz, R. J. (1971). Invisible retainers. Am. J. Orthod. 59 (3), 266–272. doi:10.1016/0002-9416(71)90099-6
Pratsinis, H., Papageorgiou, S. N., Panayi, N., Iliadi, A., Eliades, T., and Kletsas, D. (2022). Cytotoxicity and estrogenicity of a novel 3-dimensional printed orthodontic aligner. Am. J. Orthod. Dentofac. Orthop. 162 (3), e116–e122. doi:10.1016/j.ajodo.2022.06.014
Quinzi, V., Gallusi, G., Carli, E., Pepe, F., Rastelli, E., and Tecco, S. (2022). Elastodontic devices in orthodontics: an in-vitro study on mechanical deformation under loading. Bioeng. (Basel) 9 (7), 282. doi:10.3390/bioengineering9070282
Rajasekaran, A., and Chaudhari, P. K. (2023). Integrated manufacturing of direct 3D-printed clear aligners. Pap. Present. A. T. Front. Dent. Med. 3. doi:10.3389/fdmed.2022.1089627
Rajput, A. S., Patil, A., Das, M., and Kapil, S. (2023). Post processing of low cost Aligners fabricated by additive manufacturing process to enhance the surface quality and functionality. J. Mech. Behav. Biomed. Mater 144, 106003. doi:10.1016/j.jmbbm.2023.106003
Raszewski, Z., Chojnacka, K., Kulbacka, J., and Mikulewicz, M. (2022). Mechanical properties and biocompatibility of 3D printing acrylic material with bioactive components. J. Funct. Biomater. 14 (1), 13. doi:10.3390/jfb14010013
Reymus, M., Lümkemann, N., and Stawarczyk, B. (2019). 3D-printed material for temporary restorations: impact of print layer thickness and post-curing method on degree of conversion. Int. J. Comput. Dent. 22 (3), 231–237.
Rossini, G., Parrini, S., Castroflorio, T., Deregibus, A., and Debernardi, C. L. (2015). Periodontal health during clear aligners treatment: a systematic review. Eur. J. Orthod. 37 (5), 539–543. doi:10.1093/ejo/cju083
Ryu, J. H., Kwon, J. S., Jiang, H. B., Cha, J. Y., and Kim, K. M. (2018). Effects of thermoforming on the physical and mechanical properties of thermoplastic materials for transparent orthodontic aligners. Korean J. Orthod. 48 (5), 316–325. doi:10.4041/kjod.2018.48.5.316
Shannon, T., and Groth, C. (2021). Be your own manufacturer: 3D printing intraoral appliances. Seminars Orthod. 27 (3), 184–188. doi:10.1053/j.sodo.2021.09.004
Sheridan, J. J., LeDoux, W., and McMinn, R. (1993). Essix retainers: fabrication and supervision for permanent retention. J. Clin. Orthod. 27 (1), 37–45.
Shirey, N., Mendonca, G., Groth, C., and Kim-Berman, H. (2023). Comparison of mechanical properties of 3-dimensional printed and thermoformed orthodontic aligners. Am. J. Orthod. Dentofac. Orthop. 163 (5), 720–728. doi:10.1016/j.ajodo.2022.12.008
Šimunović, L., Jurela, A., Sudarević, K., Bačić, I., Haramina, T., and Meštrović, S. (2023). Influence of post-processing on the degree of conversion and mechanical properties of 3D-printed polyurethane aligners. Polym. (Basel) 16 (1), 17. doi:10.3390/polym16010017
Subramanian, A. K., and Harikrishnan, S. (2023). 3D printing in orthodontics: a narrative review. J. Int. Oral Health 15, 15. doi:10.4103/jioh.jioh_83_22
Suganna, M., Kausher, H., Tarek Ahmed, S., Sultan Alharbi, H., Faraj Alsubaie, B., Ds, A., et al. (2022). Contemporary evidence of CAD-CAM in dentistry: a systematic review. Cureus 14 (11), e31687. doi:10.7759/cureus.31687
Sycińska-Dziarnowska, M., Szyszka-Sommerfeld, L., Woźniak, K., Lindauer, S. J., and Spagnuolo, G. (2022). Predicting interest in orthodontic aligners: a google trends data analysis. Int. J. Environ. Res. Public Health 19 (5), 3105. doi:10.3390/ijerph19053105
Tamburrino, F., D'Antò, V., Bucci, R., Alessandri-Bonetti, G., Barone, S., and Razionale, A. V. (2020). Mechanical properties of thermoplastic polymers for aligner manufacturing: in vitro study. Dent. J. (Basel) 8 (2), 47. doi:10.3390/dj8020047
Tartaglia, G. M., Mapelli, A., Maspero, C., Santaniello, T., Serafin, M., Farronato, M., et al. (2021). Direct 3D printing of clear orthodontic aligners: current state and future possibilities. Materials 14 (7), 1799. doi:10.3390/ma14071799
Tian, Y., Chen, C., Xu, X., Wang, J., Hou, X., Li, K., et al. (2021). A review of 3D printing in dentistry: technologies, affecting factors, and applications. Scanning 2021, 9950131. doi:10.1155/2021/9950131
Tsoukala, E., Lyros, I., Tsolakis, A. I., Maroulakos, M. P., and Tsolakis, I. A. (2023). Direct 3D-printed orthodontic retainers. A systematic review. Child. (Basel) 10 (4), 676. doi:10.3390/children10040676
Uzunov, T., Yordanova-Ignatova, T., Grozdanova-Uzunova, R. G., Stoyanova, N., Stoev, Y., Apostolov, N., et al. (2023). Optical properties of 3D printed orthodontic retainers. J. Phys. Conf. Ser. 2487, 012020. doi:10.1088/1742-6596/2487/1/012020
Venezia, P., Ronsivalle, V., Rustico, L., Barbato, E., Leonardi, R., and Lo Giudice, A. (2022). Accuracy of orthodontic models prototyped for clear aligners therapy: a 3D imaging analysis comparing different market segments 3D printing protocols. J. Dent. 124, 104212. doi:10.1016/j.jdent.2022.104212
Willi, A., Patcas, R., Zervou, S. K., Panayi, N., Schätzle, M., Eliades, G., et al. (2023). Leaching from a 3D-printed aligner resin. Eur. J. Orthod. 45 (3), 244–249. doi:10.1093/ejo/cjac056
Williams, A., Bencharit, S., Yang, I. H., Stilianoudakis, S. C., Carrico, C. K., and Tüfekçi, E. (2022). Effect of print angulation on the accuracy and precision of 3D-printed orthodontic retainers. Am. J. Orthod. Dentofac. Orthop. 161 (1), 133–139. doi:10.1016/j.ajodo.2021.01.020
Wu, E. (2022). The application of in-office aligners in the combination treatment protocol. Seminars Orthod. 28 (2), 85–91. doi:10.1053/j.sodo.2022.10.004
Xia, Y., He, Y., Zhang, F., Liu, Y., and Leng, J. (2021). A review of shape memory polymers and composites: mechanisms, materials, and applications. Adv. Mater 33 (6), e2000713. doi:10.1002/adma.202000713
Zheng, M., Liu, R., Ni, Z., and Yu, Z. (2017). Efficiency, effectiveness and treatment stability of clear aligners: a systematic review and meta-analysis. Orthod. Craniofac Res. 20 (3), 127–133. doi:10.1111/ocr.12177
Keywords: clear aligners, 3D-printing, orthodontics, composite resins, biocompatible materials
Citation: Niu C, Li D, Zhang Y, Wang Y, Ning S, Zhao G, Ye Z, Kong Y and Yang D (2024) Prospects for 3D-printing of clear aligners—a narrative review. Front. Mater. 11:1438660. doi: 10.3389/fmats.2024.1438660
Received: 26 May 2024; Accepted: 08 July 2024;
Published: 30 July 2024.
Edited by:
Roger Narayan, University of North Carolina at Chapel Hill, United StatesReviewed by:
Evangelia Vouvoudi, Aristotle University of Thessaloniki, GreeceKi Kim, Saint Louis University, United States
Antonino Lo Giudice, University of Catania, Italy
Stefano Pagano, University of Perugia, Italy
Copyright © 2024 Niu, Li, Zhang, Wang, Ning, Zhao, Ye, Kong and Yang. This is an open-access article distributed under the terms of the Creative Commons Attribution License (CC BY). The use, distribution or reproduction in other forums is permitted, provided the original author(s) and the copyright owner(s) are credited and that the original publication in this journal is cited, in accordance with accepted academic practice. No use, distribution or reproduction is permitted which does not comply with these terms.
*Correspondence: Donghong Yang, eWFuZ2Rvbmdob25nQGptc3UuZWR1LmNu Yu Kong, MzU2MjQyODI3NEBxcS5jb20=;
†These authors have contributed equally to this work and share first authorship