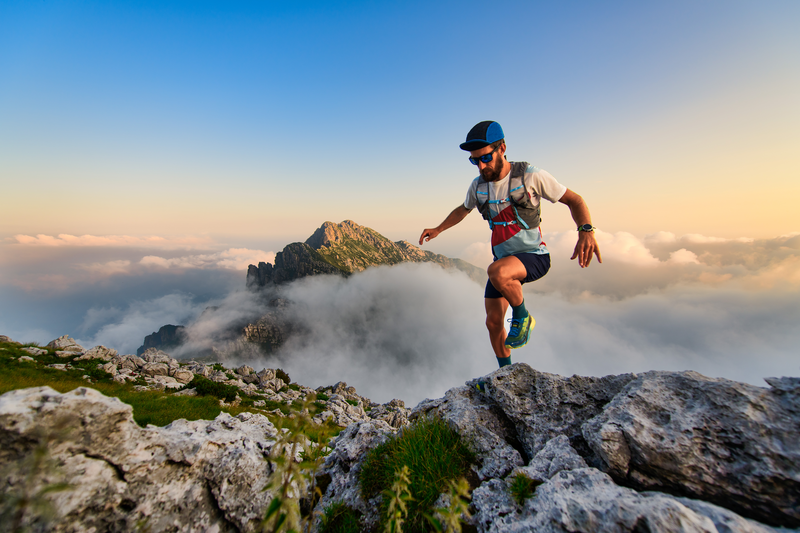
95% of researchers rate our articles as excellent or good
Learn more about the work of our research integrity team to safeguard the quality of each article we publish.
Find out more
REVIEW article
Front. Mater. , 26 August 2024
Sec. Carbon-Based Materials
Volume 11 - 2024 | https://doi.org/10.3389/fmats.2024.1432704
This article is part of the Research Topic Biomass Conversion and Biomass-Derived Carbon-Based Materials for Remediation of Emerging Pollutants in Soil and Water View all 5 articles
Wastewaters consist of organic pollutants that have environmental concerns. Wastewaters are treated by different methods, but efficient, low-cost, and sustainable techniques still need to be developed. Algae-based water pollution remediation techniques are considered to be sustainable approaches. This review exclusively discusses the facets of macro and microalgae in the treatment of organic toxicants. The current trends of algae-mediated water treatments have been discussed under adsorption and degradation methods. A focus on algae fuel cell, algae mediated activation of oxidizing agents, Fenton-like reactions, and photocatalysis was given. The need of algae-based adsorptive and catalytic materials was mentioned. The role of algae in the synthesis of catalysts which were employed in pollutant removal methods was also explained. The integrated algae-mediated water treatment techniques were also highlighted. The toxicant removal performances of different algae-based materials in the water medium were summarized. The conclusion and future prospects derived from the literature survey were described. This review will be helpful for researchers who are working in the field of sustainable water pollution remediation.
Natural water ecosystems such as lake (Luarte et al., 2022), river (Qin et al., 2019) and sea (Sanganyado et al., 2021) are seriously polluted owing to the discharge of multiple kinds of chemical pollutants including inorganic species and organic compounds. For instance, the existence of 22 kinds of volatile organic compounds in Poyang lake water (sampling points = 28) was identified (Qin et al., 2019). Methylene chloride was found to be a predominant pollutant (708.19 ng L−1) in this study. Another study has found that the organo-chlorine (polychlorinated biphenyls) and organo-bromo (polybrominated diphenyl ethers) compounds are present (used European eel as indicator) in Rasa River, Croatia (Kljakovic-Gaspic et al., 2023). Jiang et al. (2023) have determined the presence of phenolic compounds, polycyclic aromatic hydrocarbon and organochlorine compounds in the Yongding river basin (sampling points = 15). The composition and concentration of organic pollutants are found to be different in different regions of the Yongding river basin. The occurrence of organochlorine compounds in Mediterranean Sea water were also reported by using deep-sea organisms (Chondrichthyes) (Consales et al., 2023). Organic pollutants occur in surface and ground waters by direct discharge of industrial effluents (Singh et al., 2022), urbanization, wastewater treatment plants (Jiang et al., 2023), grey water irrigation (Turner et al., 2019) and other human activities (Li et al., 2021). An illustration of pollution of water ecosystems is given as Figure 1. Harmful organic pollutants such as antibiotics, pesticides, phenolics compounds and azo dyes show harmful effects including stroke, carcinogenicity, allergies, reproductive, neurological, and behavioral effects (Dev et al., 2023; Monisha et al., 2023). Hence, it is essential to remove those harmful pollutants from the water. But it is not an easy procedure owing to good stability and recalcitrant behaviors of these pollutants.
Several water treatment techniques such as biological, physical (evaporation, filtration, and reverse osmosis), and chemical (precipitation, catalytic oxidation and reduction, electrochemical techniques, ion-exchange) methods were established in recent decades (Rai et al., 2022). Traditional water treatment techniques consist of multiple stages to eliminate organic pollutants, and these techniques need huge space, energy and capital expenditure. Expensive chemicals are employed for different purposes including oxidizing reagents, precipitating agents, and catalysts. These undesirable features urge the researchers to develop more efficient and inexpensive techniques to treat organic pollutants in water. Industries also need sustainable and eco-friendly techniques to treat their effluents. It should be also mentioned that the United Nations Sustainable Goal 6 regards the access of safe drinking water by all (United Nations, 2024). It is possible by decreasing water pollution through sustainable water treatment techniques.
Microbes such as bacteria, algae, and fungi were applied in different water treatment techniques for the elimination of toxic organic pollutants from water medium (Rios-Miguel et al., 2023). Particularly, research studies on algae-mediated water treatment methods for organic pollution remediation have increased considerably during the last decade (from 2014 to 2024). According to the Scopus search (as on June, 2024), by using a keyword “algae + organic + water treatment”, the number of research articles (year of publications is mentioned within the bracket) are found as 53 (2010), 93 (2014), 174 (2018), 189 (2022) and 145 (June, 2024). Reviews on algae-mediated water treatments were published by different research groups. Specifically, algae-based adsorption, bioaccumulation, and degradation of wastewater containing chemical pollutants were discussed in a review written by Bhatt et al., 2022. Martínez-Ruiz et al., 2022 have focused on microalgae-mediated remediation methods for the treatment of landfill leachate in their review article. In addition, the simultaneous generation of value-added products including fuel, plastics, and soil enhancers has also been discussed. A review, written by Deka et al., 2022 has been focused on algae-mediated microbial fuel cells developed for the degradation of toxic organic dyes. Khan et al., 2022 have performed a literature survey on the microalgal-derived biochars with their dyes and heavy metals removal performances (biosorption). These review articles either focused on a particular algae-mediated water treatment technique or algae-derived materials. A review focusing on the application of algae and algae-based materials in different water treatment techniques for organic decontamination is rare.
This review aims to explore the application of macro and microalgae in the treatment of water containing harmful organic toxicants including dyes, antibiotics and pesticides. Particularly, algae-mediated adsorption and catalytic degradation methods were targeted. Bare algae, modified and algae derived materials were particularly considered. The role of algae in different water treatments was highlighted. Future perspectives for algae-based water treatments were further proposed.
Algae are eukaryotic or prokaryotic (autotrophic) organisms that are generally classified into microalgae, and macroalgae based on their cellular types. They live in water environments, including ponds, lakes, rivers, oceans and wastewaters (Salman et al., 2023). Macroalgae are multicellular organisms, whereas microalgae are unicellular organisms. The lignin content of microalgae is low, and hence it can be treated by facile pre-treatment techniques. Microalgae can boom in effluents including industrial, domestic and salt water, since they utilize harmful inorganic and organic pollutants as nutrients for their growth. Microalgae also grow much faster and thus their availability is huge to harvest. Algae are used in food, health supplements, pharmaceuticals, energy storage, cosmetics, and biofuels (Biris-Dorhoi et al., 2020; Al-Ghamdi et al., 2023; Fernandez et al., 2023). The major components are fat, proteins, ash, lipids, and carbohydrates (Babich et al., 2022). The chemical composition of micro and macro is remarkably varied. Figure 2 displays the key advantages of algae which make them potential in water treatments. Photosynthesis ability and assimilation tendency to utilize organic pollutants (as a carbon source) made them efficient to apply in water treatment (Cuellar-Bermudez et al., 2017). Algae biomass possesses different surface functional groups which will be helpful to eliminate organic pollutants as well as inorganic contaminants (Bhatt et al., 2022). Algae can be cultivated in a wide range of aquatic environments with higher yields, and thus their availability is promising (Singh et al., 2021; van der Meer et al., 2023). Adsorbents derived from algae are possessing high porosity, stability, and functional groups which are vastly needed features of an adsorbent (Khan et al., 2022). Dead algae biomass can also be used for the decontamination of polluted waters. The utilization of dead algae is beneficial since they are not affected by the toxicity of the pollutants.
Algae have been potentially used in various techniques to remove organic contaminants from water. In this section, algae-mediated adsorption and catalytic degradation methods were particularly discussed (Figure 3).
The biomass of algae has suitable features to adsorb harmful organic as well as inorganic entities from their aqueous solutions (biosorption). Biosorption is a cheap, effective, and high-performance method for wastewater treatment (Ramesh et al., 2023). The algae cells consist of various functional groups like amino (-NH2), carboxyl (-COOH), and hydroxyl (-OH), which are responsible for algae’s sorption ability in water medium (Khan et al., 2022). There is no need to supply nutrients and maintain special conditions for dead algae biomass. Adsorption can occur between pollutants and the pre-treated algae biomass via chelation/complexation, ion exchange, or hydrogen bonding between pollutants. The following pretreatment is required to have algae biosorbent: First, algae are collected from natural or industrial sites, washed with plenty of potable water (to abolish extraneous particles), cleaned with pure water, dried (under natural sunlight or in a hot air oven), milled and then sieved to achieve the desired particle size (Mokhtar et al., 2017). The drying process may take some hours to a few days (Mokhtar et al., 2017; Elgarahy et al., 2023). Different algae species have been harvested and employed as sorbent for capturing harmful organic molecules in simulated contaminated water (Table 1).
The inferences from the Table 1 are as follows: Algae biomass can be obtained from natural environments, including coastal regions and ponds, and is also possible to cultivate in laboratories (Gul et al., 2019; Tukarambai, 2022). The biosorption capacity of a particular algal species is dependent on the molecular structure of organic pollutants. For instance, Ulva fasciata adsorbent showed different adsorption capacity towards rhodamine B and methyl orange dyes in water (Elgarahy et al., 2023). Similarly, Codiumd decorticatum based adsorbent has shown difference in the adsorption capacity of crystal violet and Congo red dyes under the same conditions (Oualid et al., 2020).
Algae biosorbent’s performance can be further enhanced by applying ultrasonic treatment. By employing ultrasonic wave illumination (frequency = 35 kHz), the U. fasciata adsorbent could adsorb rhodamine B and methyl orange from water within 30 min, while the maximum adsorption equilibrium time without ultrasonic treatment is 180 min (Elgarahy et al., 2023). Algae adsorbents remove organic pollutant molecules via binding modes like hydrogen bonding, ionic attraction, and n-π stacking (Abdulhameed et al., 2022; Yadav et al., 2022; Fontoura et al., 2017). The biosorption performance is dependent on the type of algae. An example, Mokhtar et al. (2017) have used red (K. striatum, K. alverezii and E. spinosum), green (C. lentillifera), and brown (S. polycystum) algae as adsorbents for capturing methylene blue from water medium. Among others, E. spinosum showed the best methylene blue adsorption performance.
Algae like brown algae have water soluble organic compounds (carbohydrates, and pigments) that dissolve in water during biosorption (Bulgariu and Bulgariu, 2016). It causes secondary pollution and thus it is essential to modify the algae biomass (Rocha de Freitas et al., 2018). Generally, acid, salt, and organic molecules have been used for the modification process, thereby decreasing organic leaching from algae biomass. Further addition of chemical compounds has also been attempted (Bulgariu and Bulgariu, 2016). For example, Cobas et al. (2014) have pretreated the F. vesiculosus by using a solution of calcium chloride (CaCl2), potassium chloride (KCl), or magnesium chloride (MgCl2) (algae:salt solution = 2.5:50). The pre-treated algal was used for the treatment of Sella solid blue, Sella solid orange, burdeuox, and special violet (leather dyes). About 88% of targeted dyes have been eliminated by 0.84 M CaCl2-treated F. vesiculosus sorbent (pH = 5.69 and algae dosage = 1.37 g). Lebron et al. (2019) conducted a research study in which methylene blue was removed from water by using modified Fucus vesiculosus, C. pyrenoidosa and Spirulina maxima, separately as adsorbents. Zinc chloride (ZnCl2) and phosphoric acid (H3PO4, 85%) were used as modifying agents, whereas the time and temperature for the chemical modification process are 24 h and 28°C respectively. This modification step causes the formation of oxygen-containing chemical moieties (carboxylic groups) on the surface of algae biomass. The carboxylic group has pKa = 4.5, and thus it will be in an anionic state (deprotonated form), which can interact with the cationic methylene blue molecule effectively. Furthermore, the thermal stability of algae has also been improved. The H3PO4-modified F. vesiculosus showed adsorption capacity of 1,162.9 mg g−1 (equilibrium time = 40 min). In this study, the biosorbent’ dosage, equilibrium time, and pH of the medium were 2 g L−1, 4 h, and six, respectively. In another study, 96% sulfuric acid-treated Laminaria japonica (a brown alga) was applied as a biosorbent in the adsorptive elimination of methylene blue from its solution (Shao et al., 2017). Sulfuric acid treatment leads to the formation of lactonic groups via the reaction between amino acids and sodium alginate. At the optimized experimental conditions (biosorbent = 0.6 g L−1; pH = 6; contact time = 60 min; temperature = 308 K), acid treated L. japonica biosorbent exhibited the highest methylene blue adsorption capacity of 549.45 mg g−1, while untreated L. japonica biosorbent showed 418.41 mg g−1. The mode of methylene blue interaction with biosorbent was determined to be complex formation and ionic attraction.
Alkali treated algae biosorbents have also been reported. For instance, Ulva Lactuca treated with calcined eggshell powder has been prepared for the separation of cationic (methylene blue) and anionic (Congo red) dye molecules from their mixture solution (Qulatein and Yilmaz, 2023). Also, when compared to untreated Ulva Lactuca, calcined eggshell-treated Ulva Lactuca presented a maximum (methylene blue) adsorption capacity of 2000 mg g−1 (Langmuir isotherm) within 15 min. The adsorption of methylene blue and Congo red is effective in alkaline and acidic conditions, respectively. This study also reported that the adsorption of methylene blue involved ionic interaction and intermolecular hydrogen bonding, whereas Congo red’s adsorption was attributed to hydrogen bonding that happened between the hydroxyl and the amine (–NH2) groups.
Algae doped iron (II, III) oxide (Fe3O4)/chitosan composite was synthesized via a two-step approach, and the resultant biocomposite has been used to adsorb Direct Red 31 and Direct Red 28 from water (Solanki et al., 2022). About 94% of Direct Red 31 removal efficiency was reported when the biocomposite dosage, contact time, and initial pH is 0.6 g L−1, 10 min, and 5, respectively. In the case of Direct Red 28, 90% removal efficiency was obtained under the following conditions: biocomposite dosage = 7 g L−1; adsorption time = 20 min; and pH = 3. A copper (Cu)-benzenetricarboxylic acid/sulphated U. fasciata composite (specific surface area = 950 m2 g−1) was constructed for the selective adsorption of methyl orange and methylene blue in an aqueous solution (Abdelhameed et al., 2020). Within 10 min, the adsorptive removal efficiency toward methylene blue and methyl orange was 97% and 68%, respectively. By following the Langmuir model, the adsorption capacity obtained for methylene blue and methyl orange is 162 and 72 mg g−1, respectively. An effective capturing of methylene blue by this composite adsorbent was determined by electrostatic attraction.
Carbon-based adsorbents were synthesized using algae biomass, since it is possible to harvest more algal biomass per unit volume (Chen et al., 2022). Biochar synthesised from algal biomass has shown good activity in wastewater treatment. For example, phycocyanin-extracted algal bloom biomass was utilized to synthesize hydrochar via hydrothermal carbonization (Zhang et al., 2017). According to Langmuir’s isotherm, the obtained hydrochar (4 g L−1), displayed a maximum malachite green (100 mg L−1) adsorption capability of 89.05 mg g−1. The functional groups present in hydrochar are actively participating in the adsorption of malachite green. Further, Caulerpa scalpelliformis-derived biochar was applied in the adsorption of remazol dyes from the water (Gokulan et al., 2019). The optimum adsorbent dosage, pH, dye concentration, and temperature are reported as 2 g L−1, 2, 0.05 mmol L−1 and 30°C, respectively. The order of adsorption efficiency of biochar derived from C. scalpelliformis is Remazol brilliant orange 3R > Remazol brilliant blue R > Remazol brilliant violet 5R > Remazol black B. On the other hand, Undaria pinnatifida dried powder (Yao et al., 2020) was used to synthesize activated biochar [activating agent = potassium hydroxide (KOH)] and used in the adsorption of few selected dye molecules. The surface area and functional groups of synthesised biochar is 1,156.25 m2 g−1 (volume = 0.67 cm3 g−1) and hydroxyl/carbonyl, respectively. The adsorption capacities obtained for activated biochar towards malachite green, methylene blue, and rhodamine B is 4066.96, 841.64, and 533.77 mg g−1 respectively. Diffusion, van der Waals attraction, hydrogen bonding, π-π stacking and electrostatic attraction are the major adsorption modes on the biochar adsorbent.
The activated carbon was obtained from Sargassum oligocystum at a carbonization temperature of 500°C (3 h) (Foroutan et al., 2019). The obtained activated carbon was coupled with Fe3O4 nanoparticles, and the resultant composite adsorbent has been applied for methylene blue and methyl violet elimination. The adsorbent dosage, contact time, ultrasonic frequency, and pH of this adsorption experiment were followed as 0.15 g/100 mL, 50 min, 45 kHz, and 7, respectively. The determined adsorption capacities of this composite adsorbent toward methylene blue and methyl violet are 60.60 and 59.88 mg g−1 (Freundlich isotherm), respectively.
The biochar was synthesised by using a mixer (weight ratio = 1:2) of algal biomass (major stains = Spirulina sp., Chlorella sp., Synechocystis sp., and Scenedesmus sp.,) and a kombucha-symbiotic culture of bacteria and yeast (Pathy et al., 2022). The carbonization temperature used for composite synthesis is 500°C (45 min). About 500 mg g−1 of malachite green adsorption capacity was reported for the composite adsorbent.
The assimilation tendency of algae towards the dissolved chemical pollutants in water is the basis for algae-mediated pollutants’ degradation methods (Rai et al., 2022; Satya et al., 2023). Generally, the live algal cells are added to polluted water and kept for certain days under optimized conditions. The algae absorb the organic pollutants from the water via their cell walls and use them as nutrients for their growth (Bhatt et al., 2022). The absorbed organic pollutants are converted into simple molecules via different metabolic pathways, thereby giving polluted water its cleanliness (Bhatt et al., 2022). The removal of pollutants can be monitored by withdrawing solution from the reactor and subjecting it to analysis using analytical methods such as UV-visible spectroscopy and chromatographic techniques (Moradi et al., 2021; Hu et al., 2021). Different harmful organic pollutants were degraded by different algae species. For example, in order to treat atrazine (C8H14ClN5) aqueous solution, Chlorella sp. has been used by Hu et al., 2021. Chlorella sp. culture was exposed for 8 days to pure atrazine and atrazine treated solutions (40 and 80 μg L−1). Atrazine solution was initially treated by (0.1 g L−1) titanium dioxide (TiO2)-mediated photocatalytic process for 60 min under an ultraviolet lamp (250 W). The removal efficiency in 40 and 80 μg L−1 of atrazine solutions was determined to be 83.0% and 64.3%, respectively. The removal efficiency of a pure atrazine solution has been found to be higher than that of a prior degraded atrazine solution. This study has found that the atrazine’ degradation products inhibited the photosynthetic ability of Chlorella sp. by suppressing light harvesting. On the one hand, the direct green 6 (C34H22N8Na2O10S2) dye molecules were degraded by live and dead cells of Spirulina platensis (Moradi et al., 2021). Compared to dead cells, live S. platensis cells have the possibility of being re-used for the degradation of direct green 6 dye with no loss of activity. In addition, the generation of valuable products like polyhydroxybutyrate is also enhanced.
It has been explored that the addition of redox mediators (organic compounds) further accelerates the algae-mediated degradation process. Meng et al., 2014 have determined that the acid red 27 (C20H11N2Na3O10S3) decolorization (reduction) efficiency of Shewanella algae was improved by anthraquinone-2,6-disulfonate or humic acids as redox mediators, even in saline conditions. It should be mentioned that the addition of chemicals (redox mediators) may increase the cost of the treatment method. Algae have also been coupled with other microorganisms like bacteria to explore the sustainable water treatment technique (Khoo et al., 2021). A synergistic effect was observed when algae coupled with microbes, thereby boosting the degradation performance (protocooperation). As an example, a mixture of Chlorella sorokiniana XJK (algae) and Aspergillus sp. XJ-2 (fungus) has been used for the degradation of disperse red 3B, and the degradation process gave low toxic degradation products (Tang et al., 2019). About 98.09% of the degradation rate was obtained for this consortium. The participation of manganese peroxidase and lignin peroxidase in the degradation of disperse red 3B has been confirmed. Li et al. (2022) have also investigated the performance of an algae (Chlorella) and bacteria consortium in the decolorization of simulated coloured (disperse red 3B) water (pH = 7.8). The degradation efficiency and chemical oxygen demand (COD) removal efficiency of the algae-bacteria consortium were reported as 90% and 91%, respectively. Algae particularly degraded the dye molecules, whereas the degradation by-products were further decomposed by bacteria.
The capability of algae to grow in different wastewaters makes it possible to utilize them in fuel cells. Algae fuel cells are used for generating electricity and instantaneous treatment of polluted water (Deka et al., 2022). In an algal fuel cell, the anodic compartment consists of organic compounds (pollutants) which will be oxidized by biofilm that attached to the anode and produce electrons and protons. Both electrons and protons travel through the algal cathode by means of circuit connection and an ion selective membrane, respectively. At the cathode, protons are reduced to water in the presence of oxygen molecules (Figure 4) (Deka et al., 2022).
Researchers have employed various industrial wastewaters in algae fuel cells for effective treatment. In a study, landfill leachate diluted with domestic wastewater was taken into an algae/carbon fibre brush cathode chamber, whereas the anode chamber was filled by domestic wastewater added with sodium acetate (CH3COONa) (Nguyen et al., 2017). The reported COD removal at the anode and cathode chambers is 97% and 52% (10% leachate), respectively. In addition, algae growth at the cathode chamber also favoured the successful elimination of ammonium (98.7% ± 1.8% for 10% leachate, 5 days) and phosphorus (80.28% for 5% leachate, 5 days) in highly diluted landfill leachate. But it has been determined that the COD removal efficiency was decreased by adding a high volume of landfill leachate. Noticeably, the highest potential of this fuel cell was 300 mV, when the leachate concentration is 5%. On the other hand, the photo-cathode algal fuel cell containing Chlorella vulgaris was used to treat wastewater and generate electricity concurrently (Commault et al., 2017). The maximum removal rate of COD and ammonium is 0.19 g L−1 d−1 and 5 mg L−1 d−1, whereas the highest power density was reported as 34.2 ± 10.0 mW m−2 (without C. vulgaris = 15.6 ± 9.7 mW m−2).
Instead of a single fuel cell, an integrated algal fuel cell has also been proposed for efficient water treatment. A microbial fuel cell integrated with algae biofilm (Scenedesmus quadricauda SDEC-8) has been constructed by Yang et al., 2018 to remove nutrients (nitrogen, phosphorous) and COD from domestic wastewater and produce electricity simultaneously. The total nitrogen, phosphorous, and COD removal efficiency is 96.0%, 91.5%, and 80.2%, respectively. The performance (power density = 62.93 mW m−2) of this integrated device is found to be better than that of a sole microbial fuel cell or algae biofilm. Hou et al., 2020 have coupled an anaerobic digestion tank (as anodic chamber) with algae-assisted microbial fuel cell to treat food wastes which consist of food preparation leftovers as well as uneaten food. The algae and its concentration were Golenkinia sp. SDEC-16 and 150 mg L−1, respectively. About 89% COD removal rate was observed for this coupled technology. In order to treat mariculture effluent (antibiotics, phosphate, and heavy metals), a constructed wetland–microbial fuel cells/electroactive bacteria–algae biofilm/siphon aeration technology was developed by Wang T. et al. (2023). By this integrated technology, about 97.38% ± 3.62% sulfamethoxazole and 80.99% ± 1.3% chemical oxygen demand removal efficiencies were obtained (voltage = of 354 ± 23 mV).
Recently, Yang et al. (2021) have fabricated a triple-chamber fuel cell that is composed of two cathodic and one anodic compartment for the elimination of Cu2+ ions, nitrogen, COD from synthetic wastewater along with electricity production. The removal efficiency reported for Cu2+ ions, nitrogen, and COD is 99.9%, 79%, and 84.1%, respectively. Algae takes the ammonium, while the as-formed nitrogen oxides are removed in the dark by the cathode. The maximum power density achieved by this fuel cell was 420 mW m−2.
It should be mentioned that the algae mediated fuel cells and its integrated technologies not only remove pollutants/wastes but also showed considerable advances in the production of electrical energy (Mahmoud et al., 2022).
Algae have been used as carbon sources, thereby synthesizing different carbon-based catalysts. The performances of algae-derived catalysts in the degradation of organic pollutants in water were given in Table 2.
Table 2. Algae derived nanoparticles and their catalysts performance in the removal organic pollutants.
Table 2 clearly reveals that the alga-derived carbon and nanomaterial-based catalysts perform better in catalytic oxidation (activation of peroxymonosulfate and peroxydisulfate species), Fenton-like processes, and photocatalysis. The degradation mechanisms were highlighted below:
(a). Activation of oxidant: A persulfate activation mediated decomposition of tetracycline in presence of FeOX (Fe0 + iron oxide)@Z700 (algae-derived biochar) composite was explained as follows: FeOX@Z700 accelerated the cleavage of O–O bond in persulfate (S2O82−), and subsequent production of O2•−/SO4•−radicals which led to the decomposition of tetracycline (Yin et al., 2023a). This study has reported that the Fe0/Fe2+ system played a key role in the production of O2•− and SO4•−radicals (Equations 1–5) (Yin et al., 2023a).
(b). Fenton-like reaction mechanism was reported for iron (III) oxide (Fe2O3)/Taihu blue algae-derived activated biochar composite catalyst in the methylene blue degradation reaction (Mi et al., 2023). Under the catalytic reaction conditions, the functional groups present in the biochar and metal sites favoured the production of persistent free radicals (carbon-centered free radicals) which led the Fe(III)-complex formation, and subsequently generated Fe(II) ions. Hydroxyl radicals were efficiently generated by Fe(II) ions via the hydrogen peroxide (H2O2) decomposition. Thus, the generated hydroxyl radicals effectively decomposed the methylene blue.
(c). The photocatalytic degradation pathway of malachite green by cobalt (Co)-doped zinc oxide (ZnO)/algae/visible light system was described by the following manner (Rabie et al., 2020): The incident visible light photons induced the creation of holes and electron which are responsible for degradation of malachite green (Equations 6–9).
Alternatively, algae-derived carbon catalysts were also modified with suitable reagents to enhance their catalytic activity. Recently, Spirulina biomass has been used to synthesize potassium permanganate (KMnO4)-modified biochar, which was employed in the catalytic oxidation of malachite green in water (Zhu and Zou, 2022). The modified biochar has a porous structure (pore diameter = 22.596 nm) with a surface area of 2.766 m2 g−1 and showed remarkable catalytic activity in the presence of 5 mmol L−1 H2O2 towards the oxidation of malachite green. The malachite green oxidation efficiency (8000 mg L−1) was found to be 99% within the reaction time of 30 min.
Nanomaterials have a relatively larger surface area, tunable surface groups, and charges, which are desirable for good catalysts (Nasrollahzadeh et al., 2021). Extracts of algae have been extensively used to synthesize nanomaterials, including metals and metal compounds (Khan et al., 2022). The major role of algae extract is as a complexing/reducing agent and capping agent to yield nanoparticles with high stability. Algal extracts contain fats, proteins, carbohydrates, and polyphenols that are accountable for the reduction and stabilization of nanoparticles. The general procedure involved in the algae-extract assisted synthesis of nanomaterials is illustrated in Figure 5.
Figure 5. General procedure for synthesis of metal/metal compound nanoparticles assisted by algae sources.
Some examples of nanocatalysts prepared by using algae extracts were discussed below. Silver (Ag0) nanospheres with particle size of 10 ± 2 nm have been prepared by using Caulerpa serrulate (Aboelfetoh et al., 2017). The catalytic potential of silver nanospheres was tested in the reduction of Congo red molecules in water. The catalytic efficiency of silver nanospheres prepared at 95°C is 99.3% (time = 12 min). An aqueous extract of Saragassum cervicorne was prepared at 85°C–90°C (1 h) and used to prepare palladium (Pd0) nanoparticles via a reduction process (Anwar et al., 2021). Extracts of Saragassum cervicorne have been used as an alginate source that reduces palladium ions into palladium nanoparticles. The as-prepared palladium nanoparticles were used as a catalyst for the reduction of Congo red, methyl red, and methyl orange dyes in water. The catalytic reduction efficiency reported for methyl orange (catalyst dosage = 60 μg μL−1), Congo red (catalyst dosage = 40 μg μL−1), methyl red (catalyst dosage = 80 μg μL−1) is 99.66% (10 min), 99.25% (9 min), and 95.45% (10 min), respectively. Recently, bimetallic nanoparticles were also prepared efficiently using algae extract. The silver/palladium (ratio = 1:1) bimetallic nanoparticles with greater ultraviolet light triggered photocatalytic degradation efficiency in the Congo red dye degradation than the monometallic nanoparticles have been demonstrated (Al-Enazi, 2022). The algae extract employed to prepare mono and bimetallic nanoparticles is Bacillariophyceae. An extract was prepared by boiling a suspension containing 1 g of Bacillariophyceae powder in 100 mL of double distilled water at 60°C for 30 min.
Apart from metallic nanoparticles, metal oxides like copper oxide (CuO) and ZnO with high catalytic activities have been synthesized. For instance, CuO crystalline nanoparticles were prepared using Cystoseira trinodis extracts along with ultrasonic irradiation (Gu et al., 2018). The frequency and power of ultrasonic waves are 20 kH and 400 W, respectively. The CuO nanoparticles (dosage = 50 mg) have been applied as a photocatalyst in the degradation of methylene blue (5 mg L−1, 100 mL) in water. Under ultraviolet irradiation, the photocatalytic efficiency was 98%. The ZnO nanoparticles (20 ± 2.2 nm) were prepared by using Chlorella extract (Khalafi et al., 2019). The role of Chlorella extract as a reducing as well as stabilizing agent was confirmed through Fourier transform infrared spectroscopic analysis. Hydroxyl groups in the algae reduce zinc ions (Zn2+) into elemental zinc (Zn0) nanoparticles, which subsequently undergo oxidation during the air-drying stage to form ZnO nanoparticles. Aggregation of ZnO nanoparticles would be avoided by biomolecules present in the algae extract. Chlorella extract-assisted synthesis ZnO nanoparticles display 97% photocatalytic dibenzothiophene degradation efficiency. The concentrations of dibenzothiophene and ZnO nanoparticles is 10 mg L−1 and 0.01 g, respectively. Copper sulfide (CuS) nanoparticles were synthesized by using Chlorella ellipsoidea extract which acted as a capping and reducing reagent (Borah et al., 2022). CuS nanoparticles have been utilized as an efficient sunlight-active photocatalyst for the (1 mL, 1 mM) sodium borohydride (NaBH4)-mediated degradation of methylene blue in aqueous solution (25 mL, 1 × 10−4 M), and the reported rate constant is 0.03692 min−1 (catalyst dosage = 1 mg).
It should be mentioned that a cadmium oxide (CdO)/ZnO nanocomposite with a particle size range of 20–50 nm was also prepared by precipitation approach in the presence of Padina gymnospora (Rajaboopathi and Thambidurai, 2017). Reactive Blue 198 degradation efficiency of CdO/ZnO in the presence of different light sources is 99.57% (natural sunlight), 95.2% (36 W fluorescent lamp, visible light), and 94.3% (15 W UV lamp, ultraviolet). The best photocatalytic performance was determined under sunlight irradiation (time = 15 min). The photocatalyst dosage, dye concentration, and volume are 0.5 g L−1, 10 mg L−1 and 100 mL, respectively.
Algae are potential materials in the treatment of organic pollutants in water due to their favorable properties such as abundance, assimilation of pollutants as nutrients, high biomass yield, and fast growth. Different algae have been effectively employed in adsorptive and catalytic pollutant removal techniques. Algae biomass shows great biosorption capacity for organic pollutants. Algae types and ultrasound play a foremost role in the pollutant’s removal process. Chemical treatment or the addition of nanomaterials remarkably improved the performance of algae. Biosorption of organic pollutants by algae occurs via physical and chemical interactions. Biochar, hydrochar, and activated carbon adsorbents with good adsorption capacity can be obtained using algae species. Redox mediators and microbes like bacteria and fungi can boost the degradation performance of algae and also provide other value-added products. Algae fuel cells are promising for wastewater treatment and power production. Light, concentration, and volume of wastewater, combined with other techniques and cell design, determine the performance of algal fuel cells. Integrated algae fuel cells might have much attention in water pollution remediation and energy production. Algae have been used as source materials for the synthesis of (catalyst in the activation of oxidizing agent, Fenton-like, and photocatalysis) catalysts, which have shown high performance in the degradation of organic pollutants. Further, algae-extracts also functioned as reducing and/or capping reagents in the preparation of monometallic, bimetallic nanoparticles, metal oxides (like ZnO, CuO), metal sulfides (like CuS), and composite nanocatalysts. Algae are being source materials and reagents in the development of adsorbents and catalysts. Effective utilization of algae-related adsorbents and catalysts might be beneficial in-terms of environmental protection and economic aspects, as it avoids usage of harmful chemicals and minimize harmful effects of algae.
Besides, further research studies are essential to determine the performance of algae-based adsorbents and catalysts in real wastewater treatment plants. Research can be focussed on the algae-mediated synthesis of sustainable nanomaterial-based adsorbents and catalysts. More understandings are needed to explore the role of algae in nanomaterials synthesis. The biocompatibility of algae-derived nanomaterials needs to be evaluated. The removal performance of algae in the presence of nanomaterials must be studied using different kinds of organic pollutants. Research should be focused on the conversion of highly toxic algae into useful materials and pollutant adsorbed algae biomass. Research studies should also focus on the cost analysis of algae-based materials.
RS: Data curation, Formal Analysis, Methodology, Validation, Writing–original draft. SaR: Conceptualization, Formal Analysis, Supervision, Validation, Writing–review and editing. W-HC: Conceptualization, Formal Analysis, Investigation, Methodology, Supervision, Writing–review and editing. MS-M: Formal Analysis, Investigation, Methodology, Validation, Writing–original draft. TS: Formal Analysis, Investigation, Methodology, Supervision, Writing–original draft. AJ: Data curation, Investigation, Methodology, Project administration, Validation, Writing–original draft. SuR: Conceptualization, Formal Analysis, Investigation, Methodology, Supervision, Validation, Writing–review and editing.
The author(s) declare that no financial support was received for the research, authorship, and/or publication of this article.
The authors declare that the research was conducted in the absence of any commercial or financial relationships that could be construed as a potential conflict of interest.
All claims expressed in this article are solely those of the authors and do not necessarily represent those of their affiliated organizations, or those of the publisher, the editors and the reviewers. Any product that may be evaluated in this article, or claim that may be made by its manufacturer, is not guaranteed or endorsed by the publisher.
Abdelhameed, R. M., Alzahrani, E., Shaltout, A. A., and Moghazy, R. M. (2020). Development of biological macroalgae lignins using copper based metal-organic framework for selective adsorption of cationic dye from mixed dyes. Int. J. Biol. Macromol. 165, 2984–2993. doi:10.1016/j.ijbiomac.2020.10.157
Abdulhameed, A. S., Jawad, A. H., Kashi, E., Radzun, K. A., Alothman, Z. A., and Wilson, L. D. (2022). Insight into adsorption mechanism, modeling, and desirability function of crystal violet and methylene blue dyes by microalgae: box-Behnken design application. Algal Res. 67, 102864. doi:10.1016/j.algal.2022.102864
Aboelfetoh, E. F., El-Shenody, R. A., and Ghobara, M. M. (2017). Eco-friendly synthesis of silver nanoparticles using green algae (Caulerpa serrulata): reaction optimization, catalytic and antibacterial activities. Environ. Monit. Assess. 189, 349. doi:10.1007/s10661-017-6033-0
Al-Enazi, N. M. (2022). Optimized synthesis of mono and bimetallic nanoparticles mediated by unicellular algal (diatom) and its efficiency to degrade azo dyes for wastewater treatment. Chemosphere 303, 135068. doi:10.1016/j.chemosphere.2022.135068
Al-Ghamdi, S. A., Darwish, A. A. A., Hamdalla, T. A., Pasha, A., Elnair, M. E., Al-Atawi, A., et al. (2023). Biological synthesis of novel carbon quantum dots using Halimeda opuntia green algae with improved optical properties and electrochemical performance for possible energy storage applications. Int. J. Electrochem. Sci. 18, 100102. doi:10.1016/j.ijoes.2023.100102
Annamalai, S., and Shin, W. S. (2023a). Algae-derived metal-free boron-doped biochar acts as a catalyst for the activation of peroxymonosulfate toward the degradation of diclofenac. Environ. Pollut. 331, 121850. doi:10.1016/j.envpol.2023.121850
Annamalai, S., and Shin, W. S. (2023b). In-situ pyrolysis of Undaria pinnatifida as a green carbo-catalyst for degradation of organic contaminants: role of inherent N and P in the degradation pathway. Chem. Eng. J. 465, 142813. doi:10.1016/j.cej.2023.142813
Anwar, S. J., Bhat, I. U. H., Yusoff, H. M., Razali, M. H., Kadir, M. A., and Ern, L. K. (2021). Brown algae-based preparation, characterization and application of Pd nanocatalyst for enhanced reductive azo dye degradation. Clean. Eng. Technol. 4, 100172. doi:10.1016/j.clet.2021.100172
Babich, O., Sukhikh, S., Larina, V., Kalashnikova, O., Kashirskikh, E., Prosekov, A., et al. (2022). Algae: study of edible and biologically active fractions, their properties and applications. Plants 11, 780. doi:10.3390/plants11060780
Bayat, M., Salehi, E., and Mahdieh, M. (2023). Chromochloris zofingiensis microalgae as a potential dye adsorbent: adsorption thermo-kinetic, isothermal, and process optimization. Algal Res. 71, 103043. doi:10.1016/j.algal.2023.103043
Bhatt, P., Bhandari, G., Turco, R. F., Aminikhoei, Z., Bhatt, K., and Simsek, H. (2022). Algae in wastewater treatment, mechanism, and application of biomass for production of value-added product. Environ. Pollut. 309, 119688. doi:10.1016/j.envpol.2022.119688
Bibi, S., Bibi, A., Mullungal, M. N., Abu-Dieyeh, M., and Al-Ghouti, M. A. (2023). Macroalgae as an eco-friendly and successful green technology for the removal of crystal violet from synthetic and real wastewater. Arab. J. Chem. 16, 105191. doi:10.1016/j.arabjc.2023.105191
Biris-Dorhoi, E. S., Michiu, D., Pop, C. R., Rotar, A. M., Tofana, M., Pop, O. L., et al. (2020). Macroalgae - a sustainable source of chemical compounds with biological activities. Nutrients 12, 3085. doi:10.3390/nu12103085
Borah, D., Saikia, P., Sarmah, P., Gogoi, D., Rout, J., Ghosh, N. N., et al. (2022). Composition controllable alga-mediated green synthesis of covellite CuS nanostructure: an efficient photocatalyst for degradation of toxic dye. Inorg. Chem. Commun. 142, 109608. doi:10.1016/j.inoche.2022.109608
Bulgariu, D., and Bulgariu, L. (2016). Potential use of alkaline treated algae waste biomass as sustainable biosorbent for clean recovery of cadmium(II) from aqueous media: batch and column studies. J. Clean. Prod. 112, 4525–4533. doi:10.1016/j.jclepro.2015.05.124
Chen, B., Gu, Z., Wu, M., Ma, Z., Lim, H. R., Khoo, K. S., et al. (2022). Advancement pathway of biochar resources from macroalgae biomass: a review. Biomass Bioenergy 167, 106650. doi:10.1016/j.biombioe.2022.106650
Cobas, M., Sanromán, M. A., and Pazos, M. (2014). Box–Behnken methodology for Cr(VI) and leather dyes removal by an eco-friendly biosorbent: F. vesiculosus. Bioresour. Technol. 160, 166–174. doi:10.1016/j.biortech.2013.12.125
Commault, A. S., Laczka, O., Siboni, N., Tamburic, B., Crosswell, J. R., Seymour, J. R., et al. (2017). Electricity and biomass production in a bacteria-Chlorella based microbial fuel cell treating wastewater. J. Power Sources 356, 299–309. doi:10.1016/j.jpowsour.2017.03.097
Consales, G., Bottaro, M., Mancusi, C., Neri, A., Sartor, P., Voliani, A., et al. (2023). Persistent organic pollutants (POPs) in three bathyal chondrichthyes from the north-western Mediterranean Sea. Mar. Pollut. Bull. 196, 115647. doi:10.1016/j.marpolbul.2023.115647
Cuellar-Bermudez, S. P., Aleman-Nava, G. S., Chandra, R., Garcia-Perez, J. S., Contreras-Angulo, J. R., Markou, G., et al. (2017). Nutrients utilization and contaminants removal. A review of two approaches of algae and cyanobacteria in wastewater. Algal Res. 24, 438–449. doi:10.1016/j.algal.2016.08.018
Deka, R., Shreya, S., Mourya, M., Sirotiya, V., Rai, A., Khan, M. J., et al. (2022). A techno-economic approach for eliminating dye pollutants from industrial effluent employing microalgae through microbial fuel cells: barriers and perspectives. Environ. Res. 212, 113454. doi:10.1016/j.envres.2022.113454
Denardin da Rosa, A. L., Carissimi, E., Dotto, G. L., Sander, H., and Feris, L. A. (2018). Biosorption of rhodamine B dye from dyeing stones effluents using the green microalgae Chlorella pyrenoidosa. J. Clean. Prod. 198, 1302–1310. doi:10.1016/j.jclepro.2018.07.128
Dev, P., Chakravarty, K., Pandey, M., Ranjan, R., Cyriac, M., Mishra, V. N., et al. (2023). Effect of persistent organic pollutants in patients with ischemic stroke and all stroke: a systematic review and meta-analysis. Toxicology 494, 153567. doi:10.1016/j.tox.2023.153567
Elgarahy, A. M., Maged, A., Elwakeel, K. Z., El-Gohary, F., and El-Qelish, M. (2023). Tuning cationic/anionic dyes sorption from aqueous solution onto green algal biomass for biohydrogen production. Environ. Res. 216, 114522. doi:10.1016/j.envres.2022.114522
Fernandez, L. A. G., Ramos, V. C., Polo, M. S., and Castillo, N. A. M. (2023). Fundamentals in applications of algae biomass: a review. J. Environ. Manage. 338, 117830. doi:10.1016/j.jenvman.2023.117830
Fontoura, T. d., Rolim, G. S., Mella, B., Farenzena, M., and Gutterres, M. (2017). Defatted microalgal biomass as biosorbent for the removal of Acid Blue 161 dye from tannery effluent. J. Environ. Chem. Eng. 5, 5076–5084. doi:10.1016/j.jece.2017.09.051
Foroutan, R., Mohammadi, R., Razeghi, J., and Ramavandi, B. (2019). Performance of algal activated carbon/Fe3O4 magnetic composite for cationic dyes removal from aqueous solutions. Algal Res. 40, 101509. doi:10.1016/j.algal.2019.101509
Gokulan, R., Avinash, A., Ganesh Prabhu, G., and Jegan, J. (2019). Remediation of remazol dyes by biochar derived from Caulerpa scalpelliformis—an eco-friendly approach. J. Environ. Chem. Eng. 7, 103297. doi:10.1016/j.jece.2019.103297
Gu, H., Chen, X., Chen, F., Zhou, X., and Parsaee, Z. (2018). Ultrasound-assisted biosynthesis of CuO-NPs using brown alga Cystoseira trinodis: characterization, photocatalytic AOP, DPPH scavenging and antibacterial investigations. Ultrason. Sonochem. 41, 109–119. doi:10.1016/j.ultsonch.2017.09.006
Gul, U. D., Tastan, B. E., and Bayazıt, G. (2019). Assessment of algal biomasses having different cell structures for biosorption properties of acid red P-2BX dye. S. Afr. J. Bot. 127, 147–152. doi:10.1016/j.sajb.2019.08.047
Hou, Q., Yang, Z., Chen, S., and Pei, H. (2020). Using an anaerobic digestion tank as the anodic chamber of an algae-assisted microbial fuel cell to improve energy production from food waste. Water Res. 170, 115305. doi:10.1016/j.watres.2019.115305
Hu, C., Chen, Q., Wu, S., Wang, J., Zhang, S., and Chen, L. (2024). Coupling harmful algae derived nitrogen and sulfur co-doped carbon nanosheets with CeO2 to enhance the photocatalytic degradation of isothiazolinone biocide. J. Environ. Manage. 356, 120621. doi:10.1016/j.jenvman.2024.120621
Hu, N., Xu, Y., Sun, C., Zhu, L., Sun, S., Zhao, Y., et al. (2021). Removal of atrazine in catalytic degradation solutions by microalgae Chlorella sp. and evaluation of toxicity of degradation products via algal growth and photosynthetic activity. Ecotoxicol. Environ. Saf. 207, 111546. doi:10.1016/j.ecoenv.2020.111546
Hung, C. M., Chen, C. W., Huang, C. P., Cheng, J. W., and Dong, C. D. (2022). Algae-derived metal-free boron-doped biochar as an efficient bioremediation pretreatment for persistent organic pollutants in marine sediments. J. Clean. Prod. 336, 130448. doi:10.1016/j.jclepro.2022.130448
Jiang, J., Zhao, J., Zhao, G., Liu, L., Song, H., and Liao, S. (2023). Recognition, possible source, and risk assessment of organic pollutants in surface water from the Yongding River Basin by non-target and target screening. Environ. Pollut. 331, 121895. doi:10.1016/j.envpol.2023.121895
Khalafi, T., Buazar, F., and Ghanemi, K. (2019). Phycosynthesis and enhanced photocatalytic activity of zinc oxide nanoparticles toward organosulfur pollutants. Sci. Rep. 9, 6866. doi:10.1038/s41598-019-43368-3
Khan, F., Shahid, A., Zhu, H., Wang, N., Rizwan Javed, M., Ahmad, N., et al. (2022). Prospects of algae-based green synthesis of nanoparticles for environmental applications. Chemosphere 293, 133571. doi:10.1016/j.chemosphere.2022.133571
Khoo, K. S., Chia, W. Y., Chew, K. W., and Show, P. L. (2021). Microalgal-bacterial consortia as future prospect in wastewater bioremediation, environmental management and bioenergy production. Indian J. Microbiol. 61, 262–269. doi:10.1007/s12088-021-00924-8
Kljakovic-Gaspic, Z., Dvorscak, M., Orct, T., Sekovanic, A., Klincic, D., Jagic, K., et al. (2023). Metal(loid)s and persistent organic pollutants in yellow European eel from the Rasa River, Croatia. Mar. Pollut. Bull. 187, 114527. doi:10.1016/j.marpolbul.2022.114527
Lebron, Y. A. R., Moreira, V. R., and Santos, L. V. S. (2019). Studies on dye biosorption enhancement by chemically modified Fucus vesiculosus, Spirulina maxima and Chlorella pyrenoidosa algae. J. Clean. Prod. 240, 118197. doi:10.1016/j.jclepro.2019.118197
Li, P., Karunanidhi, D., Subramani, T., and Srinivasamoorthy, K. (2021). Sources and consequences of groundwater contamination. Arch. Environ. Contam. Toxicol. 80, 1–10. doi:10.1007/s00244-020-00805-z
Li, Y., Cao, P., Wang, S., and Xu, X. (2022). Research on the treatment mechanism of anthraquinone dye wastewater by algal-bacterial symbiotic system. Bioresour. Technol. 347, 126691. doi:10.1016/j.biortech.2022.126691
Luarte, T., Tucca, F., Nimptsch, J., Woelfl, S., Casas, G., Dachs, J., et al. (2022). Occurrence and air-water diffusive exchange legacy persistent organic pollutants in an oligotrophic north Patagonian lake. Environ. Res. 204, 112042. doi:10.1016/j.envres.2021.112042
Mahmoud, R. H., Samhan, F. A., Ibrahim, M. K., Ali, G. H., and Hassan, R. Y. A. (2022). Waste to energy conversion utilizing nanostructured Algal-based microbial fuel cells. Electrochem. Sci. Adv. 2, e2100071. doi:10.1002/elsa.202100071
Martínez-Ruiz, M., Molina-Vazquez, A., Santiesteban-Romero, B., Reyes-Pardo, H., Villasenor-Zepeda, K. R., Melendez-Sanchez, E. R., et al. (2022). Micro-algae assisted green bioremediation of water pollutants rich leachate and source products recovery. Environ. Pollut. 306, 119422. doi:10.1016/j.envpol.2022.119422
Meng, X., Liu, G., Zhou, J., and Fu, Q. S. (2014). Effects of redox mediators on azo dye decolorization by Shewanella algae under saline conditions. Bioresour. Technol. 151, 63–68. doi:10.1016/j.biortech.2013.09.131
Mi, Y., Zhang, S., Zhao, Y., Sun, G., and Cao, Z. (2023). Pyrrolic N and persistent free radical synergistically promote catalytic degradation of dyes via Fe2O3/activated biochar derived from Taihu blue algae. Colloids Surf. A Physicochem. Eng. Asp. 667, 131393. doi:10.1016/j.colsurfa.2023.131393
Mokhtar, N., Aziz, E. A., Aris, A., Ishak, W. F. W., and Mohd Ali, N. S. (2017). Biosorption of azo-dye using marine macro-alga of Euchema Spinosum. J. Environ. Chem. Eng. 5, 5721–5731. doi:10.1016/j.jece.2017.10.043
Monisha, B., Sridharan, R., Kumar, P. S., Rangasamy, G., Krishnaswamy, V. G., and Subhashree, S. (2023). Sensing of azo toxic dyes using nanomaterials and its health effects - a review. Chemosphere 313, 137614. doi:10.1016/j.chemosphere.2022.137614
Moradi, Z., Haghjou, M. M., Zarei, M., Colville, L., and Raza, A. (2021). Synergy of production of value-added bioplastic, astaxanthin and phycobilin co-products and Direct Green 6 textile dye remediation in Spirulina platensis. Chemosphere 280, 130920. doi:10.1016/j.chemosphere.2021.130920
Nasrollahzadeh, M., Sajjadi, M., Iravani, S., and Varma, R. S. (2021). Green-synthesized nanocatalysts and nanomaterials for water treatment: current challenges and future perspectives. J. Hazard. Mater. 401, 123401. doi:10.1016/j.jhazmat.2020.123401
Nguyen, H. T. H., Kakarla, R., and Min, B. (2017). Algae cathode microbial fuel cells for electricity generation and nutrient removal from landfill leachate wastewater. Int. J. Hydrog. Energy 42, 29433–29442. doi:10.1016/j.ijhydene.2017.10.011
Oualid, H. A., Abdellaoui, Y., Laabd, M., Ouardi, M. E., Brahmi, Y., Iazza, M., et al. (2020). Eco-efficient green seaweed Codium decorticatum biosorbent for textile dyes: characterization, mechanism, recyclability, and RSM optimization. ACS Omega 5, 22192–22207. doi:10.1021/acsomega.0c02311
Palanimuthu, K., Subbiah, U. B., Sundharam, S., and Munusamy, C. (2023). Spirulina carbon dots: a promising biomaterial for photocatalytic textile industry Reactive Red M8B dye degradation. Environ. Sci. Pollut. Res. 30, 52073–52086. doi:10.1007/s11356-023-25987-6
Patar, S., Mittal, R., Dutta, A., Bhuyan, B. K., and Borthakur, L. J. (2024). Algae derived N-doped mesoporous carbon nanoflakes fabricated with nickel ferrite for photocatalytic removal of Congo red and rhodamine B dyes. Surf. Interfaces 51, 104710. doi:10.1016/j.surfin.2024.104710
Pathy, A., Krishnamoorthy, N., Chang, S. X., and Paramasivan, B. (2022). Malachite green removal using algal biochar and its composites with kombucha SCOBY: an integrated biosorption and phycoremediation approach. Surf. Interfaces 30, 101880. doi:10.1016/j.surfin.2022.101880
Qin, P., Cao, F., Lu, S., Li, L., Guo, X., Zhao, B., et al. (2019). Occurrence and health risk assessment of volatile organic compounds in the surface water of Poyang Lake in March 2017. RSC Adv. 9, 22609–22617. doi:10.1039/c9ra02450f
Qulatein, H. A., and Yilmaz, M. S. (2023). Preparation of low-cost and non-conventional macroalgae-based biosorbent for fast and effectively selective dye adsorption. Mater. Chem. Phys. 303, 127741. doi:10.1016/j.matchemphys.2023.127741
Rabie, A. M., Abukhadra, M. R., Rady, A. M., Ahmed, S. A., Labena, A., Mohamed, H. S. H., et al. (2020). Instantaneous photocatalytic degradation of malachite green dye under visible light using novel green Co–ZnO/algae composites. Res. Chem. Intermed. 46, 1955–1973. doi:10.1007/s11164-019-04074-x
Rai, A., Sirotiya, V., Mourya, M., Khan, M. J., Ahirwar, A., Sharma, A. K., et al. (2022). Sustainable treatment of dye wastewater by recycling microalgal and diatom biogenic materials: biorefinery perspectives. Chemosphere 305, 135371. doi:10.1016/j.chemosphere.2022.135371
Rajaboopathi, S., and Thambidurai, S. (2017). Green synthesis of seaweed surfactant based CdO-ZnO nanoparticles for better thermal and photocatalytic activity. Curr. Appl. Phys. 17, 1622–1638. doi:10.1016/j.cap.2017.09.006
Ramesh, B., Saravanan, A., Senthil Kumar, P., Yaashikaa, P. R., Thamarai, P., Shaji, A., et al. (2023). A review on algae biosorption for the removal of hazardous pollutants from wastewater: limiting factors, prospects and recommendations. Environ. Pollut. 327, 121572. doi:10.1016/j.envpol.2023.121572
Rios-Miguel, A. B., van Bergen, T. J. H. M., Zillien, C., Ragas, A. M. J., van Zelm, R., Jetten, M. S. M., et al. (2023). Predicting and improving the microbial removal of organic micropollutants during wastewater treatment: a review. Chemosphere 333, 138908. doi:10.1016/j.chemosphere.2023.138908
Rocha de Freitas, G., Vieira, M. G. A., and Carlos da Silva, M. G. (2018). Batch and fixed bed biosorption of copper by acidified algae waste biomass. Ind. Eng. Chem. Res. 57, 11767–11777. doi:10.1021/acs.iecr.8b02541
Salman, J. M., Majrashi, N., Hassan, F. M., Al-Sabri, A., Abdul-Adel Jabar, E., and Ameen, F. (2023). Cultivation of blue green algae (Arthrospira platensis Gomont, 1892) in wastewater for biodiesel production. Chemosphere 335, 139107. doi:10.1016/j.chemosphere.2023.139107
Sanganyado, E., Chingono, K. E., Gwenzi, W., Chaukura, N., and Liu, W. (2021). Organic pollutants in deep sea: occurrence, fate, and ecological implications. Water Res. 205, 117658. doi:10.1016/j.watres.2021.117658
Satya, A. D. M., Cheah, W. Y., Yazdi, S. K., Cheng, Y. S., Khoo, K. S., Vo, D. V. N., et al. (2023). Progress on microalgae cultivation in wastewater for bioremediation and circular bioeconomy. Environ. Res. 218, 114948. doi:10.1016/j.envres.2022.114948
Serrà, A., Artal, R., García-Amorós, J., Sepúlveda, B., Gómez, E., Nogués, J., et al. (2020). Hybrid Ni@ZnO@ZnS-Microalgae for Circular Economy: a smart route to the efficient integration of solar photocatalytic water decontamination and bioethanol production. Adv. Sci. 7, 1902447. doi:10.1002/advs.201902447
Shao, H., Li, Y., Zheng, L., Chen, T., and Liu, J. (2017). Removal of methylene blue by chemically modified defatted brown algae Laminaria japonica. J. Taiwan Inst. Chem. Eng. 80, 525–532. doi:10.1016/j.jtice.2017.08.023
Sharma, G., Bhogal, S., Gupta, V. K., Agarwal, S., Kumar, A., Pathania, D., et al. (2019). Algal biochar reinforced trimetallic nanocomposite as adsorptional/photocatalyst for remediation of malachite green from aqueous medium. J. Mol. Liq. 275, 499–509. doi:10.1016/j.molliq.2018.11.070
Singh, A., Pal, D. B., Kumar, S., Srivastva, N., Syed, A., Elgorban, A. M., et al. (2021). Studies on Zero-cost algae based phytoremediation of dye and heavy metal from simulated wastewater. Bioresour. Technol. 342, 125971. doi:10.1016/j.biortech.2021.125971
Singh, A. K., Kumar, A., and Chandra, R. (2022). Environmental pollutants of paper industry wastewater and their toxic effects on human health and ecosystem. Bioresour. Technol. Rep. 20, 101250. doi:10.1016/j.biteb.2022.101250
Solanki, S., Sinha, S., Bisaria, K., Singh, R., and Saxena, R. (2022). Accurate data prediction by fuzzy inference model for adsorption of hazardous azo dyes by novel algal doped magnetic chitosan bionanocomposite. Environ. Res. 214, 113844. doi:10.1016/j.envres.2022.113844
Tang, W., Xu, X., Ye, B. C., Cao, P., and Alia, A. (2019). Decolorization and degradation analysis of Disperse Red 3B by a consortium of the fungus Aspergillus sp. XJ-2 and the microalgae Chlorella sorokiniana XJK. RSC Adv. 9, 14558–14566. doi:10.1039/c9ra01169b
Tukarambai, M. (2022). Efficacy of Algae powder (BAST) on uprooting of methylene blue dye (MBD): optimization. Mater. Today Proc. 62, 4355–4364. doi:10.1016/j.matpr.2022.04.864
Tukaram Bai, M., Anudeep, Y. V., Raju, Ch. A. I., Venkata Rao, P., and Chittibabu, N. (2021). Decolourization of Eosin yellow (EY) dye using a variety of brown algae. Mater. Today Proc. 42, 1130–1137. doi:10.1016/j.matpr.2020.12.495
Turner, R. D. R., Warne, M. S. J., Dawes, L. A., Thompson, K., and Will, G. D. (2019). Greywater irrigation as a source of organic micro-pollutants to shallow groundwater and nearby surface water. Sci. Total Environ. 669, 570–578. doi:10.1016/j.scitotenv.2019.03.073
United Nations (2024), Goal 6: ensure access to water and sanitation for all. Available at: https://unric.org/en/sdg-6/ (Accessed on July 01, 2024).
van der Meer, T. V., Davey, C. J. E., Verdonschot, P. F. M., and Kraak, M. H. S. (2023). Removal of nutrients from WWTP effluent by an algae-mussel trophic cascade. Ecol. Eng. 190, 106930. doi:10.1016/j.ecoleng.2023.106930
Wang, H., Wang, H., and Yan, Q. (2022). Peroxymonosulfate activation by algal carbocatalyst for organic dye oxidation: insights into experimental and theoretical. Sci. Total Environ. 816, 151611. doi:10.1016/j.scitotenv.2021.151611
Wang, T., Zhou, L., Cai, C., Ni, Z., Chen, X., Kuang, B., et al. (2023a). Electroactive bacteria–algae biofilm coupled with siphon aeration boosts high-salinity wastewater purification: a pilot study focusing on performance and microbial community. J. Water Process Eng. 53, 103841. doi:10.1016/j.jwpe.2023.103841
Wang, X., Zhang, C., Zhang, Y., Ma, X., Xie, B., Wang, S., et al. (2023b). Activation of peroxymonosulfate by an Enteromorpha prolifera derived biochar supported CoFe2O4 catalyst for highly efficient lomefloxacin hydrochloride degradation under a wide pH range. Sep. Purif. Technol. 316, 123846. doi:10.1016/j.seppur.2023.123846
Yadav, M., Thakore, S., and Jadej, R. (2022). Removal of organic dyes using Fucus vesiculosus seaweed bioadsorbent an ecofriendly approach: equilibrium, kinetics and thermodynamic studies. Environ. Chem. Ecotoxicol. 4, 67–77. doi:10.1016/j.enceco.2021.12.003
Yang, Z., Li, J., Chen, F., Xu, L., Jin, Y., Xu, S., et al. (2021). Bioelectrochemical process for simultaneous removal of copper, ammonium and organic matter using an algae-assisted triple-chamber microbial fuel cell. Sci. Total Environ. 798, 149327. doi:10.1016/j.scitotenv.2021.149327
Yang, Z., Pei, H., Hou, Q., Jiang, L., Zhang, L., and Nie, C. (2018). Algal biofilm-assisted microbial fuel cell to enhance domestic wastewater treatment: nutrient, organics removal and bioenergy production. Chem. Eng. J. 332, 277–285. doi:10.1016/j.cej.2017.09.096
Yao, X., Ji, L., Guo, J., Ge, S., Lu, W., Chen, Y., et al. (2020). An abundant porous biochar material derived from wakame (Undaria pinnatifida) with high adsorption performance for three organic dyes. Bioresour. Technol. 318, 124082. doi:10.1016/j.biortech.2020.124082
Yin, K., Peng, L., Chen, D. D., Liu, S., Zhang, Y., Gao, B., et al. (2023b). High-loading of well dispersed single-atom catalysts derived from Fe-rich marine algae for boosting Fenton-like reaction: role identification of iron center and catalytic mechanisms. Appl. Catal. B Environ. 336, 122951. doi:10.1016/j.apcatb.2023.122951
Yin, Q., Yan, H., Liang, Y., Jiang, Z., Wang, H., and Nian, Y. (2023a). Activation of persulfate by blue algae biochar supported FeOX particles for tetracycline degradation: performance and mechanism. Sep. Purif. Technol. 319, 124005. doi:10.1016/j.seppur.2023.124005
Yin, Q., Yan, H., Liang, Y., Wang, H., Duan, P., and Lai, B. (2023c). Nitrogen doped biochar derived from algae as persulfate activator for the degradation of tetracycline: role of exogenous N doping and electron transfer pathway. Sep. Purif. Technol. 318, 123970. doi:10.1016/j.seppur.2023.123970
Zhang, H., Zhang, F., and Huang, Q. (2017). Highly effective removal of malachite green from aqueous solution by hydrochar derived from phycocyanin-extracted algal bloom residues through hydrothermal carbonization. RSC Adv. 7, 5790–5799. doi:10.1039/c6ra27782a
Keywords: wastewater treatment, algae technology, nanomaterials, biosorption, bioremediation, bio-catalysis, algae fuel cell
Citation: Suresh R, Rajendran S, Chen W-H, Soto-Moscoso M, Sundaram T, Jalil AA and Rajamani Sekar SK (2024) A review on algae-mediated adsorption and catalytic processes for organic water pollution remediation. Front. Mater. 11:1432704. doi: 10.3389/fmats.2024.1432704
Received: 14 May 2024; Accepted: 12 August 2024;
Published: 26 August 2024.
Edited by:
Sathishkumar Kuppusamy, Bharathidasan University, IndiaReviewed by:
Zafar Hussain Ibupoto, University of Sindh, PakistanCopyright © 2024 Suresh, Rajendran, Chen, Soto-Moscoso, Sundaram, Jalil and Rajamani Sekar. This is an open-access article distributed under the terms of the Creative Commons Attribution License (CC BY). The use, distribution or reproduction in other forums is permitted, provided the original author(s) and the copyright owner(s) are credited and that the original publication in this journal is cited, in accordance with accepted academic practice. No use, distribution or reproduction is permitted which does not comply with these terms.
*Correspondence: Saravanan Rajendran, c2FyYXZhbmFuMy5yYWpAZ21haWwuY29t; Wei-Hsin Chen, d2VpaHNpbmNoZW5AZ21haWwuY29t; Aishah Abdul Jalil, YWlzaGFoYWpAdXRtLm15; Suresh Kumar Rajamani Sekar, c3VyZXNoLmt1bWFyQGFtdS5lZHUuZXQ=
Disclaimer: All claims expressed in this article are solely those of the authors and do not necessarily represent those of their affiliated organizations, or those of the publisher, the editors and the reviewers. Any product that may be evaluated in this article or claim that may be made by its manufacturer is not guaranteed or endorsed by the publisher.
Research integrity at Frontiers
Learn more about the work of our research integrity team to safeguard the quality of each article we publish.