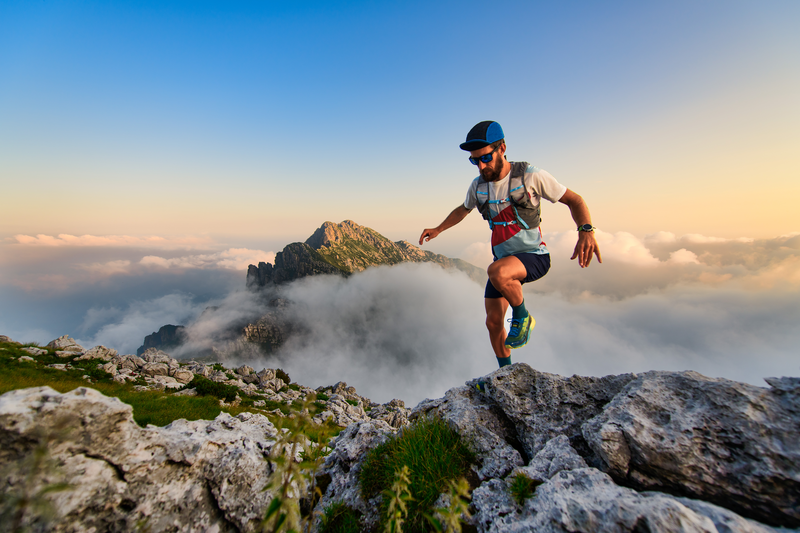
94% of researchers rate our articles as excellent or good
Learn more about the work of our research integrity team to safeguard the quality of each article we publish.
Find out more
ORIGINAL RESEARCH article
Front. Mater. , 11 September 2024
Sec. Structural Materials
Volume 11 - 2024 | https://doi.org/10.3389/fmats.2024.1428700
Construction using eco-friendly materials reduces environmental impact and promotes sustainable practices. This research uses sawdust and steel fibers to design sustainable concrete. The main goal is to improve mechanical properties and reduce embodied carbon emissions. This study examines the mechanical properties of concrete with different sawdust and steel fiber combinations to fill a gap in the literature. In this research synergistic effect of saw dust and steel fiber on concrete characteristics have been studied. The research also examines these pairings’ environmental benefits. This study used a response surface methodology (RSM) to design an experimental program and assess the effects of input variables (sawdust and steel fiber percentages) on output responses like compressive strength (CS), split tensile strength (STS), flexural strength (FS), modulus of elasticity (MOE), embodied carbon (EC), and eco-strength efficiency (ESE). Established testing methodologies and RSM provided an optimum prediction model based on specimen mechanical properties. Sawdust and steel fibers enhances concrete’s mechanical properties. Varying proportions of both materials were added in mix; sawdust (0%–12%) and steel fiber (0%–2%). The experimental findings suggest that the optimized composition achieved the following mechanical properties: 13.85 MPa compressive strength, 1.4 MPa split tensile strength, 3.67 MPa flexural strength, 18.027 GPa modulus of elasticity, 211.272 kg CO2e/m3 embodied carbon, and 0.065487 eco-strength efficiency. This research showed that the aims of improving mechanical properties and reducing embodied carbon were achieved. As per multi-objective optimization, optimal percentages of saw dust and steel fibers in concrete are 11.81% and 0.063% respectively. The investigation yielded many suggestions. To test the optimal blend composition of ecologically friendly concrete in real-world building projects, start with realistic projects. Finally, life cycle evaluations and cost studies are needed to determine the environmental and economic impacts of eco-friendly concrete compared to standard options.
The widespread utilization of concrete in construction is attributed to its exceptional robustness and permanence. The conventional production process of concrete necessitates substantial utilization of natural resources and energy, resulting in noteworthy environmental consequences. The development of sustainable concrete composites has amassed increasing attention after 2020, with a focus on utilizing waste materials and implementing reinforcement techniques to improve their mechanical properties (Liu et al., 2020; Basser et al., 2021). Notwithstanding endeavors to create sustainable concrete composites, a strong demand exists in the optimization of the properties of these composites through the simultaneous integration of waste materials and reinforcement methods. The majority of research attempts have concentrated on singular waste materials or methods of reinforcement with a restricted investigation of mixed additives in concrete (Awolusi et al., 2019; Kropidłowska, 2022).
Saw Dust (SD) is an easily available by-product from the timber industry and usually taken to be one of the inevitable wastes. These include the respective reduced dependency on the traditional aggregates, which have environmental impacts (Shoughy, 2013). Another possible way is replacing part of the natural aggregates with SD, which might help reduce the Embodied Carbon of Concrete (EC) to the tune of 20%. Further, the incorporation of SD into concrete can enhance the thermal insulating characteristics of concrete, thus favoring sustainable building techniques which have the prospect to achieve energy conservation of about 30% (He et al., 2022; Turner and Collins, 2013).
The addition of steel fibers (SF) to concrete results in improved mechanical properties, including flexural and tensile strength improvements (Lehner et al., 2020; Osei and Jackson, 2016). In some reports, there is an increase of about 20% in Flexural Strength (FS) with the addition of SF at a volume fraction of 1% (Ahmad Farooq et al., 2023; Ahmad et al., 2023). The result was a reduction in the probability of cracking of the concrete composite with time, and, therefore, improved durability due to that specific strengthening method. This further reflects in the extension of the service life of materials and the reduction in repair and replacement frequencies, ultimately saving more than 35% in cost (Huda Suliman et al., 2019).
Further Response Surface Methodology (RSM) is the common area of application in the fields of design of experimentation and optimization (Maity et al., 2023). This is best as it helps in the investigation of the complex associations existing between most of the variables and the corresponding outcomes (Veza et al., 2023; Bolooki Poorsaheli et al., 2021). The present work of research was focused on the use of RSM to develop the optimized models for predicting the mechanical characteristics of eco-friendly concrete containing a combination of SD and SF. This involves the human step to achieve a proportionate mix design to attain the required properties with minimal environmental damage using systematic variation of SD and SF proportions (Waqar et al., 2023a; Zaid et al., 2021). This study reports on the enhanced efforts to improve the physical and mechanical characteristics of an environmentally-friendly concrete mixture including SD and SF, using the RSM approach. The objective of this study is to carry out the analysis of the effect of different compositions of SD and SF, their effect on the mechanical properties of eco-friendly concrete with RSM (Ahmed, 2017; R.S. EFFECT, 2016). At this juncture, the interesting mechanical properties to mention are compressive strength (CS), flexural strength (FS), split tensile strength (STS), and modulus of elasticity (MOE). Second, the measurement of the extent of decrease in EC and its corresponding environmental consequences as an outcome of integration of SD and SF in eco-friendly concrete. Thirdly, design and development of the modified RSM model that can predict accurately the mechanical properties of eco-friendly concrete manufactured using a combination of SD and SF. Additionally, the efficacy of the model is assessed by comparing the anticipated outcomes with the experimental findings. Finally, identification of the most favorable combination of SD and SF in concrete mixtures that can yield sustainable concrete with exceptional mechanical characteristics, while simultaneously attaining the desired decrease in EC emissions.
In this study, experimental investigation was conducted to determine the mechanical properties of concrete that incorporates both sawdust and steel fibers. According to existing literature, this is the first time these two materials have been used together as additives in concrete. The novelty of work lies in this innovative combination, which has not been previously explored for enhancing concrete properties. Additionally, statistical models were developed using Response Surface Methodology (RSM) to predict the mechanical behavior of the concrete. This approach not only enhances our understanding of the material’s performance but also provides a robust predictive tool for future applications. The significance of this research is highlighted by its potential to promote sustainable construction practices through the use of waste materials like sawdust, thereby reducing environmental impact, while also improving the mechanical properties of concrete with the inclusion of steel fibers. This dual approach of material innovation and statistical modeling underscores the scientific rigor and practical relevance of study.
The construction industry has witnessed a notable surge in the adoption of sustainable construction practices in recent times, primarily driven by the imperative to mitigate the environmental impact of construction activities (Waqar et al., 2023b). Concrete is a ubiquitous construction material that contributes significantly to carbon dioxide emissions during its manufacturing process and currently it has a share of 8% in global warming and 56% in the net construction sector pollution. In order to tackle this concern, scholars have directed their attention towards the creation of sustainable concrete composites that integrate discarded materials and reinforcement methodologies to augment mechanical characteristics and diminish the ecological impact (Abdulridha et al., 2022; Batool et al., 2021).
The utilization of SD, a residual material derived from the timber industry, has unveiled encouraging outcomes as an eco-friendly supplement in the manufacturing of concrete. Numerous research studies have examined its impact on the mechanical characteristics. As established by scholarly investigations, the inclusion of SD in concrete at different substitution levels resulted in a decrease in CS, which varied from 5% to 20% (Wang et al., 2023; Bernardo et al., 2021). However, the above decrease may be relieved through the optimization of the mixture proportions and the inclusion of supplementary cementitious material. In an investigation carried out by Abdulhameed et al. (2023), found that crack load also decreases from 71% to 51% by the inclusion of 45% of sawdust. Further, it has been established that an increase in the utilization of an admixture, such as SD with other strengthening agents, increases the flexural and tensile strength of concrete (Thilakarathna et al., 2020; Mwango and Kambole, 2019). In their study Sharba. (2022), found that reference concrete has the ductility factor of 9.39 and with the addition of SD this value decreased to 5.56. These effects of SD on MOE, a parameter characterizing concrete rigidity, require further elaboration for a better understanding within this relationship (Prabath and Ramadoss, 2022; Alabduljabbar et al., 2020). Experimental research was conducted to evaluate the impact of SD on crack occurring in concrete and it was found that addition of SD resulted in reducing the micro and macro cracks (Khan et al., 2020).
The inclusion of SF as a reinforcement material in concrete is a very common practice used to enhance the mechanical features. Incorporation of SF in concrete enhances tensile strength and ductility for the material. Research findings found that with the addition of SF in the concrete, it could increase the tensile strength of the concrete up to 30%. Considerable values of improvement in FS of concrete by adding SF into it have been observed, and the enhancement reported varies from 10% to 40% (Zarei et al., 2022). The use of SF in concrete can, therefore, improve its MOE, increasing stiffness and cracking resistance by more than 15% for the case of warm climates and by 18% for the case of cold climates. This requires a delicate balance of both the dosage and aspect ratio of SF to yield the desired mechanical properties. Recent study conducted by Wu et al. (2024), found that fiber helps in bridging the cracks and result in providing the additional support and improves the mechanical strength. It was also found that durability of concrete was also improved because of reducing the widths and propagation of cracks. Another study conducted by Helal et al. (2024) to study the effects of steel fibers on long term compressive strength of concrete and it was found that 1% and 1.5% of steel fibers in concrete significantly enhances the long term compressive strength of concrete.
An important aspect in sustainable building practice is the minimization of EC in concrete. The use of waste material, such as SD, in concrete could considerably help in the reduction of EC (Prabath and Ramadoss, 2022; Abbass et al., 2018). This research finding suggests that EC emissions could be minimized by adding SD as a partial replacement for conventional aggregates, according to the cited reductions (Mujalli et al., 2022; Silva et al., 2020) from 10% to 40%. Further addition of SF into concrete increases its mechanical properties, therefore lengthening the structural operational life of construction, and subsequently reducing frequent maintenance or replacement needs through the durability aspect, which counts to almost 40% of the structure costs over the service life. Such an approach of implementation may then lead to colossal reductions in carbon emissions over the building’s lifetime.
Previous research studies revealed that the use of RSM was widely used to optimize the properties of concrete composites based on consideration of multiple correlated variables. RSM allows researchers to explore effectively the effects of different variables and their relationships with the response variables of interest for building optimized models (Gao et al., 2022; Karimipour et al., 2021). RSM has been efficiently utilized in multiplicative research studies to optimize the characteristics of environmentally friendly concrete composites and improve efficiency more than 25% in some cases (Awolusi et al., 2019). RSM optimized the mixture composition of eco-friendly concrete that incorporated recycled aggregates (Yang et al., 2021; Sadowska-Buraczewska et al., 2020).
The study was based on the possible effect of different factors, such as the molar ratio of alkali activators, curing temperature, and curing time on the performance (Köksal et al., 2013; Fattouh et al., 2023). The optimization with RSM gave a remarkable improvement in the CS of the geopolymer concrete. Precisely, an increment of 25% was observed when compared with the control mix design (Collins, 2010; Kumar et al., 2021). Furthermore, it was also applied to the optimization of the mixture composition for fiber-reinforced concrete (Dilip et al., 2014; Zhu et al., 2020).
Researchers have taken great care to account for such variables as the proportion of fibers to the total volume, the ratio of water to cement, and the time during which the curing took place. A refined model was formulated by means of RSM analysis. It was found that there was a 20% increase in FS of the FRC (fiber-reinforced concrete) compared to the original mixture composition (Memon et al., 2017; Bošnjak et al., 2019). The results from these studies give enough proof on how effective RSM is in optimizing sustainable characteristics of concrete composites (Lee et al., 2022).
Through the implementation of systematic manipulation of variables of interest and subsequent statistical analysis, researchers have successfully constructed optimized models that enhance the mechanical characteristics of concrete. RSM provides a robust framework for comprehending intricate associations among variables and responses, facilitating the creation of concrete materials that are more sustainable and exhibit superior performance (Babaie et al., 2020; Khan et al., 2022).
Largely, the utilization of SD presents a sustainable approach to waste management while simultaneously improving the flexural and tensile strength of concrete. In contrast, SF offers enhanced mechanical characteristics, including heightened tensile and FS. The attainment of desired outcomes is contingent upon the optimization of mix proportions and the aspect ratio of SF. Moreover, the integration of such additives has the potential to decrease the amount of EC emissions associated with the manufacturing of concrete.
The significance of this research study lies in examination of innovative approaches to formulate the sustainable and environmental friendly concrete with SD and SF with enhanced mechanical properties. As the building industry continuously facing the challenge to reduce the carbon footprint in construction and utilize the waste construction materials, this study addresses the critical need of eco-friendly construction materials. By using the SD in concrete which is agriculture byproduct this study not only contributes to the reduction of waste but also promotes the sustainability of construction projects. Furthermore, inclusion of SF improves the ductility and tensile strength of concrete. This combination of SF and SD provides the significant advancements in the field of performance based engineering. This study have combine benefits of resource efficiency as well as structural integrity. Eventually, this research contributes to the efforts in concrete materials innovation and paving the way for more sustainable construction material solution that align with the global environmental goals.
This experimental study employed diverse materials to fabricate a durable concrete composite with sustainable properties. The chosen materials for this study are widely accessible within Malaysia, thereby enhancing the practicality and relevance of the research outcomes within the local milieu. The constituents used in the construction process comprise Cement Type 1 (53 Grade), fine river sand, coarse aggregate, tap water, SD, and SF. It ordered cement by engaging YTL Cement, a famous Malaysian trademark in common use during constructions (Köksal et al., 2022; Zeybek et al., 2022). This material is known for its perfect resistance to compressive forces and stable operational capacity. The fine river sand is selected from a local supplier in Malaysia based on its particle size distribution and quality to fit with the appropriate particle size distribution and quality (Memon et al., 2018; Plotnikov and Kochetkov, 2021). The sand with a fineness modulus of 2.5 is used from local regions, thus ensuring the practicability of the research outcomes for applications in the construction sector in Malaysia. Coarse aggregates: The size range 9.5–37.5 mm used in this work was bought from a supplier situated in Malaysia. The utilization of coarse aggregate procured from nearby sources guarantees conformity with regional building methodologies and accessibility (Kavya Sameera and Keshav, 2022). The concrete composite employs tap water as the primary mixing medium.
The concrete composite integrates SD, which is obtained from the Malaysian timber market, as an unwanted waste material. This substance is a by-product of the forestry sector and is easily accessible. The incorporation of SD into concrete mixture is a viable approach towards sustainable waste management strategies and mitigating the ecological footprint of concrete manufacturing. The SF utilized in this study possess a length of 15 mm and a diameter of 1 mm (Ubachukwu et al., 2022; El-Nadoury, 2021). Reinforcement materials are frequently found in the Malaysian market and are utilized to augment the mechanical characteristics of concrete composites. Incorporating SF into concrete enhances its tensile and FS.
The incorporation of SD and SF in concrete results in a notable influence on the composite’s mechanical characteristics. Both materials provide distinct properties that augment the overall functionality of the concrete. At the Nano level, SD displays characteristics such as elevated surface area and porosity, which are attributed to its fibrous morphology (Pinheiro Simão Ribeiro et al., 2021; Baserah et al., 2021). The aforementioned properties have the potential to impact both the water absorption and workability of the concrete mixture. Upon integration into the concrete composite, SD functions as a filler material. This may further enhance the thermal insulation properties of the composite. The relative percentage of silicon dioxide (SiO2) in the SD is on the higher side, hence revealing pozzolanic reactivity to improve the strength and durability of concrete with time. Comparatively, SF, which are microfibers, have good tensile strength and aspect ratio. SF added to the concrete mix enhance tensile and flexure strength of the composite (Sharba et al., 2022). Surface of micro-SF shows mechanical interlocking with the cement matrix, thus enhancing the load transfer mechanism of concrete and crack resistance of concrete. These high aspect ratios serve to increase adhesion between fibers and the surrounding matrix in SF, hence developing the potential for energy dissipation and ductility (Pinheiro Simão Ribeiro et al., 2021; Baserah et al., 2021).
Combined addition of SD and SF to concrete leads to a synergistic effect, where the distinct properties of each component complement those of the other. In the composites, when used as a filler, SD reduces the density and the weight of the composite, while SF in the composite improves the strength and toughness. On the other side, the incorporation of SF, facilitated by the nanoscale porous structure of SD, can improve the dispersion of the fibers throughout the concrete matrix (Ruiz et al., 2019; Ghafari et al., 2014). The composite material of these fillers integrated with the polymer will have enhanced mechanical properties compared to the matrix compound since the integration of these methods will yield a solid composite material that shows superior mechanical characteristics, including heightened CS, enhanced tensile strength, In general, the mechanical properties are significantly controlled by the nanoscale features of SD and SF in a concrete composite, including the surface area, porosity, tensile strength, and aspect ratio (Devadasu et al., 2020; Da Silva et al., 2021). The synergy of the said materials within a concrete mix leads to the latter—a concrete composite sustainable enough to have enhanced strength, durability, and energy dissipation capacity.
The sustainable concrete composite’s mix proportions were designed and optimized in accordance with the principles of RSM. The objective of the study was to examine the influence of SD and SF on the growth of the concrete composite through the creation of a refined model. Table 1 displays the mix proportions in conjunction with their corresponding Mix IDs. The Mix ID denotes distinct percentages of SD and SF within the concrete composite (Abdul Awal et al., 2016a; Abdul Awal et al., 2016b). The selection of mix ratios was conducted with precision to assess the impact of different proportions of SD and SF on the mechanical characteristics of the concrete.
The Mix ID denoted as “SD0%SF0%” signifies the control mix, which denotes the absence of SD or SF in the mixture. It functions as a point of reference for comparative analysis with the other blends. The following formulations, namely, “SD3%SF0.5%”, “SD6%SF1%”, “SD9%SF1.5%”, and “SD12%SF2%”, involve progressively higher proportions of SD and SF. The diverse mix ratios facilitate an inclusive comprehension of the effect of said materials on the concrete composite. The optimized mix designs were developed through the utilization of RSM, whereby the percentages of SD and SF were systematically modified. The mix proportions were selected based on statistical analysis and optimization techniques to achieve desired mechanical properties, such as CS, tensile strength, FS, and MOE (Khan et al., 2020; Amin et al., 2022). The application of RSM in formulating the mixture proportions guarantees a robust for investigating the impacts of SD and SF on the advancement of the eco-friendly concrete composite. RSM analysis is anticipated to yield optimized mix designs that can facilitate the attainment of an optimal equilibrium among the constituent materials, thereby leading to a concrete composite that exhibits improved mechanical properties and decreased environmental footprint.
The ratios of the mix are specified in Table 2, corresponding to the mix ratios; the concrete composite cast was made by mixing its constituent materials in the specified ratios. The constituent materials include careful proportioning and mixing of cement, sand, SD, coarse aggregate, and SF in the predetermined ratios. In the mixing process, the whole amount of material was mixed for a period of 10 min, which was considered a full homogenization (Ananthkumar et al., 2019). The mixing allowed homogeneous dispersion, hence making possible adequate hydration and interplay between the cementitious components and the aggregates. To ensure and eliminate any potential variations that may arise from the mixing duration, the mixing time was therefore maintained at a constant level for all mix ratio. Upon completion of the procedure, the concrete composite was deemed suitable for the casting process (Ganapathy et al., 2022; Frazão et al., 2022). Accordingly, three cylindrical samples were prepared for each mix ratio used in the CS test at 28 days.
These were fabricated to meet the set standards of ensuring adequate densification and elimination of any trapped air in them. After this, they were placed under a controlled environment for the process of hydration to proceed and mechanical strength to develop. In the view of which, in addition to the above, conducting the CS test, a set of three cylindrical samples was also prepared for the STS test on each mix ratio after 28 days. The STS test brings forward the tensile properties of the concrete composite, giving valuable information for the assessment of its property against cracking and deformation capabilities (Abdul Awal et al., 2016b; Ganapathy et al., 2022). Furthermore, three beam specimens were prepared for each mix proportion to determine the FS test after 28 days. The beam specimens were manufactured according to established protocols to guarantee accurate measurements and correct casting for testing the flexural and bending capabilities of the concrete containing SD and SF (Mangi et al., 2019; Arjomandi et al., 2023). There is an assurance of the dependability and replicability of the experimental results in this study from adherence to standardized mixing and casting protocols. The above approach for sample preparation would facilitate getting precise results for the tested mechanical characteristics among various mix ratios of the eco-friendly concrete composite and comparing with each other.
The results from the tests that have been carried out on the sustainable concrete composite have been calculated for compressive strength as shown in Figure 1. The figure has shown certain differences in compressive strength in regard to the concrete composite through the use of various mixtures of SD and SF. The CS was found to be unique for each mix, such as, SD0%SF 0% (23.00 MPa), SD3%SF0.5% (23.50 MPa), SD6%SF1% (25.38 MPa), SD9%SF1.5% (17.03 MPa), SD12%SF2% (16.50 MPa), SD3%SF0% (21.00 MPa), SD6%SF0% (19.80 MPa), SD9%SF0% (16.75 MPa), SD12%SF0% (13.00 MPa), SD12%SF1% (14.30 MPa), SD3%SF1% (25.88 MPa), and SD6%SF2% (25.89 MPa). The effect on the strength properties of concrete with an incorporation of SD and SF in different ratios is significant (Kavya Sameera and Keshav, 2022; El-Nadoury, 2021). Examination of the results with previous research shows that the CSs achieved in the current investigation are in line with the patterns reported in previous scholarly works. A CS of 25.375 MPa is observed to be higher in the mixture of 6% SD -1% SF, indicating that the combination of both SD and SF in the mix is likely to be beneficial for the characteristics of strength. This discovery is consistent with prior research that has examined the impact of integrating SF and organic substances on the potency of concrete (Köksal et al., 2022; Kavya Sameera and Keshav, 2022). The observed rise in CS can be attributed to the synergistic chemistry between the fibrous structure of SD and the reinforcing properties of SF. Nevertheless, certain blend combinations, such as SD12%SF0% and SD9%SF1.5%, demonstrate reduced CS of 13 MPa and 17.03 MPa, correspondingly. The observed outcomes could be ascribed to the elevated proportions of SD or an inequity in the composition ratios, resulting in diminished strength properties. The optimization of mix design is of utmost importance in order to attain superior mechanical properties in such instances. The findings of this study exhibit the originality and distinctiveness of the research, as they furnish precise perspectives on the impact of amalgamating SD and SF on the compressive potency of eco-friendly concrete composites (Turner and Collins, 2013; Lehner et al., 2020). The aforementioned discoveries augment the existing corpus of knowledge concerning sustainable building materials and furnish valuable insights for the development and enhancement of concrete blends with enhanced mechanical characteristics.
The impact of SF and SD on the CS at 28 days of curing is illustrated in Figure 2through a 2D plot and a 3D diagram of responses. The data plots demonstrate that optimizing the SF concentration while minimizing the SD concentration results in a noteworthy enhancement in CS. The aforementioned results are consistent with the experimental observations presented in Figure 2, wherein mixtures containing greater proportions of SF exhibited elevated levels of CS (Awolusi et al., 2019; Shoughy, 2013). The contour plot and response surface diagram illustrate the positive impact of SF on CS and offer valuable insights for optimizing the blend proportions to attain improved strength characteristics in the eco-friendly concrete composite.
Figure 2. 2D contour plot (Left) and 3D response surface diagram (Right) of CS at 28 days of curing.
Figure 3 displays the outcomes of the STS examination conducted on the eco-friendly concrete composite. From the analyzed data, it is evident that there is a benefit in incorporating SF into the concrete composite in relation to the STS. Mixtures containing a higher percentage of SF show a higher tendency to show higher STS values, which indicate better tensile strength properties. An example can be given where Mix SD6%SF2% was a good sample with an STS value of 2.83 MPa, effectively indicating that a higher SF content can improve tensile strength. The STS was found to be unique for each mix, such as, SD0%SF 0% (2.25 MPa), SD3%SF0.5% (2.36 MPa), SD6%SF1% (2.54 MPa), SD9%SF1.5% (1.73 MPa), SD12%SF2% (1.48 MPa), SD3%SF0% (2.00 MPa), SD6%SF0% (1.79 MPa), SD9%SF0% (1.55 MPa), SD12%SF0% (1.40 MPa), SD12%SF1% (1.45 MPa), SD3%SF1% (2.78 MPa), SD6%SF2% (2.83 MPa). The above results agree with the work done by Awolusi et al. (2019) and Wang et al. (2023) in the respective area (Awolusi et al., 2019; Wang et al., 2023). Some studies have been undertaken that specifically dealt with concrete composites and, after the incorporation of SF and organic materials, recorded an increase in tensile strength. The fusion of SF with SD increases adhesion and weight-bearing ability and hence elevates STS metrics (He et al., 2022; Turner and Collins, 2013). The following results are to be confirmed and do stand valid as a method of increasing the tensile strength of concrete by using added naturalistic resources like SD and SF.
The effect of SF and SD on STS at 28 days is plotted on a 2D plot and 3D diagram of responses, which can be seen in Figure 4. The response surface diagram and contour plot would be able to describe the mixture proportion effect of SF and SD on the resultant STS values. The authors mentioned that when the SF component is optimized and the SD component is minimized, there is a significant improvement in STS (Lehner et al., 2020; Osei and Jackson, 2016). Graphical presentations give proof to validate the research findings indicating that there is an increase in the split tensile strength of the environmentally friendly concrete by the addition of steel fibers.
Figure 4. 2D contour plot (Left) and 3D response surface diagram (Right) of STS at 28 days of curing.
On the whole, results of these experiments, along with the contour plot and response surface diagram, point towards the beneficial influence of SF on the STS of the concrete composite. Results of this investigation are aligned with previous work and evidently indicate that there is more possibility for improvement in the mix compositions to obtain the desired tensile strength features (Shoughy, 2013; Turner and Collins, 2013). Integration of such sustainable elements as SD and SF may prove to be a useful material in creating ecologically sound concrete composites with superior performance properties.
The result of the FS test conducted with the sustainable concrete composite is displayed in Figure 5. It may thus be stated that the FS of the concrete composite may be influenced by the SD replacement level based on the test result. Mixtures in which the SF percentage was high showed a tendency to record higher FS values, meaning the FS properties were better. The FS was found to be unique for each mix, such as, SD0%SF 0% (9.42 MPa), SD3%SF0.5% (10.78 MPa), SD6%SF1% (5.22 MPa), SD9%SF1.5% (4.89 MPa), SD12%SF2% (4.55 MPa), SD3%SF0% (7.24 MPa), SD6%SF0% (4.77 MPa), SD9%SF0% (4.12 MPa), SD12%SF0% (3.30 MPa), SD12%SF1% (4.10 MPa), SD3%SF1% (10.94 MPa), and SD6%SF2% (5.78 MPa). The material Mix SD3%SF1% showed a notable FS value of 10.94 MPa, which means a higher content of SF resulted in effectively improving the capacity of the material to resist forces from bending. The above results agree with the previous research work, which reported an investigation of the effect of SF on the flexural strength of concrete composites (He et al., 2022). The literature consistently demonstrates that the incorporation of SF can augment the composite material’s capacity to withstand bending and cracking. Incorporating SF into the composite material enhances stress distribution and reinforces the matrix, leading to enhanced FS characteristics (Köksal et al., 2013; Kumar et al., 2021). The findings derived from this investigation corroborate the pre-existing pool of information and underscore the possibility of integrating supplementary cementitious materials into eco-friendly concrete blends, thereby augmenting their resistance to bending.
The 2D plot and 3D diagram of responses are shown in Figure 6 and illustrate the FS after curing for 28 days. From the response graphs, a pictorial form is able to show the relationship between the mixture proportions developed against their respective FS values. The result, shown in the contour plot and response surface diagram, unearths that the optimization of SF content and minimization of SD content bring a marked improvement in FS. The above plots show the critical significance of SF in augmenting the FS and give a coherent understanding of the most favorable mix design parameters. All these suggest that the inclusion of SF in concrete does have a beneficial effect on FS through the experimental findings, contour plot, and response surface diagram. Results are in accordance with the efficacy of SF in order to increase the resistance of the material against forces of deformation, as suggested in previous investigations (Osei and Jackson, 2016; Huda Suliman et al., 2019). Visual representation of the contour plot and response surface diagram both supported the relation between mix proportions and FS values, proving SF content optimization. Integration with this sustainable compound will be able to produce concrete composites that will have improved FS and more competent structural behavior.
Figure 6. 2D contour plot (Left) and 3D response surface diagram (Right) of FS at 28 days of curing.
The experimental results of MOE conducted on sustainable concrete composites are shown in Figure 7. The data analysis indicates a noticeable and significant effect of adding steel fibers (SF) on the modulus of elasticity (MOE) of the concrete. Mixtures containing a higher percentage of SF typically show raised MOE values, indicating raised stiffness and elastic characteristics. MOE was not experimentally calculated but was determined by the standard formula represented in Equation 1.
The MOE was found to be unique for each mix, such as, SD0%SF 0% (23.98 GPa), SD3%SF0.5% (24.24 GPa), SD6%SF1% (25.19 GPa), SD9%SF1.5% (20.63 GPa), SD12%SF2% (20.31 GPa), SD3%SF0% (22.91 GPa), SD6%SF0% (22.25 GPa), SD9%SF0% (20.46 GPa), SD12%SF0% (18.03 GPa), SD12%SF1% (18.91 GPa), SD3%SF1% (25.44 GPa), and SD6%SF2% (25.44 GPa). In the SD6%SF2% mix, there was an improvement in the MOE value of 25.44 GPa, which had a statistical significance value. This would, therefore, imply that the material showed improved stiffness and structural integrity.
These results were compatible with the previous research that investigated the effect of SF on the MOE of concrete composites. Several studies have concordantly reported that the inclusion of SF in a composite material increases its stiffness and resistance to deformation (Abdul Awal et al., 2016a; Amin et al., 2022). The addition of SF shows an increase in the loading capacity of the material and resistance to some external force. Hence, the MOE is improved significantly. The obtained results from the conducted study are in complete compliance with other studies that suggest SF can be well used as an improved, effective source of the mechanical properties in the sustainable concrete blend.
The MOE at 28 days of curing is illustrated in Figure 8 using both a 2D plot and a 3D response diagram. These graphical illustrations help visually justify the relationship between the mixing proportions and the resultant MOE values. The contour plot and response surface diagram are utilized to clearly illustrate the impact of varying levels of SF and SD on the modulus of elasticity (MOE) of the concrete composite. The diagrams show that maintaining a high content of SF while minimizing SD leads to a significant improvement in MOE (Devadasu et al., 2020; Abdul Awal et al., 2016b). This visualization underscores the critical role of SF in boosting the material’s rigidity and elasticity.
Figure 8. 2D contour plot (Left) and 3D response surface diagram (Right) of MOE at 28 days of curing.
Overall, the results of the experiments, along with the graphical representations, demonstrate that SF significantly affects the MOE of the eco-friendly concrete composite. These outcomes are consistent with prior research, confirming SF’s effectiveness in increasing the stiffness and resistance to deformation of the material (Amin et al., 2022; Frazão et al., 2022). The contour plots and response surface diagrams visually reinforce the relationship between the mix proportions and MOE, highlighting the need for optimizing SF content. This approach lays a robust foundation for developing concrete composites with enhanced rigidity and superior structural performance through the sustainable integration of SD and SF.
Assessment of concrete environmental impact: this greatly depends on the correct calculation of the EC of concrete. The “embodied carbon” includes all the carbon dioxide (CO2) emissions that are attributed to the production and transportation of materials for use in concrete composition. The current study found the individual EC values of the materials for the determination of overall EC associated with each mixture. To this end, the resulting quantities of materials used in the mixture proportions were multiplied by their corresponding EC values in order to ascertain the EC associated with each mixture (He et al., 2022; Lehner et al., 2020). Thus, for mix SD6%SF1%, respective quantities of cement, SD, fine aggregate (FA), and coarse aggregate (CA) are to be multiplied by their respective EC values. The aggregate EC linked with this particular blend is subsequently the result of adding the respective contributions. The EC metrics allow incorporating the ecological footprint assessment associated with single blends. It is in these lines that the findings may offer significant perspectives to the carbon emissions linked to the manufacturing and utilization of the concrete composite (Huda Suliman et al., 2019; Zaid et al., 2021). It enables researchers and professionals to easily compare the different blends and come up with easily identifiable alternatives that will portray a reduced ecological footprint.
A properly designed concrete structure with the concept of EC is of immense importance toward moving forward with sustainability and reducing the emission of greenhouse gases. This makes it feasible to manufacture concrete composites that will show the reduced level of EC by optimal combinations of ingredients and contribute significantly to the efforts aimed at the spread of such mitigating climate changes (Bolooki Poorsaheli et al., 2021; Waqar et al., 2023b). Thus, derived EC metrics will allow the evaluation of the ecological efficiency for the new eco-friendly concrete composite and allow informed judgments in the choice and formulation of materials.
As seen in Figure 9 SD0%SF0% is having the minimum EC of the aggregates, quantified to be 210.081 kg of carbon dioxide. The material used comprises cement, sand, and coarse aggregate, but without any SD and SF. The omission of SD and SF results in a significant decrease in the EC in comparison to alternative mixtures that incorporate these constituents. SD3%SF0.5% comprises a minute proportion of SD (0.378 kg) and a minimal percentage of SF (0.5%), resulting in a total EC of 211.704 kg CO₂. The incorporation of SD and SF results in a marginal increase in the EC, albeit insignificant owing to the limited amounts employed. The aggregate EC content of SD6%SF1% mixture amounts to 213.238 kg of carbon dioxide. The composition comprises an elevated proportion of SD, specifically 0.756 kg, and a 1% concentration of SF. The augmented quantities of said materials lead to a greater EC in contrast to the preceding blends. SD9%SF1.5% indicates a cumulative EC of 214.906 kg of carbon dioxide. The composition comprises a greater ratio of SD, weighing 1.134 kg, and SF, constituting 1.5% of the mixture. The augmented quantities of said materials result in an elevated carbon footprint in contrast to the preceding blends. SD12%SF2% exhibits a significantly elevated EC value of 216.485 kg CO₂, which can be attributed to the comparatively larger proportions of SD (1.512 kg) and SF (2%) present within its composition (He et al., 2022; Lehner et al., 2020). The augmented ratios of these substances substantially contribute to the elevated carbon footprint observed in this blend.
The findings of the critical analysis indicate that there is a positive correlation between the quantities of SD and SF in the mixes and the EC. The utilization of SD, a byproduct of the timber industry, exhibits a comparatively reduced EC in contrast to cement and SF. On the other hand, SF exhibit an elevated EC as a result of the energy-intensive production procedures implicated in steel fabrication. The dissimilarities in EC across the mixes can be ascribed to the differing ratios of SD and SF. The evaluation of the performance-enhancing attributes of SD and SF and their corresponding EC is a crucial consideration. The optimal mix proportions should endeavor to attain a harmonious equilibrium between the attainment of targeted mechanical characteristics and the reduction of EC. Overall, the EC values of the mixtures are consistent with prior research that has emphasized the influence of diverse materials on carbon emissions (Basser et al., 2021; Shoughy, 2013). The findings accentuate the significance of the selection of materials and the design of the mixture in mitigating the ecological impact of concrete constructions. Sustainable concrete solutions can be attained through the optimization of mix proportions, taking into account both performance and environmental impact (Lehner et al., 2020; Osei and Jackson, 2016). Further, Figure 10 indicates the 2D contour plot and response surface diagrams constructed on the basis of EC results. It is clearly indicated that addition of steel fiber have resulted in increasing the EC of concrete because of its high value of EC as compared to other materials in concrete. The comparative impact of addition of steel fiber and SD shows that SF are not suited for minimizing EC after certain level. SD shows less impact or change in EC because of its low EC value.
Figure 10. 2D contour plot (Left) and 3D response surface diagram (Right) of EC at 28 days of curing.
The Eco-strength efficiency (ESE) metric is utilized in evaluating the environmental effectiveness of concrete blends. This is achieved by analyzing the ratio of CS to the total EC. The ESE values were computed for every Mix ID, which denotes distinct ratios of SD and SF in the concrete mixture, as indicated in Figure 11. By examining the ESE values, one can obtain valuable insights regarding the influence of incorporating SD and steel fiber on the environmental efficacy of the concrete blends. Mixtures containing lower proportions of SD and SF, such as SD0%SF0%, SD3%SF0.5%, and SD3%SF0%, demonstrate comparatively elevated ESE values (0.109, 0.111, and 0.100, respectively). The aforementioned blends exhibit superior ecological robustness efficacy, denoting an elevated compressive potency attained per EC unit. Incorporating restricted quantities of SD and SF into these blends facilitates a more eco-friendly equilibrium between potency efficacy and EC (Kropidłowska, 2022; Lehner et al., 2020). An upward trend in ESE values is observed as the proportions of SD and SF in the mix design increase. The blends comprising SD6%SF1%, SD9%SF1.5%, and SD12%SF2% exhibit reduced ESE values, specifically 0.119, 0.079, and 0.076, correspondingly. Elevated levels of SD and SF lead to a rise in the EC content, thereby diminishing the efficacy of eco-strength. This implies that although these blends may demonstrate enhanced mechanical characteristics, they are accompanied by elevated levels of carbon emissions. It is noteworthy that the blends that lack SF addition, namely, SD6%SF0%, SD9%SF0%, and SD12%SF0%, exhibit comparatively reduced ESE values (0.094, 0.079, and 0.062, respectively). The findings suggest that the eco-strength efficiency is only marginally improved when SD is used as the sole reinforcement material in the absence of SF. The text highlights the importance of SF in enhancing the mechanical characteristics of a material while simultaneously maintaining a balance with environmental factors (Shoughy, 2013; He et al., 2022). Mixtures with higher levels of SF, such as SD12%SF1% and SD6%SF2%, show moderate Eco-Strength Efficiency (ESE) values of 0.067 and 0.120, respectively. These mixtures successfully achieve a balance between enhanced strength properties through the incorporation of SF and effective control of the associated EC.
The findings indicate that attaining an optimal eco-strength efficiency in concrete necessitates a meticulous choice of mix proportions, particularly with regards to the addition of SD and SF. Through the reduction of SD and steel fiber quantities or the utilization of sustainable material alternatives, it is feasible to attain elevated ESE values and mitigate the ecological repercussions of concrete (Prabath and Ramadoss, 2022; Zarei et al., 2022). The aforementioned results are consistent with prior research that has emphasized the compromises between strength-related capabilities and the amount of carbon embodied in a given system. The findings underscore the significance of taking into account sustainable materials and mix designs in order to attain eco-efficient concrete solutions that strike a balance between mechanical properties and environmental factors (Waqar et al., 2023a; Wang et al., 2023). The same effect is indicated in Figure 12 where both 2D plot and 3D response diagram show reduction in ESE as SF content is increased as compared to addition of SD.
Figure 12. 2D contour plot (Left) and 3D response surface diagram (Right) of ESE at 28 days of curing.
Table 3 presents the ANOVA table, which furnishes statistical data pertaining to the significance of the response surface model concerning CS. The aforementioned statement implies that the model adeptly captures the correlation between the variables A-SD and B-SF, and the dependent variable, CS. Moreover, it can be observed that the individual factors A-SD and B-SF exhibit significant impacts on the CS, as indicated by the p-value being less than 0.0001. The CS of the concrete mix is significantly affected by two factors, namely, SD (A-SD) and SF (B-SF). The statistical analysis reveals that the interaction term AB, involving A-SD and B-SF, significantly impacts the compressive strength (CS) of the material, evidenced by a p-value of 0.0315. This analysis includes details about the residual inconsistency, which denotes the unaccounted-for variation remaining after applying the model, with a residual mean square value of 1.62 highlighting the magnitude of unexplained variability. This residual variability arises from the components of lack of fit (LOF) and pure error (Kropidłowska, 2022; He et al., 2022).
Further, ANOVA statistics for split tensile strength (STS) demonstrate the statistical significance of both the model and its individual factors. The model shows a very high level of significance (p-value <0.0001), indicating its capability to accurately represent the observed fluctuations in STS. The factors A-SD (SD), B-SF (SF), and their combined effect AB all exhibit remarkable impacts on STS, with p-values less than 0.0001, confirming their high significance as shown by their high F-values and low p-values (Gao et al., 2022; Khan et al., 2022). The residual variability, encompassing lack of fit and pure error, is comparatively small relative to the explained variation of the model. In summary, the ANOVA results validate the model’s effectiveness and the significant influence of the variables A-SD, B-SF, and their interaction on the mechanical properties of the material, particularly affecting split tensile strength.
ANOVA on Flexural Strength (FS) reveals that both the model and individual factors exhibit significant statistical influence. The statistical model is highly significant (p-value <0.0001), indicating its capability to explain observed variations in FS. Notably, factors A-SD (SD) and A2 (squared SD) are identified as having considerable impact on FS, supported by their low p-values of less than 0.0001 and 0.0008, respectively. The B-SF factor, related to the inclusion of SF, shows a comparatively elevated p-value of 0.0667, indicating a lesser influence on FS. p-values greater than 0.05 for the interaction term AB and the squared factor B2 suggest these factors do not significantly affect FS (Zarei et al., 2022; Lee et al., 2022).
For Modulus of Elasticity (MOE), the ANOVA demonstrates the model’s high level of significance (p-value <0.0001), affirming its effectiveness in explaining variability in MOE. Both A-SD and B-SF are significant factors affecting MOE, as evidenced by their very low p-values (less than 0.0001 and 0.0002, respectively) (Kropidłowska, 2022; Zarei et al., 2022). The residual variability, which includes lack of fit and pure error, is relatively small compared to the explained variation, underscoring the model’s adequacy (Khan et al., 2022; Köksal et al., 2022).
In terms of Embodied Carbon (EC), ANOVA findings also show a high level of significance for the model (p-value <0.0001), highlighting its capacity to account for observed fluctuations in EC. Both A-SD and B-SF significantly influence EC, as indicated by their low p-values (less than 0.0001). However, the interaction term AB and the squared terms A2 and B2 do not show significant impacts on EC, demonstrating the primary influence of individual factors A-SD and B-SF on EC (Prabath and Ramadoss, 2022; Lee et al., 2022). Overall, ANOVA results validate the significance of the statistical model and highlight the considerable impact of individual factors and their combinations in predicting the mechanical properties and environmental impact of concrete composites, guiding material selection and mixture optimization for enhanced sustainability.
For eco-strength efficiency, the statistical significance of the model is notable, with a p-value of less than 0.0001, indicating the model’s strong ability to explain the observed variations in eco-strength efficiency. Both A-SD (SD) and B-SF (SF) significantly influence eco-strength efficiency, as shown by their low p-values (<0.0001). Moreover, the interaction term AB also shows a significant impact on eco-strength efficiency, evidenced by its p-value of 0.0364 (Wang et al., 2023; Fattouh et al., 2023). The residual variability, which includes components of lack of fit and pure error, is minimal compared to the variation explained by the model (Gao et al., 2022; Lee et al., 2022). This robust statistical foundation supports the model’s effectiveness in assessing and optimizing eco-strength efficiency, demonstrating the significant influence of both individual material components and their interactions.
From Table 4, the validation metrics of the models would include the R-squared, Adeq Precision, and others for each response variable. Coefficient of determination, as commonly referred to, R-squared, denotes how the variations in a dependent variable are explained by one or several independent variables in a model. Adeq Precision.
Adeq Precision performs evaluation using signal-to-noise ratio and checks the suitability of the model for predictive purposes (Kropidłowska, 2022; Abdulridha et al., 2022). Coefficient of determination (R-squared) is 0.9413 for CS, which explains that this model can independently explain about 94.13% of the variations in CS. The R-squared coefficient being high shows a strong relationship between independent and dependent variables that are CS’s variables. Adeq Precision is 19.7471, which indicates that the model is predicting CS at a satisfactory level of precision. The Adeq Precision value for Adeq Precision should be generally above 4 (Wang et al., 2023; Fattouh et al., 2023). Coefficient of determination (R-squared) for the STS model is 0.9744, an indication that the model explains about 97.44% of the total variation observed in STS. A high value of R-squared is an indication of a very good relationship between the predictors and STS. The Adeq Precision value of 28.0558 infers that the model has an excellent level of precision when predicting STS. The coefficient of determination (R-squared) for FS is at 0.9464, hence about 94.64% of the variation observed in FS may be explained by the model. High R-squared means a strong association between the independent variables and the dependent variable, i.e., FS (Kropidłowska, 2022; Abdulridha et al., 2022). An Adeq Precision score of 14.9179 suggests that the level of precision the model possesses in forecasting FS is moderate. While this is the case of MOE, the coefficient of determination (R-squared) is 0.9207, pointing towards a fact that the model can explain about 92.07% of the variations observed in MOE. Adeq Precision at the value of 22.3676 indicates that the model has the precision in prediction of MOE with a high degree of precision (Wang et al., 2023; Fattouh et al., 2023).
The relationship between the predictors and EC is very strong, with the R-squared at 0.9999. The R-squared coefficient is high, hence indicating a large fraction of the variation in EC is explained by the model. Adeq Precision score is 392.696, which signifies that the model has precision in predicting EC to a very high degree. The R-squared of 0.9412 for ESE indicates that the model gives an indication of about 94.12% variability within ESE. A score of 19.6894 for the Adeq Precision suggests that the model has a high precision level in predicting ESE (Zarei et al., 2022; Mujalli et al., 2022).
In general, the relationship among all the predictors and the response is strong, indicated by large R-square values. In addition, the values of Adeq Precision show the models are able to make predictions within a limit of precision for the respective response variable (Osei and Jackson, 2016; Ghafari et al., 2014). The data given proves statistically the dependability and effectiveness of the response surface models in predicting the properties of the concrete mixes.
Equation 2 through (7) have been derived using ANOVA and model validation statistics. The above six equations represent mathematical relationships between the predictor variables A and B and their respective response variables CS, STS, FS, MOE, EC, and ESE. The coefficients in the above equations were determined through statistical analysis. Also, these statistical indicators used to validate the model, such as R-squared, Adequate Precision, and other metrics, are evaluations of the effectualness and preciseness of the model in forecasting the responses (Dilip et al., 2014; Abdul Awal et al., 2016b). The above statistics validate the reliability and predictability of the developed equations. The above mathematical Equations 2–7 are reliable representations of the relationships that exist between the predictor variables and the response variables. Further support even comes from ANOVA and validation model statistics, depicting the reliability of the source.
The predicted versus actual plot and the normal probability plot of residuals are two important graphical methods used in model validation and checking the adequacy of fit of regression models. These are presented from Figures 13–18. The normal percentage probability in relation to externally standardized residuals and the correlation between the expected and actual values are illustrated by these instruments, respectively. The present study involved the construction of plots for a set of response variables, namely, CS, STS, FS, MOE, EC, and ESE. The customary approach for visualizing residuals involves plotting the externally studentized residuals against their corresponding normal percentage probabilities on a normal plot. Assuming normality of residuals, the data points depicted on the graph are expected to cluster near the linear regression line. Uniform distribution of points along a line is indicative of normal distribution of residuals, thereby confirming the model’s assumption of normality. This implies that the model effectively encompasses the diversity present in the dataset (Osei and Jackson, 2016; Abbass et al., 2018).
The plot depicting predicted versus actual values facilitates a comparison between the predicted values derived from the regression model and the observed values. Ideally, the alignment of points on the plot should be in close proximity to the diagonal line, which signifies a high level of concordance between the predicted and actual values. When the data points exhibit a tight clustering around the diagonal line, it is indicative of a favorable correspondence between the prognostications of the model and the actual observed data.
The uniform distribution of points on or near the standard line in both plots indicates a favorable goodness-of-fit for the regression models of CS, STS, FS, MOE, EC, and ESE. The normality assumption is validated by the normal distribution of residuals as depicted in the normal plot (Memon et al., 2017; Memon et al., 2018). The plot comparing predicted values to actual observations demonstrates a high degree of correspondence between the model’s predictions and the observed values, suggesting that the models are dependable and precise in forecasting the response variables (Turner and Collins, 2013; Collins, 2010). In general, the aforementioned plots offer empirical support that the regression models pertaining to CS, STS, FS, MOE, EC, and ESE are appropriately fitted and may be deemed suitable for subsequent scrutiny and explication.
Table 5 displays the statistical data pertaining to multi-objective optimization, wherein diverse input factors (SD and SF percentages) are optimized with the aim of attaining the desired objectives for the output factors (namely, CS, split tensile strength, FS, MOE, EC, and eco-strength efficiency). The objective of optimization outcomes is to identify the most favorable values of the input variables that satisfy the predetermined objectives for the output variables. The variables SD and SF denote the proportions of two distinct constituents within the amalgam.
These comprise the first range of SD values, which will be from 0% to 12% and the second range of SD values at 0%–2% for SF values, and in so doing, then form the boundaries for the specified values for the optimization process. The output factors deal with the varied attributes and characteristics found in the concrete composition. The specified objectives for each factor are defined as “in-range” or within a certain target range (Ahmed, 2017; Memon et al., 2017). The optimization process aims at reducing the number of ECs while improving the strength-related characteristics and, at the same time, eco-strength effectiveness (Abbass et al., 2018; Mangi et al., 2019).
These values obtained for the output factors were the outcome of this optimization process. It has been found in this study that values of other concrete strength parameters such as CS, split tensile strength, and FS were within an acceptable limit along with MOE. The study also evaluated EC, giving the optimized values of 3.67, and eco-strength efficiency, giving the optimized values of 18.027. These findings are helping in understanding the performance of concrete and its effect on the environment (Sharba et al., 2022; Chigozie, 2022). The above values give the best achievable values of output factors with respect to the given ranges of input factors.
It is an overall quality of the optimized solution, with the desirability achieving 0.934. In fact, the numerical value equal to 1 would indicate that all the output factors have accurately achieved their intended objectives with the greatest possible precision. The obtained desirability value of 0.934 shows that the optimized solution is really very desirable, seeing how closely it approaches the desired objective for the output factors. It is the process of multi-objective optimization that provides compromise points toward the equilibrium among diverged objectives, which are conflicting among themselves (He et al., 2022; Fattouh et al., 2023). Its nature is to give and take among the merits and demerits of a variable in order to reach an optimal resolution. The reason for this process of optimization in this case is to find the favorable amalgamation of input factors, which will make available for maximum strength properties with minimum EC and the best utilization of materials. This is further reinforced by the outcomes of multi-objective optimization that tend to suggest that it is theoretically viable to have a suitable concrete mix with the desired properties with a careful proportion of SD and SF (Wang et al., 2023; Fattouh et al., 2023). Through the optimization of input variables, it is feasible to achieve a blend that satisfies predetermined objectives for strength characteristics, EC, and eco-strength efficiency, thereby presenting a concrete solution that is more sustainable and efficient.
The ramp diagram, indicated in Figure 19, is a visual depiction that illustrates the outcomes of the optimization procedure. The graphical representation illustrates the relationship between the output factors, namely, CS, split tensile strength, FS, MOE, EC, and eco-strength efficiency, and the input factors, specifically the SD and SF percentages, as they undergo variation. The diagram depicting a ramp showcases the correlation between the input and output variables, accentuating the most favorable values attained via the process of optimization (Waqar et al., 2023a; Waqar et al., 2023b).
Table 6 displays the anticipated and observed outcomes for diverse response parameters, encompassing CS, split tensile strength, FS, and MOE. The analysis reveals a noticeable discrepancy between the anticipated and empirical outcomes for every response parameter. The expected CS is 13.85 MPa, while the empirical value ranked slightly higher at 14.3 MPa, hence percentage error ranked at 3.24%. The results show a marginal difference in split tensile strength, where the expected value of 1.4 MPa is little smaller compared to the observed experimental value of 1.43 MPa, thereby giving an error of 2.14%. The FS, however, of imprecision is slightly higher, as shown by the estimated value of 3.67 MPa compared to the empirical value of 3.5 MPa, leading to a difference of 4.63%. Lastly, MOE presents an empirical point of 17.5 GPa, comparing the lowest levels of discrepancy with those of its estimation at 18.027 GPa, with an error in measurement of 2.92%.
The noted errors, therefore, between the predicted and experimental values suggest the presence of a discrepancy in some of the model parameters by the RSM against the real results from the experiment. The differences between the two experiments could be ascribed to different factors, for example, changes in the experimental conditions or limitations of the model’s prediction capacity, or even hidden variables that influence the dependent variables (Kropidłowska, 2022; Zarei et al., 2022). Most importantly, it is critically important to assess the influence level these errors could have on the model’s overall performance through a critical analysis (Waqar et al., 2023b; Fattouh et al., 2023). This easily reveals the magnitudes of the errors to enable one to judge the reliability and accuracy of the RSM model, hence finding areas of improvement. Further studies could be conducted to identify the parameters responsible for the deviations and, hence, model improvement in order to further the forecast precision (Kropidłowska, 2022; Abdulridha et al., 2022). The terms computed through RSM were found to be a very good framework for fitting response variables. Nevertheless, the existence of such errors simply reinforces the importance of validating and improving the model with further experimentation to make sure it is accurate and reliable.
The results of this research on sustainable concrete formulated with sawdust and steel fiber have practical implications for the construction industry. The improved mechanical properties, such as enhanced compressive strength, split tensile strength, flexural strength, and modulus of elasticity, show the potential for creating stronger and more durable concrete structures. By incorporating sawdust and steel fibers, the performance of the concrete is not only improved, but it also addresses sustainability by reducing embodied carbon and enhancing eco-strength efficiency. This innovative approach offers a practical solution for developing eco-friendly concrete, paving the way for construction projects to meet higher sustainability standards while maintaining structural integrity and longevity.
The objective of the study was to assess the effect of different combinations of SD and SF on the mechanical properties of environmentally sustainable concrete through the aid of RSM. This research aligns with the global sustainability goals. This study utilizes the SD waste that help in reducing the waste and incorporating that waste in concrete as a sustainable material for construction, paving the way for innovative and sustainable construction material. The study put forward an analysis regarding the mechanical properties of concrete and quantification of EC, a more accurate model of RSM, and the best proportional combination of materials to produce the sustainable concrete needed for the work.
• For response surface models formation and multi response optimization, the input variables for RSM consists of two independent variables Saw dust (0%–12%) and Steel fiber (0%–2%); while dependent variables are CS, STS, FS, MOE, EC and ESE, which are predicted and adjusted.
• 2FI models were deemed significant for CS, STS and ESE, while quadratic models were deemed significant for FS and EC and liner model was significant for MOE.
• After conducting an analysis of variance (ANOVA) with a 95% confidence level and a 5% significance level, it was found that the response models were statistically significant, as indicated by the coefficient of determination (R2). This finding held true even though the significance level was set at 5%, which is below the 95% confidence threshold. For all the responses that were taken into consideration, R2 values ranging from 92.07% to 99.99%.
• Experimental investigation has revealed that addition of 6% of saw dust with 2% steel fiber in concrete enhances the CS, STS and MOE by 12.56%, 25.78% and 6% respectively. Addition of steel fibers improves the mechanical characteristics of concrete because of bridging the cracks and fiber provides the additional support which results in improving the mechanical properties.
• The RSM model showed a high accuracy in predicting the reduced emission regarding EC. Model validation through statistical analysis came with a considerably high value of R2, rated at 0.9999, showing a strong association between the variables. Further justification was on the validation of the model since the predicted responses closely approximated the experimental data. ESE, on the other hand, obtained acceptable results from the analysis. On their part, the R2 value for the RSM model was at 0.9412, whereas the precision of adequacy was 19.6894. The prediction made by ESE was very accurate; the errors in percent were very low, at 0.065%. Based on the carried out model validation and statistical ANOVA tests, the model’s statistical significance and accuracy for all considered variables have been confirmed.
• The findings of multi-response optimization have revealed that the variables attained their optimum values as follows: saw dust exhibits a concentration of 11.811%, whereas steel fibers have a concentration of 0.063%. The aforementioned settings successfully achieved the intended enhancement in characteristics while maintaining responsiveness. The validation experiment of the models demonstrated a mismatch of under 5% among the actual and anticipated values of responses.
To evaluate the long-term performance of eco-friendly concrete, full-scale durability tests are essential, focusing on freeze-thaw cycles, chemical degradation, and mechanical stress resistance. Optimizing mix design through adjustments in water-cement ratio, aggregate gradation, and additives can enhance workability, strength, and durability while reducing embodied carbon. Implementing genetic algorithms or machine learning can further refine the mix. Life cycle assessment (LCA) combined with cost analysis can compare the environmental impacts of eco-friendly concrete to conventional materials, aiding in the adoption of sustainable construction practices.
The raw data supporting the conclusions of this article will be made available by the authors, without undue reservation.
AW: Conceptualization, Data curation, Formal Analysis, Methodology, Visualization, Writing–original draft. MK: Conceptualization, Data curation, Investigation, Methodology, Software, Validation, Writing–review and editing. TN: Funding acquisition, Project administration, Resources, Supervision, Writing–review and editing. HA: Supervision, Validation, Visualization, Writing–review and editing. OB: Funding acquisition, Resources, Supervision, Writing–review and editing.
The author(s) declare that financial support was received for the research, authorship, and/or publication of this article. This research was funded by Taif University, Saudi Arabia, Project No. (TU-DSPP-2024-33).
The authors extend their appreciation to Taif University, Saudi Arabia, for supporting this work through project number (TU-DSPP-2024-33).
The authors declare that the research was conducted in the absence of any commercial or financial relationships that could be construed as a potential conflict of interest.
All claims expressed in this article are solely those of the authors and do not necessarily represent those of their affiliated organizations, or those of the publisher, the editors and the reviewers. Any product that may be evaluated in this article, or claim that may be made by its manufacturer, is not guaranteed or endorsed by the publisher.
Abbass, W., Khan, M. I., and Mourad, S. (2018). Evaluation of mechanical properties of steel fiber reinforced concrete with different strengths of concrete. Constr. Build. Mater. 168, 556–569. doi:10.1016/j.conbuildmat.2018.02.164
Abdul Awal, A. S. M., Mariyana, A. A. K., and Hossain, M. Z. (2016a). Some aspects of physical and mechanical properties of sawdust concrete. Int. J. GEOMATE. doi:10.21660/2016.21.5304
Abdul Awal, A. S. M., Mariyana, A. A. K., and Hossain, M. Z. (2016b). Some aspects of physical and mechanical properties. Int. J. GEOMATE.
Abdulhameed, A. A., Hason, M. M., Sharba, A. A. K., Hanoon, A. N., Amran, M., Magbool, H. M., et al. (2023). Experimental and environmental investigations of the impacts of wood sawdust on the performance of reinforced concrete composite beams. Case Stud. Constr. Mater. 19, e02550. doi:10.1016/j.cscm.2023.e02550
Abdulridha, M., A., Salman, M., Banyhussan, M. ; S., and Effect, Q. (2022). Polypropylene of fiber on drying shrinkage cracking of concrete pavement using response surface methodology. J. Eng. Sustain. Dev. doi:10.31272/jeasd.25.3.2
Ahmad, J., Zhou, Z., and Deifalla, A. F. (2023). Steel fiber reinforced self-compacting concrete: a comprehensive review. Int. J. Concr. Struct. Mater. 17, 51. doi:10.1186/s40069-023-00602-7
Ahmad Farooq, M., Alomayri, T., Ali, B., Sultan, T., and Yosri, A. M. (2023). Influence of hybrid coir-steel fibres on the mechanical behaviour of high-performance concrete: step towards a novel and eco-friendly hybrid-fibre reinforced concrete. Constr. Build. Mater. 389, 131728. doi:10.1016/j.conbuildmat.2023.131728
Ahmed, M. (2017). Effect of some additives on mechanical and physical properties of cob building in Iraq. IOSR J. Mech. Civ. Eng. 14, 65–71. doi:10.9790/16841402066571
Alabduljabbar, H., Huseien, G. F., Sam, A. R. M., Alyouef, R., Algaifi, H. A., and Alaskar, A. (2020). Engineering properties of waste sawdust-based lightweight alkali-activated concrete: experimental assessment and numerical prediction. Mater. (Basel) 13, 5490. doi:10.3390/ma13235490
Amin, M. N., Ahmad, W., Khan, K., and Ahmad, A. (2022). Steel fiber-reinforced concrete: a systematic review of the research progress and knowledge mapping. Mater. (Basel) 15, 6155. doi:10.3390/ma15176155
Ananthkumar, M., Yuvan Karthick, M. C., and Rajashekar, A. (2019). “Steel incorporated sawdust concrete,” in Proceedings of the IOP conference series: materials science and engineering.
Arjomandi, A., Mousavi, R., Tayebi, M., Nematzadeh, M., Gholampour, A., Aminian, A., et al. (2023). The effect of sulfuric acid attack on mechanical properties of steel fiber-reinforced concrete containing waste nylon aggregates: experiments and RSM-based optimization. J. Build. Eng. 64, 105500. doi:10.1016/j.jobe.2022.105500
Awolusi, T. F., Oke, O. L., Akinkurolere, O. O., and Sojobi, A. O. (2019). Application of response surface methodology: predicting and optimizing the properties of concrete containing steel fibre extracted from waste tires with limestone powder as filler. Case Stud. Constr. Mater. 10, e00212. doi:10.1016/j.cscm.2018.e00212
Babaie, R., Abolfazli, M., and Fahimifar, A. (2020). Mechanical properties of steel and polymer fiber reinforced concrete. J. Mech. Behav. Mater. 28, 119–134. doi:10.1515/jmbm-2019-0014
Baserah, S. J., Bashar, N. A. M., and Min, Y. H. (2021). Reinforced concrete slab with added steel fibers for engineering application: preliminary experimental investigations. Civ. Eng. Archit. 9, 128–137. doi:10.13189/cea.2021.091315
Basser, H., Shaghaghi, T. M., Afshin, H., Ahari, R. S., and Mirrezaei, S. S. (2021). An experimental investigation and response surface methodology-based modeling for predicting and optimizing the rheological and mechanical properties of self-compacting concrete containing steel fiber and PET. Constr. Build. Mater. 315, 125370. doi:10.1016/j.conbuildmat.2021.125370
Batool, F., Islam, K., Cakiroglu, C., and Shahriar, A. (2021). Effectiveness of wood waste sawdust to produce medium-to low-strength concrete materials. J. Build. Eng. 44, 103237. doi:10.1016/j.jobe.2021.103237
Bernardo, L. F. A., Filho, B. M. V. C., and Horowitz, B. (2021). Efficient softened truss model for prestressed steel fiber concrete membrane elements. J. Build. Eng. 40, 102363. doi:10.1016/j.jobe.2021.102363
Bolooki Poorsaheli, H., Behravan, A., and Tabatabaei Aghda, S. T. (2021). Durability performance of hybrid reinforced concretes (steel fiber + polyolefin fiber) in a harsh marine tidal zone of Persian gulf. Constr. Build. Mater. 266, 121176. doi:10.1016/j.conbuildmat.2020.121176
Bošnjak, J., Sharma, A., and Grauf, K. (2019). Mechanical properties of concrete with steel and polypropylene fibres at elevated temperatures. Fibers 7, 9. doi:10.3390/FIB7020009
Chigozie, O. I. (2022). Properties of sawdust concrete. J. Build. Mater. Sci. 4, 1–9. doi:10.30564/jbms.v4i2.4818
Collins, F. (2010). Inclusion of carbonation during the life cycle of built and recycled concrete: influence on their carbon footprint. Int. J. Life Cycle Assess. 15, 549–556. doi:10.1007/s11367-010-0191-4
Da Silva, T. R., Cecchin, D., De Azevedo, A. R. G., Alexandre, J., Valadão, I. C. R. P., Bernardino, N. A., et al. (2021). Soil-cement blocks: a sustainable alternative for the reuse of industrial solid waste. Rev. Bras. Ciências Ambient. 56, 673–686. doi:10.5327/z21769478956
Devadasu, S., Sonawane, S. H., and Suranani, S. (2020). “Self-healing corrosion inhibition coatings with PH-responsive activity by incorporation of Nano cellulose in two pack epoxy polyamide system,” in Proceedings of the materials today: proceedings.
Dilip, K., Singh, S., Kumar, N., and Gupta, A. (2014). Low cost construction material for concrete as sawdust. Glob. J. Res. Eng.
El-Nadoury, W. W. (2021). Production of sustainable concrete using sawdust. Mag. Civ. Eng. doi:10.34910/MCE.105.7
Fattouh, M. S., Tayeh, B. A., Agwa, I. S., and Elsayed, E. K. (2023). Improvement in the flexural behaviour of road pavement slab concrete containing steel fibre and silica fume. Case Stud. Constr. Mater. 18, e01720. doi:10.1016/j.cscm.2022.e01720
Frazão, C., Barros, J., Bogas, J. A., García-Cortés, V., and Valente, T. (2022). Technical and environmental potentialities of recycled steel fiber reinforced concrete for structural applications. J. Build. Eng. 45, 103579. doi:10.1016/j.jobe.2021.103579
Ganapathy, G. P., Keshav, L., Ravindiran, G., and Razack, N. A. (2022). Strength prediction of self-consolidating concrete containing steel fibre with different fibre aspect ratio. J. Nanomater. 2022. doi:10.1155/2022/7604383
Gao, Y., Wang, B., Liu, C., Hui, D., Xu, Q., Zhao, Q., et al. (2022). Experimental investigation on static compressive toughness of steel fiber rubber concrete. Rev. Adv. Mater. Sci. 61, 576–586. doi:10.1515/rams-2022-0260
Ghafari, E., Costa, H., and Júlio, E. (2014). RSM-based model to predict the performance of self-compacting UHPC reinforced with hybrid steel micro-fibers. Constr. Build. Mater. 66, 375–383. doi:10.1016/j.conbuildmat.2014.05.064
He, H., Cheng, S., Chen, Y., and Lan, B. (2022). Compression performance analysis of multi-scale modified concrete based on response surface method. Case Stud. Constr. Mater. 17, e01312. doi:10.1016/j.cscm.2022.e01312
Helal, Z., Salim, H., Ahmad, S. S. E., Elemam, H., Mohamed, A. I. H., and Elmahdy, M. A. R. (2024). Sustainable bacteria-based self-healing steel fiber reinforced concrete. Case Stud. Constr. Mater. 20, e03389. doi:10.1016/j.cscm.2024.e03389
Huda Suliman, N., Atif Abdul Razak, A., Mansor, H., Alisibramulisi, A., and Mohd Amin, N. (2019). Concrete using sawdust as partial replacement of sand: is it strong and does not endanger health? MATEC Web Conf. 258, 01015. doi:10.1051/matecconf/201925801015
Karimipour, A., Ghalehnovi, M., Golmohammadi, M., and de Brito, J. (2021). Experimental investigation on the shear behaviour of stud-bolt connectors of steel-concrete-steel fibre-reinforced recycled aggregates sandwich panels. Mater. (Basel) 14, 5185. doi:10.3390/ma14185185
Kavya Sameera, V., and Keshav, L. (2022). Properties and performance of steel fiber reinforced concrete beam structure – review. Mater. Today Proc. 66, 916–919. doi:10.1016/j.matpr.2022.04.643
Khan, E. U., Khushnood, R. A., and Baloch, W. L. (2020). Spalling sensitivity and mechanical response of an ecofriendly sawdust high strength concrete at elevated temperatures. Constr. Build. Mater. 258, 119656. doi:10.1016/j.conbuildmat.2020.119656
Khan, I. U., Gul, A., Khan, K., Akbar, S., and Irfanullah, (2022). Mechanical properties of steel-fiber-reinforced concrete. Eng. Proc. 245, 6. doi:10.3390/engproc2022022006
Köksal, F., Beycioğlu, A., and Dobiszewska, M. (2022). Optimization based on toughness and splitting tensile strength of steel-fiber-reinforced concrete incorporating silica fume using response surface method. Mater. (Basel) 15, 6218. doi:10.3390/ma15186218
Köksal, F., Şahin, Y., Gencel, O., and Yiǧit, I. (2013). Fracture energy-based optimisation of steel fibre reinforced concretes. Eng. Fract. Mech. 107, 29–37. doi:10.1016/j.engfracmech.2013.04.018
Kropidłowska, O. (2022). Application of sawdust concrete in construction. Bud. i Archit. 21, 005–018. doi:10.35784/bud-arch.2927
Kumar, R., Shafiq, N., Kumar, A., and Jhatial, A. A. (2021). Investigating embodied carbon, mechanical properties, and durability of high-performance concrete using ternary and quaternary blends of metakaolin, nano-silica, and fly ash. Environ. Sci. Pollut. Res. 28, 49074–49088. doi:10.1007/s11356-021-13918-2
Lee, M. G., Wang, W. C., Wang, Y. C., Hsieh, Y. C., and Lin, Y. C. (2022). Mechanical properties of high-strength pervious concrete with steel fiber or glass fiber. Buildings 12, 620. doi:10.3390/buildings12050620
Lehner, P., Konečný, P., and Ponikiewski, T. (2020). Comparison of material properties of scc concrete with steel fibres related to ingress of chlorides. Crystals 10, 220. doi:10.3390/cryst10030220
Liu, Y. W., Lin, Y. Y., and Cho, S. W. (2020). Abrasion behavior of steel-fiber-reinforced concrete in hydraulic structures. Appl. Sci. 10, 5562. doi:10.3390/app10165562
Maity, S. (2023). “2 - applications of selected response surface design of experiments and advanced control charts in textile engineering,” in The textile institute book series. Editors R. Chattopadhyay, S. K. Sinha, and M. L. B. T.-T. C. Regar (Woodhead Publishing), 13–55. 978-0-323-99041-7.
Mangi, S. A., Jamaluddin, N. B., Memon, S. A., and Bin Wan Ibrahim, M. H. (2019). Utilization of sawdust in concrete masonry blocks: a review. Mehran Univ. Res. J. Eng. Technol. 38, 487–494. doi:10.22581/muet1982.1902.23
Memon, R. P., Abdul, A. R., Abdul Awal, A. S. M., and Achekzai, L. (2017). Mechanical and thermal properties of sawdust concrete. J. Teknol. 79. doi:10.11113/jt.v79.9341
Memon, R. P., Achekzai, L., Abdul, A. R., Abdul Awal, A. S. M., and Memon, U. (2018). Performance of sawdust concrete at elevated temperature. J. Teknol. doi:10.11113/jt.v80.9826
Mujalli, M. A., Dirar, S., Mushtaha, E., Hussien, A., and Maksoud, A. (2022). Evaluation of the tensile characteristics and bond behaviour of steel fibre-reinforced concrete: an overview. Fibers 10, 104. doi:10.3390/fib10120104
Mwango, A., and Kambole, C. (2019). Engineering characteristics and potential increased utilisation of sawdust composites in construction—a review. J. Build. Constr. Plan. Res. 07, 59–88. doi:10.4236/jbcpr.2019.73005
Osei, D. Y., and Jackson, E. N. (2016). Compressive strength of concrete using sawdust as aggregate. Int. J. Sci. Eng. Res.
Pinheiro Simão Ribeiro, V., das Graças Machado Freire, M., Andreia Ferreira Hernández, G., Picanço Oliveira, M., and Ferreira de Oliveira, B. Proposal for a new monolithic constructive system using mycocomposite nucleus.; 2021.
Plotnikov, N., and Kochetkov, I. (2021). “Possibility of using sawdust in sawdust concrete,” in Proceedings of the E3S web of conferences.
Prabath, N. V. N., and Ramadoss, P. (2022). Evaluation of mechanical performance of high performance hybrid fiber reinforced concrete containing micro silica. Civ. Eng. Archit. 10, 210–223. doi:10.13189/cea.2022.100118
R.S. Effect (2016). Of length of polypropylene fiber reinforced sand on load settlement characteristic of model footings. Int. J. Res. Eng. Technol. doi:10.15623/ijret.2016.0507022
Ruiz, G., de la Rosa, Á., and Poveda, E. (2019). Relationship between residual flexural strength and compression strength in steel-fiber reinforced concrete within the new eurocode 2 regulatory framework. Theor. Appl. Fract. Mech. 103, 102310. doi:10.1016/j.tafmec.2019.102310
Sadowska-Buraczewska, B., Szafraniec, M., Barnat-Hunek, D., and Lagód, G. (2020). Flexural behavior of composite concrete slabs made with steel and polypropylene fibers reinforced concrete in the compression zone. Mater. (Basel) 13, 3616. doi:10.3390/MA13163616
Sharba, A. A. K., Hason, M. M., Hanoon, A. N., Qader, D. N., Amran, M., Abdulhameed, A. A., et al. (2022). Push-out test of waste sawdust-based steel-concrete – steel composite sections: experimental and environmental study. Case Stud. Constr. Mater. 17, e01570. doi:10.1016/j.cscm.2022.e01570
Shoughy, M. I. (2013). BIOMASS TECHNOLOGY PRODUCTION FROM AGRO-PROCESSING WASTES BY AN EXPELLER MACHINE. Misr J. Agric. Eng. 30, 537–558. doi:10.21608/mjae.2013.102075
Silva, W. V., Silva, R., Bezerra, L. M., Freitas, C. A. S., and Bonilla, J. (2020). Experimental analysis of space trusses using spacers of concrete with steel fiber and sisal fiber. Mater. (Basel) 13, 2305. doi:10.3390/ma13102305
Thilakarathna, P. S. M., Seo, S., Baduge, K. S. K., Lee, H., Mendis, P., and Foliente, G. (2020). Embodied carbon analysis and benchmarking emissions of high and ultra-high strength concrete using machine learning algorithms. J. Clean. Prod. 262, 121281. doi:10.1016/j.jclepro.2020.121281
Turner, L. K., and Collins, F. G. (2013). Carbon dioxide equivalent (CO2-e) emissions: a comparison between geopolymer and OPC cement concrete. Constr. Build. Mater. 43, 125–130. doi:10.1016/j.conbuildmat.2013.01.023
Ubachukwu, O. A., Ubi, S. E., Esochaghi, K. P., and Nwokoukwu, K. B. (2022). Properties of eco-friendly concrete produced by partial replacement of sand with sawdust with emphasis on water-cement ratio. Niger. J. Technol. 41. doi:10.4314/njt.v41i1.4
Veza, I., Spraggon, M., Fattah, I. M. R., and Idris, M. (2023). Response surface methodology (RSM) for optimizing engine performance and emissions fueled with biofuel: review of RSM for sustainability energy transition. Results Eng. 18, 101213. doi:10.1016/j.rineng.2023.101213
Wang, H., Zhao, X., Gao, H., Yuan, T., Liu, X., and Zhang, W. (2023). Effects of alkali-treated plant wastewater on the properties and microstructures of alkali-activated composites. Ceram. Int. 49, 8583–8597. doi:10.1016/j.ceramint.2022.11.028
Waqar, A., Bheel, N., Almujibah, H., Benjeddou, O., Alwetaishi, M., Ahmad, M., et al. (2023a). Effect of coir fibre ash (CFA) on the strengths, modulus of elasticity and embodied carbon of concrete using response surface methodology (RSM) and optimization. Results Eng. 17, 100883. doi:10.1016/j.rineng.2023.100883
Waqar, A., Bheel, N., Shafiq, N., Othman, I., Khan, M. B., Mansoor, M. S., et al. (2023b). Effect of volcanic pumice powder ash on the properties of cement concrete using response surface methodology. J. Build. Pathol. Rehabil. 8, 17. doi:10.1007/s41024-023-00265-7
Wu, C., Shi, Y., Xu, J., Luo, M., Lu, Y., and Zhu, D. (2024). Experimental study of mechanical properties and theoretical models for recycled fine and coarse aggregate concrete with steel fibers. Mater. Basel, Switz. 17, 2933. doi:10.3390/ma17122933
Yang, D., Zhang, B., and Liu, G. (2021). Experimental study on spall resistance of steel-fiber reinforced concrete slab subjected to explosion. Int. J. Concr. Struct. Mater. 15, 23. doi:10.1186/s40069-021-00459-8
Zaid, O., Ahmad, J., Siddique, M. S., and Aslam, F. (2021). Effect of incorporation of rice husk ash instead of cement on the performance of steel fibers reinforced concrete. Front. Mater. 8. doi:10.3389/fmats.2021.665625
Zarei, A., Rooholamini, H., and Ozbakkaloglu, T. (2022). Evaluating the properties of concrete pavements containing crumb rubber and recycled steel fibers using response surface methodology. Int. J. Pavement Res. Technol. 15, 470–484. doi:10.1007/s42947-021-00049-7
Zeybek, Ö., Özkılıç, Y. O., Çelik, A. İ., Deifalla, A. F., Ahmad, M., and Sabri Sabri, M. M. (2022). Performance evaluation of fiber-reinforced concrete produced with steel fibers extracted from waste tire. Front. Mater. 9. doi:10.3389/fmats.2022.1057128
Keywords: sustainable concrete, saw dust, steel fibers, response surface methodology, concrete material
Citation: Waqar A, Khan MB, Najeh T, Almujibah HR and Benjeddou O (2024) Performance-based engineering: formulating sustainable concrete with sawdust and steel fiber for superior mechanical properties. Front. Mater. 11:1428700. doi: 10.3389/fmats.2024.1428700
Received: 06 May 2024; Accepted: 26 August 2024;
Published: 11 September 2024.
Edited by:
Yu-Fei Wu, RMIT University, AustraliaReviewed by:
Ionut Ovidiu Toma, Gheorghe Asachi Technical University of Iași, RomaniaCopyright © 2024 Waqar, Khan, Najeh, Almujibah and Benjeddou. This is an open-access article distributed under the terms of the Creative Commons Attribution License (CC BY). The use, distribution or reproduction in other forums is permitted, provided the original author(s) and the copyright owner(s) are credited and that the original publication in this journal is cited, in accordance with accepted academic practice. No use, distribution or reproduction is permitted which does not comply with these terms.
*Correspondence: Muhammad Basit Khan, bXVoYW1tYWRfMjEwMDIwMTRAdXRwLmVkdS5teQ==; Taoufik Najeh, dGFvdWZpay5uYWplaEBsdHUuc2U=
Disclaimer: All claims expressed in this article are solely those of the authors and do not necessarily represent those of their affiliated organizations, or those of the publisher, the editors and the reviewers. Any product that may be evaluated in this article or claim that may be made by its manufacturer is not guaranteed or endorsed by the publisher.
Research integrity at Frontiers
Learn more about the work of our research integrity team to safeguard the quality of each article we publish.