- EHV Power Transmission Company of China Southern Power Grid Co., Ltd, Dali, China
Neat high-temperature vulcanized silicone rubber (HTV SR) showed bad hydrophobicity due to the existence of a large number of inorganic reinforcing fillers on its surface, which affected its security application in outdoor insulation systems. In this work, hydrophobic silane chains were grafted onto the surface of inorganic particles on the skin layer of HTV SR by a one-step coupling modification to improve its hydrophobicity. The effects of coupling agent types and coupling reaction conditions on the hydrophobicity of HTV SR were investigated. It was determined that the perfluorooctane trichlorosilicon with a large number of low surface energy fluorine atoms was the preferred coupling agent. The contact angle of HTV SR was increased by 15.70% when the optimal coupling reaction conditions were determined to be a reaction temperature of 60°C, a reaction time of 4 h, and an amount of coupling agent of 0.5%. The study found that both etching reaction and grafting reaction occurred during the whole coupling modification process, which led to the disappearance or decrease of a lot of holes on the HTV SR surface. After the fluorination coupling reaction, the surface of HTV SR became smooth and dense that led to the decrease of water absorption. The result of Fourier-transform infrared spectroscopy analysis showed the formation of C-F bond in HTV SR after fluorination modification, and the energetic dispersive spectroscopy analysis showed that the fluorine content on the surface of the fluorinated HTV SR increased significantly. Moreover, the stability analysis showed that the fluorinated HTV SR still had a good thermal stability and mechanical property stability.
1 Introduction
High-temperature vulcanized silicone rubber (HTV SR) is a kind of polymer material with Si-O bonds as the main chain with dimethyl or methyl vinyl structure as the side chain normally. It is usually prepared by a vulcanization reaction at a high temperature and high pressure by crosslinking methyl vinyl silicone rubber, methyl phenyl vinyl silicone rubber, etc. as the raw rubber with the addition of a large number of inorganic fillers, structure-controlling agents, vulcanizing agents, and other auxiliaries (Zhang et al., 2018; Kokalis et al., 2021; Pal et al., 2021). With the advantages of high- and low-temperature resistance, corrosion resistance, dirt flash resistance, and excellent electrical insulation, it has a wide range of applications in the fields of automotive electronics, aerospace, insulated power transmission, etc. In particular, composite insulators prepared by HTV SR are widely used in high-voltage circuits of the national power grid system (hang et al., 2023). Compared with ceramic insulators and glass insulators, the light weight of HTV SR insulators can significantly reduce the pressure on other components such as pylons and towers in high-voltage lines, ensuring their safety and operation (Shen et al., 2019).
However, the large number of inorganic fillers added during the preparation process of HTV SR is partially exposed on the surface. In addition, the surface of HTV SR shows a large number of tiny microstructure pores usually. Its hydrophilic properties and the accommodation-adsorption effect on water vapor makes the hydrophobicity of HTV SR insufficient. The contact angle of HTV SR is usually only in the range of 90–110o (Pan et al., 2022). When there is bad weather such as heavy rain and long-term humidity outdoors, the surface of the HTV SR insulator is prone to absorb water and even form a local water film, resulting in temporary loss of hydrophobicity, decrease of insulation strength, and even serious accidents such as flashover breakdown or explosion (Ullah et al., 2023). Therefore, it is of great significance to further improve the hydrophobicity of HTV SR materials.
At present, there are few studies on the hydrophobicity improvement of HTV silicone rubber. It is mainly achieved by increasing surface roughness or fluorination to reduce surface energy as reported in the literature (Gao et al., 2009; Peng et al., 2018; Peng et al., 2019). Hu et al. (2022) reversibly swelled the HTV SR, then used the sol–gel method to grow flower-like ZnO nanoparticles in situ on the HTV SR surface, and finally obtained a hydrophobic surface by increasing the roughness. Hu et al. (He et al., 2023) also prepared hydrophobic HTV SR materials by growing in situ polyacrylamide-coated titanium dioxide on its surface by sol–gel method. He et al. (Hu et al., 2021) physically polished the surface of HTV SR first and covered it with a layer of polydimethylsiloxane and then used the surface inspergation to introduce silica powder to obtain a hydrophobic surface. Chen et al. (2022) etched the surface of HTV SR by femtosecond laser technology to form a small mastoid morphology on the surface to construct a micro-nanocomposite layer structure, which increased the surface roughness and effectively improved the hydrophobicity of HTV SR. Gao and Zhou (2013) used the coupled plasma technology to treat the surface of SR, and the hydrophobic modification was carried out on the surface of HTV SR by etching and fluorination of low-temperature plasma. The existing preparation methods have a good effect on improving the hydrophobicity of HTV SR. However, the method of increasing the roughness by growing nanoparticles in situ on the surface of HTV SR has the problem of weak bonding of inorganic particles to the HTV SR matrix, and the introduction of inorganic particles by inspergation is not evenly distributed. The methods of swelling, polishing, or etching on the surface will cause certain damage to the surface of HTV SR. For example, Wang et al. (2016) carried out direct fluorination etching of HTV SR by introducing F2 gas into the reactor, only to find that the hydrophobicity increased significantly but the thickness of the HTV SR decreased by nearly 46%. A large number of destructive gullies appeared on the surface that significantly reduced the original mechanical properties of HTV SR. In addition, the existing process to enhance the hydrophobicity of HTV SR is technically complex, expensive, and inconvenient to operate, which has non-negligible limitations for its large-scale applications.
Functional modification of inorganic surfaces by silane coupling is widely applied to improve its hydrophobicity in the field of surface chemistry. However, generally, the modified object by this method is nanoparticles or solid powders. This method directly employed to improve the hydrophobicity of the HTV SR polymer product has not been reported. In view of the fact that plenty of hydroxyl groups exist on the surface of HTV SR due to the self-characteristics of silicone rubbers and also the large number of exposed inorganic particles, the hydrophobic silane coupling agents can be used to modify the surface of HTV SR to improve its hydrophobicity by coupling grafting reaction. There are many kinds of silanes used to modify the inorganic particles. Amino silane is the most common one due to its excellent improvement of interfacial compatibility between the organic and inorganic phases. In contrast, fluoro silane contains plenty of fluorine atoms with low surface energy and can also be considered as a good alternate option for the improvement of hydrophobicity of inorganic particles. The introduction of pernuoro organic long chains with low surface energy characteristics to coat the inorganic particles on the surface of silicone rubber can effectively improve the hydrophobicity of HTV SR. In this work, the effects of coupling agent type, coupling reaction conditions, and other factors on the contact angle and water absorption of HTV SR were investigated. The surface microstructure and composition of fluorinated coupling-modified HTV SR were studied using Fourier-transform infrared spectroscopy (FTIR) and SEM analysis; the effects of fluorination degree on the mechanical properties and thermal stability of HTV SR were analyzed; and the mechanism was explored.
2 Materials and methods
2.1 Materials
HTV SR was provided by China Southern Power Grid Co., Ltd. 3-Aminopropyl triethoxysilane (analytical reagent), perfluorooctane trichlorosilicon (analytical reagent), and sodium hydroxide (analytical reagent) were purchased from Shanghai Aladdin Biochemical Technology Co. Ltd., and the deionized water was self-manufactured.
2.2 Preparation process
Pure HTV silicone rubber was cut into 2 × 10 × 0.5-cm sized silicone rubber sheets, ultrasonically cleaned for 10 min to remove dust and stains on the surface, and then was placed in 100 mL of ethanol–water mixture (8:2, v/v). The mass concentrations of the coupling agents with different contents and types were 0.1, 0.2, 0.3, 0.5, 0.7, and 1.0 wt%. NaOH aqueous solution (1 mol/L) was added dropwise to adjust its pH value to 8.0 and then the temperature was raised to 30, 40, 50, 60, 70, and 80°C, with continuous stirring for 2, 4, 6, 8, and 10 h, respectively. After the reaction, the HTV SR sheet was picked up, washed, and dried to obtain hydrophobic coupling-modified HTV SR.
2.3 Testing and characterization
Contact angle analysis: The hydrophobicity of the prepared hydrophobic modified HTV SR was tested by OCA20 optical video measuring instrument (German Dataphysics). The contact angle was averaged by taking five µL of ultrapure water and dropping it onto the surface of the silicone rubber material at five different locations.
Water absorption rate: The hydrophobic coupling-modified HTV SR was fully dried and its mass was weighed. Then it was soaked in deionized water for 24 h at room temperature, and the water on the surface was wiped with filter paper; the mass change was tested to calculate the water absorption.
SEM analysis: The prepared hydrophobic modified HTV SR material was ion sputtered and sprayed with gold for 1 min and its microstructure before and after coupling modification was observed by TM3030 scanning electron microscope (Hitachi, Japan) at ×1,000 magnification. The test used thermionic electron source, backscattered electron detector, and 15 kV accelerating voltage.
Energetic dispersive spectroscopy (EDS) analysis: The Sigma 300 (German ZEISS) scanning electron microscope was used to analyze the distribution and relative content of the four elements C, O, F, and Si on the surface of the silicone rubber material before and after coupling modification with mapping mode. The test used SE2 secondary electronic detector, Smartedx EDS, and Schottky field emission gun.
FTIR analysis: The Nicolet iS20 (Thermo Scientific, United States) Fourier-transform infrared spectrometer was used to analyze the structure of HTV SR before and after hydrophobic coupling modification. The test was conducted in ATR absorbance mode with a scanning range of 400–4,000 cm−1, a scanning speed of 20 min−1, and a resolution ratio of 4 cm−1.
Mechanical properties: The mechanical properties of the prepared hydrophobic modified HTV SR were tested by AGS-X-50N Electronic Universal Testing Machine (Shimadzu, Japan) with a tensile rate of 200 mm/min.
Thermogravimetric analysis: Approximately 10 mg material was cut from the whole HTV SR sample by a blade, and its thermal stability was tested by Discovery TGA 550 thermogravimetric analyzer (TA, United States) in a nitrogen atmosphere, with a temperature range of room temperature to 700°C and a temperature increase rate of 10oC/min.
3 Results and discussion
3.1 Coupling modification mechanism of hydrophobic HTV SR
Pure HTV SR system contains a large number of inorganic particle fillers, most of which are SiO2 and Al(OH)3. Some inorganic particles are exposed on the surface of the silicone rubber sheet and make the poor hydrophobicity of the silicone rubber surface (Zhang et al., 2017; Vazirinasab et al., 2019). There are plenty of hydroxyl groups on the surface of inorganic particles and SRs. It can be modified by coupling reaction to improve its hydrophobicity by introducing a large number of hydrophobic alkyl chains. As shown in Figure 1, the modification mechanism of the two coupling agents, perfluorooctane trichlorosilicon and 3-aminopropyl triethoxysilane, is shown respectively. The chlorine group of perfluorooctane trichlorosilicon and the ethoxy group of 3-aminopropyl triethoxysilane are easily hydrolyzed in alkaline solution to form silicon hydroxyl, which can react with a large number of hydroxyl groups on the surface of HTV SR. The two can form Si-O-Si chemical bonds through condensation reaction, thus coating hydrophobic organic alkyl chains on the surface of HTV SR. As seen in Figure 1, after modified by perfluorooctane trichlorosilicon, many F atoms are introduced onto the surface of HTV SR. So, this modified material is marked as F-HTV SR. Contrastingly, many H atoms cover the surface of HTV SR after modified by 3-aminopropyl triethoxysilane. So, this modified material is marked as H-HTV SR.
3.2 Contact angle analysis of hydrophobic modified HTV SR
3.2.1 Effect of coupling time on contact angle
The hydrophobicity of the silicone rubber surface grafted with different coupling agents was characterized by contact angle analysis, as shown in Figure 2, which is the effect of coupling modification time on the contact angle. It can be seen from the figure that the neat HTV SR (0 h point) showed relative low contact angle. With the extension of the modification time, the water contact angle of HTV SR material increases gradually and reaches the maximum at 4 h and then decreases. This may be due to the low grafting rate of organic alkyl chains when the coupling reaction time is short, and the hydrophobicity improvement of silicone rubber is not obvious. When the coupling modification time is too long, part of the SiO2 particles on the surface of the silicone rubber material dissolve in the NaOH solution to form sodium silicate, so that the grafted organic hydrophobic alkyl chain decrease, and the contact angle is reduced. It can be seen from the figure that the optimal coupling modification time of the two coupling agents is 4 h. Among them, the contact angle of silicone rubber grafted with perfluorooctane trichlorosilicon was increased by about 15.70%. In contrast, the contact angle of silicone rubber grafted with 3-aminopropyl triethoxysilane only was increased by 5.45%, and at any same coupling reaction time, its improvement on contact angle is significantly lower than that of perfluorooctane trichlorosilicon.
3.2.2 Effect of coupling temperature on contact angle
The effect of coupling modification reaction temperature on the contact angle of the silicone rubber surface was further investigated. As shown in Figure 3, with the elevation of the reaction temperature, the grafting reaction of the silane coupling agent was speeded, the grafting rate of the hydrophobic silane long chain on the surface of the silicone rubber was increased, and its hydrophobicity increased significantly which reached the maximum at 60°C. However, with the further elevation of the reaction temperature, the contact angle of the silicone rubber gradually decreased instead. This was possible because of the existence of competition between the coupling grafting reaction on the surface of silicone rubber and the etching reaction of alkali solution on silicone rubber during the process. When the reaction temperature was low, the reaction system was dominated by the coupling grafting reaction, which showed positive hydrophobic effect. However, at high temperatures, the etching reaction of the alkali solution was significant and the coupling reaction between coupling agents was enhanced, resulting in a reduction of grafting reaction on the surface of the silicone rubber, which showed a negative effect of hydrophobicity. It can be seen from the figure, the optimal coupling modification reaction was at 60°C. In addition, at any same coupling reaction temperature, the enhancement effect of perfluorooctane trichlorosilicon on the contact angle of silicone rubber was significantly better than that of 3-aminopropyl triethoxysilane.
3.2.3 Effect of coupling agent dosage on contact angle
The concentration of the coupling agent in the reaction system also affects its grafting rate, thus affecting the hydrophobicity of the surface of silicone rubber. The contact angle of the surface of silicone rubber at different coupling agent dosages was shown in Figure 4. Compared with pure HTV SR, as the amount of coupling agent increases, the contact angle of the SR surface showed a trend of decreasing first, then increasing and reaching a steady level. This may be due to the fact that when the coupling agent content was low, there was little grafting modification reaction of the coupling agent in the reaction system, and the etching reaction of the alkali solution on the silicone rubber played a dominant role, resulting in the decrease of the contact angle of the silicone rubber and the deterioration of its hydrophobicity. With the gradual increase in the amount of coupling agent, the graft modification reaction of the coupling agent in the reaction system increased and gradually played a leading role and the hydrophobicity enhanced significantly. With the continual increase in the amount of coupling agent, limited by the grafting active sites on the surface of the silicone rubber, the grafting of silane chains reached saturation. Then the contact angle was no longer increased and the hydrophobicity of HTV SR reached stable. It can be seen from the figure that the hydrophobicity of the system grafted with perfluorooctane trichlorosilicon and that grafted with 3-aminopropyl triethoxysilane reached the maximum when its dosage was 0.5% and 0.7%, respectively. Similarly, at the same amount of coupling agent, the contact angle of silicone rubber grafted with perfluorooctane trichlorosilicon was higher than that of the system grafted with 3-aminopropyl triethoxysilane. The possible reason was analyzed to be the difference of their composition and structure. In contrast, the molecular chain length of 3-aminopropyl triethoxysilane was shorter and its coating function on inorganic particles was limited. However, the molecular chain length of perfluorooctane trichlorosilicon is longer and the H atoms on its methylene group are all replaced by the most electronegative F atoms. The bonding energy of the C-F bonds is significantly higher than that of the C-H bonds. The shielding effect of the F atom electron cloud to the C-C bonds is stronger than that of the H atom. The electrons in the C-F bond are tightly bound around the nucleus, thus the surface energy of the silicone rubber is significantly reduced (Zhou et al., 2020; Yang et al., 2021) and the hydrophobicity is greatly improved after the grafting of perfluorooctane trichlorosilicon. Therefore, the HTV SR grafting modified with perfluorooctane trichlorosilicon is used as the research object in the subsequent study.
3.3 Water absorption of hydrophobic modified HTV SR
The effect of different coupling modification conditions on the water absorption of HTV SR is shown in Figure 5. The abscissa axis with different colors indicates the different modification times, modification temperatures, or modifier dosages. The hydrophilicity/hydrophobicity of the surface of HTV SR significantly changed due to the coupling grafted modification, which also has a significant effect on its water absorption. From the diagram, it can be seen that compared with pure HTV SR, the water absorption rate of HTV SR after coupling modification showed a certain degree of reduction. In addition, the effect of coupling agent type showed that the water absorption of HTV SR grafted with perfluorooctane trichlorosilicon was lower than that grafted with 3-aminopropyl triethoxysilane under the same conditions, which further indicated that perfluorooctane trichlorosilicon had a better effect on the improvement of the hydrophobicity of the HTV SR surface. The water absorption of the fluorinated HTV SR reached the lowest at the fluorinated coupling time of 4 h, the reaction temperature of 60°C, and the coupling agent dosage of 0.5%. This result was basically consistent with the results of contact angle analysis. The possible reason was analyzed and deduced to that the surface of HTV SR was covered with a layer of organic hydrophobic chain after the fluorinated grafting, which showed a certain repulsion function to water and thus led to a decrease in water absorption. In addition, after fluorinated coupling modification, the large number of micropores on the surface of HTV SR disappeared and thereby losing the ability to accommodate water, leading to the decrease in water absorption.
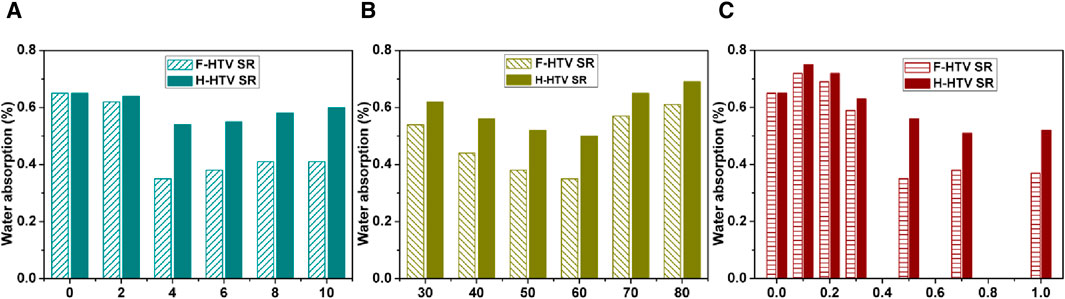
Figure 5. Effect of the coupling modification condition on water absorption of HTV SR: (A) Time, (B) Temperature, (C) Content.
3.4 Microstructure analysis of hydrophobic modified HTV SR
The effect of coupling modification on the microstructure of HTV SR was observed by scanning electron microscopy. Figure 6 is the surface morphology of the silicone rubber under different coupling modification times. As can be seen from Figure 6A of neat HTV SR without any treatment, there is a large number of pore morphology on the surface of pure HTV SR. At the same time, a large number of exposed inorganic SiO2 fillers are clearly visible. After modification with coupling agent, its micromorphology changed obviously. When the coupling modification time was short (Figure 6B), the degree of grafting and etching reactions was low and the morphology of HTV SR hardly changed. The comparative example with NaOH only showed a similar microstructure with that of silane/NaOH mixed system. With the extension of the coupling modification time (Figure 6C), its morphology changed significantly and the large number of holes on the surface gradually disappeared. When the reaction time came to 6 h, the obvious holes were basically difficult to be observed and the surface structure became dense (Figure 6D). This might be due to that the etching reaction gradually played a leading role with the extension of coupling reaction time and thus the silicone rubber around the holes was etched. At the same time, the pores would be filled with the byproducts generated during the etching reaction process. Thus, the surface of HTV SR became smooth and dense after modification.
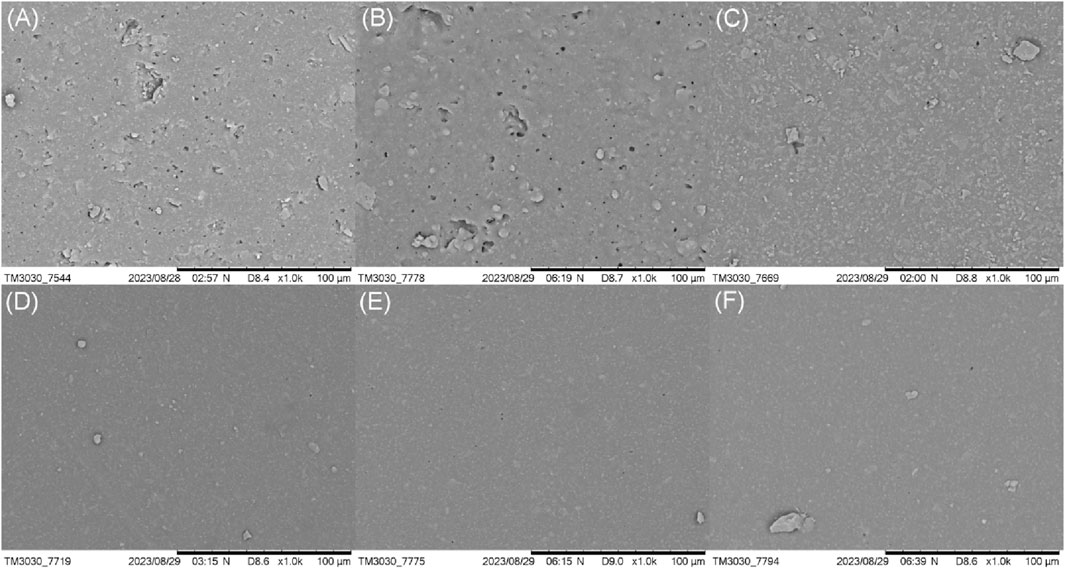
Figure 6. Effect of coupling reaction time on the microstructure of the HTV SR surface: (A) 0 h, (B) 2 h, (C) 4 h, (D) 6 h, (E) 8 h, and (F) 10 h).
The effect of different coupling reaction temperatures on the surface morphology of HTV SR was further observed. As shown in Figure 7, the microstructures of the silicone rubber modified at different coupling temperatures were similar. The surfaces were relatively smooth and dense and the holes were rare or even difficult to be observed. This phenomenon was consistent with the effect of the reaction time. With the increase of reaction temperature, the surface morphology of the silicone rubber did not changed evidently and no obvious difference could be observed.
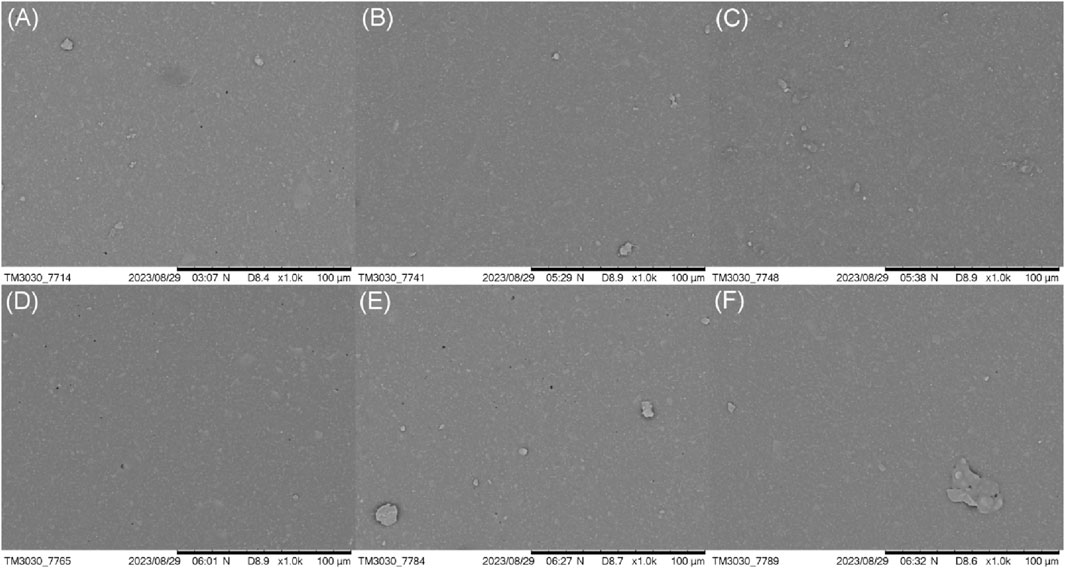
Figure 7. Effect of the coupling reaction temperature on the microstructure of the HTV SR surface: (A) 30°C, (B) 40°C, (C) 50°C, (D) 60°C, (E) 70°C, and (F) 80°C.
The micromorphology of the HTV SR surface under different coupling agent dosages was further observed. As shown in Figure 8, the pure HTV SR (Figure 8A) showed a porous and rough structure. When the amount of coupling agent was low, the amount of micropores on the surface of grafted SR was decreased (Figure 8B). With the further increase of the amount of coupling agent in the reaction system, the holes on the microsurface of the silicone rubber disappeared gradually (Figure 8C). When the amount of coupling agent was high, the holes on the surface of silicone rubber were even difficult to be observed (Figure 8D). The grafting and coating of a large number of hydrophobic silane long chains on the surface was also benefited to the smooth surface of the silicone rubber.
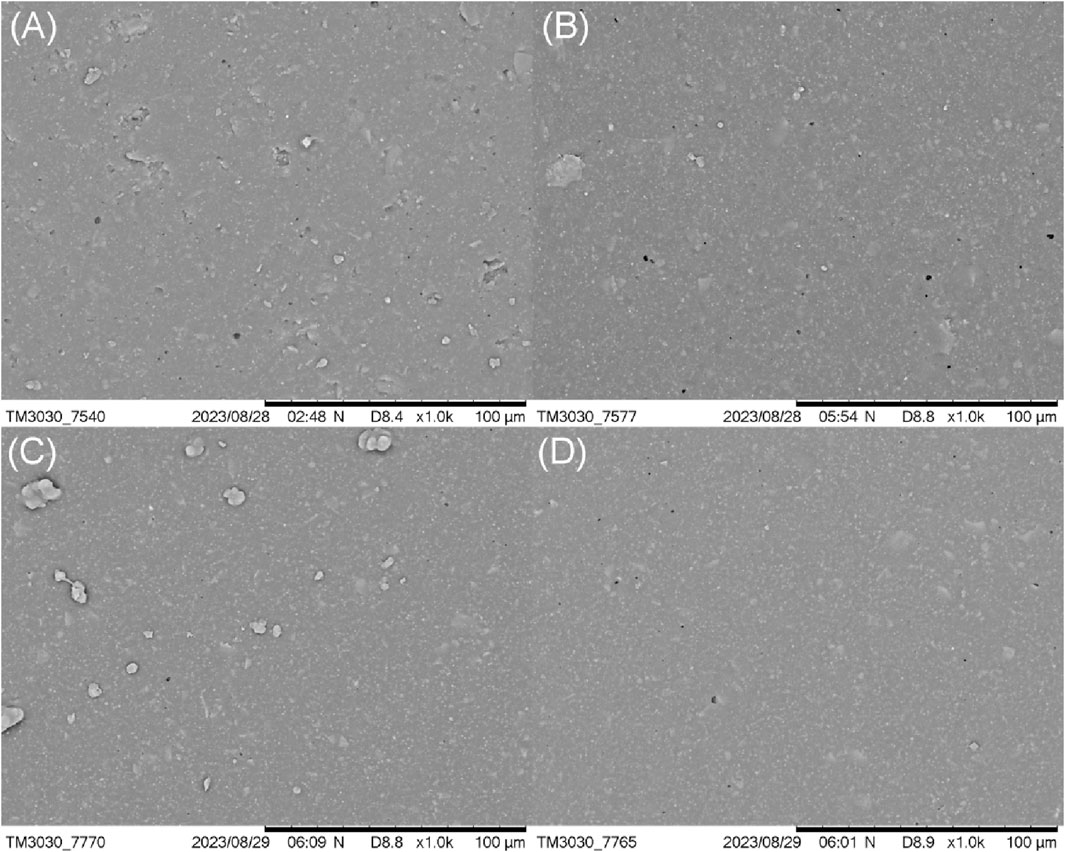
Figure 8. Effect of the coupling agent content on the microstructure of the HTV SR surface: (A) 0.0%, (B) 0.1%, (C) 0.3%, and (D) 0.5%.
SEM-EDS technique was used to analyze the element distribution of HTV SR surface before and after fluorination modification. The surface element distribution of pure HTV SR was shown in Figure 9A. It can be seen from the figure that the main elements on the surface of pure HTV SR were C, O, and Si element, which accounted for the vast majority. The detection of the small amount of F element may be due to the introduction during the test process or the addition of F-containing additives in the preparation process of pure HTV SR. It can be seen that the distribution of F element is uniform. In fact, it is not surprising that the functional modification is taken place on the whole rubber surface because of the relatively uniform distribution of inorganic fillers in HTV SR materials. In contrast, the F content of fluorinated HTV SR significantly increased. As shown in Figure 9B, where the C, O, and Si were still the main elements presented, but the relative content of F element increased from 0.52% to 2.75%, which was mainly due to the grafting of perfluorooctane trichlorosilicon coupling agent on the surface of HTV SR. Perhaps, the true content of fluorine atom may be not very accurate due to the defect of test method, but the variation tendency of fluorine in the surface of HTV SR is reliable. In addition, after fluorination modification, the relative content of C on the surface of HTV SR increased from 25.92% to 30.38%, while the relative content of O and Si decreased from 43.33% to 30.23%–39.70% and 27.17%, respectively. The big change in the C content perhaps resulted due to the cracking of hydrocarbons and subsequent deposition of carbon on the surface of the investigated samples.
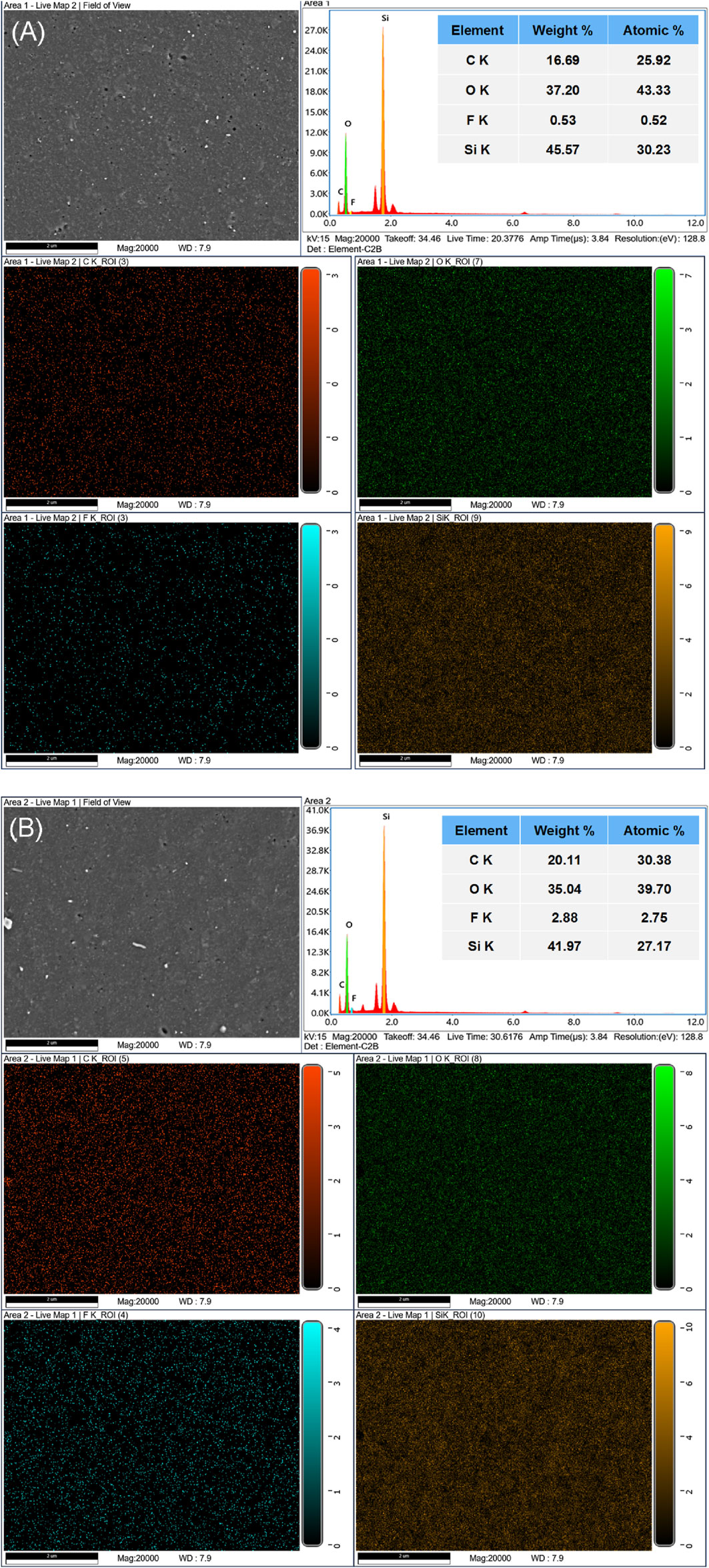
Figure 9. Element distribution of EDS analysis on the HTV SR surface before and after fluorinated coupling modification. (A) Neat HTV SR and (B) F-HTV SR.
3.5 Structural analysis of hydrophobic modified HTV SR
According to the analysis of the contact angle results mentioned before, it can be seen that the hydrophobicity of the surface of the silicone rubber was most enhanced when perfluorooctane trichlorosilicon was used as the coupling agent under the conditions of 4 h of coupling reaction time, 60°C of temperature, and 0.5% of coupling agent. Therefore, FTIR was used to analyze the surface structure of HTV SR before and after coupling modification under optimal reaction condition. As shown in Figure 10, for pure HTV SR and F-HTV SR grafted with perfluorooctane trichlorosilicon, the characteristic absorption peaks for -OH, -CH2-, Si-CH3, and Si-O-Si groups appeared at 3,500, 2,965, 1,263, 1,023, and 799 cm−1 in the FTIR spectra, respectively, which indicated that the coupling and grafting modification did not affect the main molecular chain structure of the silicone rubber. In addition to these main absorption peaks, the IR spectra of F-HTV SR showed several new absorption peaks at 2,917.38, 2,849.39, 1,556.49, 1,470.91, 1,380.39, 1,339.41, and 1,320.98 cm−1 with different intensities. The absorption peaks at 2,917.38 and 2,849.39 cm−1 corresponded to the absorption peak of -CH on the molecular chain of perfluorooctane trichlorosilicon coupling agent. The peaks at 1,556.49, 1,470.91, 1,380.39, 1,339.41, and 1,320.98 cm−1 were attributed to the vibration absorption peaks of C-F on the molecular chain of perfluorooctane trichlorosilicon coupling agent (Yu et al., 2020). The FTIR results showed that the hydrophobic perfluorooctane trichlorosilicon molecular chain was successfully grafted onto the surface of HTV SR by coupling modification reaction.
3.6 Mechanical properties of hydrophobic modified HTV SR
The mechanical properties of hydrophobic modified HTV SR are important parameters for its application feasibility. The stress–strain curves of HTV SR with different fluorination degrees were shown in Figure 11. It can be seen from the figure that the breaking strength of pure HTV SR was approximately 3.0 MPa and the elongation at break was about 210%. Ignoring the test error of the experiment, the breaking strength and elongation at break of the fluorinated silicone rubber did not change significantly. These results showed that the mechanical properties of HTV SR were hardly affected by fluorinated hydrophobic modification and was irrelevant with respect to the degree of fluorination. This may be due to the fact that although the etching reaction and grafting reaction occurred during the modification process, the degree of etching reaction was low and was just limited to the surface area. Thus, the overall mechanical properties of HTV SR were not significantly affected. The grafting reaction did not involve the silicone rubber molecular chain and its influence can be ignored. Therefore, it can be concluded that the fluorinated HTV SR showed a good mechanical stability.
3.7 Thermal stability of hydrophobic modified HTV SR
The thermal stability of hydrophobic modified HTV SR is another important parameter for judging its application feasibility. The thermogravimetric analysis curves for low-fluorinated silicone rubber (LF-HTV SR), high-fluorinated silicone rubber (HF-HTV SR), and pure HTV SR are shown in Figure 12. It can be seen from Figure 12A that compared with pure HTV SR, the mass residual rate of fluorinated SR increased to varying degrees. This may be due to the slight etching of the surface layer of HTV SR under alkaline conditions during the fluorination grafting process that led to the slight increase of inorganic component related content. The mass residual rate of the HF-HTV SR was lower than that of LF-HTV SR. This may be due to the grafting of a certain amount of perfluorooctane trichlorosilicon coupling agent molecular chain which will increase the relative content of the organic component on the surface. In addition, it can be seen from Figure 12A that the thermal decomposition process of HTV SR can be divided into two stages. The first stage from 150°C to 360°C was the decomposition of side chains such as -CH3, -CH = CH2 on the silicone rubber molecular chain. The second stage from 360°C to 600°C was the decomposition of Si-O bonds on the main molecular chain of SR. The derivative TG (DTG) curve of Figure 12B showed that the two pyrolysis stages were very evident. According to the DTG curve, the thermal performance parameters such as the initial decomposition temperature (Tinitial) and maximum degradation rate temperature (Tmax) of modified HTV SR with different fluorination degrees in each pyrolysis stage can be obtained. The results of Figure 12B show that the degree of fluorination had slight effect on the thermal stability of HTV SR and the Tinitial and Tmax of each pyrolysis stage were almost the same. However, compared with pure HTV SR, the Tinitial and Tmax of HTV SR after fluorination increased to a certain extent in each pyrolysis stage, indicating that its thermal stability improved. This may be due to the etching effect in the process of fluorination coupling grafting, which led to the increase of inorganic phase content in the surface layer, and limited the movement of HTV molecular chain and improved the thermal stability.
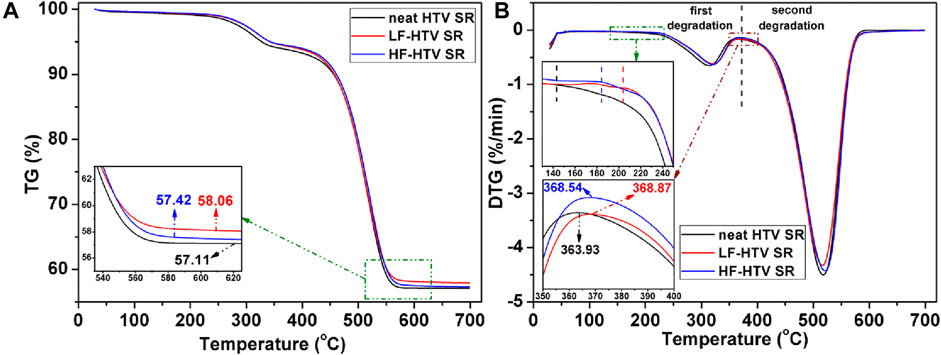
Figure 12. TG analysis of HTV SR with different fluorination degrees. (A) TG curve and (B) DTG curve].
4 Conclusion
There were a large number of inorganic particles and micropores exposed on the surface of pure HTV SR materials generated during the vulcanization preparation process, leading to its poor hydrophobicity. To improve the hydrophobicity of HTV SR, the coupling grafting reaction between silane coupling agent and inorganic particles was carried out to modify and graft hydrophobic organic chains on its surface, thus improving the hydrophobicity of HTV SR by one-step coupling modification. The optimum coupling reaction conditions were determined by investigating the effects of coupling agent type, coupling reaction temperature and time, and coupling agent dosage on the contact angle and water absorption of HTV SR. The effects of coupling modification on the surface morphology and surface element distribution of HTV SR were studied. The structural composition was analyzed by FTIR. The effects of coupling modification on mechanical properties and thermal stability of HTV SR were studied. The results showed that pure HTV SR had poor hydrophobicity. After coupling and grafting modification, its contact angle increased significantly. When the optimum coupling reaction conditions were 60°C of reaction temperature, 4 h of reaction time, and 0.5% of coupling agent dosage, the contact angle of modified HTV SR reached the maximum. Due to the large number of fluorine atoms with low surface energy for perfluorinated coupling agent, the hydrophobicity of HTV SR after fluorination coupling modification was more significant when compared to that after fluorination with the aminopropyl coupling agent. During the process of hydrophobic modification, there were both etching reaction and coupling grafting reaction on the surface of HTV SR. Because of the combined action of etching reaction and coupling grafting reaction, the large number of pores disappeared and the surface of HTV SR became smooth and dense after the modification, which improved the hydrophobicity and reduced water absorption significantly. The C-F absorption peaks appeared on the FTIR spectra for fluorinated HTV SR. Elemental analysis showed that the fluorine content on the surface of HTV SR increased significantly after fluorination coupling modification. The degree of fluorination had little effect on the stability of fluorinated HTV SR with slightly improved thermal stability and basically unchanged mechanical properties.
Data availability statement
The original contributions presented in the study are included in the article/Supplementary Material; further inquiries can be directed to the corresponding author.
Author contributions
QL: conceptualization, methodology, writing–original draft, and writing–review and editing. ML: data curation, software, and writing–review and editing. ZL: data curation, software, and writing–review and editing. QG: investigation, validation, and writing–review and editing. YS: validation and writing–review and editing. XW: validation and writing–review and editing.
Funding
The author(s) declare that financial support was received for the research, authorship, and/or publication of this article. This work was supported by Key Technology Project of China Southern Power Grid (CGYKJXM20220103), which are all gratefully acknowledged.
Conflict of interest
Authors QL, ML, ZL, QG, YS, and XW were employed by EHV Power Transmission Company of China Southern Power Grid Co., Ltd.
Publisher’s note
All claims expressed in this article are solely those of the authors and do not necessarily represent those of their affiliated organizations, or those of the publisher, the editors, and the reviewers. Any product that may be evaluated in this article, or claim that may be made by its manufacturer, is not guaranteed or endorsed by the publisher.
References
Chen, L., Nie, Q., Guo, F., Hu, T., Wang, Y., and Liu, D. (2022). Vitisin A, a resveratrol tetramer, improves scopolamine-induced impaired learning and memory functions in amnesiac ICR mice. China Laser 10 (10), 273–282. doi:10.3390/biomedicines10020273
Gao, S., Wen, L., Zhou, K., Liu, Y., and Lei, M. (2009). A study on the double hydrophobic modification of silicone rubber surface by CF4 RF capacitive coupled plasma. Funct. Mater. (01), 79–81. doi:10.3321/j.issn:1001-9731.2009.01.022
Gao, S., and Zhou, K. (2013). Hydrophobic modification of silicone rubber insulator surface by CF4 radio frequency plasma. Polym. Mater. Sci. Eng. (08), 101–104+109. doi:10.16865/j.cnki.1000-755.2013.08.025
hang, W., Qian, L., and Wei, T. (2023). High frequency dielectric properties of PP g-PVS compatibilizer PP/silicone rubber (MQ). Plast. Ind. (01), 67–73. doi:10.3969/j.issn.1005-5770.2023.01.011
He, Q., Wang, X., and Ning, M. (2023). Preparation of silicone rubber superhydrophobic coatings by powder spreading method. China Surf. Eng. (02), 146–154+179. doi:10.11933/j.issn.1007-9289.20220409001
Hu, Y., Shi, X., Ma, X., Bi, H., Li, M., and Sun, J. (2022). In situ growth of ZnO nanoflowers on the surface of silicone rubber to construct a stable superhydrophobic surface. J. Compos. Mater. (04), 1638–1647. doi:10.13801/j.cnki.fhclxb.20210611.01
Hu, Y., Yu, L., Ma, X., Bi, H., Sun, J., Jiang, Q., et al. (2021). Analysis of risk factors of PICC-related bloodstream infection in newborns: implications for nursing care. J. Qingdao Univ. Sci. Technol. Nat. Sci. Ed. 26 (03), 80–84. doi:10.1186/s40001-021-00546-2
Kokalis, C. C. A., Kontargyri, V. T., and Gonos, I. F. (2021). A proposal for the evaluation of HTV silicone rubber composite insulators. Polymers 13 (21), 3610. doi:10.3390/polym13213610
Pal, A. K., Baral, A., and Lahiri, A. (2021). Studies on prospect of HTV silicone rubber as dielectric material when reinforced with TiO2 nano particles. IET. Sci. Meas. Technol. 15 (3), 292–301. doi:10.1049/smt2.12030
Pan, W., Wan, X., Nan, J., Cheng, B., Dai, S., and Wu, Z. (2022). Research on surface aging, modification, and repair of silicone rubber. Surf. Technol. (02), 108–115. doi:10.16490/j.cnki.issn.1001-3660.2022.02.010
Peng, W., Gou, X., Qin, H., Zhao, M., Zhao, X., and Guo, Z. (2018). Creation of a multifunctional superhydrophobic coating for composite insulators. Chem. Eng. J. 352, 774–781. doi:10.1016/j.cej.2018.07.095
Peng, W., Gou, X., Qin, H., Zhao, M., Zhao, X., and Guo, Z. (2019). Robust Mg (OH)2/epoxy resin superhydrophobic coating applied to composite insulators. Appl. Surf. Sci. 466, 126–132. doi:10.1016/j.apsusc.2018.10.039
Shen, W., Gao, H., Zhang, P., Zuo, K., Tian, Y., Yang, W., et al. (2019). Research on UV aging performance of polymer composite insulators. China Electr. Power (09), 73–78+85. doi:10.11930/j.issn.1004-9649.201809100
Ullah, R., Abd Rahman, R., Ahmed, R., Wali, K., and Ullah, I. (2023). Aging mechanism of HTV silicone rubber loaded with hybrid nano/micro silica and alumina exposed to concurrent multistress. Polym. Test. 121, 107985. doi:10.1016/j.polymertesting.2023.107985
Vazirinasab, E., Jafari, R., and Momen, G. (2019). Evaluation of atmospheric-pressure plasma parameters to achieve superhydrophobic and self-cleaning HTV silicone rubber surfaces via a single-step, eco-friendly approach. Surf. Coat. Tech. 375, 100–111. doi:10.1016/j.surfcoat.2019.07.005
Wang, F., Wen, G., Fan, F., Zhang, T., and Li, J. (2016). “Turn hydrophobic to superhydrophobic of composite insulators by surface fluorination,” in 2016 IEEE international conference on high voltage engineering and application (ICHVE) (IEEE), 1–4. doi:10.1109/ICHVE.2016.7800815
Yang, S., Wang, J., Wang, Y., Ding, Y., Zhang, W., and Liu, F. (2021). Interfacial polymerized polyamide nanofiltration membrane by demulsification of hexane-in-water droplets through hydrophobic PTFE membrane: membrane performance and formation mechanism. Sep. Purif. Technol. 275, 119227. doi:10.1016/j.seppur.2021.119227
Yu, H., Wang, X., Qi, Z., Wang, X., Zhang, R., and Rong, Y. (2020). Three-step MIR spectroscopy study of PTFE C—F stretching vibration mode. Org. Fluor. Ind. (03), 12–16+38.
Zhang, H., Yang, H., Shentu, B., Chen, S., and Chen, M. (2018). Effect of titanium dioxide on the UV-C ageing behavior of silicone rubber. J. Appl. Polym. Sci. 135 (14), 46099. doi:10.1002/app.46099
Zhang, R., H, Q., Xia, Y., and Li, S. (2017). Plasma jet array treatment to improve the hydrophobicity of contaminated HTV silicone rubber. Plasma Sci. Technol. 19 (10), 105505. doi:10.1088/2058-6272/aa7c16
Keywords: HTV silicone rubber, coupling modification, perfluorooctane trichlorosilicon, hydrophobicity, stability
Citation: Li Q, Lei M, Li Z, Gan Q, Sun Y and Wei X (2024) Preparation and mechanism research on hydrophobic coupling modification of HTV silicone rubber. Front. Mater. 11:1405274. doi: 10.3389/fmats.2024.1405274
Received: 22 March 2024; Accepted: 21 June 2024;
Published: 30 July 2024.
Edited by:
Guilherme Mariz de Oliveira Barra, Federal University of Santa Catarina, BrazilReviewed by:
Khubab Shaker, National Textile University, PakistanSabine Hirth, BASF, Germany
Mehmet Gürsoy, Konya Technical University, Türkiye
Copyright © 2024 Li, Lei, Li, Gan, Sun and Wei. This is an open-access article distributed under the terms of the Creative Commons Attribution License (CC BY). The use, distribution or reproduction in other forums is permitted, provided the original author(s) and the copyright owner(s) are credited and that the original publication in this journal is cited, in accordance with accepted academic practice. No use, distribution or reproduction is permitted which does not comply with these terms.
*Correspondence: Qiang Li, liqiang.outlook@qq.com