- 1Laboratory of Chemical and Environmental Technology, Department of Chemistry, Aristotle University of Thessaloniki, Thessaloniki, Greece
- 2Laboratory of Environmental Engineering and Planning, Department of Civil Engineering, Aristotle University of Thessaloniki, Thessaloniki, Greece
- 3Université Paris-Saclay, INRAE, AgroParisTech, Micalis Institute, Jouy-en-Josas, France
- 4Laboratory of Engineering Mechanics, Department of Civil Engineering, Aristotle University of Thessaloniki, Thessaloniki, Greece
The prolonged duration of future manned space missions conceals potential threats associated with microbial contamination. Such closed environments are susceptible to formation of complex biofilm communities, where microorganisms can thrive and further evolve. The objective of this study was to evaluate the impact of surface type, surface treatment and shear stress on biofilm formation in water facilities. To that aim, the ability of Pseudomonas fluorescens SBW25 to adhere on three space applications related materials, including passivated (SS) and both passivated and electropolished (SSEP) stainless steel, as well as Ti-6Al-4V (Ti) alloy was studied under stagnant and shear stress conditions after 24 h of exposure. Results indicated that surface type strongly affects bacterial adhesion under the same conditions. Surface coverage during static experiments was in the following order: SS > Ti > SSEP, while SS exhibited a fourfold surface coverage compared to SSEP highlighting the significance of surface treatment. Moreover, SS and Ti stimulate the formation of several microcolonies and their growth. On the other hand, the application of shear stress diminished bacterial attachment to the studied materials, the degree of which relied on the material type. In this case, bacterial settlement on SS and Ti was dependent on the surface texture, implying that surface roughness may also play an important role in cell adhesion under shear conditions. Furthermore, the metallic surfaces did not hinder bacterial attachment when silver ions were previously deposited on their surface. The deposition that occurs on metallic surfaces when in contact with water disinfected with silver ions, for example, during space missions, highlights its impact on the loss of disinfection capacity of silver ions.
1 Introduction
The need for space and planetary exploration has increased dramatically. The National Aeronautics and Space Administration (NASA) already designs future manned missions with the aim to shed light on important questions that concern humanity (Nat Microbiol, 2022). However, microbes are considered in most cases an enemy to this attempt. These tiny life forms can adhere either on biotic or abiotic surfaces (Noirot-Gros et al., 2019), proliferate, and generate structurally complex and dynamic communities named biofilms. Such a growth lifestyle is a widespread survival mode among microbes providing them protection from harsh conditions while reaping the benefits of co-living at the same time (Hall-Stoodley et al., 2004; Jefferson, 2004). To that goal, microorganisms that participate in the biofilm formation synthesize an extracellular matrix (EPS) consisting of bio-macromolecules such as extracellular DNA (eDNA), polysaccharides and proteins, as well as they notably reprogram their metabolic activities compared to their non-biofilm-counterparts (Penesyan et al., 2021; Malviya et al., 2023). The ability of microbial adhesion on a surface is a complex phenomenon that relies on a variety of factors, such as the physicochemical features of the substrate, e.g., chemistry, roughness, wettability, charge, stiffness, topography, surface orientation, the environmental conditions, e.g., pH, temperature, pressure, O2 availability, microgravity, osmolarity, specific ion and nutrient presence, as well as the kind of microorganism itself (Goller and Romeo, 2008; Acres et al., 2021; Zheng et al., 2021; Jha et al., 2022).
Biofilms’ mode thrives in wet environments such as those provided by water systems addressing potentially detrimental effects on safety (Kretschmer et al., 2021). Nevertheless, this is not an issue associated only with Earth’s environment. Despite the applied purification procedures, several microorganisms have been isolated from International Space Station (ISS) potable water systems, thus raising concerns about the quality of water and efficiency of facilities (Bruce et al., 2005; O’Rourke et al., 2020; Yang et al., 2021). Taking also into account the exposure to microgravity, microbial biofilms can display unpredicted behavior related to increased virulence and antimicrobial resistance (Yang et al., 2021). It is perfectly understood that for any extended space mission, including the upcoming travel to the Moon and Mars (Vélez Justiniano et al., 2023), uncontaminated water is really essential. Therefore, it is pivotal to diminish the microbiological threats that could possibly risk the crew’s health and mission success. More specifically, biofilm generation constitutes a significant threat to the life of astronauts who depend on clean water for drinking, cooking and hygiene purposes, whereas its accumulation in space water systems can lead to clogging of pipes and filters and subsequent corrosion of equipment (Liu et al., 2016).
The careful selection of surface materials is of vital importance, as surfaces are the foundation upon which biofilms grow. Currently, the construction of space water distribution systems involves the use of various materials based on their unique properties and suitability to withstand the harsh space environment (Finckenor, 2018; Ghidini, 2018). Among them, metallic surfaces such as stainless steel (SS), either passivated or electropolished and titanium alloy (Ti-6Al-4V) are primarily used as construction material of pipes, junctions, etc (Petala et al., 2020). Regarding the preservation of water, NASA intends to utilize biocidal silver in order to ensure the quality of potable water and prevent the harmful effects of microorganisms (Petala et al., 2017). The antimicrobial potential of silver is already well-known including deactivation of macromolecules such as DNA, RNA and proteins, cell membrane disruption and reactive oxygen species (ROS) generation (Kędziora et al., 2021). However, according to ESA and NASA documents (Rebeyre, 2012; Li et al., 2018; Li et al., 2019; Muirhead et al., 2020) as well the studies of our research group (Petala et al., 2017; Mintsouli et al., 2018), silver is depleted from water when it is in contact with metallic surfaces due to deposition phenomena. Based on those findings, the aim of the present work is to evaluate the contribution of space-relevant parameters to the potential biofilm formation inside water distribution systems. More specifically, the main objective is the impact of different kind of surface materials, and of shear stress conditions (i.e., stagnant vs. shaking) on the initial stages of biofilm formation. Preventing the process at this stage can inhibit the evolvement into mature biofilms, thereby diminishing the biofilm-related issues that could threaten an extended space-mission (Fu et al., 2021). To this regard, various parameters were tested during the formation of biofilm utilizing a widely-used strain, prior to implementing more complex studies with multi-strain or mixed-species cultures. For our purpose, Pseudomonas fluorescens SBW25, a well-studied bacterium for its biofilm formation capacity (Koza et al., 2009; Jerdan et al., 2020), is used as a model microorganism to investigate bacteria adhesion, which reflects the effectiveness of initial stages of biofilm attachment and is considered as the first step in biofilm formation that governs the mature biofilm structure cultivated on top wetted surfaces and in contact to the cultivation medium (Flemming and Wingender, 2010; Huang et al., 2015). P. fluorescens belongs to Gram-negative bacteria, which constitute predominantly identified species in space water systems and it has been isolated from ISS potable sources and Mir station (Bruce et al., 2005; Song and Leff, 2005). To our knowledge, it is the first time that the ability of these bacteria to form biofilms is studied at the solid-liquid interfaces, which are space applications related materials.
2 Materials and methods
2.1 Tested coupons
All metallic coupons (ISS grade) including stainless steel SS316L passivated (SS) according to NASA/JSC PRC-5002 type VI specification, SS316L passivated as previously and electropolished (SSEP), as well as titanium alloy (Ti-6Al-4V) were purchased from Metal Samples (Alabama, United States) and met the specifications defined for water pipes or fitting for space applications. Dimensions of each coupon corresponding to Length × Width × Depth were 76 × 12.7 × 1.6 mm, respectively. Details about both the elemental composition and fabrication procedure are described previously (Petala et al., 2017).
2.1.1 Surface cleaning
The sterilization of metallic coupons was performed in two steps. During the first step, each surface was gently washed with commercial detergent with the aid of a soft toothbrush. Afterwards, the surfaces were rinsed with distilled water and immersed into 70% v/v ethanol solution for 20 min. Then, they were rinsed again with distilled water and finally were dried once left for 1–2 h inside a laminar flow cabinet, class II, type A2 (Savvy, Lamsystems), to prevent airborne contamination.
2.2 Roughness measurement
Analysis of clean metallic surfaces was carried out employing a 3D Optical Surface Metrology System Leica DCM8 device according to ISO 25178. Three random images of 850 μm × 650 μm were obtained (×20 magnification) for each coupon. Images were subsequently analyzed employing the Leica Map software (LeicaGmbH, Germany) and the topographical profile was evaluated. More specifically, the parameters of the roughness profile (Sdr, %), the root mean square height of the surface (Sq, μm), the maximum height of peaks (Sp, μm), the maximum height of valleys (Sv, μm), the maximum height of the surface (Sz, μm) and the arithmetical mean height of the surface (Sa, μm) were determined.
2.3 Contact angle measurement
The surface wettability was estimated using the mobile surface analyzer One-Click SFE (Krüss Scientific) by calculating the contact angle (CA) between each metallic coupon and a drop (5 μL) of distilled water. For each coupon, three drops were measured for each metallic surface to investigate repeatability for each coupon, while two different coupons were tested to investigate the repeatability for each type of material.
2.4 Silver deposition to metallic surfaces
First, water was prepared based on Russian water standards for disinfection water, containing high silver ions concentration (equal to 10 mg/L), used to condition water storage tanks (Petala et al., 2017). Silver ions were introduced to water using a CSG-1 Silver Water Generator in a sterile environment. Afterwards, the water was filtered through a 0.2 μm pore-size filter. Subsequently, one metallic coupon of each material was immersed in a rectangular-shaped polypropylene cell (that is inert to silver deposition) of a multicell plate and it was exposed to silver-ion treated water at a S/V (surface/volume) equal to 1.0 cm−1, containing 10 mg/L silver. The multicell plate was placed at 30°C for 5 days in dark allow deposition of silver ions. Afterwards, coupons were dried and used for testing, while silver ion concentration was determined in the bulk water to verify silver deposition, as described elsewhere (Petala et al., 2017). The dissolved silver in water was estimated prior and after the exposure period spectrophotometrically utilizing the LCK 354 method by Hach Lange.
2.5 Bacterial cultures and growth conditions
Pseudomonas fluorescens SBW25 was selected for this study as a model microorganism due to its non-pathogenic nature and its ability to form biofilms. A frozen glycerol stock (−80°C) of P. fluorescens was streaked out on a sterile tryptic soy agar (TSA) 1.5% plate and incubated at 30°C for 24 h. Afterwards, a colony was picked and suspended in 10 mL of fresh, sterile tryptic soy broth (TSB) to obtain the overnight culture, which was placed in a shaking incubator at 30°C for 12–16 h. Then, the overnight culture was diluted to OD600 = 0.1 in fresh, sterile TSB and incubated at 30°C under shaking until it reached OD600 = 0.5. The same procedure was repeated for each experiment.
2.6 Bacterial adhesion assay
P. fluorescens was cultivated over metallic coupons, either containing deposited silver or not, at 30°C for 24 h using fresh, sterile TSB, which is widely preferred as a growth nutrient medium in biofilm formation assays (Lade et al., 2019). The samples were placed horizontally in sterile Petri dishes (9 cm diameter). More specifically, 18 mL of TSB and 2 mL of bacteria at OD600 = 0.5 (1:10 dilution) were added in each Petri dish. The experiments were performed either under static or shaking (100 rpm) conditions. After 24 h, the medium was carefully removed using a pipette and metallic surfaces were rinsed with 10 mL of distilled water in order to remove the planktonic cells and ensure the presence of only strongly attached cells. The surfaces were hold with a sterile tong at the corner so as to reduce the impact on the biofilm, while each mL of water was gently applied at the edge of the coupon and flowed across the surface. No planktonic or loosely attached bacteria were found on the surface after this procedure. Three independent experiments were performed.
2.7 Fluorescence microscopy
For visualization of bacterial adhesion on metallic surfaces, the protocol of co-staining with Syto nine and Propidium Iodide (PI) (FilmTracer LIVE/DEAD Biofilm Viability kit, Invitrogen) was performed, according to the instructions of the supplier. Briefly, working stock solutions of stains were freshly prepared adding 3 μL of each stain in 1 mL of distilled water, while 200 μL of final solution were used in order to stain the metallic coupons. After incubation for 30 min in dark place at room temperature, the surfaces were rinsed with distilled water to remove the excess of staining. A Nikon Eclipse Ci fluorescence microscope equipped with a Color Camera Nikon DS-Fi-3 was used for capturing random images of each surface (×20 magnification, 0.17 μm/pixel).
2.7.1 Image processing analysis
Image analysis was performed employing the free-access processing package Fiji (ImageJ, US National Institutes of Health, Bethesda, Maryland, United States) (Katsipis et al., 2023). Concerning the analysis, the experimental images were firstly converted to 8-bit greyscale images. Subsequently, the auto local threshold Bernsen method was applied to generate binary images distinguishing background from the items of interest. Analysis of particles was carried out providing total surface coverage (%), number and size of detected particles. For each surface, at least six random images of the surface were obtained and further analyzed statistically.
3 Results
3.1 Surface roughness
Confocal microscopy was employed to evaluate the roughness of the clean metallic coupons (Figure 1). Based on Sdr which is considered the ratio between the area of the “projected” surface and the area of the “real” developed surface, the roughness of the material can be evaluated. The higher this parameter, the greater the roughness of the surface. It can be observed that among the studied surfaces, SS displayed the highest roughness (Sdr = 10.8 ± 0.5%), while electropolishing technique led to a final material with a considerably lower roughness (Sdr = 0.06 ± 0.01%). The Ti alloy surface was less rough than SS (Sdr = 3.9 ± 0.5%). The presence of grooves along the Ti and SS coupons were also visible by naked eye. Parameters obtained by 3D profiles describing surface topology are summarized in Supplementary Table S1. It should be mentioned that the maximum height of the peaks was calculated to be 6.4 ± 0.6, 3.8 ± 0.1 and 0.6 ± 0.06 μm for SS, Ti and SSEP, respectively, while the maximum height of the valleys was 8.8 ± 0.4, 5.2 ± 0.8 and 1.7 ± 0.5 μm, respectively. A single P. fluorescens cell has a diameter of approximately (0.9 ± 0.1) and length (3.1 ± 0.8) μm (Ping et al., 2013).
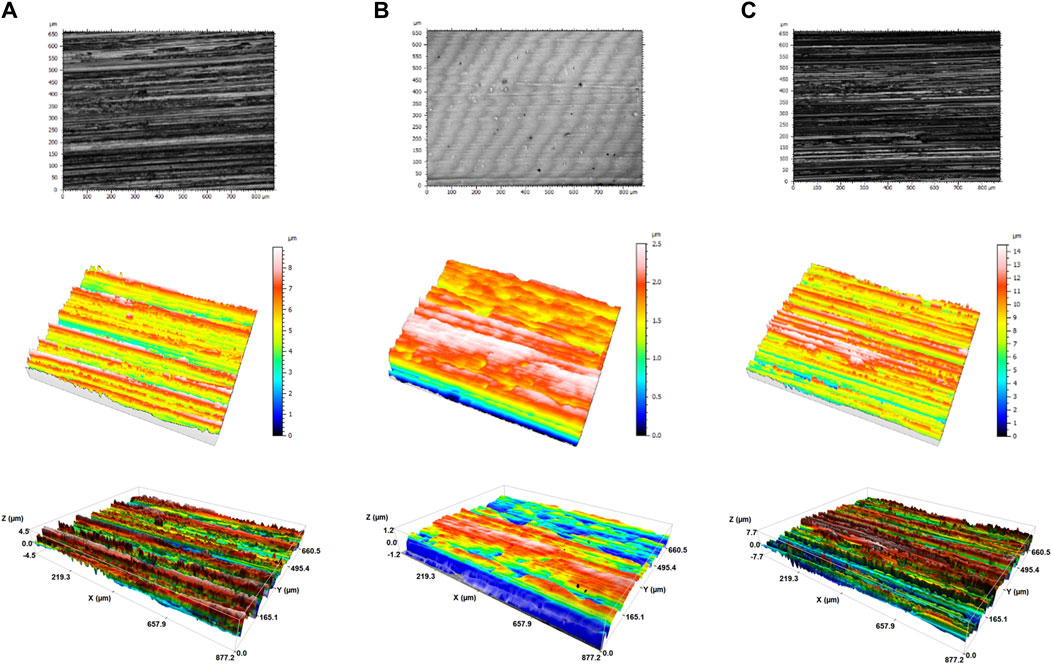
Figure 1. 3D images of clean surfaces (A) Ti-6Al-4V (B) SSEP and (C) SS obtained by confocal microscopy (3D Optical Surface Metrology System Leica DCM8).
3.2 Surface wettability
The wettability of each surface was estimated by measuring the contact angle of water droplets (θw). When θw < 90 the surface is considered hydrophilic, while when θw > 90 the surface is hydrophobic (Wang et al., 2023). Notably, θw concerning the rough surfaces of SS and Ti, respectively, exhibits an anisotropic effect dependent on the planar direction of the surface. More specifically, when the contact angle was estimated perpendicular to the grooves of SS, it was found 76.3° ± 3.0°, displaying a hydrophilic nature. However, when the measurements took place parallel to the grooves, the contact angle was 100.6° ± 5.5°, presenting hydrophobic properties. A similar behavior of anisotropy was detected by employing the Ti surface. The contact angle was 61.8° ± 1.2° for perpendicular and 83.9° ± 0.9° for parallel measurements, respectively. On the contrary, the smoothness of the SSEP surface resulted in a constant contact angle of 85.1° ± 1.8° at both directions. The average values of measured contact angles and representative photos are shown in Figure 2.
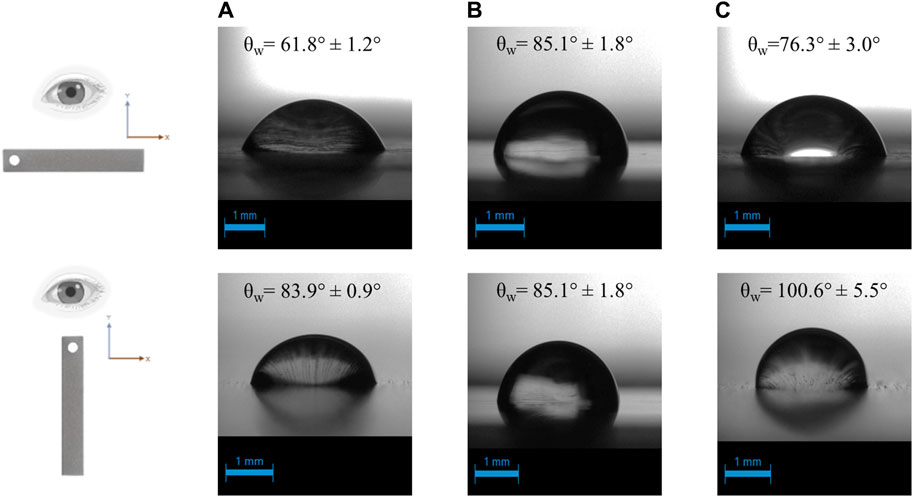
Figure 2. Contact angle of water droplet on (A) Ti-6Al-4V (B) SSEP and (C) SS. The measurement was planar-dependent for stainless steel and titanium surface owing to their physical irregularities.
3.3 Evaluation of Pseudomonas fluorescens adhesion on metallic surfaces under static conditions
The ability of P. fluorescens SBW25 to adhere on different metallic surfaces, including SSEP, SS and Ti was evaluated using the Syto 9/PI co-staining method. Syto nine can penetrate all bacteria independently to their membrane integrity and bind to their DNA emitting a green signal. On the other hand, PI can penetrate only bacteria with damaged membranes emitting red fluorescence. As a result, green signals are interpreted as live bacteria, whereas red or yellow/orange signals are considered as dead (Rosenberg et al., 2019). Before visualization, samples were rinsed sufficiently to ensure the presence only of strongly adherent bacteria or potential irreversibly attached cells.
As shown in fluorescence images (Figure 3), P. fluorescens displays a higher affinity for SS among the three studied surfaces under static conditions. Moreover, it is observed that SS and Ti surfaces favor the formation of cell clusters compared to SSEP, where single cells are mainly observed. It should be underlined, that the images captured for SS and Ti was a difficult task to deal with, taking into consideration the surface roughness which lead to a loss of local focus. Nevertheless, images revealed that SS and Ti surfaces were more prone to biofilm formation compared to SSEP. Furthermore, bacterial adhesion was rather independent to surface texture, as no indication of single cells or cluster arrangements were detected with respect to grooving-patterns.
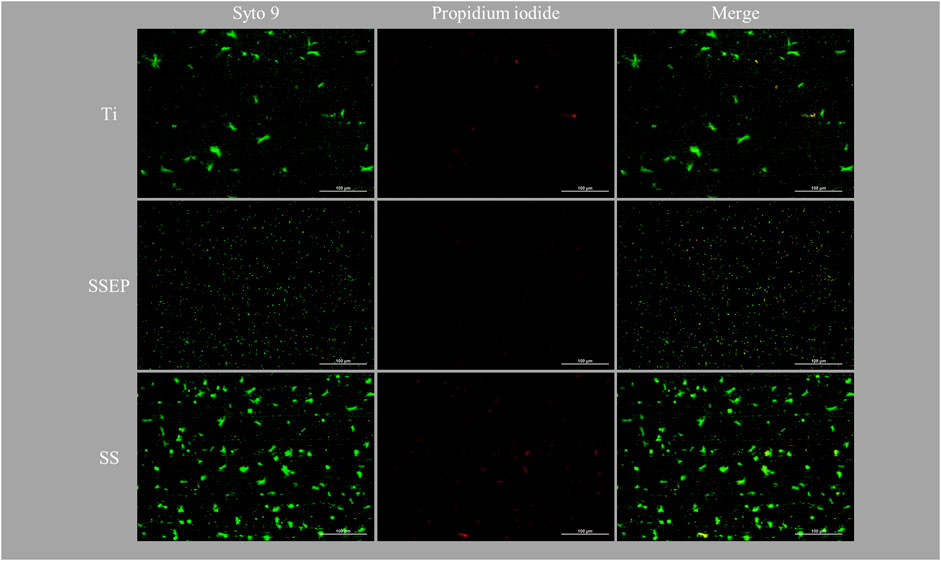
Figure 3. Impact of material surface on 24 h adhesion of Pseudomonas fluorescens under static conditions employing i) Ti-6Al-4V ii) SSEP and iii) SS. Bacteria were stained with co-staining method of Syto 9/PI. The composites of two fluorescence channels are also displayed.
The observations were further confirmed quantitively by image processing analysis (Figure 4 and Supplementary Table S2). Each experimental image represents an area of about 0.17 mm2. SS exhibits surface coverage of 4.7% ± 0.2%, whereas Ti and SSEP present surface coverage of 2.4% ± 0.2% and 1.2% ± 0.3%, respectively (Figure 4A), after 24 h of exposure. Additionally, from size distribution analysis (Figure 4B), there is a particle size shift to smaller ones for SSEP, indicating that P. fluorescens is mainly found at a single cell state or small clusters of odd number of cells after 24 h of contact between medium and SSEP. On the contrary, Ti and SS seem to stimulate the formation of bigger clusters, with the latter one to a higher extent, with more evident the presence of dead cells, as well (Figure 4B). Moreover, comparing the number of particles, SS shows approximately threefold increase compared to SSEP (Figure 4C).
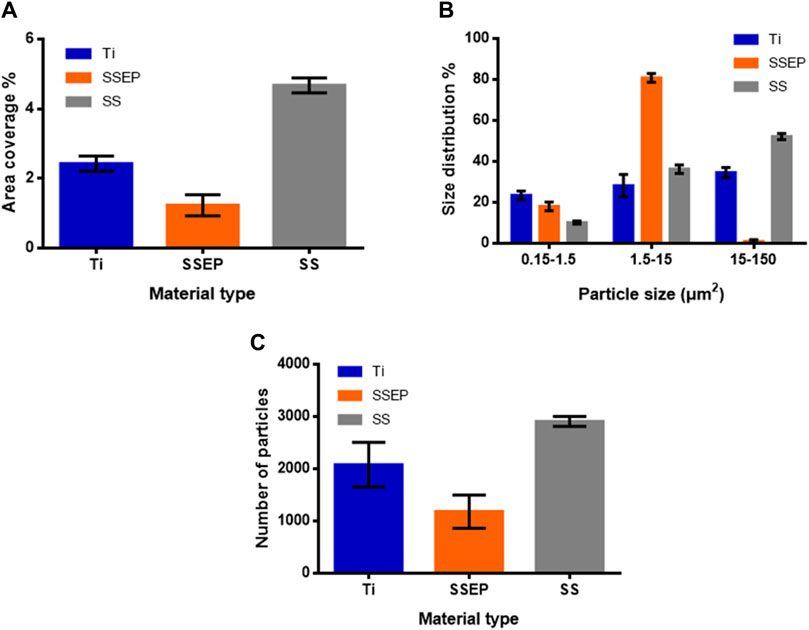
Figure 4. Quantitative analysis of Pseudomonas fluorescens adhesion on different metallic surfaces under static conditions derived from image processing with ImageJ software. (A) Surface coverage (%) (B) distribution (%) of particles based on their size (C) number of detected particles. Displayed graphs include error bars representing standard deviation (SD) from three independent experiments.
3.4 Evaluation of Pseudomonas fluorescens adhesion on metallic surfaces under shaking conditions
While many studies evaluate the effect of shaking conditions after a time period of static bacterial adhesion on the surface, e.g., 2 h, here we assessed the impact of shear stress on bacteria attachment by starting to shake at 100 rpm from the beginning of each test (t = 0 h), i.e., without static incubation period. As shown in fluorescence images (Figure 5), shaking conditions affect intensely the adhesion of P. fluorescens on all the studied surfaces. This may be attributed either to difficulty of cells to reach and adhere to the wetted surface or to cell growth in planktonic form due to efficient oxygen conduction from the liquid-air interface and local nutrient availability due to mixing conditions. Similar to static conditions, SSEP displays the lowest bacterial coverage among the surfaces. However, in contrast to static mode, it seems that biofilm surface coverage was comparable between SS and Ti, without statistically significant changes. Furthermore, by close observation of Figure 5 for SS and Ti, microorganisms appear oriented along the grooves of these surfaces, roughly indicating that surface affects initial stages of biofilm formation.
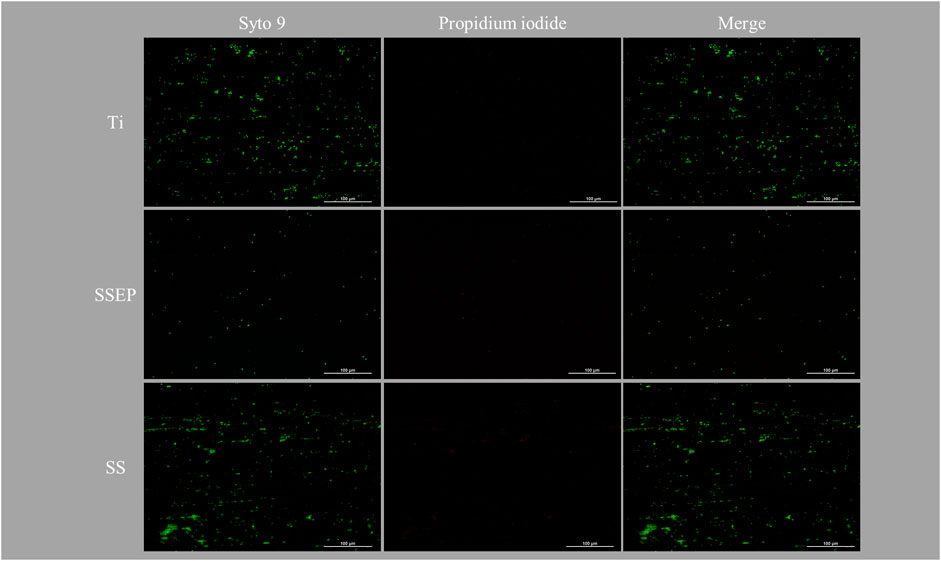
Figure 5. Impact of material surface on 24 h adhesion of Pseudomonas fluorescens under shaking conditions employing i) Ti-6Al-4V ii) SSEP and iii) SS. Bacteria were stained with co-staining method of Syto 9/PI. The composites of two fluorescence channels are also displayed.
Image analysis provides more insights with respect to this behavior. Based on the derived graphs (Figure 6 and Supplementary Table S2), the detected particles are obviously less compared to static conditions indicating the inhibition of bacterial adhesion under shaking conditions (Figure 6C). In addition, SSEP displays the lowest surface coverage (0.2% ± 0.04%), compared to SS (1.1% ± 0.2%) and Ti (1.1% ± 0.08%) which exhibit an identical behavior under shaking mode (Figure 6A). Moreover, bacteria tend to generate bigger clusters on these surfaces. In total, the size distribution is shifted towards smaller cells. In particular, on SSEP, single cells or even smaller forms -vesicles- that are formed due to stress conditions, account for more than 30% of total counts (Figure 6B).
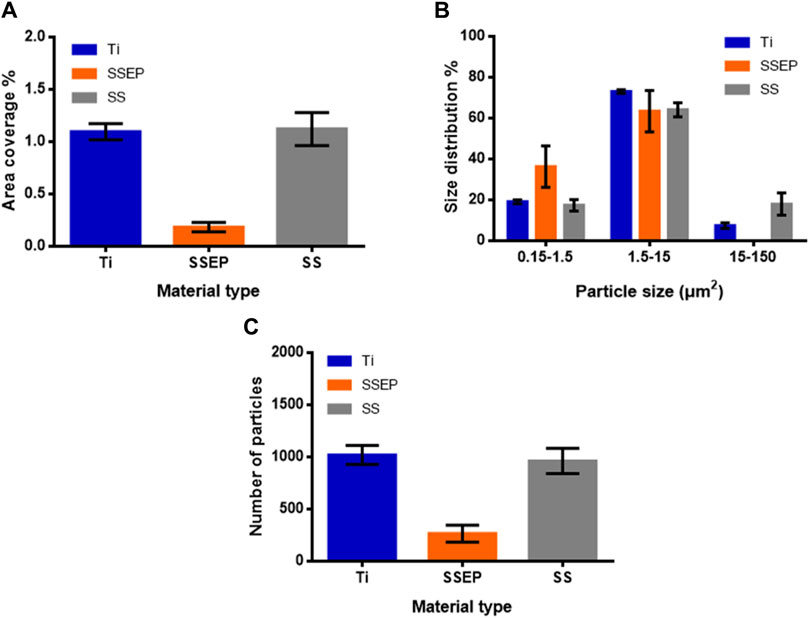
Figure 6. Quantitative analysis of Pseudomonas fluorescens adhesion on different metallic surfaces under shaking conditions (100 rpm) derived from image processing with ImageJ software. (A) Surface coverage (%) (B) distribution (%) of particles based on their size (C) number of detected particles. Displayed graphs include error bars representing standard deviation (SD) from three independent experiments.
3.5 Evaluation of Pseudomonas fluorescens adhesion on silver-ion exposed metallic surfaces
Finally, we evaluated the ability of P. fluorescens to attach to surfaces, which have been previously exposed to water disinfected with silver ions that ultimately results in silver deposition on the surfaces, mimicking the processes described during water transportation in the frame of previous ATV missions to ISS. Silver deposition was confirmed by performing silver measurements in water bulk and comparing them with previous results. All results were in accordance to our previous studies (Petala et al., 2017; Mintsouli et al., 2018). Based on the obtained fluorescence images (Figure 7), no evident changes are demonstrated compared to the results of untreated surfaces under static conditions (Figure 3). The observed effect was further validated by results of image analysis (Figure 8A–C). More specifically, SSEP displays a coverage of 1.3% ± 0.2%, while Ti and SS show coverages of 2.3% ± 0.2% and 4.6% ± 0.2%, respectively, pointing out that silver deposition on metallic surfaces did not affect the surface behavior towards bacterial adhesion (Figure 8A). The results are also confirmed by the live/dead bacteria analysis as shown in Supplementary Figure S1. It can be noticed that the presence of dead cells is more prominent for the SS under the applied conditions. Furthermore, according to size distribution graph, silver deposition did not also affect the sizes of bacterial aggregates, as P. fluorescens is mainly found as single cells on SSEP, while Ti and SS promote the formation of bigger clusters (Figure 8B). A summary with all the calculated parameters derived from the aforementioned experiments are presented in Supplementary Table S2.
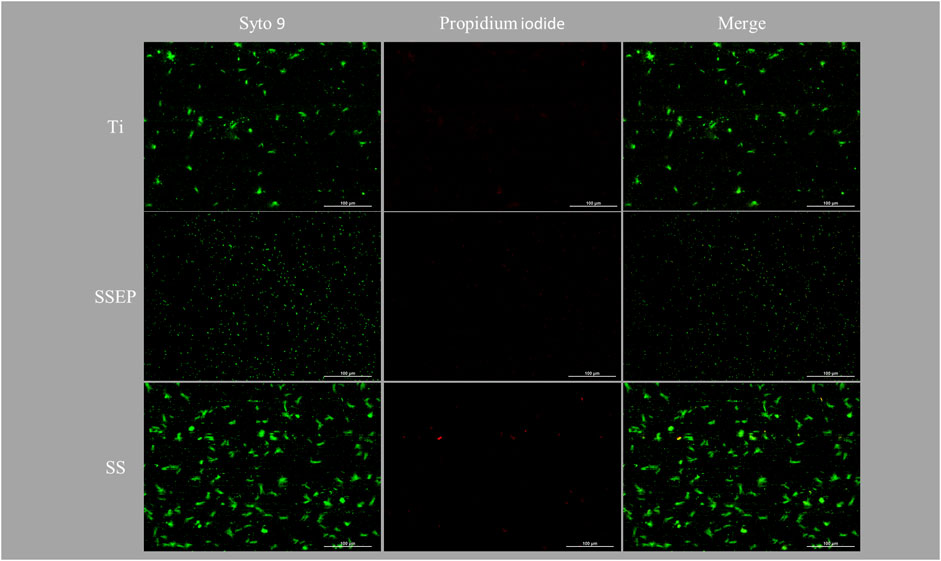
Figure 7. Impact of material surface on 24 h adhesion of Pseudomonas fluorescens under static conditions employing silver-ion treated i) Ti-6Al-4V ii) SSEP and iii) SS. Bacteria were stained with co-staining method of Syto 9/PI. The composites of two fluorescence channels are also displayed.
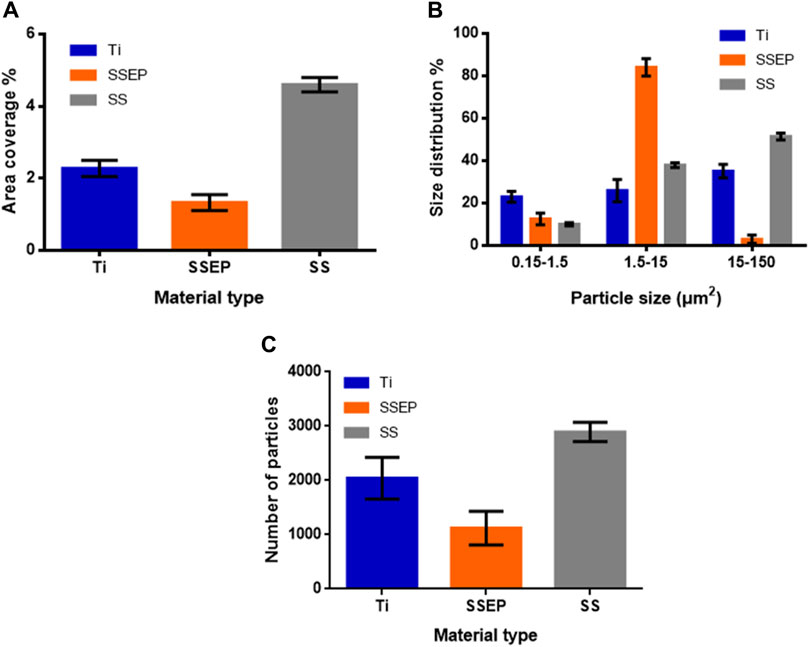
Figure 8. Quantitative analysis of Pseudomonas fluorescens adhesion on different metallic, silver-ion water exposed surfaces under static conditions derived from image processing with ImageJ software. (A) Surface coverage (%) (B) distribution (%) of particles based on their size (C) number of detected particles. Displayed graphs include error bars representing standard deviation (SD) from three independent experiments.
4 Discussion
The aim of this study was to evaluate the role of surface type and treatment, as well as shear stress met in water systems of space missions-related infrastructure on biofilm formation.
To this direction, the ability of P. fluorescens SBW25 to adhere on different metallic surfaces under different applied conditions was examined, while Ti, passivated SS and SSEP surfaces, which are used in several parts of space water distribution systems (tubes, junctions, etc.), were selected as substrates for investigating bacterial adhesion, which is critical for later biofilm structure cultivated under culture medium (Flemming and Wingender, 2010; Huang et al., 2015). The behavior of P. fluorescens was evaluated after 24 h exposure, which is considered a premature stage of biofilm formation. Biofilms at an early stage are more fragile exhibiting vigorous metabolic activities and higher susceptibility to disinfectants and antimicrobial agents, thus it is easier to remove them at that point (Fu et al., 2021). In addition, these early biofilm configurations strongly affect the regulation of more developed structures. Hence, it is important to understand what factors affect their evolvement. The results of this study should be used to further expand its scope, so as to include the understanding of the behavior of more complex systems (containing different bacterial strains and/or species) close to real space environments.
The wetted surfaces found in water systems serve as the basis upon which microbes grow and generate their biofilm communities, thus it is important to evaluate their features. Based on the confocal measurements, it is found that passivated SS was the roughest surface among the three substrates, while SSEP was the smoothest. Ti surface exhibited an intermediate roughness. In addition, wettability experiments provided interesting results concerning the material properties. More specifically, wetting behavior was not uniform in all directions for the rough surfaces SS and Ti, respectively. A high degree of anisotropy was noticed particularly for SS. This could be attributed to the grooves and crevices of these materials (Tardelli et al., 2023). If water enters the grooves, the energy cost is higher for the molecules to disperse perpendicular compared to flowing along them. This occurs due to the fact that groove walls function as pinning points, providing additional support with the surface tension. As a result, the contact angles were larger when observing the droplet from the direction of the grooves (Park et al., 2021). It should be mentioned here that not only the wettability of a surface could affect the biofilm formation, but also the biofilm formation could impact the wetting characteristics of a surface (Recupido et al., 2020; Castigliano et al., 2021; Kampouraki et al., 2022; Recupido et al., 2022; Recupido et al., 2023). Moreover, rough surfaces are colonized more easily providing an increased contact area for cell adhesion, as well as a protected environment for microorganisms against shear stress (Zheng et al., 2021). This is attributed to their features such as grooves and irregularities which favor microbial attachment (Tardelli et al., 2023). However, there are some studies pointing out contradictory outcomes regarding this parameter. Wu et al. reported that Gram-negative Pseudomonas aeruginosa and Gram-positive Staphylococcus aureus colonized a stainless steel electropolished surface more efficiently compared to the untreated rougher surface (Wu et al., 2018). In another study, increased roughness did not affect the adherence of Gram-positive Staphylococcus epidermis on titanium and ceramic substrates, while it enhanced the adhesion of Streptococcus sanguinis, especially on ceramic surfaces (Wassmann et al., 2017). However, the above observed results may not be exclusively attributed to roughness, but other factors as well, e.g., surface chemistry.
In the present work, passivated SS was more prone to bacterial adhesion under static conditions, followed by Ti and SSEP, respectively. Apparently, there is a positive correlation between adhesion of P. fluorescens and surface roughness. Furthermore, it was observed that after 24 h exposure, the rough surfaces promoted the formation of bigger clusters varying in size and shape, compared to SSEP where single cells were mainly noticed. The distribution of these clusters exhibits a spatial organization enabling bacteria to communicate with each other through molecular mechanisms, i.e., quorum sensing (van Hoogstraten et al., 2023). Microscopic images similar to those for SSEP were captured for smooth glass and polydimethylsiloxane surface (PDMS), when exposed to cultures of P. aeruginosa PAO1 and P. aeruginosa PA-14, respectively (Epstein et al., 2013; Shah et al., 2019).
Besides surface roughness, chemical interactions can play crucial role in biofilm development. In fact, electropolishing is a technique that not only provides smoothness on a surface, but it also alters the exterior layer of the given material. Analyzing the XPS graphs from our previous works (Petala et al., 2016; Petala et al., 2017) for passivated SS and SSEP, respectively, it is shown that SS surface has a high peak corresponding to iron, an element which is absent from SSEP surface. Iron is a crucial agent for cell viability and biofilm development. High iron concentration acts as a stimulus for P. aeruginosa to form microcolonies and subsequently a mature biofilm, whereas low concentration hinders the biofilm growth (Soldano et al., 2020). Furthermore, it is reported that bacteria utilize elements such as iron and magnesium from the metal surfaces on which they are attached, in order to exploit them as terminal electron acceptors in their vital respiration process (Tuson and Weibel, 2013). When oxygen is absent, iron can serve as the terminal electron acceptor in the deep layer of the biofilm (Charron-Lamoureux et al., 2023). In our case, during static culture conditions where the diffusion of oxygen is poor, it might be presumed that microenvironments of low oxygen or no oxygen are derived, especially at the boundary region separating the solid from the liquid phase. Such a condition could impede important biological activities of bacteria which will search eventually for alternative solutions. As a consequence, the chemistry of the surface in terms of elemental composition might also be a crucial factor that determines the extent of bacterial adhesion. Apart from iron, other elements might also act as signaling molecules regulating gene expression and communication or the secretion of EPS which is essential for biofilm formation and maintenance (Wu et al., 2022).
In general, concerning the material type, it is proved that electropolishing constitutes a particularly effective treatment preventing bacterial accumulation. Owing to its better and more uniform texture, without local wetting changes compared to SS appears as a surface that could withstand early biofilm formation. The higher bacterial coverage of SS, as well as the formation of several big clusters indicated by the graph-shift to higher size ranges, implies also a faster kinetic model of bacterial colonization and the stimulation of the P. fluorescens adhesion. On the other hand, Ti is a chemically different surface. Due to the combination of high strength and low weight, it is also considered a great candidate for future space missions. Although it seems to resist bacterial adhesion compared to SS, the detection of many particles on the surface, which could act as nuclei, might potentially lead to high levels of biofilm formation in the long term.
Τhe impact of applied shear stress was also investigated to provide insights on biofilm formation. Herein, the effect was intense for all the studied surfaces exhibiting a significantly lower bacterial attachment compared to stagnant conditions. Similar to static mode, SSEP proved to accommodate remarkably less adherent bacteria compared to SS and Ti. However, in contrast to static conditions, SS and Ti presented the same behavior without differences in bacterial adhesion. Taking also into account the observed orientation of bacteria along the grooves of these surfaces, might imply that such irregularities could indeed serve as protected environments against shear stress. Since SS and Ti are of different chemical composition but of comparable roughness, it is suggested that gravity and texture features of wetted surfaces are more crucial factors in cells’ attachment and biofilm formation compared to surface chemistry for the tested materials. On the whole, it is important to test the material type under different conditions for a better surface evaluation before its application. For longer periods of biofilm growth, the application of hydrodynamic stress on P. fluorescens B52 biofilms over borosilicate glass substrates was critical and led to intensification of biofilm growth, most probably due to higher production of EPS that demonstrated modified macromolecule composition of extracellular matrix compared to the static mode. The formation of thicker and denser biofilms under the tested conditions was attributed to the increased rate of nutrient diffusion and the agitation-assisted attachment of the free-living counterparts to the already generated biofilm colonies (Hou et al., 2018; Jara et al., 2021). Other studies underline the impact of shear stress on the biofilm growth as well, however they all refer to more mature and well-structured biofilms and not to first stages of biofilm formation, as in this study (Tsagkari and Sloan, 2018; Jara et al., 2021). Furthermore, the use of a specific culture medium could influence the interpretation of the results. Different culture media could affect the growth and metabolism of bacteria due to varying concentrations in carbon and nitrogen sources, minerals and other nutrients. The aforementioned components are utilized by microorganisms for cell growth, energy demands and synthesis of extracellular polymeric substances, therefore their availability, as well as their concentration in the environment could either promote or hinder biofilm formation.
The ability of microorganisms to attach to a surface derives from their motility and their passive movement governed by processes such as Brownian motion, gravitational sedimentation and convective mass transfer (Krsmanovic et al., 2021). However, without the proximity between cells and surface, the attachment will not occur (Marra et al., 2022). During shaking mode, it is expected that fewer cells will be near the solid-liquid interface available for attachment compared to static conditions. The final number of cells close to interface is dependent on flow characteristics (leading to passive approach to the surface) or active movement towards to the surface due to cell motility. Yet, cell attachment is related to its contact with the surface and subsequent successful anchoring, which is driven by different underlying mechanisms. In our study, it is justified that the surface type dominates the final bacterial attachment, since applied experimental conditions and shear stress are the same for all materials, while surface coverage is significantly lower for SSEP compared to Ti and SS (Figure 5; Figure 6). Bacterial settlement along the grooves of Ti and SS (materials of different chemical composition) imply that texture might be an important feature affecting the initial adhesion. Motility could be the tool that enables bacteria to navigate through the surface irregularities and detect appropriate sites for attachment.
It should be stressed that in all cases, the stained bacteria or clusters are considered strongly attached to wetted surfaces, due to handling practices chosen in this study, to avoid misinterpretations and overestimations. Sample rinsing is a critical step before visualization. In the study of Reddersen et al., it was shown that the P. fluorescens coverage was reduced about ten times after rinsing of the substrate (Reddersen et al., 2021). This finding raises concerns about the proper way of sample handling. It is known that the accumulation of microorganisms on surfaces normally results to biofilm formation (Wang et al., 2023). However, it must be taken into consideration that initial attachment does not lead inevitably to irreversible adhesion, as microorganisms might firstly sense the surface by means of appendage proteins that they possess (Kimkes and Heinemann, 2019). In fact, the cells that are attached irreversibly on the substrate will divide, generate microcolonies and subsequently produce the EPS that is characteristic of biofilms (Donlan, 2001). In addition, biofilm formation is mainly evaluated when surfaces are placed horizontally (Jha et al., 2022). However, due to gravitational sedimentation of bacteria, there is a high rate of cells that might be loosely attached. Actually, when the impact of a surface is studied under specific conditions, we should be interested exclusively in the forces between the substrate and the microorganism such as van der Waals forces, electrostatic forces and hydrophobic interactions (Zhao et al., 2023). Appropriate rinsing assures the presence only of strongly attached cells, which might subsequently be responsible for biofilm formation. The fact that there is no standard assay about this process could lead to misinterpretation of results. In particular, the volume of washing buffer and the way of rinsing, i.e., dripping rinsing of the surface with washing buffer (Kumar Jha et al., 2020) vs. immersion of the surface in a washing buffer bath (Niboucha et al., 2022) could have a different effect. It should be highlighted that biofilms are a scientific field with so many contradictory studies concerning the contribution of important physicochemical parameters, thus there is an imperative need for a standardized method. Such issues can be avoided by employing non-invasive methods such as Optical Coherence Tomography (Hou et al., 2019). However, due to the lack of sufficient thickness and a defined structure during the early stages of biofilms, confocal or fluorescence microscopy are mainly suggested for evaluation of phenomenon.
During space missions, water systems undergo different operational conditions, i.e., flow, non-flow, sloshing. To this regard, alterations in surface characteristics may also occur. For example, as described earlier (Petala et al., 2017), silver ions of potable water bulk may be depleted and migrate to water tank or water pipe wall. In this case, disinfection capacity of silver ions is lost in the water bulk and silver is deposited in various forms depending on the type of water surface. This behavior was examined in this study with respect to potential biofilm formation in case of water contamination. It was observed that silver deposition did not alter the adhesion of P. fluorescens regardless of deposition mechanism. More specifically, in the case of SS and SSEP, silver deposits in its metallic form, while in the case of Ti it deposits as oxide (Petala et al., 2017; Mintsouli et al., 2018). It is known that the ionic form of silver presents antibacterial activity, while the non-ionic form is inert (Durmus and Molina Jordá, 2021). This could be an important reason for the absence of antimicrobial effect. Nevertheless, chemical composition of SS and Ti was not altered drastically after silver deposition, while essential elements, such as iron still exist as it is shown from XPS graphs in previous work (Petala et al., 2017; Mintsouli et al., 2018). Interestingly, silver deposition occurs to a considerably lesser extent on SSEP compared to SS and Ti, indicating that biocidal silver ions concentration in bulk is better preserved when SSEP is the wetted surface.
Overall, both the material type and the application of shear stress are crucial parameters that could affect the biofilm formation in space water systems. More specifically, SSEP rises as the best repellent substrate among the three studied surfaces concerning the bacterial accumulation and the potentially subsequent biofilm formation. This finding highlights also the importance of surface treatment. Although passivated SS was more prone to bacterial colonization, electropolished SS was proved to be resistant. Even so, aging of surfaces should be addressed to investigate long term impacts. As a consequence, different treatments might result to different outcomes, something that should be taken into consideration during the manufacturing process. Moreover, the efficiency of material type against bacterial colonization is enhanced under applied shear stress, underlying the impact of shear on cells' behavior. Still, material type was crucial to biofilm surface coverage. However, deposition of silver on metallic surfaces does not influence bacterial adhesion. The silver depletion from water is challenging, as the decrease of concentration renders water susceptible to microbial contamination, while its deposition on metallic surfaces causes loss of its biocidal effect due to deactivation. In this case, the application of SSEP could offer a dual advantage, presenting simultaneously a better microbial resistance and a resistance to silver depletion from water based on our previous findings.
5 Conclusion
As space missions extend their duration, the potential risks associated with microbial contamination increase. Such circumstances could compromise both the astronauts’ health and integrity of spacecraft’s facilities. The water distribution systems constitute an excellent candidate for bacterial growth, colonization and eventually biofilm formation. Therefore, it is essential to perform risk analysis studies and prepare risk management plans related to contamination issues. Towards this direction, the present study indicates that the careful selection of materials used in the construction of space water systems is of vital importance. Parameters such as texture, treatment and chemistry of a surface should be strictly taken into consideration. Controlling the platforms of microbial communities’ growth could eliminate the possible harmful effects. Moreover, the applied conditions to which these surfaces are exposed is another critical aspect. Understanding the dynamics of bacterial biofilm formation could lead to the development of new effective antimicrobial strategies or optimization of existing.
Data availability statement
The original contributions presented in the study are included in the article/Supplementary Material, further inquiries can be directed to the corresponding author.
Author contributions
DIA: Conceptualization, Methodology, Formal Analysis, Investigation, Writing–original draft. MP: Conceptualization, Methodology, Supervision, Writing–review and editing. RB: Writing–review and editing. YD: Methodology, Writing–review and editing. M-FN-G: Writing–review and editing. AK: Validation, Writing–review and editing. MK: Data curation, Writing–review and editing. TDK: Conceptualization, Project administration, Resources, Writing–review and editing.
Funding
The author(s) declare that no financial support was received for the research, authorship, and/or publication of this article.
Conflict of interest
The authors declare that the research was conducted in the absence of any commercial or financial relationships that could be construed as a potential conflict of interest.
The handling editor MH declared a past co-authorship with the authors TDK, MK, RB, MFNG
Publisher’s note
All claims expressed in this article are solely those of the authors and do not necessarily represent those of their affiliated organizations, or those of the publisher, the editors and the reviewers. Any product that may be evaluated in this article, or claim that may be made by its manufacturer, is not guaranteed or endorsed by the publisher.
Supplementary material
The Supplementary Material for this article can be found online at: https://www.frontiersin.org/articles/10.3389/fmats.2024.1401764/full#supplementary-material
References
Acres, J. M., Youngapelian, M. J., and Nadeau, J. (2021). The influence of spaceflight and simulated microgravity on bacterial motility and chemotaxis. NPJ Microgravity 7, 7. doi:10.1038/s41526-021-00135-x
Bruce, R. J., Ott, C. M., Skuratov, V. M., and Pierson, D. L. (2005) Microbial surveillance of potable water sources of the international space station. United States: SAE International.
Castigliano, M., Recupido, F., Petala, M., Kostoglou, M., Caserta, S., and Karapantsios, T. D. (2021). Wetting of dehydrated hydrophilic Pseudomonas fluorescens biofilms under the action of external body forces. Langmuir 37, 10890–10901. doi:10.1021/acs.langmuir.1c00528
Charron-Lamoureux, V., Haroune, L., Pomerleau, M., Hall, L., Orban, F., Leroux, J., et al. (2023). Pulcherriminic acid modulates iron availability and protects against oxidative stress during microbial interactions. Nat. Commun. 14, 2536. doi:10.1038/s41467-023-38222-0
Donlan, R. M. (2001). Biofilm Formation: a clinically relevant microbiological process. Clin. Infect. Dis. 33, 1387–1392. doi:10.1086/322972
Durmus, F. C., and Molina Jordá, J. M. (2021). Silver foams with hierarchical porous structures: from manufacturing to antibacterial activity. ACS Appl. Mater Interfaces 13, 35865–35877. doi:10.1021/acsami.1c06057
Epstein, A. K., Hong, D., Kim, P., and Aizenberg, J. (2013). Biofilm attachment reduction on bioinspired, dynamic, micro-wrinkling surfaces. New J. Phys. 15, 095018. doi:10.1088/1367-2630/15/9/095018
Finckenor, M. M. (2018). “Materials for spacecraft,” in Aerospace materials and applications (Reston ,VA: American Institute of Aeronautics and Astronautics Inc.), 403–434. doi:10.2514/5.9781624104893.0403.0434
Flemming, H. C., and Wingender, J. (2010). The biofilm matrix. Nat. Rev. Microbiol. 8, 623–633. doi:10.1038/nrmicro2415
Fu, J., Zhang, Y., Lin, S., Zhang, W., Shu, G., Lin, J., et al. (2021). Strategies for interfering with bacterial early stage biofilms. Front. Microbiol. 12, 675843. doi:10.3389/fmicb.2021.675843
Ghidini, T. (2018). Materials for space exploration and settlement. Nat. Mater 17, 846–850. doi:10.1038/s41563-018-0184-4
Goller, C. C., and Romeo, T. (2008). ““Environmental influences on biofilm development,” in bacterial biofilms,” in Current topics in microbiology and immunology. Editor T. Romeo (Berlin: Springer), 37–66. doi:10.1007/978-3-540-75418-3_3
Hall-Stoodley, L., Costerton, J. W., and Stoodley, P. (2004). Bacterial biofilms: from the natural environment to infectious diseases. Nat. Rev. Microbiol. 2, 95–108. doi:10.1038/nrmicro821
Hou, J., Veeregowda, D. H., van de Belt-Gritter, B., Busscher, H. J., and van der Mei, H. C. (2018). Extracellular polymeric matrix production and relaxation under fluid shear and mechanical pressure in Staphylococcus aureus biofilms. Appl. Environ. Microbiol. 84, e01516. doi:10.1128/AEM.01516-17
Hou, J., Wang, C., Rozenbaum, R. T., Gusnaniar, N., de Jong, E. D., Woudstra, W., et al. (2019). Bacterial density and biofilm structure determined by optical coherence Tomography. Sci. Rep. 9, 9794. doi:10.1038/s41598-019-46196-7
Huang, Q., Wu, H., Cai, P., Fein, J. B., and Chen, W. (2015). Atomic force microscopy measurements of bacterial adhesion and biofilm formation onto clay-sized particles. Sci. Rep. 5, 16857. doi:10.1038/srep16857
Jara, J., Alarcón, F., Monnappa, A. K., Santos, J. I., Bianco, V., Nie, P., et al. (2021). Self-adaptation of Pseudomonas fluorescens biofilms to hydrodynamic stress. Front. Microbiol. 11, 588884. doi:10.3389/fmicb.2020.588884
Jefferson, K. K. (2004). What drives bacteria to produce a biofilm? FEMS Microbiol. Lett. 236, 163–173. doi:10.1016/j.femsle.2004.06.005
Jerdan, R., Iungin, O., V. Moshynets, O., Potters, G., and Spiers, J. (2020). “Extending an eco-evolutionary understanding of biofilm-formation at the air-liquid interface to community biofilms,” in Bacterial biofilms (London, UK: IntechOpen). doi:10.5772/intechopen.90955
Jha, P. K., Dallagi, H., Richard, E., Deleplace, M., Benezech, T., and Faille, C. (2022). Does the vertical vs horizontal positioning of surfaces affect either biofilm formation on different materials or their resistance to detachment? Food control. 133, 108646. doi:10.1016/j.foodcont.2021.108646
Kampouraki, Z. C., Petala, M., Boumpakis, A., Skordaris, G., Michailidis, N., Deliyanni, E., et al. (2022). Wetting and imbibition characteristics of Pseudomonas fluorescens biofilms grown on stainless steel. Langmuir 38, 9810–9821. doi:10.1021/acs.langmuir.2c00828
Katsipis, G., Avgoulas, D. I., Geromichalos, G. D., Petala, M., and Pantazaki, A. A. (2023). In vitro and in silico evaluation of the serrapeptase effect on biofilm and amyloids of Pseudomonas aeruginosa. Appl. Microbiol. Biotechnol. 107, 7269–7285. doi:10.1007/s00253-023-12772-1
Kędziora, A., Speruda, M., Wernecki, M., Dudek, B., Kapczynska, K., Krzyżewska, E., et al. (2021). How bacteria change after exposure to silver nanoformulations: analysis of the genome and outer membrane proteome. Pathogens 10, 817. doi:10.3390/pathogens10070817
Kimkes, T. E. P., and Heinemann, M. (2019). How bacteria recognise and respond to surface contact. FEMS Microbiol. Rev. 44, 106–122. doi:10.1093/femsre/fuz029
Koza, A., Hallett, P. D., Moon, C. D., and Spiers, A. J. (2009). Characterization of a novel air-liquid interface biofilm of Pseudomonas fluorescens SBW25. Microbiol. (N Y) 155, 1397–1406. doi:10.1099/mic.0.025064-0
Kretschmer, M., Schüßler, C. A., and Lieleg, O. (2021). Biofilm adhesion to surfaces is modulated by biofilm wettability and stiffness. Adv. Mater Interfaces 8. doi:10.1002/admi.202001658
Krsmanovic, M., Biswas, D., Ali, H., Kumar, A., Ghosh, R., and Dickerson, A. K. (2021). Hydrodynamics and surface properties influence biofilm proliferation. Adv. Colloid Interface Sci. 288, 102336. doi:10.1016/j.cis.2020.102336
Kumar Jha, P., Dallagi, H., Richard, E., Benezech, T., and Faille, C. (2020). Formation and resistance to cleaning of biofilms at air-liquid-wall interface. Influence of bacterial strain and material. Food control. 118, 107384. doi:10.1016/j.foodcont.2020.107384
Lade, H., Park, J. H., Chung, S. H., Kim, I. H., Kim, J. M., Joo, H. S., et al. (2019). Biofilm formation by staphylococcus aureus clinical isolates is differentially affected by glucose and sodium chloride supplemented culture media. J. Clin. Med. 8, 1853. doi:10.3390/jcm8111853
Li, W., Buhrow, J. W., Diaz, A. M., Irwin, T. D., Calle, L. M., and Callahan, M. R. (2019). “Effects of surface treatments on stainless steel 316 exposed to potable water containing silver disinfectant,” in 49th International Conference on Environmental Systems ICES-2019-273, Boston, Massachusetts, 7-11 July 2019.
Li, W., Calle, L. M., Hanford, A. J., Stambaugh, I., and Callahan, M. R. (2018). “Investigation of silver biocide as a disinfection Technology for spacecraft-an early literature review,” in International Conference on Environmental Systems, Albuquerque, NM, United States, July 8-12, 2018.
Liu, S., Gunawan, C., Barraud, N., Rice, S. A., Harry, E. J., and Amal, R. (2016). Understanding, monitoring, and controlling biofilm growth in drinking water distribution systems. Environ. Sci. Technol. 50, 8954–8976. doi:10.1021/acs.est.6b00835
Malviya, J., Alameri, A. A., Al-Janabi, S. S., Fawzi, O. F., Azzawi, A. L., Obaid, R. F., et al. (2023). Metabolomic profiling of bacterial biofilm: trends, challenges, and an emerging antibiofilm target. World J. Microbiol. Biotechnol. 39, 212. doi:10.1007/s11274-023-03651-y
Marra, D., Recupido, F., Toscano, G., and Caserta, S. (2022). Bacterial motility in biofilm under shear flow. Chem. Eng. Trans. 93, 325–330. doi:10.3303/CET2293055
Mintsouli, I., Tsiridis, V., Petala, M., Pliatsikas, N., Rebeyre, P., Darakas, E., et al. (2018). Behavior of Ti-6Al-4 V surfaces after exposure to water disinfected with ionic silver. Appl. Surf. Sci. 427, 763–770. doi:10.1016/j.apsusc.2017.08.031
Muirhead, D. L., Button-Denby, A., Smyth, C. M., Nelson, J., and Callahan, M. R. (2020) Chemistry of ionic silver and implications for design of potable water systems.
Nat Microbiol (2022). Expect the unexpected in space microbiology. Nat. Microbiol. 7, 465–466. doi:10.1038/s41564-022-01108-w
Niboucha, N., Goetz, C., Sanschagrin, L., Fontenille, J., Fliss, I., Labrie, S., et al. (2022). Comparative study of different sampling methods of biofilm formed on stainless-steel surfaces in a CDC biofilm reactor. Front. Microbiol. 13, 892181. doi:10.3389/fmicb.2022.892181
Noirot-Gros, M. F., Forrester, S., Malato, G., Larsen, P. E., and Noirot, P. (2019). CRISPR interference to interrogate genes that control biofilm formation in Pseudomonas fluorescens. Sci. Rep. 9, 15954. doi:10.1038/s41598-019-52400-5
O’Rourke, A., Lee, M. D., Nierman, W. C., Craig Everroad, R., and Dupont, C. L. (2020). Genomic and phenotypic characterization of Burkholderia isolates from the potable water system of the International Space Station. PLoS One 15, e0227152. doi:10.1371/journal.pone.0227152
Park, J. H., Shin, B. S., and Jabbarzadeh, A. (2021). Anisotropic wettability on one-dimensional nanopatterned surfaces: the effects of intrinsic surface wettability and morphology. Langmuir 37, 14186–14194. doi:10.1021/acs.langmuir.1c02634
Penesyan, A., Paulsen, I. T., Kjelleberg, S., and Gillings, M. R. (2021). Three faces of biofilms: a microbial lifestyle, a nascent multicellular organism, and an incubator for diversity. NPJ Biofilms Microbiomes 7, 80. doi:10.1038/s41522-021-00251-2
Petala, M., Tsiridis, V., Darakas, E., and Kostoglou, M. (2020). Longevity aspects of potablewater disinfected by ionic silver: kinetic experiments and modeling. WaterSwitzerl. 12, 258. doi:10.3390/w12010258
Petala, M., Tsiridis, V., Darakas, E., Mintsouli, I., Sotiropoulos, S., Kostoglou, M., et al. (2016). “Silver deposition on wetted materials used in the potable water systems of the International Space Station,” in 46th International Conference on Environmental Systems, Vienna, Austria, 1–12.
Petala, M., Tsiridis, V., Mintsouli, I., Pliatsikas, N., Spanos, T., Rebeyre, P., et al. (2017). Silver deposition on stainless steel container surfaces in contact with disinfectant silver aqueous solutions. Appl. Surf. Sci. 396, 1067–1075. doi:10.1016/j.apsusc.2016.11.090
Ping, L., Birkenbeil, J., and Monajembashi, S. (2013). Swimming behavior of the monotrichous bacterium Pseudomonas fluorescens SBW25. FEMS Microbiol. Ecol. 86, 36–44. doi:10.1111/1574-6941.12076
Recupido, F., Petala, M., Caserta, S., Kostoglou, M., Guido, S., and Karapantsios, T. D. (2022). Wetting properties of dehydrated biofilms under different growth conditions. Colloids Surf. B Biointerfaces 210, 112245. doi:10.1016/j.colsurfb.2021.112245
Recupido, F., Petala, M., Caserta, S., Marra, D., Kostoglou, M., and Karapantsios, T. D. (2023). Forced wetting properties of bacteria-laden droplets experiencing initial evaporation. Langmuir 39, 8589–8602. doi:10.1021/acs.langmuir.3c00179
Recupido, F., Toscano, G., Tatè, R., Petala, M., Caserta, S., Karapantsios, T. D., et al. (2020). The role of flow in bacterial biofilm morphology and wetting properties. Colloids Surf. B Biointerfaces 192, 111047. doi:10.1016/j.colsurfb.2020.111047
Reddersen, K., Güllmar, A., Tonndorf-Martini, S., Sigusch, B. W., Ewald, A., Dauben, T. J., et al. (2021). Critical parameters in cultivation of experimental biofilms using the example of Pseudomonas fluorescens. J. Mater Sci. Mater Med. 32, 96. doi:10.1007/s10856-021-06568-w
Rosenberg, M., Azevedo, N. F., and Ivask, A. (2019). Propidium iodide staining underestimates viability of adherent bacterial cells. Sci. Rep. 9, 6483. doi:10.1038/s41598-019-42906-3
Shah, M. D., Kharkar, P. S., Sahu, N. U., Peerzada, Z., and Desai, K. B. (2019). Potassium 2-methoxy-4-vinylphenolate: a novel hit exhibiting quorum-sensing inhibition in: Pseudomonas aeruginosa via LasIR/RhlIR circuitry. RSC Adv. 9, 40228–40239. doi:10.1039/c9ra06612h
Soldano, A., Yao, H., Chandler, J. R., and Rivera, M. (2020). Inhibiting iron mobilization from bacterioferritin in Pseudomonas aeruginosa impairs biofilm formation irrespective of environmental iron availability. ACS Infect. Dis. 6, 447–458. doi:10.1021/acsinfecdis.9b00398
Song, B., and Leff, L. G. (2005). Identification and characterization of bacterial isolates from the Mir space station. Microbiol. Res. 160, 111–117. doi:10.1016/j.micres.2004.10.005
Tardelli, J. D. C., Bagnato, V. S., and Reis, A. C. dos (2023). Bacterial adhesion strength on titanium surfaces quantified by atomic force microscopy: a systematic review. Antibiotics 12, 994. doi:10.3390/antibiotics12060994
Tsagkari, E., and Sloan, W. T. (2018). Turbulence accelerates the growth of drinking water biofilms. Bioprocess Biosyst. Eng. 41, 757–770. doi:10.1007/s00449-018-1909-0
Tuson, H. H., and Weibel, D. B. (2013). Bacteria-surface interactions. Soft Matter 9, 4368–4380. doi:10.1039/c3sm27705d
van Hoogstraten, S. W. G., Kuik, C., Arts, J. J. C., and Cillero-Pastor, B. (2023). Molecular imaging of bacterial biofilms—a systematic review. Crit. Rev. Microbiol., 1–22. doi:10.1080/1040841X.2023.2223704
Vélez Justiniano, Y. A., Goeres, D. M., Sandvik, E. L., Kjellerup, B. V., Sysoeva, T. A., Harris, J. S., et al. (2023). Mitigation and use of biofilms in space for the benefit of human space exploration. Biofilm 5, 100102. doi:10.1016/j.bioflm.2022.100102
Wang, L., Wong, Y. C., Correira, J. M., Wancura, M., Geiger, C. J., Webster, S. S., et al. (2023a). The accumulation and growth of Pseudomonas aeruginosa on surfaces is modulated by surface mechanics via cyclic-di-GMP signaling. NPJ Biofilms Microbiomes 9, 78. doi:10.1038/s41522-023-00436-x
Wang, S., Yu, P., Li, X., Zhao, Z., Dong, Y., and Li, X. (2023b). Design and fabrication of functional hydrogels with specific surface wettability. Colloids Interface Sci. Commun. 52, 100697. doi:10.1016/j.colcom.2023.100697
Wassmann, T., Kreis, S., Behr, M., and Buergers, R. (2017). The influence of surface texture and wettability on initial bacterial adhesion on titanium and zirconium oxide dental implants. Int. J. Implant Dent. 3, 32. doi:10.1186/s40729-017-0093-3
Wu, R. X., Zhang, Y., Guo, Z. Q., Zhao, B., and Guo, J. S. (2022). Role of Ca2+ and Mg2+ in changing biofilm structure and enhancing biofilm formation of P. stutzeri strain XL-2. Colloids Surf. B Biointerfaces 220, 112972. doi:10.1016/j.colsurfb.2022.112972
Wu, S., Altenried, S., Zogg, A., Zuber, F., Maniura-Weber, K., and Ren, Q. (2018). Role of the surface nanoscale roughness of stainless steel on bacterial adhesion and microcolony formation. ACS Omega 3, 6456–6464. doi:10.1021/acsomega.8b00769
Yang, J., Barrila, J., Mark Ott, C., King, O., Bruce, R., McLean, R. J. C., et al. (2021). Longitudinal characterization of multispecies microbial populations recovered from spaceflight potable water. NPJ Biofilms Microbiomes 7, 70. doi:10.1038/s41522-021-00240-5
Zhao, A., Sun, J., and Liu, Y. (2023). Understanding bacterial biofilms: from definition to treatment strategies. Front. Cell Infect. Microbiol. 13, 1137947. doi:10.3389/fcimb.2023.1137947
Keywords: biofilm, space mission, water system, Pseudomonas fluorescens, stainless steel, titanium, electropolishing, silver deposition
Citation: Avgoulas DI, Petala M, Briandet R, Dergham Y, Noirot-Gros M-F, Konstantinidis A, Kostoglou M and Karapantsios TD (2024) Effect of surface treatment and shear flow on biofilm formation over materials employed in space water storage and distribution systems. Front. Mater. 11:1401764. doi: 10.3389/fmats.2024.1401764
Received: 15 March 2024; Accepted: 13 May 2024;
Published: 30 May 2024.
Edited by:
Malgorzata Holynska, European Space Research and Technology Centre (ESTEC), NetherlandsReviewed by:
Hassan Latrache, Université Sultan Moulay Slimane, MoroccoSergio Caserta, University of Naples Federico II, Italy
Copyright © 2024 Avgoulas, Petala, Briandet, Dergham, Noirot-Gros, Konstantinidis, Kostoglou and Karapantsios. This is an open-access article distributed under the terms of the Creative Commons Attribution License (CC BY). The use, distribution or reproduction in other forums is permitted, provided the original author(s) and the copyright owner(s) are credited and that the original publication in this journal is cited, in accordance with accepted academic practice. No use, distribution or reproduction is permitted which does not comply with these terms.
*Correspondence: Thodoris D. Karapantsios, a2FyYXBhbnRAY2hlbS5hdXRoLmdy