- 1Department of Microbiology, Lovely Professional University, Phagwara, Punjab, India
- 2Department of Medical Laboratory Sciences, School of Allied and Healthcare Sciences, GNA University, Phagwara, Punjab, India
- 3Department of Biological Sciences, Al Hussein Bin Talal University Ma’an, Ma’an, Jordan
- 4Deaprtment of Microbiology, Graphic Era (Deemed to be University), Dehradun, Uttarakhand, India
- 5Department of Biomedical Sciences, Institute of Health, Jimma University, Jimma, Ethiopia
Introduction: Microbial-induced calcite precipitation (MICP) involves various microorganisms, such as bacteria, fungi, and algae. This study focuses on producing bio-cement using fungal species and selecting potential candidates isolated from alkaline soil of different regions of Punjab, namely, Majha, Malwa, and Doaba.
Methods: The selection of fungi isolates capable of bio-cement production involves several tests, including a urease assay and calcium precipitation. Isolates having high urease enzyme production and the ability to perform calcite precipitation are selected for instrumental analyses such as X-ray diffraction (XRD) and scanning electron microscopy (SEM). The isolates selected for further analysis are S1 (3) with 8.879 ± 2.94 µg/ml, S1 (18) with 8.421 ± 0.13 µg/ml, and S4 (1) with 10.057 ± 0.45 µg/ml urease activity and least free calcium ions that are 2.337 ± 0.5 µg/ml, 3.339 ± 0.5 µg/ml, and 4.074 ± 0.1 µg/ml respectively.
Results and discussion: Calcite precipitation is confirmed through XRD and field emission scanning electron microscopy (FESEM). XRD images showing calcite precipitation with sharp crystalline peaks for S1 (3), S1 (18), and S4 (1) are shown. The calcite precipitation is evident in the micrographs of FESEM. These combined results confirm the potential of urease-positive fungi to facilitate calcite production, which could lead to bio-cement development in future research.
Highlights
• Exploring fungal-driven MICP for bio-cement production.
• Sample collection from Majha, Malwa, and Doaba regions.
• Selection of fungi through urease assay and calcium precipitation tests.
• Isolates S1 (3), S1 (18), and S4 (1) showed high urease activity and low free calcium ions.
• X-ray diffraction (XRD) and scanning electron microscopy (SEM) confirmed calcite precipitation.
1 Introduction
Microorganisms have been utilized in a variety of industries, including food, medicine, and cosmetics (Rajput et al., 2022). The global construction industry is looking for eco-friendly alternatives to traditional cement production due to concerns over its high carbon footprint and impact on greenhouse gas emissions (Huntzinger and Eatmon, 2009). Cement manufacture requires high temperatures, usually between 1,400°C–1,500°C, which leads to the combustion of fossil fuels and the release of hazardous gases such as sulfur dioxide, carbon dioxide, and carbon monoxide (Tosti, 2021). The industrial process of cement production from lime, which is a precursor of concrete, consumes 2%–3% of the world’s energy demand. This process generates 0.73–0.99 tons of CO2 per ton of cement produced, contributing to approximately 8%–10% of global anthropogenic CO2 emissions and 3.4% of total global CO2 emissions (dos Santos et al., 2021; Zhao et al., 2022). The rise in concrete consumption is due to infrastructures being vulnerable to various physical, chemical, and biological factors like temperature changes, exposure to corrosive and radioactive materials, aggressive gases, natural calamities, and microbial processes (Ye et al., 2023; Lin et al., 2023; Zhang et al., 2024). Conventional cement is susceptible to early-age cracking from environmental conditions, a problem that can be reduced by using bio-cement, which has self-healing capabilities (Safiuddin et al., 2018; Zhang et al., 2024). The rise of bio-cement manufacturing using urease-positive fungi offers a hopeful and sustainable alternative in this context.
Microbially induced carbonate precipitation (MICP) is a natural biochemical process where microorganisms trigger the creation of calcium carbonate precipitation. Microorganisms initiate carbonate precipitation by utilizing different metabolic processes such as photosynthesis, ureolysis, ammonification, denitrification, sulfate reduction, anaerobic sulfide oxidation, and methane oxidation (Castro-Alonso et al., 2019). The routes include mechanisms that elevate pH levels and enhance the concentration of dissolved inorganic carbon (DIC) (Martuscelli et al., 2020; Devgon et al., 2024). Bio-cementation procedures utilize microbially induced calcium carbonate precipitation (MICP) to solidify sand by generating calcium carbonate, leading to varied desired consequences. Calcium carbonate precipitates to create the bonding substance that holds particles together. The primary metabolic pathway of interest is urea hydrolysis, which is commonly utilized in significant research investigations (Tosti, 2021). The process consists of three clear stages: (i) introducing microbes into the medium, (ii) injecting a cementation solution with urea and a calcium source, and (iii) causing the cementing agent to precipitate, binding the sand particles and enhancing strength and stiffness (Konstantinou and Wang, 2023).
The research gap in the literature is highlighted by the limited exploration of fungal species for microbial-induced calcite precipitation (MICP) compared to the extensive focus on bacteria. Additionally, there is a lack of detailed understanding of fungal mechanisms for calcite production and their practical application in bio-cement. This work fills these gaps by investigating fungal candidates and providing new insights into their potential for sustainable bio-cement development.
Urease-positive fungi can generate urease enzymes, which are essential for converting urea into carbonate ions and ammonia (Fang et al., 2018; Konstantinou and Wang, 2023; Li et al., 2023). This enzymatic activity can be utilized to stimulate mineral precipitation, specifically the creation of calcium carbonate, an essential element in bio-cement manufacturing (Iqbal et al., 2021). Utilizing urease-positive fungi for bio-cement addresses environmental issues of traditional methods and provides a sustainable and eco-friendly alternative for construction while also tackling early-age cracking problems in regular cement (Lin et al., 2023).
This study pioneers using fungal species, rather than bacteria, for MICP in bio-cement production. Exploring urease-positive fungi and their involvement in bio-cement is crucial for comprehending their significant influence on construction methods and environmental preservation when selecting candidates for microbial-induced calcite precipitation. Isolating fungi from alkaline soils and confirming their calcite precipitation through XRD and field emission scanning electron microscopy (FESEM) expands the scope of microorganisms in bio-cement research (Figure 1). The research highlights fungi as promising candidates for sustainable bio-cement production, offering an alternative to bacteria with potential benefits like enhanced environmental tolerance. This opens new avenues for eco-friendly construction materials and future biotechnological applications.
2 Materials and methods
2.1 Isolation
The alkaline soil samples are collected from specific locations in the Punjab region: Phagwara (latitude 31.249168°, longitude 75.709499°), Moga (latitude 30.966159°, longitude 75.481656°), Muktsar (latitude 30.49625°, longitude 74.571997°) and Amritsar (latitude 31.499645, longitude 75.321991°). The soil samples are collected in a sterile polybag and stored at 4°C (Chaudhary et al., 2013). For isolation, 1 g of soil is inoculated in 10 mL autoclaved broth for 24 h at 27°C. Afterward, serial dilution is performed, and 100 µL of sample is used from both 10−5 and 10−6 tubes to spread aseptically on prepared potato dextrose agar plates containing chloramphenicol (antibacterial agent) to avoid bacterial contamination. Hyphae tips freshly grown in potato dextrose agar (PDA) plates are used to obtain pure isolates on freshly prepared autoclaved PDA plates (Acharya and Hare, 2022). All pure culture slants are prepared in slant tubes using PDA media and are stored at 4°C, and glycerol stocks are stored at −80°C (Kitamoto et al., 2002).
2.2 Urease assay
2.2.1 Qualitative urease test
A method is employed to assess the fungi’s potential to induce calcite precipitation through urease enzyme activity. Autoclaved Christensen’s agar medium and urea broths are prepared to isolate urease-positive strains. Urease activity is observed by observing a distinct color shift (FAEZI et al., 2004), specifically from yellow to pink, which serves as a positive indication. This procedural method is utilized to selectively identify fungal isolates proficient in urease enzyme production, paving the way for subsequent investigations into their calcite precipitation capabilities (Zhao et al., 2022).
2.2.2 Quantitative urease assay
A quantitative urease assay is performed to calculate the amount of urease enzyme produced in µg/mL by different isolates. The chemicals used for the assay are given below in Table 1.
Following the preparation of autoclaved media, isolates are inoculated using a cork borer for a 7-day incubation period at 30°C. After this duration, mycelia are homogenized with a homogenizer and filtered through Whatman filter paper. The mycelia obtained on the filter paper are washed using 1 M potassium phosphate buffer, followed by a wash with 0.1 M potassium phosphate buffer at pH 7.0. The homogenized mycelia suspension is then employed for the urease assay.
For the urease assay, 100 µL of the sample is mixed with 500 µL of mM urea and 500 µL of 100 mM potassium phosphate buffer at pH 8, resulting in a total volume (1:1 ratio). A 50 μL aliquot of the reaction mixture is transferred to a tube containing 500 µL of phenol-nitroprusside solution and 500 µL of alkaline hypochlorite. The tube is kept at room temperature at 30°C. Absorbance at 630 nm is measured, and the values are calculated using a standard ammonium sulfate graph. This detailed procedure ensures the accurate quantification of urease activity in the analyzed samples.
2.3 Calcium precipitation assay
Examining calcium precipitation entails titration with EDTA, Eriochrome black T dye, sodium hydroxide buffer, and HCL. Adjusting the solution’s pH to around 10 with sodium hydroxide buffer prompts the formation of calcium ions as Ca(OH)2, facilitating their interaction with EDTA. The solution is titrated using a 0.01 M EDTA solution until the color transitions from pink to blue, indicating the completion of the calcium ion reaction with EDTA. This titration is done in triplicate for precision, and the average volume of EDTA used is determined (Akoijam et al., 2021). Subsequently, the calcium content (mg) in the original sample is computed using the EDTA volume, the known concentration of EDTA, and the following formula:
Calculation of free calcium ions is calculated using the following formula:
1 mL EDTA × molarity EDTA = mmoles EDTA = mmoles Ca2+ × 40.078 g/mol = mmoles Ca2+,
aliquot 2(Ca2+ mg, aliquot) × (50.00 mL 250.00 mL) = Ca2+ mg, unknown.
The growth media to perform the calcium precipitation is given below in Table 2.
2.4 Instrumentation analysis
2.4.1 X-ray diffraction (XRD)
XRD is used to ascertain the chemical composition of the precipitation resulting from bacterial mineralization, as detailed by Fang et al. (2018). The crystal structure of the bio-cement formed by microorganisms is elucidated through XRD, following the methodology outlined by Anitha et al. (2018). To investigate the presence of calcium carbonate on the hyphae of ureolytic fungi, a diffractometer equipped with a Bruker D8 Advance is employed for the structural analysis of the fungus hyphae. The analysis is performed after a 7-day incubation period to observe any discernible calcium carbonate presence on the hyphae.
2.4.2 Field emission scanning electron microscopy (FESEM)
Field emission scanning electron microscopy (FESEM) is employed to examine the bio-cement generated by fungal isolates, as described by Fang et al. (2018). The visualization using FESEM allows for a detailed observation of the structure.
3 Results and discussion
3.1 Isolation
In microbial-induced calcite precipitation, the selected fungal strain must be urease-positive, as this research focuses on the production of calcite through urea hydrolysis. Of the different fungi isolated from the soil sample, 21 are urease-positive (Li et al., 2015).
3.2 Screening potential candidates based on urease assay and calcium precipitation
3.2.1 Qualitative urease assay
After inoculation in Christensen’s medium, a color change is observed to select urease-positive fungi. The color shifts from yellow to pink due to the hydrolysis of urea by the enzyme urease, which is produced by the fungus. Urease catalyzes the breakdown of urea into ammonia and carbon dioxide. The ammonia produced increases the pH of the medium, shifting it from an acidic to an alkaline environment. The urea broth contains the pH indicator phenol red, which is yellow under acidic conditions and turns pink in alkaline conditions, indicating a positive result (Martuscelli et al., 2020; Zhang et al., 2024). Of all the isolates, 21 are urease-positive, and a quantitative urease assay was performed to identify the highest producers of the urease enzyme.
3.2.2 Quantitative urease assay
After inoculation of isolates for 7 days at 30°C using a standard ammonia graph, concentrations in µg/mL were calculated as shown in Table 3 for all the isolates. An ammonia standard graph, or standard curve, is used to determine the amount of urease activity in a sample by plotting the values from ammonium chloride standards. It is observed that S1 (3), S1 (18), S4 (1), S4 (9), S6 (8), S6 (9), and F are the maximum producers of urease enzyme.
3.3 Calcium precipitation/cementation assay
The fungal isolate must be able to precipitate calcium for the MICP process. High urease enzyme producers will precipitate more calcium. After performing the urease assay, the high urease producers are further inoculated in growth media containing a calcium source and urea and incubated for 7 days at 28°C. It is observed that S1 (3), S1 (18), S4 (1), S1 (28), S2 (7), and S2 (8) showed maximum removal of free calcium ions in the solution with the lowest number of free calcium ions as shown in Table 4 (Figure 2). Based on the results of both the urease assay and the calcium precipitation assay, S1 (3), S1 (18), and S4 (1) are identified as isolates having higher urease enzyme production that can precipitate calcium and are selected for instrumentational analysis (Akoijam et al., 2021).
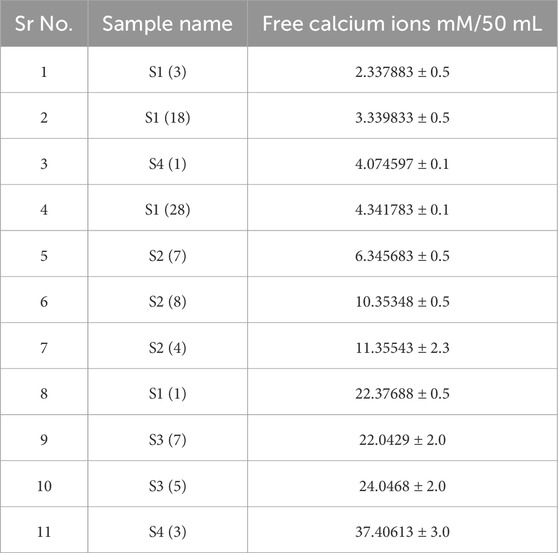
Table 4. Free calcium ion concentrations after inoculation of urease-positive fungi and incubation at 30°C for 7 days. When fewer free calcium ions are present, more calcite precipitation has occurred.
3.4 Instrumentation analysis
3.4.1 XRD analysis of calcite precipitation
The distinct peaks of the graphical representation of various fungal isolates strongly indicate the ability of S1 (3), S1 (18), and S4 (1) f to promote calcite precipitation. Specifically, the sharp peak near 29.3° for 2θ provides valuable insights into the crystallinity of calcite (calcium carbonate).
Specific 2θ values observed for S13 (29.3, 39.95, 39.3, 43.1, 47, 48, 56.5, 57.3), S41 (29.3, 31.4, 23, 47), and S1 (18) (29, 35.9, 39, 43.1, 47, 48.4, 56, 57.3) shown in Figure 3. These findings underscore the role of these fungal isolates in promoting the precipitation of calcium carbonate on their hyphae. Crystalline size is calculated and shown in Table 5.
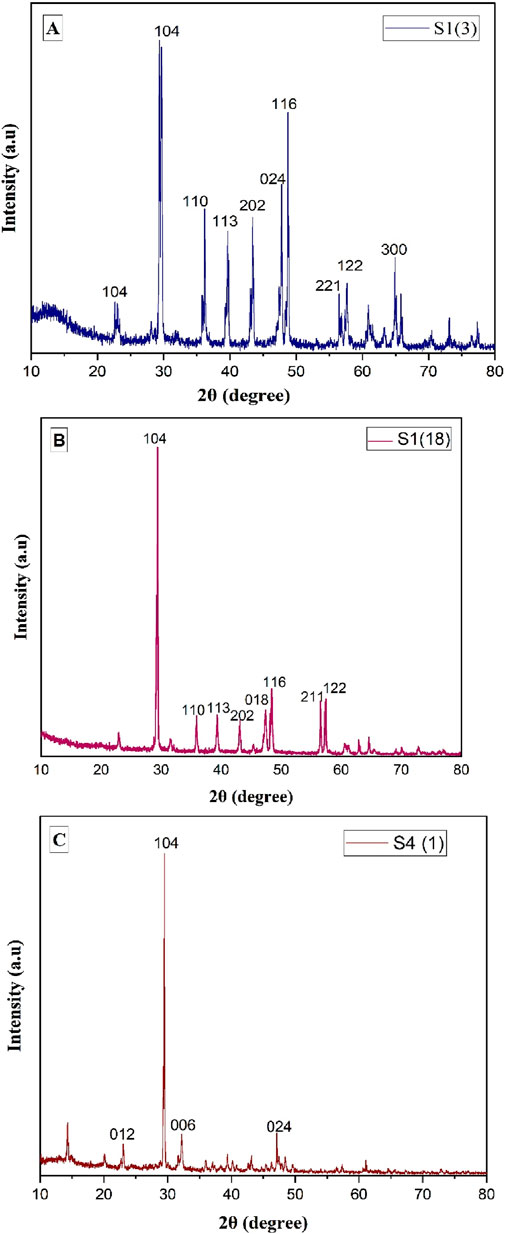
Figure 3. XRD graph of calcium precipitation for isolates (A) S1 (3), (B) S4 (1), and (C) S18. The peak corresponding to 104 indicates calcium carbonate.
3.4.2 SEM analysis of calcite precipitation
Figures 3–6 depict the SEM analysis of calcite precipitation, revealing the presence of larger mineral crystals within fungal isolates S1 (13), S1 (18), and S4 (1). These crystals serve as tangible evidence of the active involvement of fungal isolates in the formation of calcite precipitation (Burford et al., 2006). The localized accumulation of calcite near fungal hyphae suggests the existence of nucleation sites for mineralization (Fomina et al., 2006; Ye et al., 2023). Further confirmation of calcite presence was obtained through energy dispersive spectroscopy (EDS), which identified the elements Ca, C, and O, closely resembling the atomic composition of CaCO3.
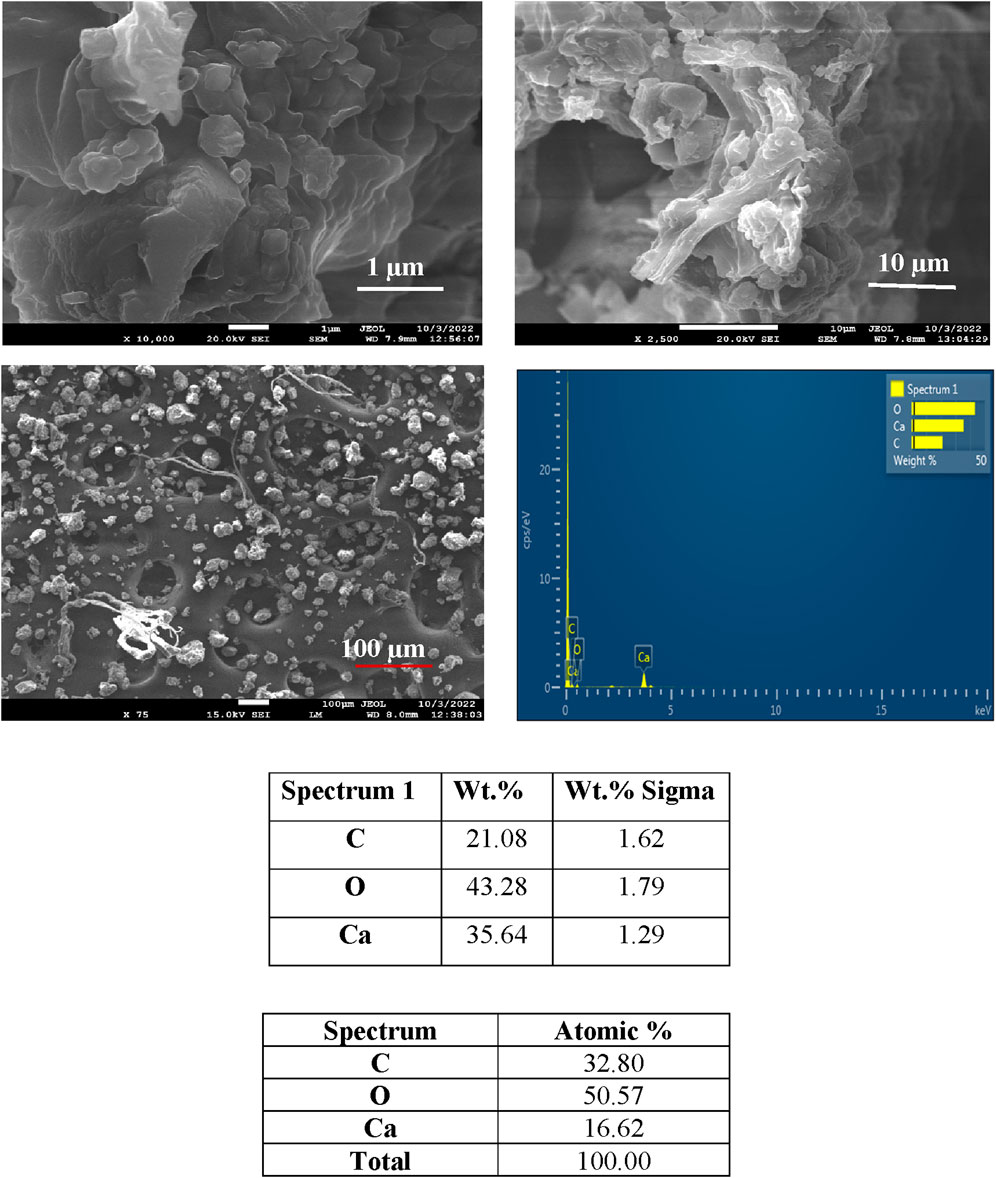
Figure 4. SEM and EDS spectra of calcite precipitation in fungal isolate S1 (3), showing deposited crystals.
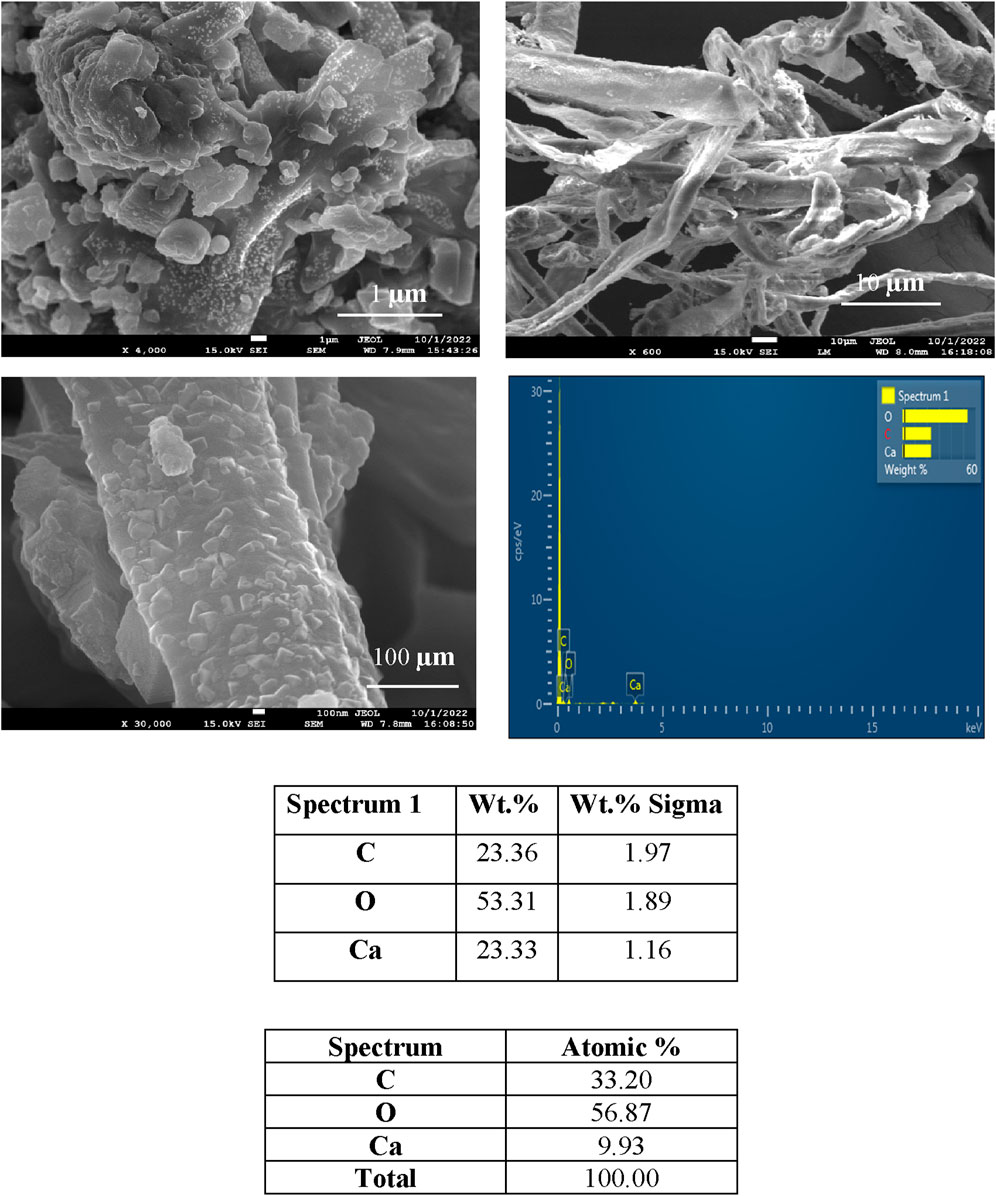
Figure 5. SEM and EDS spectra of calcite precipitation in fungal isolate S (18), showing deposited crystals.
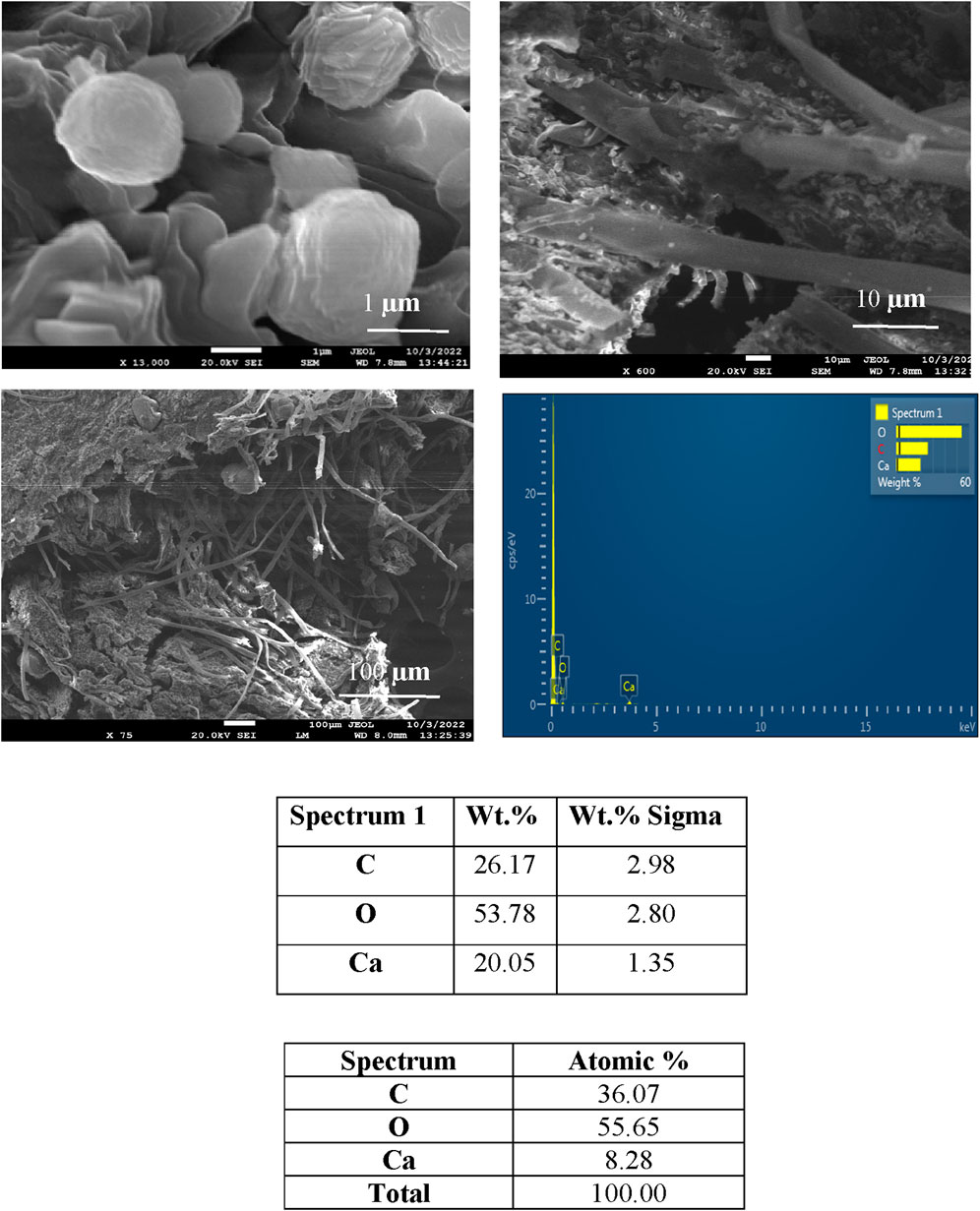
Figure 6. SEM and EDS spectra of calcite precipitation in fungal isolate S4 (1), showing deposited crystals.
4 Conclusion
This work emphasizes the promising ability of fungi to produce cement through microbially induced calcite precipitation (MICP). Although the traditional focus of MICP research has been on bacteria, our study emphasizes the unique advantages of fungi. They are promising candidates for boosting calcite precipitation owing to their abundance of nucleation sites and ability to survive for a prolonged time as spores. We isolated urease-positive fungi from different areas of Punjab, including Majha, Malwa, and Doaba. We applied a systematic selection process to discover key isolates, S1 (3), S1 (18), and S4 (1), that revealed noteworthy calcite precipitation capabilities. Comprehensive testing, including assays for calcium precipitate and instrumental evaluations with XRD and scanning electron microscopy (SEM), are used to confirm the evidence of calcite precipitation. Notably, isolates S1 (3), S1 (18), and S4 (1) displayed significant calcium precipitation. Conclusively, these outcomes highlight that the selected urease-positive fungi can produce calcite precipitation.
Data availability statement
The original contributions presented in the study are included in the article/supplementary material; further inquiries can be directed to the corresponding authors.
Author contributions
ID: Formal Analysis, Investigation, Methodology, Resources, Visualization, Writing–original draft. RK: Data curation, Formal Analysis, Methodology, Software, Validation, Writing–original draft. KR.: Data curation, Formal Analysis, Investigation, Validation, Writing–original draft. MK: Data curation, Methodology, Resources, Writing–original draft. AM: Data curation, Formal Analysis, Validation, Writing–original draft. AK: Conceptualization, Formal Analysis, Resources, Supervision, Writing–original draft, Writing–review & editing. TM: Data curation, Formal Analysis, Resources, Visualization, Writing–review & editing.
Funding
The author(s) declare that no financial support was received for the research, authorship, and/or publication of this article. No funds or grants are received.
Acknowledgments
The author is obliged to Lovely Professional University and CIF of LPU, Phagwara, to help them conduct their research.
Conflict of interest
The authors declare that the research was conducted in the absence of any commercial or financial relationships that could be construed as a potential conflict of interest.
Publisher’s note
All claims expressed in this article are solely those of the authors and do not necessarily represent those of their affiliated organizations, or those of the publisher, the editors, and the reviewers. Any product that may be evaluated in this article, or claim that may be made by its manufacturer, is not guaranteed or endorsed by the publisher.
References
Acharya, T., and Hare, J. (2022). “Sabouraud agar and other fungal growth media,” in Laboratory protocols in fungal biology: current methods in fungal biology (Cham: Springer International Publishing), 69–86.
Akoijam, N., Dutta, S., and Joshi, S. R. (2021). Biomineralization potential of a ureolytic fungus isolated from mawsmai cave in Meghalaya.
Anitha, V., Abinaya, K., Prakash, S., Seshagiri Rao, A., and Vanavil, B. (2018). Bacillus cereus KLUVAA mediated biocement production using hard water and urea. Chem. Biochem. Eng. Q. 32 (2), 257–266. doi:10.15255/cabeq.2017.1096
Burford, E. P., Hillier, S., and Gadd, G. M. (2006). Biomineralization of fungal hyphae with calcite (CaCO3) and calcium oxalate mono-and dihydrate in carboniferous limestone microcosms. Geomicrobiol. J. 23 (8), 599–611. doi:10.1080/01490450600964375
Castro-Alonso, M. J., Montañez-Hernandez, L. E., Sanchez-Muñoz, M. A., Macias Franco, M. R., Narayanasamy, R., and Balagurusamy, N. (2019). Microbially induced calcium carbonate precipitation (MICP) and its potential in bioconcrete: microbiological and molecular concepts. Front. Mater. 6, 126. doi:10.3389/fmats.2019.00126
Chaudhary, H. S., Yadav, J., Shrivastava, A. R., Singh, S., Singh, A. K., and Gopalan, N. (2013). Antibacterial activity of actinomycetes isolated from different soil samples of Sheopur (A city of central India). J. Adv. Pharm. Technol. and Res. 4 (2), 118. doi:10.4103/2231-4040.111528
Devgon, I., Sachan, R. S. K., Devgon, J., and Karnwal, A. (2024). Bio-cement: a sustainable approach in the construction sector. AIP Conf. Proc. 2986 (1), 030046. doi:10.1063/5.0194118
Dos Santos, V. H. J. M., Pontin, D., Ponzi, G. G. D., e Stepanha, A. S. D. G., Martel, R. B., Schütz, M. K., et al. (2021). Application of Fourier Transform infrared spectroscopy (FTIR) coupled with multivariate regression for calcium carbonate (CaCO3) quantification in cement. Constr. Build. Mater. 313, 125413. doi:10.1016/j.conbuildmat.2021.125413
Faezi, G. M., Bakhtiari, M. R., Falahpour, M., Nouhi, A. A., Moazami, N., and Amidi, Z. (2004). Screening of urease production by Aspergillus Niger strains.
Fang, C., Kumari, D., Zhu, X., and Achal, V. (2018). Role of fungal-mediated mineralization in biocementation of sand and its improved compressive strength. Int. Biodeterior. and Biodegrad. 133, 216–220. doi:10.1016/j.ibiod.2018.07.013
Fomina, M., Burford, E. P., and Gadd, G. M. (2006). “Fungal dissolution and transformation of minerals: significance for nutrient and metal mobility,” in Fungi in biogeochemical cycles (Cambridge: Cambridge University Press), 236–266. doi:10.1017/cbo9780511550522.01110
Garg, R., Garg, R., and Eddy, N. O. (2023). Microbial induced calcite precipitation for self-healing of concrete: a review. J. Sustain. Cement-Based Mater. 12 (3), 317–330. doi:10.1080/21650373.2022.2054477
Huntzinger, D. N., and Eatmon, T. D. (2009). A life-cycle assessment of Portland cement manufacturing: comparing the traditional process with alternative technologies. J. Clean. Prod. 17 (7), 668–675. doi:10.1016/j.jclepro.2008.04.007
Iqbal, D. M., Wong, L. S., and Kong, S. Y. (2021). Bio-cementation in construction materials: a review. Materials 14 (9), 2175. doi:10.3390/ma14092175
Kitamoto, Y., Suzuki, A., Shimada, S., and Yamanaka, K. (2002). A new method for the preservation of fungus stock cultures by deep-freezing. Mycoscience 43, 0143–0149. doi:10.1007/s102670200021
Konstantinou, C., and Wang, Y. (2023). Unlocking the potential of microbially induced calcium carbonate precipitation (MICP) for hydrological applications: a review of opportunities, challenges, and environmental considerations. Hydrology 10 (9), 178. doi:10.3390/hydrology10090178
Li, J., Bi, W., Yao, Y., and Liu, Z. (2023). State-of-the-Art review of utilization of microbial-induced calcite precipitation for improving moisture-dependent properties of unsaturated soils. Appl. Sci. 13 (4), 2502. doi:10.3390/app13042502
Li, Q., Csetenyi, L., Paton, G. I., and Gadd, G. M. (2015). C a CO 3 and S r CO 3 bioprecipitation by fungi isolated from calcareous soil. Environ. Microbiol. 17 (8), 3082–3097. doi:10.1111/1462-2920.12954
Lin, H., Zhou, M., Li, B., and Dong, Y. (2023). Mechanisms, application advances and future perspectives of microbial-induced heavy metal precipitation: a review. Int. Biodeterior. and Biodegrad. 178, 105544. doi:10.1016/j.ibiod.2022.105544
Martuscelli, C., Soares, C., Camões, A., and Lima, N. (2020). Potential of fungi for concrete repair. Procedia Manuf. 46, 180–185. doi:10.1016/j.promfg.2020.03.027
Menon, R. R., Luo, J., Chen, X., Zhou, H., Liu, Z., Zhou, G., et al. (2019). Screening of fungi for potential application of self-healing concrete. Sci. Rep. 9, 2075. doi:10.1038/s41598-019-39156-8
Qian, X., Fang, C., Huang, M., and Achal, V. (2017). Characterization of fungal-mediated carbonate precipitation in the biomineralization of chromate and lead from an aqueous solution and soil. J. Clean. Prod. 164, 198–208. doi:10.1016/j.jclepro.2017.06.195
Rajput, K., Dohroo, A., Devgon, I., and Karnwal, A. (2022). Role of plant-derived prebiotic in modulation of human gut microflora: a review. Iran. J. Med. Microbiol. 16 (5), 368–375. doi:10.30699/ijmm.16.5.368
Safiuddin, M., Kaish, A. A., Woon, C. O., and Raman, S. N. (2018). Early-age cracking in concrete: causes, consequences, remedial measures, and recommendations. Appl. Sci. 8 (10), 1730. doi:10.3390/app8101730
Tosti, L. (2021). in Doctoral dissertation (Wageningen University, Nederlands: Wageningen University and Research). Improving circularity of biomass ash: a theoretical, experimental, and modelling approach to find sustainable solutions for application in cement or soil.
Ye, P., Xiao, F., and Wei, S. (2023). Biomineralization and characterization of calcite and vaterite induced by the fungus cladosporium sp. YPLJS-14. Minerals 13 (10), 1344. doi:10.3390/min13101344
Zhang, Y. S., Liu, Y., Sun, X. D., Zeng, W., Xing, H. P., Lin, J. Z., et al. (2024). Application of microbially induced calcium carbonate precipitation (MICP) technique in concrete crack repair: a review. Constr. Build. Mater. 411, 134313. doi:10.1016/j.conbuildmat.2023.134313
Keywords: fungal calcite precipitation, urease-positive fungi, bio-cement production, environmental mineralization, fungal bio-mineralization
Citation: Devgon I, Kumar Sachan RS, Rajput K, Kumar M, Mohammad Said Al-Tawaha AR, Karnwal A and Malik T (2024) Exploring fungal potential for microbial-induced calcite precipitation (MICP) in bio-cement production. Front. Mater. 11:1396081. doi: 10.3389/fmats.2024.1396081
Received: 05 March 2024; Accepted: 04 October 2024;
Published: 28 October 2024.
Edited by:
Arun Prabhu Rameshbabu, Harvard Medical School, United StatesReviewed by:
Sharanabasava V. Ganachari, KLE Technological University, IndiaRajesh kannan Rajan, Annamalai University, India
Copyright © 2024 Devgon, Kumar Sachan, Rajput, Kumar, Mohammad Said Al-Tawaha, Karnwal and Malik. This is an open-access article distributed under the terms of the Creative Commons Attribution License (CC BY). The use, distribution or reproduction in other forums is permitted, provided the original author(s) and the copyright owner(s) are credited and that the original publication in this journal is cited, in accordance with accepted academic practice. No use, distribution or reproduction is permitted which does not comply with these terms.
*Correspondence: Arun Karnwal, YXJ1bmthcm53YWxAZ21haWwuY29t; Tabarak Malik, bWFsaWtpdHJjQGdtYWlsLmNvbQ==