- 1School of Transportation and Geomatics Engineering, Shenyang Jian Zhu University, Shenyang, China
- 2National Engineering Research Center of Highway Maintenance Technology, Changsha University of Science and Technology, Changsha, China
- 3School of Civil Engineering, Shenyang Urban Construction University, Shenyang, China
- 4Liaoning New Development Highway Technology Maintenance Co. LTD, Shenyang, China
- 5Liaoning Datong Highway Engineering Co. LTD, Shenyang, China
- 6Orient Science and Technology College of Hunan Agricultural University, Changsha, China
The bonding performance between new and old concrete affects the reliability and service life of the repair structure. However, the bonding interface is complex and has many influencing factors, so selecting appropriate repair materials and evaluation methods for repair structures under different service environments is important. This paper presents a comprehensive overview of the factors that influence bonding properties. These include the choice of repair materials, the condition of the existing concrete, the type of interfacial agents used, the service environment, and the testing methods employed. The paper concludes by examining the challenges and opportunities in developing interface bonding properties to provide insights and research directions for future theoretical analysis and experimental research.
1 Introduction
Concrete structures are widely used nowadays. The existing concrete structures show different degrees of damage due to service environments and loading factors. Repairing structures to maintain structure integrity and improve their bearing capacity and durability is necessary. One significant challenge in repairing structures is ensuring the bonding performance between new and old concrete (LIU, 2006; ZHENG and CHEN, 2008). This issue arises in various new structures, including dams, prefabricated component nodes, and fabricated buildings, high-speed railway track slabs and filling layers, and interfaces between prestressed pipe grouting materials and channels (TAYEH et al., 2012; KOTHARI et al., 2020; VALIKHANI et al., 2020). The structure of new and old concrete is depicted in Figure 1, comprising old concrete, repair material, interfaces between old concrete and repair material, and interfacial agents. Multiple factors impact bonding performance, including the types of repair material, the strength of old concrete, roughness, the water content of the interface, and types of interfacial agents. High temperatures, varying humidity, freeze-thaw cycles, and chemical corrosion impact bond performance during the service life. The evolution of bond performance further impacts serviceability and service life, making it crucial to uncover the critical factors affecting bonding performance.
Researchers have achieved many results in bonding properties between old and new concrete. This paper analyzes the influences of repair materials, interface agents, environmental factors, and test methods based on current research results. Moreover, this paper looks forward to future research that provides a theoretical and technical basis for related engineering and technology research.
2 Effect of different repair materials on bond strength
Repair materials affect early strength development and durability of repair structures, so suitable repair material is a prerequisite to ensuring molecular force between concrete (ZHENG and CHEN, 2008). Research showed that the types of repair materials influence bond properties (LIU, 2006). Common repair materials include ultra-high-performance concrete, self-compacting concrete, mortar, and fiber-reinforced material.
2.1 Ultra-high performance concrete
Compared with ordinary concrete, Ultra-high performance concrete (UHPC) has an advantage in strength, ductility, workability, and durability. This paper analyzed the effects of silica fume, fiber, and water-cement ratios on bond strength.
Silica fume is one of the cementitious components in UHPC. Research showed that the filling and pozzolanic effects can improve interfacial transition zone and bond strength (TAYEH et al., 2012). Qiao et al. found that bond strength increased and decreased with silica fume increase. Compared with a silica fume mass fraction of 3%, the 9% mass fraction shear strength and splitting tensile strength increased 39.5% and 30.6%, respectively. However, when it reached 12%, bond strength decreased. The reason is that the reaction of silica fumes with calcium hydroxide crystals on old concrete produces a C-S-H gel, which can improve interface density. However, too much silica fume and insufficient calcium hydroxide affect hydration. Scholars revealed the mechanism from a microscopic perspective. Kothari et al. studied the relationship between C-S-H composition and interface thickness through scanning electron microscopy-energy dispersive spectroscopy (SEM-EDS). They found that after adding silica fume, the calcium content of C-S-H gel at the interface was high, the calcium-silica ratio varied between 1.2 and 1.6, and the thickness of the interface area was less than 20 μm. It can be seen that silica fume can improve bonding performance (KOTHARI et al., 2020).
Contradictory research results from Fiber at UHPC have been pointed out. Researchers believed that fiber could inhibit the shrinkage of concrete (VALIKHANI et al., 2020). Chen et al. tested the bond strength of UHPC with a steel fiber volume fraction of 0–2.5%; bond strength increased with fiber content increase (CHEN et al., 2021). Kabay et al. also found that with steel fiber mixed, tensile strength increased 17% (KABAY and KIZILKANAT, 2018). On the other hand, some researchers pointed out that due to UHPC and old concrete existing in a layer of mortar, fibers do not affect the bonding interface directly (HABER et al., 2018).
Kothari et al. found that UHPC was a low water-binder ratio material; it can reduce the porosity in the interface transition zone. When the water-cement ratio of UHPC was 0.33, the porosity was only 6%. Compared with ordinary concrete, UHPC porosity was lower and bond strength was higher (KOTHARI et al., 2020). Valikhani et al. observed many hydration products with a dense structure at the interface. Because of the low water-cement ratio of UHPC, there were many unhydrated particles. If the water content on old concrete is suitable, new concrete will continue to hydrate and improve bond strength (VALIKHANI et al., 2020).
2.2 Self-compacting concrete
Self-compacting concrete (SCC) can be compacted under self-weight. Compared with ordinary concrete, self-compacting concrete has a higher cementitious content, a lower water-cement ratio, fewer coarse aggregates, and excellent fluidity and passing ability. These make it more suitable as a repair material (LACOMBE et al., 1999; CHEN and ZHENG, 2010; MENG et al., 2018). This paper discusses the impact of SCC slump flow, material composition, and rheological properties on interface properties.
Diab et al. analyzed self-compacting concrete and old concrete with slump flow values of 640 mm, 710 mm, and 800 mm. They found that as the slump flow increased, bond strength also increased (DIAB et al., 2017). However, Li Linxiang et al. discovered that as the slump flow of SCC increased, the bond strength between the self-compacting concrete and track slab tended to decrease. The bond strength slightly decreased when the slump flow was increased from 570 mm to 690 mm. When the slump flow was increased from 570 mm to 730 mm, the bond strength decreased 54%. This strength decreased because when the slump flow was large, SCC may have stratified segregation due to thick water films that weaken the interfaces’ bonding force (LI et al., 2015). The difference between the findings of Diab et al. and Li Linxiang et al. is that Diab et al. incorporated some additional factors. Li Linxiang et al. found that bond strength increased with cementitious material increase because hydration products of new and old concrete interlaced to increase the bond strength (DIAB et al., 2017).
Li Linxiang et al. found that bond strength increased with cementitious material increase because hydration products of new and old concrete interlaced to increase the bond strength. Increased air content decreases bleeding tendency, and interface bond strength improves (LI et al., 2015). The addition of latex or fiber to SCC can reduce volume shrinkage. Diab et al. found that latex can reduce hydration rate and fiber can control the shrinkage of new concrete. In addition, the addition of a 10% parametric expansion agent can also improve bonding performance (DIAB et al., 2017; MENG et al., 2018).
As self-compacting concrete (SCC), its rheological properties significantly impact the bonding performance. The rheological properties of SCC mainly include thixotropy, plastic viscosity, and yield stress. Research has found that the lower the thixotropy of SCC, the better the interlayer bonding strength (DYBEŁ and KUCHARSKA, 2020). Yuan et al. found that when SCC is used in the high-speed railway filling layer, there are two filling methods: bottom-up and horizontal pushing, which mainly depend on SCC’s plastic viscosity and yield stress. When the plastic viscosity and yield stress were low, the filling method of SCC was bottom-up, which can result in air being trapped between the filling layer of SCC and the track slab, leading to lower interfacial bond strength. Conversely, SCC was filled horizontally, effectively allowing air to be expelled (YUAN et al., 2016).
2.3 Mortar of different components
Mortar is used as a repaired material. Different types of it have different effects on interfacial bond strength. A summary is shown in Table 1.
Table 1 shows that polymer mortar-modified material can improve bond strength. Wang et al. found that with styrene-acrylate (SAE) powder added, the bond strength of ordinary mortar increased from 0.97 to 1.3 MPa to 3.12–2.87 MPa (WANG and ZHANG, 2015). Kao et al. found that adding styrene-butadiene rubber (SBR) powder to mortar resulted in a tensile strength of 3.35 MPa for repair specimens (KAO et al., 2018). When SBR polymer was added to mortar, the shrinkage measured after 1 year was 1/3 less than that of ordinary silicate cement mortar, which can significantly improve the bond strength (MOMAYEZ et al., 2005a). It is important to note that while polymer doping can be good for repairing the flexural strength of a structure when it is high, the compressive and shear properties can be adversely affected when too much is added. So, fitting polymer content is 5–10% of cementitious material. The mechanism by which polymer-modified mortar enhances bonding performance is that the polymer creates a film that decreases the porosity and permeability of the interface. This film also improves bond strength through mechanical bonding (WANG and ZHANG, 2015; LEE et al., 2020), such as the polymer film formed by the polymer at the interface, the filament, and the mesh structure. These structures connect the repaired mortar and the old concrete. Table 1 shows that bond strengths, from high to low, are SAE powder, SBR emulsion, and SAE emulsion. The researchers found that modified mortar polymer film prepared by SAE powder had high film density and good toughness. It was more suitable as a repair material than SAE emulsion (WANG and ZHANG, 2015; KAO et al., 2018). However, in underwater conditions, the poor performance of polymer mortar affects bond strength (QIAN et al., 2016). In addition, polymer film will decompose under high-temperature conditions and affect bond strength (RASHID et al., 2015).
Magnesium phosphate cement (MPC) and calcium sulfoaluminate cement (CSA) are used in cement concrete pavement repair (GUO et al., 2018). MPC has good fluidity, drying shrinkage, bond strength, and wear resistance. Compared with normal Portland cement, its shrinkage is smaller. (QIAN et al., 2014). MPC has a good pore filling effect and high bond strength. Table 1 shows that when M/P (molar ratio) is 6–10, bond strength increases as the ratio decreases. The increase in bond strength is due to magnesium contents being high and hydration products being insufficient (QIN et al., 2018). In contrast, CSA mortar has other convenient advantages. Due to the rapid growth of crystals during hydration, CSA mortar forms a non-dense network structure. However, the hydration products contain more gel, which reduces porosity and improves bond strength. As age increases, bond strength increases with increased gel content. Li et al. found that adding a 0.9% high-efficiency polycarboxylic acid water-reducing agent into CSA can improve fluidity and enhance bonding performance (LI et al., 2020). Some researchers have added 30%–50% Ferric aluminate cement (FAC) to MPC. They found that FAC generates many crystals and gel materials to form a denser structure (JIA et al., 2021).
Compared to conventional concrete, mortar has good fluidity. However, the volume stability of mortar is poor because of its large shrinkage. Adding polymer-modified materials and high-flow, high-strength cement materials such as magnesium phosphate cement can reduce shrinkage and improve bonding performance.
2.4 Fibre reinforced material
Adding fibers to new concrete can reduce shrinkage and improve bond strength. The effectiveness of the improvement depends on factors such as the type, dosage, tensile strength, and elastic modulus of the fiber used (BANTHIA et al., 2014).
(1) High modulus fiber
Fibers that possess a high elastic modulus are effective in reducing concrete shrinkage. Some examples of such fibers include steel and basalt fibers. Basalt fibers are commonly 13 mm long and have a modulus of 90–110 GPa. Steel fibers also share the same length of 13 mm and are available in end-hook type, flat, and milled varieties. Among these, end-hook-type steel fibers have the best performance (QIAO et al., 2020).
The bonding properties of old concrete are affected by the amount of steel fiber and roughness on the surface. Research shows that when the surface of old concrete is smooth, the steel fibers near the bonding surface are parallel, and there is no improvement in bonding performance. On the other hand, when the surface is rough, the steel fibers are anchored in the gaps, which can improve bond strength. However, the bond strength decreases if the steel fiber is too high. Figure 2 shows that bond strength decreases when the volume content of steel fiber increases from 0.5% to 1%. This is because steel fiber content is too high, and there is insufficient cement paste to wrap the steel fibers and fill gaps (JINCHUAN et al., 2016; ZANOTTI et al., 2018). Similarly, basalt fibers can improve bond strength by increasing the plastic shrinkage resistance of cement material, but an excessive amount of fiber reduces the fluidity of mortar. An interface agent can supplement the cementitious material to enhance bond strength further. Jiang et al. compared fiber-modified mortar with or without an interfacial agent and found that the bond strength of applying acrylic acid (AAE) interface agent increased by 38% (JIANG et al., 2010). This result shows that the superior properties of high-modulus fibers as repair materials can be better leveraged using interface agents.
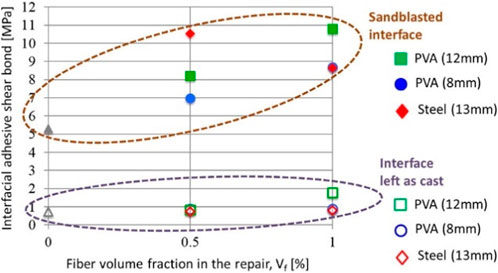
Figure 2. Bond strength of concrete with different fiber content (ZANOTTI et al., 2018).
Jiang et al. pointed out that when mixed with 0.6 kg/m3, 1.6 kg/m3 and 2.6 kg/m3 basalt fiber, bond strength increased 10.60%, 18.50% and 21.40% respectively (JIANG et al., 2010; JIANG et al., 2016). Qiao Qinghao et al. found that when the volume fraction of steel fibers is 1.2%, specimens’ shear and split tensile strengths reached 3.78 MPa and 2.99 MPa, respectively. When it increased to 1.6%, bond strength decreased to 2.98 MPa (QIAO et al., 2020). Mo et al. found that bond strength was highest when the volume fraction of steel fiber was 1.0%. When the volume fraction of steel fiber was 1.5%, the drying shrinkage value was the smallest. However, the workability of concrete was reduced (JINCHUAN et al., 2016). So, the appropriate volume fraction of steel fiber was 0.5%–1%.
(2) Low modulus fiber
Polyvinyl alcohol (PVA) fiber has low elastic modulus and good ductility, which is used to inhibit early shrinkage of concrete. PVA fiber has characteristics of a small diameter, strong hydrophilicity, and easy mixing. It can embed into pores of old concrete bases and improve bond strength. Kabiri et al., through an electron microscope, found that after the repair specimen was destroyed, the number of PVA fibers remaining in the old concrete base was the same as the damaged surface, which proved the bridging effect of PVA fibers. (KABIRI and ZANOTTI, 2019). The length of the fiber affects bonding performance. Figure 2 shows that for PVA fiber reinforced concrete with a length of 12 mm, its bonding performance is higher than 8 mm. In addition, when volume fraction of PVA fiber is 0–1%, bond strength increases with fiber content increase. However, the fiber agglomerates when more than 1% and the bond strength decreases. Qiao Qinghao et al. found that when the volume content of PVA fiber was 1.0%, shear strength reached a maximum value of 3.75 MPa (QIAO et al., 2020), so PVA fiber contents of 0.5–1% are best.
High elastic modulus fibers can improve bond strength and produce mechanical anchors on old concrete. Low elastic modulus fibers reduce the shrinkage stress at the interface by reducing the elastic modulus of cement-based materials after hardening. Both can improve bonding performance due to fibers reducing shrinkage, cracking, and bleeding at the interface. In addition, the bridging effect of fibers can improve the interfacial transition zone and inhibit the formation and development of microcracks. However, too much fiber can affect the flow of repair material. When fiber concrete is used as a repair material with an interface agent, it can supplement cementitious materials and improve bonding performance.
3 Effect of old concrete performance on bond strength
3.1 Strength of old concrete base
The sufficient strength of old concrete is an important guarantee. When the quality of old concrete is poor and there are many surface cracks, the weak parts are located in the old concrete surface. If the bonding interface strength exceeds the old concrete’s, the weak areas will be in the old concrete base. In these cases, the bonding performance is controlled by the strength of the base material. (AALETI and SRITHARAN, 2019). Generally, when repair materials have sufficient strength, fracture surfaces appear on the side of old concrete or between old concrete and interface agent. Moreover, bond strength increases with an increase in old concrete strength (ZHANG Y. et al., 2020). However, the increased strength of old concrete bases does not guarantee a higher bond strength of new and old concrete (UEDA, 2019). Using polymer fiber-modified mortar decreases bond strength as old concrete base strength increases. Because polymers and water molecules cannot penetrate high-strength old concrete bases (ZHANG et al., 2013). Courard et al. pointed out that old concrete with different strengths needs different surface treatment methods, which can ensure maximum bond strength (COURARD et al., 2014). Zhang et al. found that bond strength can increase 12%–16% when SCC is used as a repair material, but too high SCC strength has little effect on the bonding performance, and the strength difference should not exceed 5 MPa. (ZHANG X. et al., 2020). Diab et al. obtained similar results, when the strength ratio of SCC to old concrete is 1.4, the bond strength is significantly improved (DIAB et al., 2017). According to some scholars, a significant difference in stiffness between new and old concrete can lead to stress concentration at the bonding interface ends during the oblique shear test. This stress concentration can cause damage to the specimen. Therefore, minimizing the difference in elastic modulus is important to reduce this effect. (AUSTIN et al., 1999; JÚLIO et al., 2006; SANTOS and JÚLIO, 2011; DIAB et al., 2017).
3.2 Old concrete base roughness
The mechanical bite force generated by the old concrete base is an important part of the bond strength. Different surface treatments have different roughnesses. Figures 3A, B compare the bond strength test results obtained by curing for 28d, 56d, and 84d under five different surface treatments. The roughness obtained by these five surface treatments, from smallest to largest, is left-as-cast, wire-brushing, sandblasting, shotblasting, and hand-scrubbing, where L and E represent indoor curing and outdoor curing, respectively. The bond strength of the interface increased with increasing surface roughness and conditioning time under the slant-shear test. Two exceptions were identified for the hand-scrubbed surface (L56 series) and the sandblasted surface (E28 series). The effect of roughness on bond strength was more pronounced in the split test, where hand-scrubbing had the greatest bond strength. In general, the bond strength of the interface increased with the increase of the surface roughness; the bond strength of indoor curing is greater than that of outdoor curing.Bond strength after roughening treatment is stronger than without (CHAI, 2013; HABER et al., 2018; LIAO et al., 2019); an old concrete base with good roughness can increase the bond strength by several to ten times (HE et al., 2017; BENTZ et al., 2018; VALIKHANI et al., 2020). Haber pointed out that the porosity of interfaces less than 10% is suitable. If higher than 10%, it needs a certain degree of roughness to form sufficient bond strength (HABER et al., 2018). Therefore, roughening treatment is necessary, it can provide the main mechanical bite force.
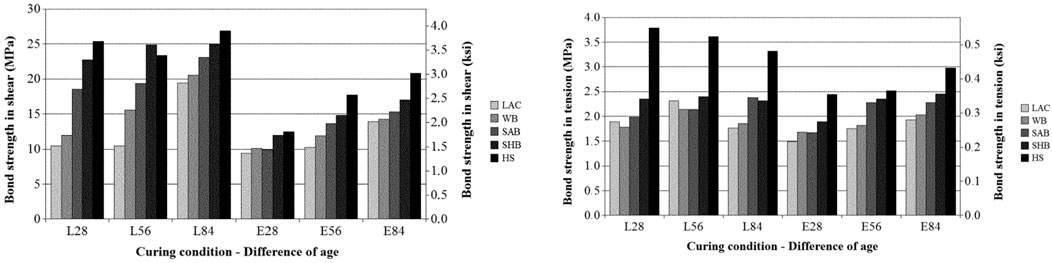
Figure 3. Test results of different old concrete treatment methods: (A) slant shear test and (B) split test (SANTOS and JÚLIO, 2011).
Currently, two commonly used techniques for treating old concrete bases are physical and chemical. Physical methods can be further divided into two categories: mechanical treatment and spray treatment. Mechanical treatment techniques include wire brushing, surface grooving, chiseling, drilling, etc. Spray treatment techniques include sandblasting, high-pressure water jet, and so on. The chemical method is to conduct chemical corrosion on old concrete to improve mechanical bite force. Each method has its advantages and limitations, mechanical treatment equipment is simple and convenient to construct, after the slotting treatment, the shear strength of the bonding surface can be similar to that of the embedded bar specimen, and even significantly improved. In addition, after old concrete is chiseled, the surface becomes loose, the bonding area becomes larger, and mortar is easy to penetrate. But microcracks and internal damage influence strength (HAN et al., 2001). Jet treatment has little disturbance to base layers, but the requirement for equipment is strict (HABER et al., 2018). Chemical treatment increases interface roughness, interfacial mechanical bite force, and van der Waals. However, this method is difficult to control and unreliable compared to the mechanical method (JIANG, 2001). Table 2 shows the roughness of different treatment methods. The roughness produced by sandblasting and high-pressure water jet methods is lower than that of mechanical treatment. Generally accepted that the sandblasting method when old concrete strength is low. On the contrary, the mechanical treatment method is adopted.
Table 3 summarizes bond strength under different roughness. Sandblasting and high-pressure water jet methods have small roughness and high bond strengths. When roughness is less than 1 mm, interface bond strength measured by the oblique shear test can reach 14.31 MPa, and measured by the splitting test, it can reach 4.1 MPa. The steel wire brush can obtain a large roughness, the interfacial bond strength measured by oblique shear can reach 10.67 MPa. Different chiseling methods produce varying levels of roughness depending on engineering requirements. As roughness increases, bond strength experiences a significant boost of up to 102.7%, with the potential to reach 1.6 MPa during splitting tests. Surface drilling, grooving, and exposing surface coarse aggregates are the most effective mechanical treatment methods for achieving roughness. Among them, the grooving method stands out with an impressive bond strength of up to 25.39 MPa in oblique shear tests, resulting in a noticeable enhancement effect. As measured by oblique shear, the interfacial bond strength experiences an 82.3% increase. Meanwhile, 34.6% and 15.5% bond strength increases are observed under splitting and tensile tests, respectively. Table 2 shows that drilling and grooving improvement was more obvious in the oblique shear test; the interfacial bond strength can increase 82.3%, under splitting and tensile tests, the bond strength increased only 34.6% and 15.5%. Some scholars molded old concrete with fixed roughness molds; these concretes have excellent bonding properties, but their application still needs verification. In summary, sandblasting and high-pressure water jet methods perform best, while chiseling and grooving have lower costs.
According to research, bond strength achieves its optimal state at a certain level of roughness, and a further increase in roughness has a negligible effect (WANG et al., 2016). Therefore, it is crucial to choose an appropriate method to assess roughness. This paper analyzed common evaluation methods, including sand filling, fractal dimension, digital image, silicon powder stacking, and aggregate exposure ratio methods. The sand-filling method is simple and suitable for small, commonly used components. But not suitable for larger area roughness. Fractal dimension methods can quantitatively and objectively evaluate surface geometry. As shown in Figure 4, combining optical methods can obtain 3D shape data from real surfaces. However, fractal dimension methods are complicated and unsuitable for construction (NA et al., 2006; SHI, 2018; ZHANG Y. et al., 2020). Digital image methods use a digital camera to photograph rough surfaces and grayly process them. The brightness of each point on the image represents roughness. Silicon powder stacking methods use 50 g of silicon powder with a particle size of 50–100 μm to form a circle in the test area, and the radius of the circular area defines the roughness index (SRI); the larger the roughness index, the smoother the interface. Aggregate exposure ratio methods define surface roughness by coarse aggregate exposure percentage. Grade A roughness: about 10% of the coarse aggregate is visible; grade B roughness: about 30–40% of the coarse aggregate is visible; grade C roughness: about 60–80% of the coarse aggregate is visible. However, this method is not accurate.
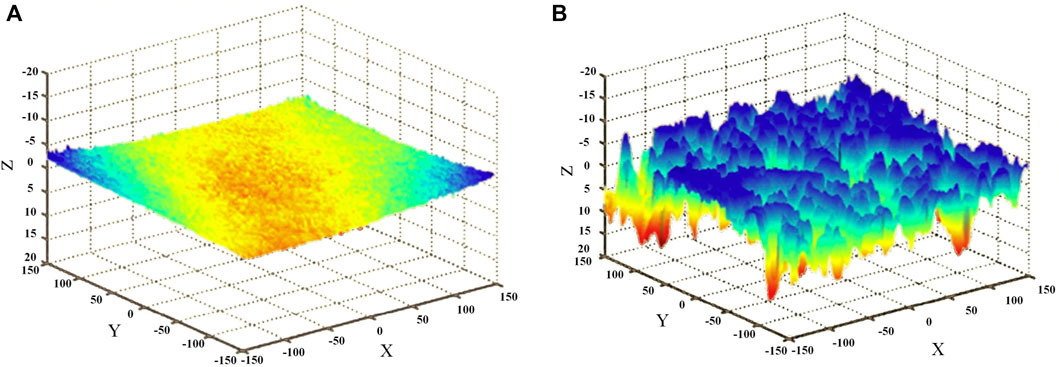
Figure 4. Surfometry profiles obtained by optomorfology (A) smooth surface and (B) hydrodemolished surface (COURARD et al., 2014).
3.3 Water content state of old concrete base
The role of water is a controversial issue. On the one hand, dry old concrete can absorb the moisture of new concrete to fill pores and improve compactness. Moreover, an increase in water content in old concrete leads to an increase in the water-cement ratio of the interface concrete, increasing porosity and decreasing bond strength (LI et al., 2020). In addition, moisture affects the bonding properties of epoxy resin. With increased moisture, fracture surfaces appear at bond interfaces, so epoxy resin is suitable for dry bases (BŁASZCZYŃSKI et al., 2006; WAN et al., 2006; LAU and BÜYÜKÖZTÜRK, 2010). On the other hand, some studies believe that dry concrete bases can damage bonding performance. When the water content of old concrete is low, water is sucked into the old concrete before new concrete forms, which affects the water cement ratio of new concrete and its strength (BEUSHAUSEN et al., 2017). Furthermore, dry old concrete will not fully hydrate new concrete, reducing bond strength (ZHANG Y. et al., 2020).
Zhang et al. found that bond strength was highest when the old concrete base remained saturated and dry. The bond strength of new and old concrete is 49.0% higher than dry surfaces (ZHANG Y. et al., 2020). Research by Santos et al. showed that saturated dry surfaces in old concrete are stronger than dry surfaces (SANTOS et al., 2012). The volume shrinkage caused by a high-water-cement ratio in the interface can be avoided when the old concrete base surface remains saturated and dry. In addition, in the process of pouring new concrete and its subsequent hydration, the decrease in water content of new concrete can be prevented, and improved bonding performance.
4 Effect of different types of interfacial agents on bond strength
Brushing a layer of inorganic or organic material on the surface of old concrete can enhance the bond performance. It is generally believed that the thickness of interfacial agents should be greater than 0.5 mm and less than 1.5 mm. Common interfacial agents include cement paste, cement mortar, and epoxy resin (XIONG et al., 2002). These agents work through different enhancement mechanisms, which this paper explains in detail.
4.1 Cement mortar
When cement mortar is used as an interface agent, due to its properties being similar to old concrete bases, the C-S-H gel and ettringite formed in the hydration process can penetrate the cracks of an old concrete base, reducing the interface porosity and form a dense interface transition zone. Xiong et al., through scanning electron microscopy, found that the ettringite penetrated the microcrack and improved the bond strength to a certain extent. (XIONG et al., 2002). However, due to the brittleness of cement mortar, it performs poorly (RASHID et al., 2020). Therefore, fly ash, expansion agent, and polymer can be added to cement mortar to improve performance. Table 4 shows fly ash can improve the interfacial transition zone and increase intermolecular force. Xiong et al. pointed out that excessive fly ash makes the calcium to silicon (C/S) ratio in C-S-H gel smaller, which is good for formatting C-S-H gel to form a dense structure (XIONG et al., 2002). Adding expansion agents has a certain compensation effect on shrinkage, and the microstructure of the interface is more compact than the interface between cement paste and old concrete (XIONG et al., 2002). Compared with cement paste, the bond strength of polymer mortar increased 31%; the polymer film can reduce the porosity of the interface and can also play a mechanical squeezing effect. However, polymers deteriorate rapidly at high temperatures and recover at low temperatures, affecting the bond strength (KUNTAL et al., 2020). Mohammadi also pointed out that polymer cement is not suitable for concrete repair engineering under high humidity (MOHAMMADI et al., 2014). In addition, adding fine aggregate to cement paste to form cement mortar can improve bond strength. Compared with cement paste, the fine aggregate enhances the interface’s mechanical bite and intermolecular forces. Under oblique shear tests, bond strength increased 12.8%. After adding fly ash modified mortar, splitting strength increased 22.7%.
4.2 Epoxy resin
Epoxy resin is an efficient interlayer adhesive and has the advantages of short initial setting time, rapid development of early strength, good toughness, corrosion resistance and high bond strength. The bond strength requirements can be met with a thickness of less than 1 mm. On the other hand, it can repair concrete defects and strengthen interlayer bonding, making stress distribution uniform (AHMED and AZIZ, 2019).
The roughness of old concrete affects the bonding properties. Santos et al. tested bonding performance under different roughness conditions and found that when the roughness of old concrete bases is large, epoxy resin has little effect on bonding performance (SANTOS et al., 2012). Rashid et al. also noted that epoxy resin has no obvious effect at large roughnesses. On the contrary, the epoxy resin acts well on a smooth surface (RASHID et al., 2020). Kuntal et al. found that epoxy resin accumulated on rougher surfaces, forming a thicker interface layer and decreasing lead bond strength (KUNTAL et al., 2020).
Brushing methods also affect bond strength. Blaszczynski et al. pointed out that when epoxy resin is used as a bonding layer, brushing it vertically in two directions can ensure uniformity. This brushing method increases the tensile strength of the bonding layer increased 1 MPa. (BŁASZCZYŃSKI et al., 2006). Moisture has a significant effect on the bonding properties of epoxy resin. However, the results show that bond strengths do not decrease after the dry-wet cycle. To clarify the bonding mechanism between the epoxy resin and old concrete, Lau et al. established a prediction model based on dynamic simulation technology, combined with a finite element method to link micro-atomic scale and macro-test results (BÜYÜKÖZTÜRK et al., 2011; LAU et al., 2012).
There are compelling reasons to consider improving bond strength with interfacial agents. Firstly, such agents can enhance the chemical force in interfacial transition zones by filling pores on the surface of old concrete. Secondly, they can prevent water from migrating from new concrete to old concrete bases, making them particularly effective for reinforcing poorly filled new concrete. Interfacial agents can produce two transition zones that may affect bond strength if the agent is inappropriate.
5 Effect of environment on bond strength
Over time, various environmental factors can impact new and existing concrete structures and their bonding surfaces, such as high temperatures, rainwater from freeze-thaw cycles, and chemical erosion. These factors can significantly affect the performance and longevity of structures. Additionally, the decay rates of repair materials can vary depending on the specific service environment. This paper aims to detail the decay laws of different repair materials in response to varying environmental conditions.
5.1 Ambient temperature
This paper discusses the impact of environmental temperature fluctuations on concrete structures exposed to extreme heat. Temperatures in Xinjiang and some parts of the Middle East and North America can reach 60°C in summer, affecting the bond strength between new and old concrete. The concrete material, ambient temperature, and interface agent performance influence the bond strength. The paper summarizes the effects of different ambient temperatures on the bonding properties of concrete. Table 5 shows that increased temperature and temperature cycle times, decrease bonding performances. Rashid et al. tested the bonding properties of polymer modified mortar; the bond strength decreased 48.16% at 60 C (RASHID et al., 2015). Al-gahtani et al. analyzed the bonding properties of different repair materials after 90 cycles at 20–70°C. The result showed that compared with cement-based materials, resin materials’ strengths decreased more. For cement-based materials, strength decreased 3.2–17.46% after 60 cycles and decreased 8.07–34.80% after 90 cycles. For resin materials, strength decreased 9.3–20.47% after 60 cycles and decreased 18.98–36.43% after 90 cycles (AL-GAHTANI et al., 1995). Temperature fluctuations in the natural environment can negatively affect the mechanical properties of the polymer, disrupt the polymer film, change the interfacial porosity, and ultimately impact the bond strength of concrete. Therefore, in high-temperature areas, it is recommended to use cement-based materials to prevent the adverse effects of polymer performance degradation on bonding properties.
The mechanism of high temperature affecting bonding performance includes two aspects: on the one hand, in the high-temperature environment, due to the new and old concrete expansion rate is different, the cement stone and aggregate expansion rate is also different; in the bonding interface to produce a larger internal stress, high pulp to bone ratio of the concrete than the low ratio strength decline is greater. On the other hand, the internal pores of concrete change at high temperatures. When the temperature is high, the pore water evaporates, and the pore pressure becomes large, resulting in small cracks in the concrete. In contrast, the drying and water loss of the concrete will cause some of the small cracks to continue to develop, and then the large cracks generated by the penetration of the small cracks will reduce the interfacial bond strength. (RASHID et al., 2015). 76 (BAZANT and KAPLAN, 1996; RASHID et al., 2019).
5.2 Freeze-thaw cycle
Table 6 shows that the freeze-thaw cycle significantly reduces interfacial bond strength (GAO and CHENG, 2006). The factors affecting the frost resistance of new and old concrete include the composition of the concrete, the state of the old concrete base, and the choice of interface agent. Repairing material is a key factor affecting bond strength after freeze-thaw cycles.
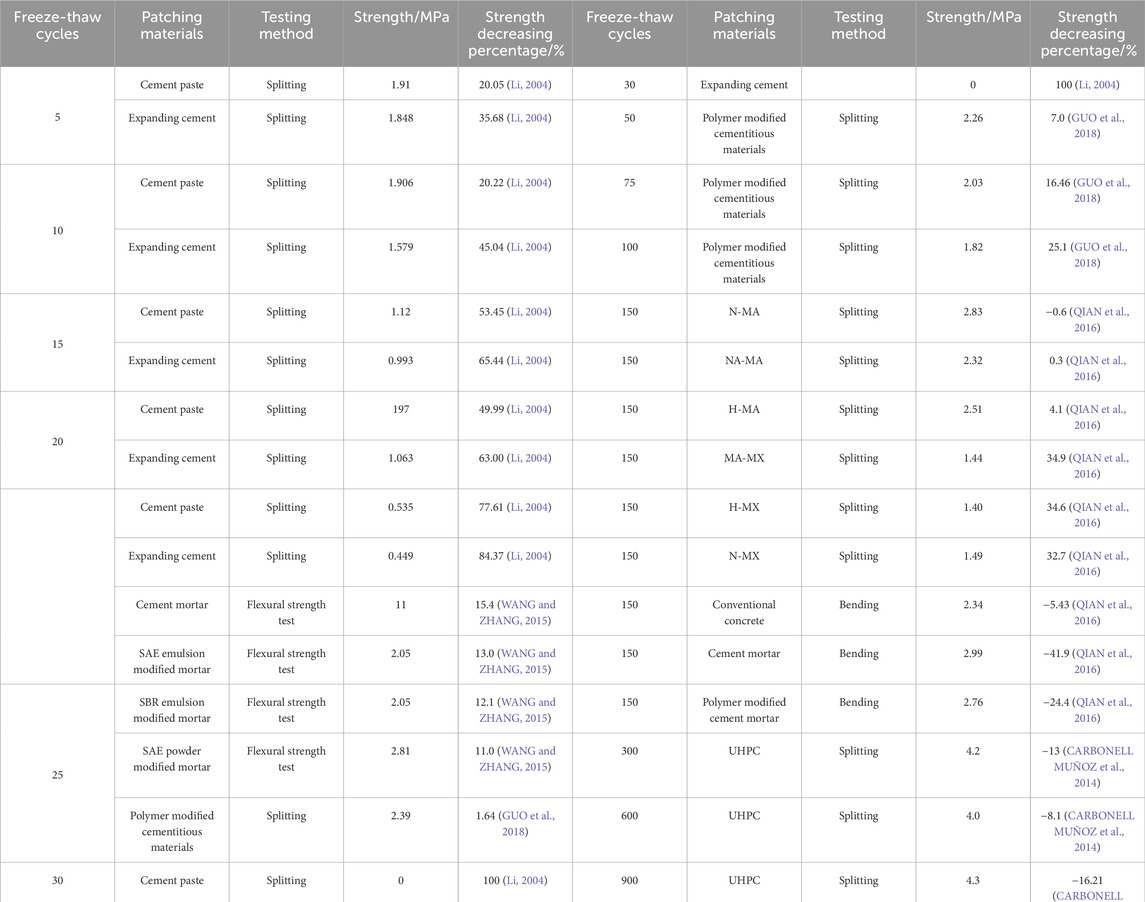
Table 6. Summary of literature review on bonding properties of new and old concrete under freeze-thaw cycles.
Table 6 shows that some scholars have studied the frost resistance of different repair materials. Qian et al. found that the bonding performance of mortar with an air-entraining agent showed no change after 150 freeze-thaw cycles, while without it, it decreased 30%. Li Ping first found that bonding performance can be doubled after adding an air-entraining agent to new concrete (Li, 2004). Another study by Qian et al. found that at the interface of PCM-modified materials, there was a high percentage of polymer film mortar, which had good resistance to water penetration with little change in strength after 150 freeze-thaw cycles and good frost resistance (QIAN et al., 2016). Ru et al. through scanning electron microscopy, observed a dense polymer film in polymer modified mortar. It had good impermeability, after 25 freeze-thaw cycles, the strength of SAE emulsion modified mortar, SBR emulsion modified mortar, and SAE powder modified mortar decreased 13.0%, 12.1%, and 11%, respectively (WANG and ZHANG, 2015).
Guo et al. found that specimens with higher roughness have stronger frost resistance (GUO et al., 2018). Fan studied freeze-thaw cycles of specimens with different roughnesses; results are shown in Figure 5. When the fractal dimension of roughness increases from 2.1 to 2.2, freeze-thaw resistance does not increase. When it was greater than 2.3, frost resistance improved. It is worth noting that Li Xianping and Cheng et al. pointed out that too much roughness reduces impermeability, so after chiseling, cleaning the surface damage of cement stone is enough. (YI et al., 2013). In addition, some studies found that bond strength increases as freeze-thaw times increase, possibly because unhydrated cement continues to hydrate during the freeze-thaw process. The results are similar to Wang et al. (WANG and GUPTA, 2021).
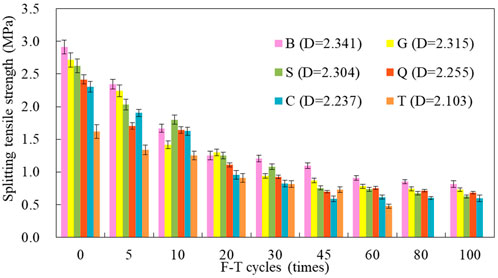
Figure 5. The influence of freeze-thaw cycle on concrete with different roughness (FAN et al., 2021).
Table 6 shows that various interface agents have different levels of frost resistance. Cement mortar has higher frost resistance than cement paste and expansive cement paste. After 25 freeze-thaw cycles, the strength of cement paste and expansive cement paste significantly decreased. In contrast, the strength of cement mortar decreased 15.4% because mortar has a larger elastic modulus and a smaller linear expansion coefficient after hardening (Li, 2004).
It is clear from the concrete freeze-thaw damage mechanism that damage is mainly due to: 1) during freezing process, water volume expands, causing pore pressure. 2) crystal growth in pores causes pressure crystallization. 3) difference in thermal characteristics between ice and cement matrix (HANJARI et al., 2011). In summary, modifying materials or interface agents can change the interface state from loose to dense, while air-entraining agents can reduce the volume expansion of crystalline ice. Both methods can improve the frost resistance of repair structures.
5.3 Ambient humidity
Ambient humidity affects the bonding performance of concrete structures. Studies showed that wet conditions affect concrete and epoxy performance (LAU and BÜYÜKÖZTÜRK, 2010). Some scholars have covered concrete specimens with a wet cloth for 4 weeks, and concrete-epoxy resin bond strength decreased 50%. After 4 weeks of continuous load and water, the strength loss is up to 77.6% (ZHOU et al., 2017). Moisture affects bonding properties in two ways. One can be seen in Figure 6A that epoxy resin absorbs water and plasticizes in water. Epoxy infiltration into old concrete base increases the toughness of the concrete top. When failure occurs, crack at the interface stays in the original area and does not propagate into concrete. It requires little energy to propagate on the bonding surface. The other can be seen in Figure 6B that water molecules hinder interaction between epoxy resin and silica under wet conditions, so epoxy is unsuitable for load-bearing structures exposed to water for a long time (FRIGIONE et al., 2006; BÜYÜKÖZTÜRK et al., 2011; LAU et al., 2012). In particular, different polymers perform differently in humid environments. Some of these polymers’ strength decreased due to the dissolution of the polymer film, and some may react with water to form stronger hydrogen bonds to increase bond strength. (RASHID et al., 2015).
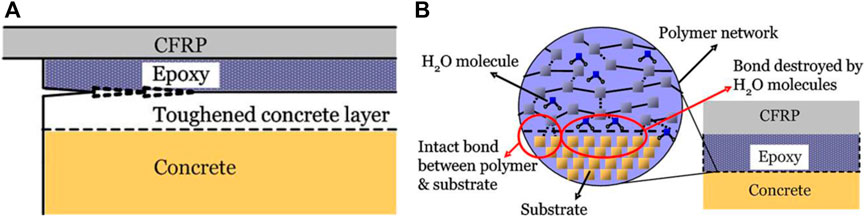
Figure 6. (A). Plasticization of epoxy-penetrated concrete layer; (B). Weakening of bond between epoxy and concrete (BÜYÜKÖZTÜRK et al., 2011).
Increasing interface density and reducing water absorption can improve bonding performance in a high-humidity environment. Long et al. pointed out that silica fume can reduce water absorption, and the filling effect reacts with volcanic ash to make microstructures dense (LONG et al., 2011). Polymer particles can also improve impermeability, making microstructures dense (WANG and ZHANG, 2015).
5.4 Chemical erosion
Chemical erosion significantly affects bonding performance. At present, typical chemical erosion in nature is divided into two types: sulfate and chloride. Table 7 shows that after the sulfate attack, ordinary mortar specimens’ bond strength decreased 27.7% and UHPC specimens decreased 29.8%. Hussein et al. immersed concrete repair specimens in a sulfate solution for 90 days and, through scanning electron microscopy, found that there were pores, microcracks, and many reaction products in the interfacial transition zone. In a high concentration sulfate environment, C-S-H gel continuously decomposed, expanded reaction products, and decreased bond strength (HUSSEIN et al., 2018). Hussein et al. pointed out that the cracking load and peak load of concrete specimens decreased after chloride ion erosion (HUSSEIN et al., 2020). Table 7 shows that in a chloride environment, the strength of ordinary mortar decreases 25% and UHPC repair material decreases 23.9%. Chen et al., through SEM-EDS, found that there were chloride ions in concrete, which proved that chloride ions can reach a certain depth (CHEN et al., 2021).
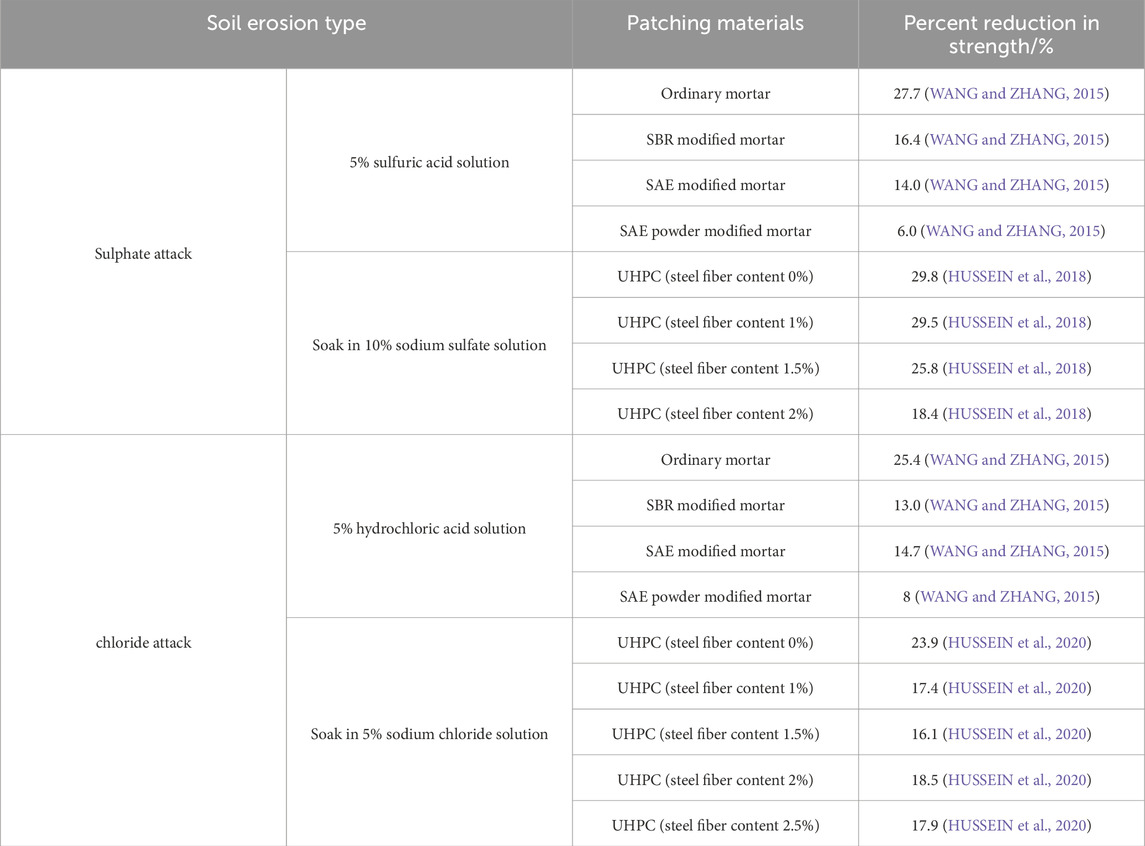
Table 7. Summary of literature review on bonding properties of new and old concrete under chemical attack.
Some scholars choose polymer, fiber, and other modified concrete as repair materials to improve chemical corrosion resistance. Table 7 shows that SAE modified mortar and fiber concrete have good sulfate resistance (WANG and ZHANG, 2015; HUSSEIN et al., 2018). After 5% sulfuric acid solution erosion, the bond strength of ordinary Portland cement mortar decreased 27.7%, while the tensile bond strength of SAE powder modified mortar was only reduced 6.0% (WANG and ZHANG, 2015). Hussein et al. found that under sulfate attack with a concentration of 10%, flexural strength and compressive strength decreased slowly as steel fiber content increased. However, the concentration of reaction products and depth of erosion increased. Chen et al. found that when the volume fraction of steel fiber is 1.5%, the resistance to chloride ion erosion is the best (CHEN et al., 2021). Excessive fiber content increases the porosity of interfaces, resulting in decreased chemical resistance (HUSSEIN et al., 2020).
The failure mechanism of the bonding interface in a chemical environment has two main aspects: 1) external acid, salt, and other chemicals react with concrete, causing erosion of the cement hydration products, which rapidly decompose into products that lose their cementing ability; 2) the erosion products generated have a certain volume expansion, which leads to crystallization and pore pressure reduction or even loss of bonding performance between new and old concrete (SCHERER, 2004).
6 Bonding performance test method
The bond strength of new and old concrete is closely related to the test method. The bond strength values obtained by different testing methods cannot be directly compared, so it is necessary to clarify the characteristics of different bond test methods. Currently, test methods are mainly divided into direct tensile, indirect tensile, shear test and non-destructive testing methods.
In a direct tensile test, tensile stress of specimen is measured by applying a force perpendicular to bonding surface, as shown in Figures 7A,B. Figure 7A is a common tensile test method, but the tensile process is susceptible to eccentricity. Figure 7B shows common tensile strength test methods, which can measure reliable results at a lower eccentricity. However, the stress state of weak points at the edge of interface changes after debonding, resulting in a small result. Therefore, the tensile test is suitable for cases where the interface strength is less than the material strength (SILFWERBRAND et al., 2011).
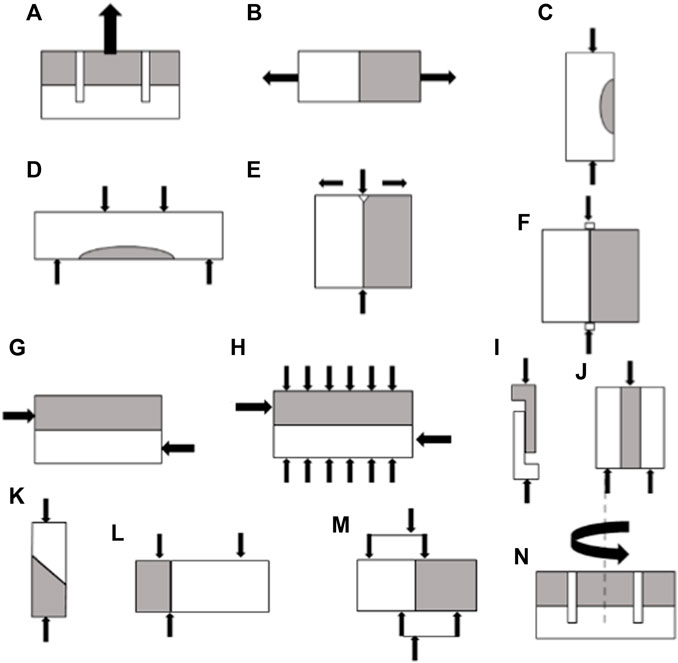
Figure 7. Various test methods to determine interface bond strength between concrete substrate and overlay (SILFWERBRAND et al., 2011). (A) Direct tensile test, (B) Direct tensile test, (C) Bending tests, (D) Bending tests, (E) Splitting tests, (F) Splitting tests, (G) Direct shear tests, (H) Direct shear tests, (I) Shear tests, (J) Shear tests, (K) Oblique shear tests, (L) Direct shear tests, (M) Direct shear tests, (N) Shear tests.
Indirect tensile tests include various bending and splitting tests, as shown in Figure 7C – Figure 7F. Bending test form is shown in Figures 7C,D. Compared to volume of specimen bending tests, load bearing bond surface areas are smaller. Only a part of the bonding plane is subjected to maximum stress, so it is suitable for bending repaired structures. The splitting test is shown in Figures 7E,F. Figure 1F is a common splitting test that allows bond strength testing of circular or cubic bonded specimens (ZHANG et al., 2017), and Figure 7E uses a wedge-shaped splitting test for more targeted testing of bond surface strength (DELATTE, 2009).
The shear test includes direct and oblique shear tests. As shown in Figures 7G – Figures 7N is a common direct shear test. Figure 1H performs shear tests by applying a uniform force perpendicular to the interface, which is suitable for actual (DELATTE, 2009). Most of the shear tests bear both shear and bending stress. Using the Z-shaped specimen in Figure 7I can reduce bending stress and data dispersion (TIAN et al., 2018; ZHANG X. et al., 2020). Figure 7J is a form of double-sided shear. Silfwerbrand et al. concluded that the bond strengths tested by this method differed from those in the field. However, Chai Min, Bai Wenjun, et al. found that the failure of specimens during double-sided shear was a one-sided failure, which was consistent with actual situation (BAI et al., 2008; CHAI, 2013), so it should be analyzed according to the real situation. Figure 7K is an oblique shear test that can test interfacial bond strength under shear and pressure (MEGID and KHAYAT, 2017; ZHANG et al., 2017; MA et al., 2019). Figure 7L applies a shear force to the bonding surface, but it is difficult to apply it accurately. The disadvantage of shear tests is that eccentric bending moments may occur. Using the test method in Figure 7M can avoid it. The above tests need to be carried out in the laboratory, resulting in a deviation from the actual project. Silfwerbrand et al. tested bond strength by applying torque to drill core on-site, as shown in Figure 7N. The results were the same as the shear test, which was more suitable for on-site construction (SILFWERBRAND, 2003). The interface shearing force of new and old concrete comes from the external load and the shrinkage of new concrete. Qian et al. used conical specimens to simulate the uncoordinated deformation of repair materials limited by multiple directions of old concrete (QIAN et al., 2014).
Compared with other test methods, oblique shear tests have a higher bond strength (MOMAYEZ et al., 2005b). Under the same conditions, the splitting test results were lower (SANTOS et al., 2007). The order of bond strengths from largest to smallest was oblique shear, double-sided shear, splitting, and tensile. Momayez et al. summarized the relationships between different test methods. The results showed that the bond strength measured by pull-out and splitting tests for cement-based repair materials is 34–48%; double-sided shear and oblique shear tests were about 67–93% of old concrete (MOMAYEZ et al., 2005b).
Non-destructive testing methods, such as interface ultrasonic pulses, interface resistivity and water permeability, and impact echo, are commonly employed to indirectly assess the bond performance between old and new concrete. Several researchers have utilized these methods for evaluating the bond properties. Qian et al. emphasized a strong correlation (with correlation coefficients above 0.9) between the initial amplitude of ultrasonic pulses, initial interface current, interface water permeability, and splitting tensile strength, indicating that larger interface porosity leads to more significant attenuation of ultrasonic amplitude and lower interface resistivity (QIAN and XU, 2018). Garbacz et al. employed the impact echo method to analyze the bond strength of old and new concrete. They observed that the peak value of the frequency spectrum is influenced by surface treatment methods of the old concrete, although statistically insignificant. Furthermore, wavelet analysis (Wavelet approach) offers better insights into the impact of voids on stress wave propagation within repair systems, with parameters describing amplitude deviation increasing with higher tensile test strengths (GARBACZ et al., 2017).
7 Prospect
Based on the above discussions, extensive research has been conducted on bond performance of interface between new and old concrete. The research mainly focuses on repair materials, interface conditions of old concrete, interface agents, and testing methods. But as the service conditions of new and old concrete structures become more complex, some issues related to bond performance of interface between new and old concrete have also been identified. For this reason, further research is needed in following areas:
(1) Currently, there is much research on repair materials. However, most of the research at this stage focuses on materials under normal temperature conditions, with limited studies on materials under negative temperature conditions. Furthermore, research on thin-layer repair materials needs to be improved. Hence, there is a need to strengthen further research in these areas.
(2) There is relatively comprehensive research on the mechanical properties of the interface between new and old concrete. Nevertheless, there is a need for further investigation into the constitutive relationship, characteristics, and evolution process of interfacial damage. Therefore, future research should focus on developing and establishing constitutive models for the interfacial bond, laying a solid foundation for quantitatively characterizing interfacial damage properties.
(3) The current research on the influence of the environment on interfacial bond strength mainly considers factors such as temperature, freeze-thaw cycles, environmental humidity, and chemical erosion. However, these studies generally consider singular environmental factors. In practical engineering applications, the interfacial bond between new and old concrete is often subjected to the coupling effects of load and environment, so it is necessary to strengthen experimental and theoretical research on interfacial bonds under the coupled action of load and environment.
(4) With the development of concrete structural forms, structures and loading conditions of the interface between new and old concrete are becoming complex. The laboratory testing methods fail to reflect interfacial bond performance in real engineering applications accurately. In future work, efforts should be made to simulate structural and loading characteristics of the interface between new and old concrete as closely as possible to provide direct data for practical applications. Non-destructive testing methods for evaluating the interfacial bond performance of new and old concrete currently lack precision and relevant theories. So, further research should be conducted to enhance non-destructive testing methods and theories related to the interfacial bond properties of new and old concrete.
Author contributions
HL: Data curation, Funding acquisition, Methodology, Project administration, Supervision, Writing–original draft. HZ: Investigation, Writing–original draft, Writing–review and editing. JinZ: Conceptualization, Investigation, Supervision, Visualization, Writing–original draft. JiZ: Writing–original draft. YT: Formal Analysis, Validation, Writing–original draft. JianZ: Writing–original draft. YG: Formal Analysis, Validation, Writing–original draft. JX: Writing–original draft.
Funding
The author(s) declare that financial support was received for the research, authorship, and/or publication of this article. This work was supported by Liaoning transportation technology project (grant number: 202328); Liaoning transportation technology project (grant number: 202217); Open Fund of National Engineering Research Center of Highway Maintenance Technology (Changsha University of Science and Technology) (grant number: kfj210105).
Conflict of interest
Author JiZ was employed by Liaoning new development highway technology maintenance Co. LTD.
Authors YT, JianZ, and YG were employed by Liaoning Datong Highway Engineering Co. LTD.
The remaining authors declare that the research was conducted in the absence of any commercial or financial relationships that could be construed as a potential conflict of interest.
Publisher’s note
All claims expressed in this article are solely those of the authors and do not necessarily represent those of their affiliated organizations, or those of the publisher, the editors and the reviewers. Any product that may be evaluated in this article, or claim that may be made by its manufacturer, is not guaranteed or endorsed by the publisher.
References
Aaleti, S., and Sritharan, S. (2019). Quantifying bonding characteristics between UHPC and normal-strength concrete for bridge deck application. J. Bridge Eng. 24 (6), 04019041. doi:10.1061/(asce)be.1943-5592.0001404
Ahmed, G. H., and Aziz, O. Q. (2019). Shear behavior of dry and epoxied joints in precast concrete segmental box girder bridges under direct shear loading. Eng. Struct. 182, 89–100. doi:10.1016/j.engstruct.2018.12.070
Al-Gahtani, A. S., Rasheeduzzafar, , and Al-Mussallam, A. A. (1995). Performance of repair materials exposed to fluctuation of temperature. J. Mater. Civ. Eng. 7 (1), 9–18. doi:10.1061/(asce)0899-1561(1995)7:1(9)
Austin, S., Robins, P., and Pan, Y. (1999). Shear bond testing of concrete repairs. Cem. Concr. Res. 29 (7), 1067–1076. doi:10.1016/s0008-8846(99)00088-5
Bai, W. J., He, Z. Y., and Hu, J. Y. (2008). Bonding strength between old and repair concrete [J]. Concrete (1), 45–48.
Banthia, N., Majdzadeh, F., Wu, J., and Bindiganavile, V. (2014). Fiber synergy in hybrid fiber reinforced concrete (HyFRC) in flexure and direct shear. Cem. Concr. Compos. 48, 91–97. doi:10.1016/j.cemconcomp.2013.10.018
Bazant, Z. P., and Kaplan, M. F. (1996). Concrete at high temperatures[J]. Proc. R. Soc. A 83 (561), 200–209.
Bentz, D. P., De La Varga, I., Muñoz, J. F., Spragg, R. P., Graybeal, B. A., Hussey, D. S., et al. (2018). Influence of substrate moisture state and roughness on interface microstructure and bond strength: slant shear vs pull-off testing. Cem. Concr. Compos. 87, 63–72. doi:10.1016/j.cemconcomp.2017.12.005
Beushausen, H., Höhlig, B., and Talotti, M. (2017). The influence of substrate moisture preparation on bond strength of concrete overlays and the microstructure of the OTZ. Cem. Concr. Res. 92, 84–91. doi:10.1016/j.cemconres.2016.11.017
Błaszczyński, T., Jasiczak, J., Ksit, B., and Siewczyńska, M. (2006). Aspects of bond layer role in concrete repairs. Archives Civ. Mech. Eng. 6 (4), 75–87. doi:10.1016/s1644-9665(12)60277-5
Büyüköztürk, O., Buehler, M. J., Lau, D., and Tuakta, C. (2011). Structural solution using molecular dynamics: fundamentals and a case study of epoxy-silica interface. Int. J. Solids Struct. 48 (14-15), 2131–2140. doi:10.1016/j.ijsolstr.2011.03.018
Carbonell Muñoz, M. A., Harris, D. K., Ahlborn, T. M., and Froster, D. C. (2014). Bond performance between ultrahigh-performance concrete and normal-strength concrete. J. Mater. Civ. Eng. 26 (8), 04014031. doi:10.1061/(asce)mt.1943-5533.0000890
Chai, M. (2013). Experimental reserch on shear properties of self-compating concrete on existing concrete[J]. J. Fuzhou Univ. Nat. Sci. Ed. (05), 922–927. doi:10.7631/issn.1000-2243.2013.05.0922
Chen, F., and Zheng, J. L. (2010). Bond and slip relationship between self-compacting concrete and old concrete[J]. J. Fuzhou Univ. Sci. Ed. 38 (2), 266–271.
Chen, Q., Ma, R., Li, H., Jiang, Z., Zhu, H., and Yan, Z. (2021). Effect of chloride attack on the bonded concrete system repaired by UHPC. Constr. Build. Mater. 272, 121971. doi:10.1016/j.conbuildmat.2020.121971
Courard, L., Piotrowski, T., and Garbacz, A. (2014). Near-to-surface properties affecting bond strength in concrete repair. Cem. Concr. Compos. 46, 73–80. doi:10.1016/j.cemconcomp.2013.11.005
Diab, A. M., Abd Elmoaty, A. E. M., and Tag Eldin, M. R. (2017). Slant shear bond strength between self compacting concrete and old concrete. Constr. Build. Mater. 130, 73–82. doi:10.1016/j.conbuildmat.2016.11.023
Dybeł, P., and Kucharska, M. (2020). Effect of placement technology on the bond strength between two layers of self-compacting concrete. Mater. 13 (15), 3330. doi:10.3390/ma13153330
Fan, J., Wu, L., and Zhang, B. (2021). Influence of old concrete age, interface roughness and freeze-thawing attack on new-to-old concrete structure. Materials 14 (5), 1057. doi:10.3390/ma14051057
Frigione, M., Aiello, M. A., and Naddeo, C. (2006). Water effects on the bond strength of concrete/concrete adhesive joints. Constr. Build. Mater. 20 (10), 957–970. doi:10.1016/j.conbuildmat.2005.06.015
Gao, D. Y., and Cheng, H. Q. (2006). Freeze-thaw cycle influencing on the bonding properties of new to old concrete[J]. Concrete (03), 29–32.
Garbacz, A., Piotrowski, T., Courard, L., and Kwaśniewski, L. (2017). On the evaluation of interface quality in concrete repair system by means of impact-echo signal analysis. Constr. Build. Mater. 134 (1), 311–323. doi:10.1016/j.conbuildmat.2016.12.064
Guo, T., Xie, Y., and Weng, X. (2018). Evaluation of the bond strength of a novel concrete for rapid patch repair of pavements. Constr. Build. Mater. 186, 790–800. doi:10.1016/j.conbuildmat.2018.08.007
Haber, Z. B., Munoz, J. F., De La Varga, I., and Graybeal, B. A., (2018). Bond characterization of UHPC overlays for concrete bridge decks: laboratory and field testing. Constr. Build. Mater. 190, 1056–1068. doi:10.1016/j.conbuildmat.2018.09.167
Han, J. H., Yuan, Q., and Zhang, L. S. (2001). Research on practical treating method for the interfacial roughness of new to old concrete[J]. Ind. Constr. (02), 1–3. doi:10.13204/j.gyjz2001.02.001
Hanjari, K. Z., Utgenannt, P., and Lundgren, K. (2011). Experimental study of the material and bond properties of frost-damaged concrete. Cem. Concr. Res. 41 (3), 244–254. doi:10.1016/j.cemconres.2010.11.007
Harris, D. K., Sarkar, J., and Ahlborn, T. T. M. (2011). Characterization of interface bond of ultra-high-performance concrete bridge deck overlays. Transp. Res. Rec. 2240 (1), 40–49. doi:10.3141/2240-07
He, Y., Zhang, X., Hooton, R. D., and Zhang, X. (2017). Effects of interface roughness and interface adhesion on new-to-old concrete bonding. Constr. Build. Mater. 151, 582–590. doi:10.1016/j.conbuildmat.2017.05.049
Hussein, L., Amleh, L., Siad, H., and Lachemi, M. (2018). Effect of very severe sulfate environment on bonded composite concrete system. Constr. Build. Mater. 191, 752–763. doi:10.1016/j.conbuildmat.2018.10.051
Hussein, L., Amleh, L., Siad, H., and Lachemi, M. (2020). Effect of severe chloride environment on the flexural behaviour of hybrid concrete systems. Mag. Concr. Res. 72 (15), 757–767. doi:10.1680/jmacr.18.00437
Jia, L., Zhao, F., Yao, K., and Du, H. (2021). Bond performance of repair mortar made with magnesium phosphate cement and ferroaluminate cement. Constr. Build. Mater. 279, 122398. doi:10.1016/j.conbuildmat.2021.122398
Jiang, C. H., Mccarthy, T. J., Chen, D., and Dong, Q. (2010). Study on the bond and anti-abrasion performance of repair materials for hydraulic concrete. Key Eng. Mater. 426, 403–408. doi:10.4028/www.scientific.net/kem.426-427.403
Jiang, C., Huang, S., Zhu, Y., Lin, Y., and Chen, D. (2016). Effect of polypropylene and basalt fiber on the behavior of mortars for repair applications. Adv. Mater. Sci. Eng. 2016, 1–11. doi:10.1155/2016/5927609
Jiang, H. (2001). Improved methode and mechanism of micro fine structure of new to old concrete repared interface layer. Shantou: Shantou University.
Jinchuan, M., Zhongwen, O., and Yahui, W. (2016). Influence of MgO and hybrid fiber on the bonding strength between reactive powder concrete and old concrete. Adv. Mater. Sci. Eng. 2016, 1–13. doi:10.1155/2016/5283908
Júlio, E. N. B. S., Branco, F. A. B., Silva, V. D., and Lourenço, J. F. (2006). Influence of added concrete compressive strength on adhesion to an existing concrete substrate. Build. Environ. 41 (12), 1934–1939. doi:10.1016/j.buildenv.2005.06.023
Kabay, N., and Kizilkanat, A. B. (2018). Mechanical properties of concrete to concrete interfaces under uniaxial and shear forces. Pamukkale Üniversitesi Mühendislik Bilim. Derg. 24 (6), 1037–1042. doi:10.5505/pajes.2018.72246
Kabiri, F. A. R. B., and Zanotti, C. (2019). Concrete–Concrete bond in mode-I: a study on the synergistic effect of surface roughness and fiber reinforcement. Appl. Sci. 9 (12), 2556. doi:10.3390/app9122556
Kao, Y. C., Chiu, C. K., Ueda, T., and Juan, Y. J. (2018). Experimental investigation on mechanical properties of sbr-modified mortar with fly ash for patch repair material. J. Adv. Concr. Technol. 16 (8), 382–395. doi:10.3151/jact.16.382
Kothari, A., Rajczakowska, M., Buasiri, T., Habermehl-Cwirzen, K., and Cwirzen, A. (2020). Eco-UHPC as repair material-bond strength, interfacial transition zone and effects of formwork type. Mater. (Basel) 13 (24), 5778. doi:10.3390/ma13245778
Kuntal, V. S., Chellapandian, M., Prakash, S. S., and Sharma, A. (2020). Experimental study on the effectiveness of inorganic bonding materials for near-surface mounting shear strengthening of prestressed concrete beams. Fibers (Basel). 8 (6), 40. doi:10.3390/fib8060040
Lacombe, P., Beaupré, D., and Pouliot, N. (1999). Rheology and bonding characteristics of self-leveling concrete as a repair material. Mater. Struct. 32 (8), 593–600. doi:10.1007/bf02480494
Lau, D. (2012). Moisture-induced debonding in concrete-epoxy interface. HKIE Trans. 19 (3), 33–38. doi:10.1080/1023697x.2012.10668995
Lau, D., and Büyüköztürk, O. (2010). Fracture characterization of concrete/epoxy interface affected by moisture. Mech. Mater. 42 (12), 1031–1042. doi:10.1016/j.mechmat.2010.09.001
Lau, D., Büyüköztürk, O., and Buehler, M. J. (2012). Characterization of the intrinsic strength between epoxy and silica using a multiscale approach. J. Mater. Res. 27 (14), 1787–1796. doi:10.1557/jmr.2012.96
Lee, S., Lee, S., Kim, G., Son, M., Choe, G., Lee, J., et al. (2020). Effect of injecting epoxy resin adhesive into cement mortar on tile adhesion performance. Appl. Sci. 10 (23), 8527. doi:10.3390/app10238527
Li, L. X., Tan, Y. B., Li, H. J., Xie, Y. J., and Fan, Z. W. (2015). Research on bond performance of self-compacted concrete for CRTS Ⅲ slab-type ballastless track. Railw. Eng. (01), 137–140. doi:10.3969/j.issn.1003-1995.2015.01.30
Li, X. P. (2004). Experimenal resaecrh on freeze thaw resistence prorerty and imperme ability for adherence of new-old concrete. Dalian: Dalian University of Technology.
Liao, W., Wang, H., Li, M., Ma, C., and Wang, B. (2019). Large scale experimental study on bond behavior between polymer modified cement mortar layer and concrete. Constr. Build. Mater. 228, 116751. doi:10.1016/j.conbuildmat.2019.116751
Liu, S. H. (2006). Discussion on repair and bond mechanism of young on old concrete [J]. Constr. Technol. 35 (8), 32–34.
Li, X., Xu, C., Du, P., Tang, Y., Zhou, C., Zhang, L., et al. (2020). The spike plays important roles in the drought tolerance as compared to the flag leaf through the phenylpropanoid pathway in wheat. Ceramics–Silikáty 64 (1), 100–111. doi:10.1016/j.plaphy.2020.05.002
Long, G. C., Ma, K. L., and Xie, Y. J. (2011). Water sorptivity and permability of new-old concrete composite system[J]. Adv. Mater. Res., 163–167. doi:10.4028/www.scientific.net/AMR.163-167.3311
Ma, Z., Zhu, F., and Ba, G. (2019). Effects of freeze-thaw damage on the bond behavior of concrete and enhancing measures. Constr. Build. Mater. 196, 375–385. doi:10.1016/j.conbuildmat.2018.11.041
Megid, W. A., and Khayat, K. H. (2017). Bond strength in multilayer casting of self-consolidating concrete. ACI Mater. J. 114 (3), 467–476. doi:10.14359/51689597
Meng, L. F., Zhu, P. N., Song, X., Miao, H. L., Ye, Y. H., et al. (2018). Study on shear performance of bonding interface between self-compacting concrete and prefabricated concrete components. Build. Struct. 48 (22), 72–77. doi:10.19701/j.jzjg.2018.22.012
Mohammadi, M., Mir Moghtadaei, R., and Ashraf Samani, N. (2014). Influence of silica fume and metakaolin with two different types of interfacial adhesives on the bond strength of repaired concrete. Constr. Build. Mater. 51, 141–150. doi:10.1016/j.conbuildmat.2013.10.048
Momayez, A., Ehsani, M. R., Rajaie, H., and Ramezanianpour, A. (2005a). Cylindrical specimen for measuring shrinkage in repaired concrete members. Constr. Build. Mater. 19 (2), 107–116. doi:10.1016/j.conbuildmat.2004.05.007
Momayez, A., Ehsani, M. R., Ramezanianpour, A. A., and Rajaie, H. (2005b). Comparison of methods for evaluating bond strength between concrete substrate and repair materials. Cem. Concr. Res. 35 (4), 748–757. doi:10.1016/j.cemconres.2004.05.027
Na, L., Yin, J., Zhang, X., and Zhou, S. Q. (2006). Application of fractal theory to study interfacial bond strength of old-new concrete[J]. J. Build. Mater. (04), 399–403. doi:10.3969/j.issn.1007-9629.2006.04.004
Qian, J., You, C., Wang, Q., Wang, H., and Jia, X. (2014). A method for assessing bond performance of cement-based repair materials. Constr. Build. Mater. 68, 307–313. doi:10.1016/j.conbuildmat.2014.06.048
Qian, P., and Xu, Q. (2018). Experimental investigation on properties of interface between concrete layers. Constr. Build. Mater. 174, 120–129. doi:10.1016/j.conbuildmat.2018.04.114
Qian, Y., Zhang, D., and Ueda, T. (2016). Interfacial tensile bond between substrate concrete and repairing mortar under freeze-thaw cycles. J. Adv. Concr. Technol. 14 (8), 421–432. doi:10.3151/jact.14.421
Qiao, Q. H., Li, Z. H., and Zhang, C. (2020). Study on bond strength of silica fume reinforced hybrid fiber cement based grouting materialand old concrete[J]. J. Funct. Mater. 51 (6), 143–149. doi:10.3969/j.issn.1001-9731.2020.06.024
Qin, J., Qian, J., You, C., Fan, Y., Li, Z., and Wang, H. (2018). Bond behavior and interfacial micro-characteristics of magnesium phosphate cement onto old concrete substrate. Constr. Build. Mater. 167, 166–176. doi:10.1016/j.conbuildmat.2018.02.018
Rashid, K., Ahmad, M., Ueda, T., Deng, J., Aslam, K., Nazir, I., et al. (2020). Experimental investigation of the bond strength between new to old concrete using different adhesive layers. Constr. Build. Mater. 249, 118798. doi:10.1016/j.conbuildmat.2020.118798
Rashid, K., Ueda, T., Zhang, D., Miyaguchi, K., and Nakai, H. (2015). Experimental and analytical investigations on the behavior of interface between concrete and polymer cement mortar under hygrothermal conditions. Constr. Build. Mater. 94, 414–425. doi:10.1016/j.conbuildmat.2015.07.035
Rashid, K., Wang, Y., and Ueda, T. (2019). Influence of continuous and cyclic temperature durations on the performance of polymer cement mortar and its composite with concrete. Compos. Struct. 215, 214–225. doi:10.1016/j.compstruct.2019.02.057
Santos, D. S., Santos, P. M. D., and Dias-Da-Costa, D. (2012). Effect of surface preparation and bonding agent on the concrete-to-concrete interface strength. Constr. Build. Mater. 37, 102–110. doi:10.1016/j.conbuildmat.2012.07.028
Santos, P. M. D., and Júlio, E. N. B. S. (2011). Factors affecting bond between new and old concrete[J]. ACI Mater. J. 108 (4), 449.
Santos, P. M. D., Júlio, E. N. B. S., and Silva, V. T. D. (2007). Correlation between concrete-to-concrete bond strength and the roughness of the substrate surface. Constr. Build. Mater. 21 (8), 1688–1695. doi:10.1016/j.conbuildmat.2006.05.044
Scherer, G. W. (2004). Stress from crystallization of salt. Cem. Concr. Res. 34 (9), 1613–1624. doi:10.1016/j.cemconres.2003.12.034
Shi, C. H. (2018). A new method and its fractal expression for evaluating roughness of concrete[J]. Bull. Chin. Ceram. Soc. 37 (03), 924–933. doi:10.16552/j.cnki.issn1001-1625.2018.03.028
Silfwerbrand, J. (2003). Shear bond strength in repaired concrete structures. Mater. Struct. 36 (6), 419–424. doi:10.1617/13859
Silfwerbrand, J., Beushausen, H., and Courard, L. (2011). Bonded cement-based material overlays for the repair, the lining or the strengthening of slabs or pavements: state-of-the-art report of the RILEM technical committee 193-RLS. New York: Springer Netherlands, 51–79.
Tatar, J., and Hamilton, H. R. (2014). “Effect of moisture exposure on the adhesive bond under direct shear,” in Proceedings of the Proc. 7th Int. Conf. on FRP Composites in Civil Engineering (CICE 2014), International Institute for FRP in Construction, Vancouver, Canada.
Tayeh, B. A., Abu Bakar, B. H., Megat Johari, M. A., and Voo, Y. L. (2012). Mechanical and permeability properties of the interface between normal concrete substrate and ultra high performance fiber concrete overlay. Constr. Build. Mater. 36, 538–548. doi:10.1016/j.conbuildmat.2012.06.013
Tian, W. L., Zhu, R. J., Xia, M. Y., Xiao, C. Z., Liu, X., et al. (2018). Experiment research on shear behavior of new and old concrete bonding interface[J]. Archit. Technol. 49 (1), 33–36.
Ueda, T. (2019). Material mechanical properties necessary for the structural intervention of concrete structures. Eng. 5 (6), 1131–1138. doi:10.1016/j.eng.2019.02.012
Valikhani, A., Jahromi, A. J., Mantawy, I. M., and Azizinamini, A. (2020). Experimental evaluation of concrete-to-UHPC bond strength with correlation to surface roughness for repair application. Constr. Build. Mater. 238, 117753. doi:10.1016/j.conbuildmat.2019.117753
Wan, B., Petrou, M. F., and Harries, K. A. (2006). The effect of the presence of water on the durability of bond between CFRP and concrete. J. Reinf. Plastics Compos. 25 (8), 875–890. doi:10.1177/0731684406065140
Wang, B., and Gupta, R. (2021). Analyzing bond-deterioration during freeze-thaw exposure in cement-based repairs using non-destructive methods. Cem. Concr. Compos. 115, 103830. doi:10.1016/j.cemconcomp.2020.103830
Wang, B., Li, Q., Liu, F., Wang, J., and Xu, S. (2018). Shear bond assessment of UHTCC repair using push-out test. Constr. Build. Mater. 164, 206–216. doi:10.1016/j.conbuildmat.2017.12.148
Wang, B., Xu, S., and Liu, F. (2016). Evaluation of tensile bonding strength between UHTCC repair materials and concrete substrate. Constr. Build. Mater. 112, 595–606. doi:10.1016/j.conbuildmat.2016.02.149
Wang, R., and Zhang, L. (2015). Mechanism and durability of repair systems in polymer-modified cement mortars. Adv. Mater. Sci. Eng. 2015, 1–8. doi:10.1155/2015/594672
Xiong, G., Liu, J., Li, G., and Xie, H. (2002). A way for improving interfacial transition zone between concrete substrate and repair materials. Cem. Concr. Res. 32 (12), 1877–1881. doi:10.1016/s0008-8846(02)00840-2
Yi, C., Lai, S. L., Zhu, H. G., Yan, S. H., Liu, J. X., and Dong, X. (2013). Freeze-thawing damage model of new-to-old concrete with different rough interfaces. Appl. Mech. Mater. 405-408, 2707–2714. doi:10.4028/www.scientific.net/amm.405-408.2707
Yuan, Q., Long, G., Liu, Z., Ma, K., Xie, Y., Deng, D., et al. (2016). Sealed-space-filling SCC: a special SCC applied in high-speed rail of China. Constr. Build. Mater. 124, 167–176. doi:10.1016/j.conbuildmat.2016.07.093
Zanotti, C., Rostagno, G., and Tingley, B. (2018). Further evidence of interfacial adhesive bond strength enhancement through fiber reinforcement in repairs. Constr. Build. Mater. 160, 775–785. doi:10.1016/j.conbuildmat.2017.12.140
Zhang, D., Ueda, T., and Furuuchi, H. (2013). Fracture mechanisms of polymer cement mortar: concrete interfaces. J. Eng. Mech. 139 (2), 167–176. doi:10.1061/(asce)em.1943-7889.0000486
Zhang, J., Ding, X., and Zhao, Q. (2017). Experimental and numerical investigation of scattering gravels on the surface bond strength of self-compacting concrete. Constr. Build. Mater. 145, 11–19. doi:10.1016/j.conbuildmat.2017.03.219
Zhang, X., Zhang, W., Luo, Y., Wang, L., Peng, J., and Zhang, J. (2020b). Interface shear strength between self-compacting concrete and carbonated concrete. J. Mater. Civ. Eng. 32 (6), 04020113. doi:10.1061/(asce)mt.1943-5533.0003229
Zhang, Y., Zhu, P., Liao, Z., and Wang, L. (2020a). Interfacial bond properties between normal strength concrete substrate and ultra-high performance concrete as a repair material. Constr. Build. Mater. 235, 117431. doi:10.1016/j.conbuildmat.2019.117431
Zheng, J. L., and Chen, F. (2008). The plastic limit analysis of adhesive shear strength between self-compacting concrete and old concrete [J]. Eng. Mech. 25 (8), 164–168.
Zheng, Z., Li, Y., Ma, X., Zhu, X., and Li, S. (2019). High density and high strength cement-based mortar by modification with epoxy resin emulsion. Constr. Build. Mater. 197, 319–330. doi:10.1016/j.conbuildmat.2018.11.167
Keywords: interface, bonding properties, adhesion agent, repairing materials, environment factors
Citation: Liu H, Zou H, Zhang J, Zhang J, Tang Y, Zhang J, Guo Y and Xiao J (2024) Interface bonding properties of new and old concrete: A review. Front. Mater. 11:1389785. doi: 10.3389/fmats.2024.1389785
Received: 22 February 2024; Accepted: 07 March 2024;
Published: 12 April 2024.
Edited by:
Mingjun Hu, Dalian University of Technology, ChinaReviewed by:
Ning Li, Qingdao University of Technology, ChinaWenxu Li, Jiangsu University of Science and Technology, China
Copyright © 2024 Liu, Zou, Zhang, Zhang, Tang, Zhang, Guo and Xiao. This is an open-access article distributed under the terms of the Creative Commons Attribution License (CC BY). The use, distribution or reproduction in other forums is permitted, provided the original author(s) and the copyright owner(s) are credited and that the original publication in this journal is cited, in accordance with accepted academic practice. No use, distribution or reproduction is permitted which does not comply with these terms.
*Correspondence: He Liu, Heliu@sjzu.edu.cn; Jingyi Zhang, dq_zjy@syucu.edu.cn