- 1Key Laboratory of Interface Science and Engineering in Advanced Materials, Ministry of Education, Taiyuan University of Technology, Taiyuan, China
- 2College of New Materials and New Energies, Shenzhen Technology University, Shenzhen, China
The poly3,4-ethylenedioxythiophene) poly(styrene sulfonate) (PEDOT:PSS) hole transport layer (HTL) has always played a crucial role in achieving high-efficiency organic solar cells (OSCs) owing to its unique advantages of suitable energy levels and high optical transparency. However, the inherent insulation and easy aggregation property of PSS results in relatively low conductivity and high surface roughness of the PEDOT:PSS film, which is unfavorable for charge transport and the morphology of the top layer. To address these problems, we use PEDOT:PSS diluted in a series of alcoholic solvents and evaluate them on the PM6:Y6 system. Among these, the PM6:Y6 devices using PEDOT:PSS modified with ethanol as the HTL demonstrate the best vertical phase segregation and carrier extraction. In addition, the PEDOT:PSS film with added ethanol also has the smoothest surface compared to those diluted in the other alcoholic solvents. Finally, a high power conversion efficiency of 18.13% was obtained with the PM6:Y6 devices based on PEDOT:PSS modified by ethanol. This work provides a sufficient reference for the alcoholic modification of PEDOT:PSS and also proposes a feasible solution for high-efficiency OSCs.
1 Introduction
Organic solar cells (OSCs) have attracted extensive attention for solving the energy problem owing to their advantages, such as light weight, semitransparency, flexibility, and roll-to-roll production (Lin et al., 2015; Li et al., 2016; Lin et al., 2017; Zhao et al., 2017; Hou et al., 2018; Li et al., 2018; Li et al., 2024; Peng et al., 2024). Recently, the power conversion efficiency (PCE) of OSCs has improved significantly upon the emergence of non-fullerene acceptors that are matched well with the absorption spectra and energy levels of the donor (Sonar et al., 2011; Lin and Zhan, 2014; Cheng et al., 2018; Hou et al., 2018; Yan et al., 2018). Through interface modifications, semiconductor material design, and morphological optimization, the PCE of OSCs has exceeded 19% (Cui et al., 2021; Li et al., 2021; Zhang et al., 2021; Chong et al., 2022; Sun et al., 2022; Zhu et al., 2022).
Suitable interface modifications can enhance charge extraction and suppress carrier recombination, thereby resulting in large photovoltages and photocurrent densities (Cheng et al., 2009; Zhang et al., 2018; Zhao et al., 2018; Zhu et al., 2021; Zhou X. M. et al., 2022; Liao et al., 2022; Yu et al., 2022; Zheng et al., 2022). The currently available high-efficiency OSCs are based on the conductive polymer poly(3,4-ethylenedioxy-thiophene) poly(styrene-sulfonate) (PEDOT:PSS) as the hole transport layer (HTL) because of its suitable energy levels, good optical transparency, and solution processability. In addition, the large work function of PEDOT:PSS (∼5.1 eV) approaches the energy level of the least unoccupied molecular orbital of the most polymeric donor material, which is conductive enough to form good ohmic contacts at the anode/active layer interface and enhance hole transport efficiency (Potscavage et al., 2009; Bouthinon et al., 2015; Xu et al., 2020; Zhang et al., 2020; Zhu et al., 2021). However, there are also some drawbacks to using PEDOT:PSS, such as its relatively moderate conductivity, strong acidity, and structural inhomogeneities caused by the insulating PSS structure, which affect the photovoltaic performance and stability of the device (Kemerink et al., 2004; Lee et al., 2014; Shi et al., 2015; Zeng et al., 2020; Zhou K. K. et al., 2022; Bertrandie et al., 2022).
It has been reported that isopropanol (IPA) can adjust the morphology, charge transport, and optical properties of a PEDOT:PSS film, thereby significantly improving its hole extraction and transport efficiencies (Ouyang, 2013; Donoval et al., 2017; Aimukhanov et al., 2021). Moreover, isopropanol can also dissolve the insulating PSS portion in PEDOT:PSS, thereby hindering the aggregation of PSS and leading to a higher PCE in OSCs (Mengistie et al., 2015; Zhu et al., 2016; Aimukhanov et al., 2021; Cassinelli et al., 2021). Nevertheless, research on the effects of the types of alcohols used with the PEDOT:PSS solution on the photovoltaic performances of OSCs remains insufficient. Moreover, the effects of alcohol-modified PEDOT:PSS on the vertical component distribution of the active layer are not yet clear, seriously limiting the application of PEDOT:PSS to high-efficiency OSCs in the future.
Herein, we selected methanol, ethanol, and IPA as the diluents for the PEDOT:PSS solution to enhance the photovoltaic performances of the OSCs. Based on the transient photovoltage (TPV) and transient photocurrent (TPC) measurements, the effects of alcoholic solvent dilution of PEDOT:PSS on the carrier recombinations and extraction process in devices were studied thoroughly. In addition, film-depth-dependent light absorption spectroscopy (FDDLAS) was employed to understand the phase component information in the active layer. The results of the investigations indicate that PM6:Y6 devices based on ethanol-modified PEDOT:PSS HTLs enable faster charge extractions and suitable phase distributions, which are conducive to efficient carrier and lower charge combinations. Thus, the corresponding OSCs exhibit a maximum PCE of 18.13%, which is much higher than the 17.42% of the control OSCs.
2 Materials and methods
PM6 and Y6 were purchased from Solarmer Material Inc. (Beijing, China), PNDIT-F3N was purchased from eFlexPV Limited (Guangdong, China), and PEDOT:PSS (Clevios PVP 4083) was purchased from Heraeus Inc. (Hanau, Germany). All of the other reagents and chemicals were purchased from Sigma-Aldrich or Aladdin and used as received.
3 Results and discussion
The schematics of the device structure and chemical structure of the photoactive layer are shown in Figures 1A, B, respectively. Clearly, the polymer donor PM6 and non-fullerene acceptor Y6 are selected as active layers, and the corresponding device architecture was stacked in the form of ITO/HTL/PM6:Y6/PNDIT-F3N/Ag. The related chemical structures of PEDOT:PSS and PNDIT-F3N are shown in Supplementary Figure S1. Subsequently, the current density vs. voltage (J–V) curves of OSCs with four different HTLs were measured under AM 1.5 G illumination at 100 mW cm−2, as shown in Figure 1C. The extracted photovoltaic performance parameters from the J–V curves are summarized in Table 1. When the pure PEDOT:PSS was used as the HTL, a relatively low short-circuit current density (JSC) of 27.27 mA cm-2 and poor fill factor (FF) of 76.00% were obtained, which resulted in a low PCE of 17.42%. When the alcoholic solvents (CH3OH, EtOH, and IPA) were added to PEDOT:PSS, the PCE of the OSCs may be increased by the enhanced JSC and large FF. The detailed optimizations of the volume ratio between PEDOT:PSS and the alcoholic solvent are shown in Supplementary Figure S2 and Supplementary Table S1. For the PEDOT:PSS:CH3OH cast device, the JSC and FF reached 27.85 mA cm-2 and 77.19%, respectively, resulting in a high PCE of 17.94%. When EtOH was introduced into the PEDOT:PSS solution, the FF of the device was greatly improved from 76.00% (PEDOT:PSS) to 78.66% (PEDOT:PSS:EtOH), resulting in an increased PCE of 18.15%. After deposition of PEDOT:PSS:IPA onto the ITO electrode, the PCE of the OSCs based on the PM6:Y6 system increased to 17.86%, which is a result of the modest increments in JSC and FF (27.32 mA cm-2 and 78.23%). Moreover, the external quantum efficiency (EQE) spectra of the OSCs with different HTLs were measured, as shown in Figure 1D. It can be seen that the EQE spectra of the devices equipped with PEDOT:PSS along with added alcoholic solvents exhibit remarkable enhancements in the range of 400–900 nm compared to the control device using pure PEDOT:PSS as the HTL. The stabilities of OSCs with different HTLs were measured in an N2 atmosphere (Supplementary Figure S3). After 600 h of storage, the PCEs of the devices with PEDOT:PSS modified by alcoholic solvents retain above 95% of their initial values, higher than that of the control device (92.9%). In particular, the PCE of the OSCs with PEDOT:PSS:EtOH as the HTL could still reach 96.3% of that of the pristine device after 600 h of exposure in the N2 atmosphere.
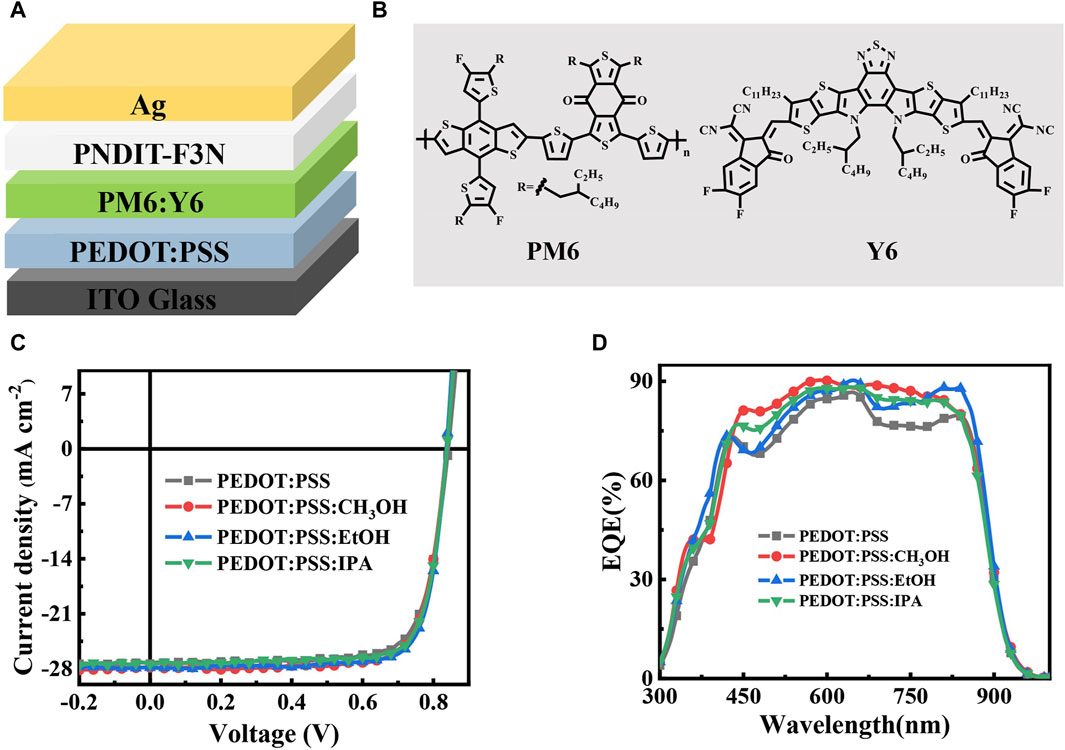
Figure 1. (A) Schematic of a conventional organic solar cell (OSC). (B) Chemical structures of the active layer materials used in this work. (C) J–V characteristics and (D) external quantum efficiency curves of the devices with four hole transport layers (HTLs).

Table 1. Extracted performance parameters of the OSCs equipped with different HTLs under AM 1.5 G illumination at 100 mW cm−2.
To explore the reasons behind the improved photovoltaic performance, the TPV and TPC curves of the OSCs were analyzed for the effects of these HTLs on carrier recombinations and extraction processes (Figure 2A; Figure 2B). Based on the TPV curve, the extracted carrier lifetime (τlifetime) values of the devices are 3.056 μs (PEDOT:PSS), 3.232 μs (PEDOT:PSS:CH3OH), 3.338 μs (PEDOT:PSS:EtOH), and 3.433 μs (PEDOT:PSS:IPA). From Figure 2B, the related charge extraction time constant (τextract) values are 0.227 μs (PEDOT:PSS), 0.203 μs (PEDOT:PSS:CH3OH), 0.182 μs (PEDOT:PSS:EtOH), and 0.226 μs (PEDOT:PSS:IPA). After adding EtOH to PEDOT:PSS, the device exhibits the shortest τextract and suitable τlifetime as compared to the other OSCs, indicating reduced carrier loss and effective extraction.
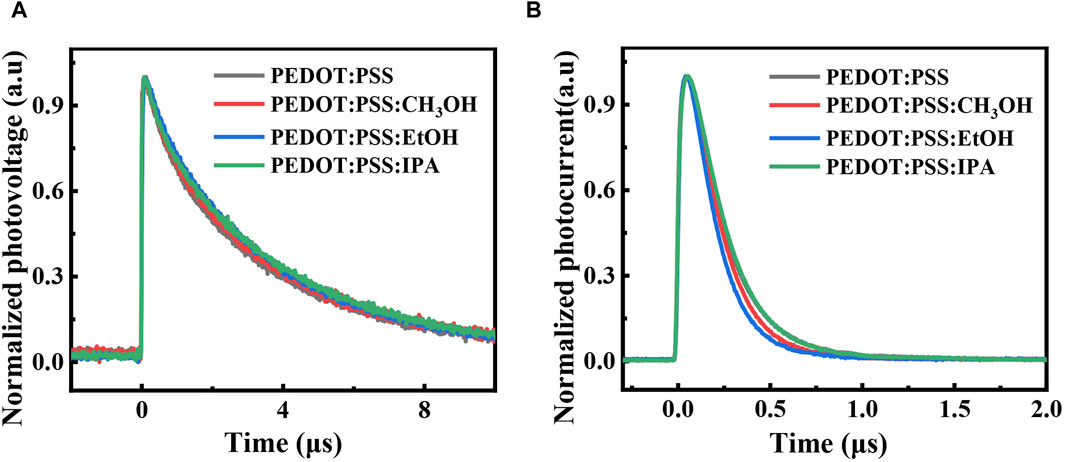
Figure 2. (A) Transient photovoltage and (B) transient photocurrent curves of the devices with HTL treatments.
FDDLAS was used to obtain the vertical distribution information of the donors and acceptors in the OSCs to analyze the effects of the HTL on the vertical gradient in the active layer (Bu et al., 2016; Wang et al., 2019). The FDDLAS data of various PM6:Y6 films with different HTLs are shown in Supplementary Figure S4. Furthermore, the vertical distributions of PM6 and Y6 were extracted by fitting the FDDLAS data with the absorption values of the PM6 and Y6 films, as displayed in Figures 3A–D. It is observed that the HTLs are able to adjust the composition distributions of the PM6:Y6 film in the top (0–30 nm) and bottom (90–100 nm) regions. Concretely, the proportions of PM6 in the active layers of the films near the HTL interfaces were 52.22% (PEDOT:PSS), 54.72% (PEDOT:PSS:CH3OH), 66.89% (PEDOT:PSS:EtOH), and 51.38% (PEDOT:PSS:IPA). The PEDOT:PSS:EtOH/PM6:Y6 structure thus achieves the highest PM6 content near the HTL interface and an optimal Y6 content near the electron transport layer (ETL) interface, which is conducive for enhancing hole transfer and reducing charge recombination at the interface between the active layer and electrode.
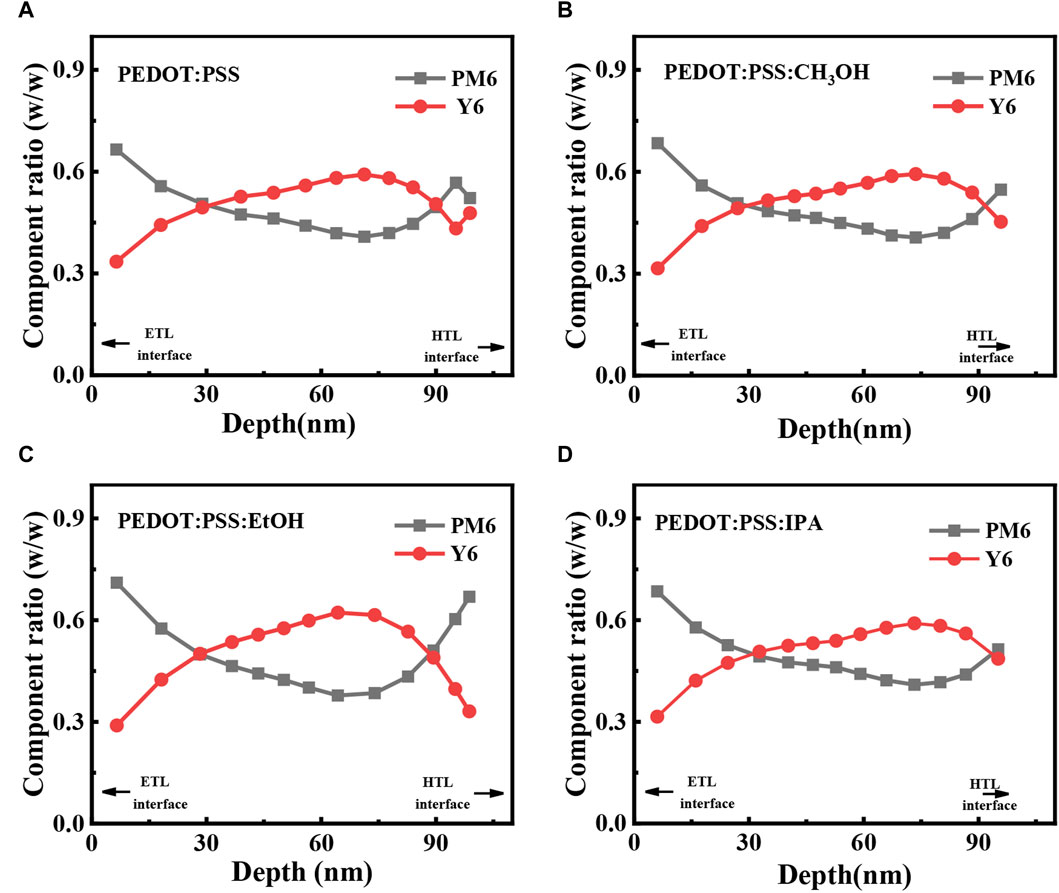
Figure 3. (A–D) Composition ratios along the vertical directions of the active layer films by film-depth-dependent light absorption spectroscopy (FDDLAS).
By combining the optical interferences of the different layers in the device, the exciton distribution profile was also extracted from the FDDLAS data based on the modified optical transfer matrix model (Figures 4A–D) (Bu et al., 2016; Gao et al., 2017). It can be seen that almost all the excitons generated by the PM6 donor (absorption at 500–620 nm) are distributed within the depth range of 0–50 nm for all sample films. However, this phenomenon is modestly different for the excitons produced by the Y6 acceptor in the blend film. For the PM6:Y6 films based on the PEDOT:PSS, PEDOT:PSS:CH3OH, and PEDOT:PSS:IPA HTLs, significant numbers of the excitons generated by the Y6 acceptor (absorption at 620–900 nm) were observed on the surfaces of the blend films near the ETL regions (depth at 0–10 nm). This implies that the free holes generated after exciton separation must travel a greater distance to reach the HTL interface, which could damage the efficiency of electron transport. When EtOH was added to PEDOT:PSS, the exciton-rich region near the surface generated by Y6 shifted from the top to the middle region in the blend film, indicating ameliorative transport of the free electrons generated by exciton separation.
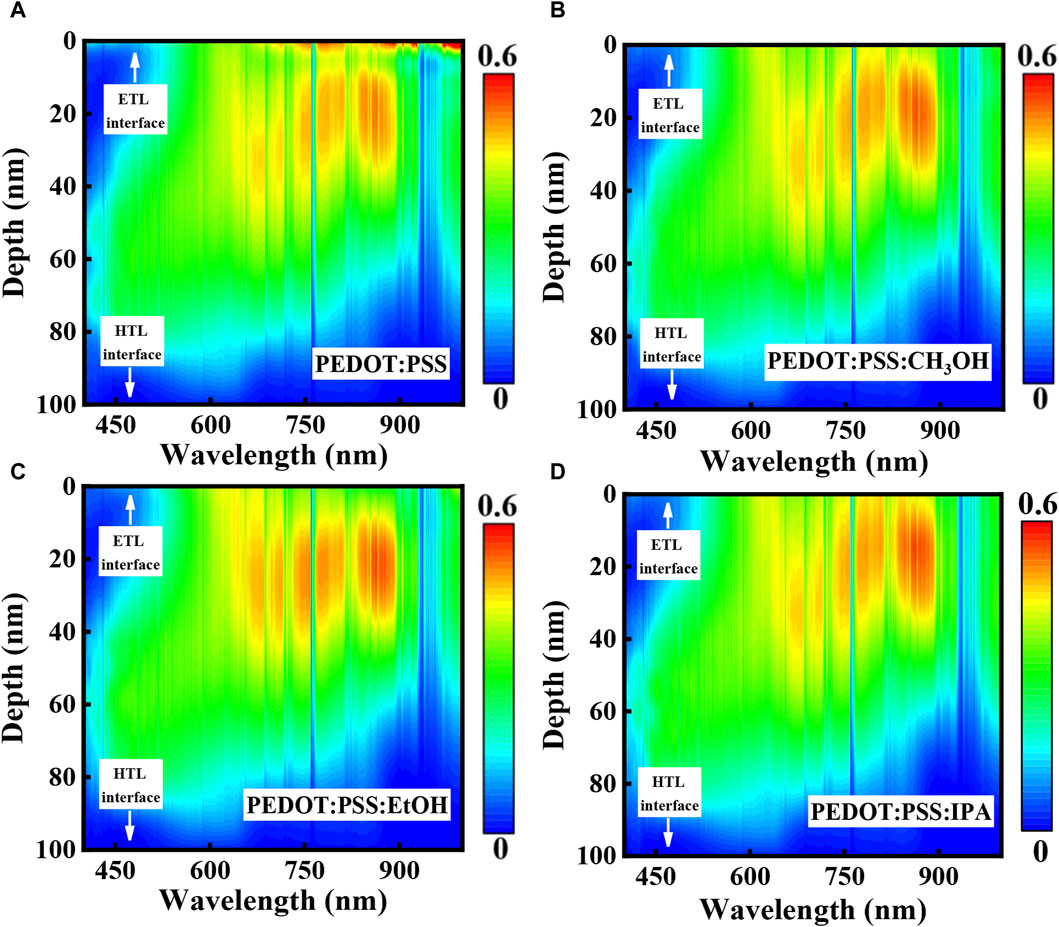
Figure 4. (A–D) Exciton generation maps along the vertical directions of the ITO/HTL/PM6:Y6/PNDIT-F3N/Ag devices; the unit of the scale bar is “nm−3 nm−1 s−1.” The ETL and HTL stand for the electron transport layer and hole transport layer, respectively.
To study the influences of the alcoholic solvents on the morphology of PEDOT:PSS in greater depth, the height images of the ITO/HTL films were tested by atomic force microscopy (AFM) using the tapping mode. As shown in Figure 5, the root mean-squared (Rq) values of the heights in these ITO/HTL films are 1.54 nm (PEDOT:PSS), 1.43 nm (PEDOT:PSS:CH3OH), 1.39 nm (PEDOT:PSS:EtOH), and 1.48 nm (PEDOT:PSS:IPA). These results indicate that the use of alcoholic solvents can reduce the roughness of PEDOT:PSS and facilitate good interfacial contact with the active layer.
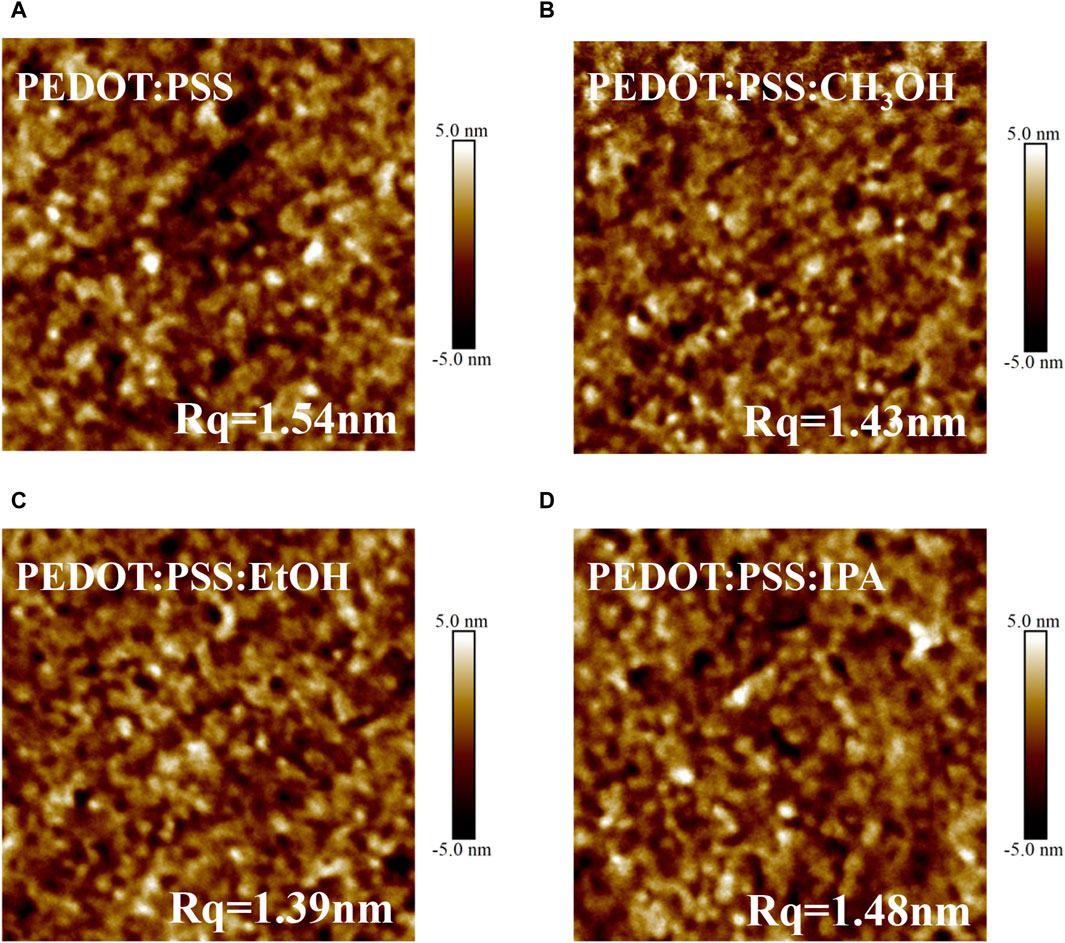
Figure 5. (A-D) Atomic force microscopy height images of the ITO substrates modified by different HTLs.
4 Conclusion
In this study, we propose a method of improving the photovoltaic performances of OSCs using diluted PEDOT:PSS with alcoholic solvents as the HTLs. The PCEs of the PM6:Y6 devices with alcohol-modified PEDOT:PSS as the HTLs are 17.94% (PEDOT:PSS:CH3OH), 18.13% (PEDOT:PSS:EtOH), and 17.86% (PEDOT:PSS:IPA), which are higher than that of OSCs based on the traditional PEDOT:PSS (17.42%). The optimal photovoltaic performance of the device with the PEDOT:PSS:EtOH HTL was attributable to the smooth surface morphology and high PM6 content near the HTL interface, which could achieve a suitable carrier lifetime and fast charge extraction. This study shows that diluting PEDOT:PSS with alcoholic solvents is an effective method of deriving high-efficiency OSCs.
Data availability statement
The original contributions presented in the study are included in the article/Supplementary Material, and any further inquiries may be directed to the corresponding authors.
Author contributions
TH: data curation, methodology, and writing–original draft. CG: data curation, formal analysis, and writing–review and editing. KS: data curation, methodology, and writing–original draft. LZ: investigation, software, and writing–original draft. LW: investigation, methodology, and writing–review and editing. WL: investigation, formal analysis, and writing–original draft. YM: conceptualization, data curation, funding acquisition, methodology, and writing–review and editing. YW: data curation, methodology, formal analysis, investigation, and writing–original draft. MQ: formal analysis, methodology, supervision, and writing–original draft. SL: formal analysis, funding acquisition, investigation, supervision, and writing–review and editing. GZ: data curation, funding acquisition, supervision, and writing–review and editing.
Funding
The author(s) declare that financial support was received for the research, authorship, and/or publication of this article. The work was supported by the Guangdong Basic and Applied Basic Research Foundation (grant nos. 2022A1515010875 and 2021A1515110017), Natural Science Foundation of Top Talent of SZTU (grant no. 20200205), Project of Education Commission of Guangdong Province of China (grant no. 2021KQNCX080), Research on the Electrochemical Reaction Mechanism of the Anode of Medium-Low Temperature Direct Ammonia SOFCs (grant no. 20231063020006), Project of All Solid-State High Energy Density Energy Storage System (grant no. 20221063010031), and Shenzhen Key Laboratory of Applied Technologies of Super-Diamond and Functional Crystals (ZDSYS20230626091303007). SL would like to acknowledge support from the Guangdong Basic and Applied Basic Research Foundation (grant no. 2019A1515011673), Education Department of Guangdong Province (grant no. 2021KCXTD045), and National Natural Science Foundation of China (grant no. 12274303). MQ thanks the Characteristic Innovation Foundation of Higher Education Institutions of Guangdong Province (grant no. 2022KTSCX116) and Industry University Cooperation and Collaborative Education Project of Ministry of Education (grant no. 220600480203919). YM thanks the Science and Technology Innovation Talent Team Project of Shanxi Province (grant no. 202204051001013).
Acknowledgments
All authors thank the Analysis and Testing Center of Shenzhen Technology University for their support with the AFM measurements.
Conflict of interest
The authors declare that the research was conducted in the absence of any commercial or financial relationships that could be construed as a potential conflict of interest.
Publisher’s note
All claims expressed in this article are solely those of the authors and do not necessarily represent those of their affiliated organizations or those of the publisher, editors, and reviewers. Any product that may be evaluated in this article or claim that may be made by its manufacturer is not guaranteed or endorsed by the publisher.
Supplementary material
The Supplementary Material for this article can be found online at: https://www.frontiersin.org/articles/10.3389/fmats.2024.1383816/full#supplementary-material
References
Aimukhanov, A. K., Rozhkova, X. S., Ilyassov, B. R., Zeinidenov, A. K., and Nuraje, N. (2021). The influence of structural and charge transport properties of PEDOT:PSS layers on the photovoltaic properties of polymer solar cells. Polym. Adv. Technol. 32 (2), 497–504. doi:10.1002/pat.5102
Bertrandie, J., Sharma, A., Gasparini, N., Villalva, D. R., Paleti, S. H. K., Wehbe, N., et al. (2022). Air-processable and thermally stable hole transport layer for non-fullerene organic solar cells. ACS Appl. Energ Mater 5 (1), 1023–1030. doi:10.1021/acsaem.1c03378
Bouthinon, B., Clerc, R., Vaillant, J., Verilhac, J. M., Faure-Vincent, J., Djurado, D., et al. (2015). Impact of blend morphology on interface state recombination in bulk heterojunction organic solar cells. Adv. Funct. Mater 25 (7), 1090–1101. doi:10.1002/adfm.201401633
Bu, L. J., Gao, S., Wang, W. C., Zhou, L., Feng, S., Chen, X., et al. (2016). Film-depth-dependent light absorption and charge transport for polymer electronics: a case study on semiconductor/insulator blends by plasma etching. Adv. Electron Mater 2 (12), 1600359. doi:10.1002/aelm.201600359
Cassinelli, M., Park, W. T., Kim, Y., Kim, J. H., Noh, Y. Y., and Caironi, M. (2021). Rationalizing the enhancement of the thermoelectric properties of PEDOT:PSS by secondary doping. Appl. Phys. Lett. 119 (3), 033301. doi:10.1063/5.0054477
Cheng, P., Li, G., Zhan, X. W., and Yang, Y. (2018). Next-generation organic photovoltaics based on non-fullerene acceptors. Nat. Photonics 12 (3), 131–142. doi:10.1038/s41566-018-0104-9
Cheng, Y. J., Yang, S. H., and Hsu, C. S. (2009). Synthesis of conjugated polymers for organic solar cell applications. Chem. Rev. 109 (11), 5868–5923. doi:10.1021/cr900182s
Chong, K. E., Xu, X. P., Meng, H. F., Xue, J. W., Yu, L. Y., Ma, W., et al. (2022). Realizing 19.05% efficiency polymer solar cells by progressively improving charge extraction and suppressing charge recombination. Adv. Mater 34 (13), 2109516. doi:10.1002/adma.202109516
Cui, Y., Xu, Y., Yao, H. F., Bi, P. Q., Hong, L., Zhang, J. Q., et al. (2021). Single-Junction organic photovoltaic cell with 19% efficiency. Adv. Mater 33 (41), 2102420. doi:10.1002/adma.202102420
Donoval, M., Micjan, M., Novota, M., Nevrela, J., Kovacova, S., Pavuk, M., et al. (2017). Relation between secondary doping and phase separation in PEDOT:PSS films. Appl. Surf. Sci. 395, 86–91. doi:10.1016/j.apsusc.2016.05.076
Gao, S., Bu, L. J., Zheng, Z., Wang, X. D., Wang, W. C., Zhou, L., et al. (2017). Probing film-depth-related light harvesting in polymer solar cells via plasma etching. AIP Adv. 7 (4), 045312. doi:10.1063/1.4982242
Hou, J. H., Inganäs, O., Friend, R. H., and Gao, F. (2018). Organic solar cells based on non-fullerene acceptors. Nat. Mater 17 (2), 119–128. doi:10.1038/nmat5063
Kemerink, M., Timpanaro, S., de Kok, M. M., Meulenkamp, E. A., and Touwslager, F. J. (2004). Three-dimensional inhomogeneities in PEDOT:PSS films. J. Phys. Chem. B 108 (49), 18820–18825. doi:10.1021/jp0464674
Lee, S. J., Kim, H. P., Yusoff, A., and Jang, J. (2014). Organic photovoltaic with PEDOT:PSS and V2O5 mixture as hole transport layer. Sol. Energy Mater Sol. Cells 120, 238–243. doi:10.1016/j.solmat.2013.09.009
Li, C., Zhou, J. D., Song, J. L., Xu, J. Q., Zhang, H. T., Zhang, X. N., et al. (2021). Non-fullerene acceptors with branched side chains and improved molecular packing to exceed 18% efficiency in organic solar cells. Nat. Energy 6 (6), 605–613. doi:10.1038/s41560-021-00820-x
Li, S. M., Gao, M. Y., Zhou, K. K., Li, M., Xian, K., Zhao, W. C., et al. (2024). Achieving record-high stretchability and mechanical stability in organic photovoltaic blends with a dilute-absorber strategy. Adv. Mater 36 (8), 2307278. doi:10.1002/adma.202307278
Li, S. X., Zhan, L. L., Liu, F., Ren, J., Shi, M. M., Li, C. Z., et al. (2018). An unfused-core-based nonfullerene acceptor enables high-efficiency organic solar cells with excellent morphological stability at high temperatures. Adv. Mater 30 (6), 1705208. doi:10.1002/adma.201705208
Li, Z. K., Jiang, K., Yang, G. F., Lai, J. Y. L., Ma, T. X., Zhao, J. B., et al. (2016). Donor polymer design enables efficient non-fullerene organic solar cells. Nat. Commun. 7, 13094. doi:10.1038/ncomms13094
Liao, Q., Kang, Q., Yang, Y., Zheng, Z., Qin, J. Z., Xu, B. W., et al. (2022). Highly stable organic solar cells based on an ultraviolet-resistant cathode interfacial layer. CCS Chem. 4 (3), 938–948. doi:10.31635/ccschem.021.202100852
Lin, Y. B., Cai, C. S., Zhang, Y. D., Zheng, W. H., Yang, J. Y., Wang, E. G., et al. (2017). Study of ITO-free roll-to-roll compatible polymer solar cells using the one-step doctor blading technique. J. Mater Chem. A 5 (8), 4093–4102. doi:10.1039/c6ta10018j
Lin, Y. Z., Wang, J. Y., Zhang, Z. G., Bai, H. T., Li, Y. F., Zhu, D. B., et al. (2015). An electron acceptor challenging fullerenes for efficient polymer solar cells. Adv. Mater 27 (7), 1170–1174. doi:10.1002/adma.201404317
Lin, Y. Z., and Zhan, X. W. (2014). Non-fullerene acceptors for organic photovoltaics: an emerging horizon. Mater Horizons 1 (5), 470–488. doi:10.1039/c4mh00042k
Mengistie, D. A., Chen, C. H., Boopathi, K. M., Pranoto, F. W., Li, L. J., and Chu, C. W. (2015). Enhanced thermoelectric performance of PEDOT:PSS flexible bulky papers by treatment with secondary dopants. ACS Appl. Mater Interfaces 7 (1), 94–100. doi:10.1021/am507032e
Ouyang, J. (2013). Secondary doping‘ methods to significantly enhance the conductivity of PEDOT:PSS for its application as transparent electrode of optoelectronic devices. Displays 34 (5), 423–436. doi:10.1016/j.displa.2013.08.007
Peng, Z. X., Li, S. M., Zhou, K. K., Zhang, Y. W., Li, M. F., Li, M., et al. (2024). Unveiling the strain-induced microstructural evolution and morphology-stretchability correlations of intrinsically stretchable organic photovoltaic films. Adv. Mater, 2304286. doi:10.1002/aenm.202304286
Potscavage, W. J., Sharma, A., and Kippelen, B. (2009). Critical interfaces in organic solar cells and their influence on the open-circuit voltage. Accounts Chem. Res. 42 (11), 1758–1767. doi:10.1021/ar900139v
Shi, H., Liu, C. C., Jiang, Q. L., and Xu, J. K. (2015). Effective approaches to improve the electrical conductivity of PEDOT:PSS: a review. Adv. Electron Mater 1 (4), 1500017. doi:10.1002/aelm.201500017
Sonar, P., Lim, J. P. F., and Chan, K. L. (2011). Organic non-fullerene acceptors for organic photovoltaics. Energy Environ. Sci. 4 (5), 1558–1574. doi:10.1039/c0ee00668h
Sun, R., Wu, Y., Yang, X. R., Gao, Y., Chen, Z., Li, K., et al. (2022). Single-Junction organic solar cells with 19.17% efficiency enabled by introducing one asymmetric guest acceptor. Adv. Mat. 34 (26), 2110147. doi:10.1002/adma.202110147
Wang, Z. H., Hu, Y. P., Xiao, T., Zhu, Y. W., Chen, X., Bu, L. J., et al. (2019). Correlations between performance of organic solar cells and film-depth-dependent optical and electronic variations. Adv. Opt. Mater 7 (10), 1900152. doi:10.1002/adom.201900152
Xu, H. T., Yuan, F., Zhou, D., Liao, X. F., Chen, L., and Chen, Y. W. (2020). Hole transport layers for organic solar cells: recent progress and prospects. J. Mater Chem. A 8 (23), 11478–11492. doi:10.1039/d0ta03511d
Yan, C. Q., Barlow, S., Wang, Z. H., Yan, H., Jen, A. K. Y., Marder, S. R., et al. (2018). Non-fullerene acceptors for organic solar cells. Nat. Rev. Mater 3 (3), 18003. doi:10.1038/natrevmats.2018.3
Yu, R. N., Wei, X. Q., Wu, G. Z., Zhang, T., Gong, Y. S., Zhao, B. A., et al. (2022). Efficient interface modification via multi-site coordination for improved efficiency and stability in organic solar cells. Energy Environ. Sci. 15 (2), 822–829. doi:10.1039/d1ee03263a
Zeng, M., Wang, X. J., Ma, R. J., Zhu, W. Y., Li, Y., Chen, Z. X., et al. (2020). Dopamine semiquinone radical doped PEDOT:PSS: enhanced conductivity, work function and performance in organic solar cells. Adv. Energy Mater 10 (25), 2000743. doi:10.1002/aenm.202000743
Zhang, G. Y., Zhao, J. B., Chow, P. C. Y., Jiang, K., Zhang, J. Q., Zhu, Z. L., et al. (2018). Nonfullerene acceptor molecules for bulk heterojunction organic solar cells. Chem. Rev. 118 (7), 3447–3507. doi:10.1021/acs.chemrev.7b00535
Zhang, H., Li, Y. X., Zhang, X. N., Zhang, Y., and Zhou, H. Q. (2020). Role of interface properties in organic solar cells: from substrate engineering to bulk-heterojunction interfacial morphology. Mat. Chem. Front. 4 (10), 2863–2880. doi:10.1039/d0qm00398k
Zhang, M., Zhu, L., Zhou, G. Q., Hao, T. Y., Qiu, C. Q., Zhao, Z., et al. (2021). Single-layered organic photovoltaics with double cascading charge transport pathways: 18% efficiencies. Nat. Commun. 12 (1), 309. doi:10.1038/s41467-020-20580-8
Zhao, F. W., Dai, S. X., Wu, Y. Q., Zhang, Q. Q., Wang, J. Y., Jiang, L., et al. (2017). Single-Junction binary-blend nonfullerene polymer solar cells with 12.1% efficiency. Adv. Mater 29 (18), 1700144. doi:10.1002/adma.201700144
Zhao, F. W., Wang, C. R., and Zhan, X. W. (2018). Morphology control in organic solar cells. Adv. Energy Mater 8 (28), 1703147. doi:10.1002/aenm.201703147
Zheng, Z., Wang, J. Q., Bi, P. Q., Ren, J. Z., Wang, Y. F., Yang, Y., et al. (2022). Tandem organic solar cell with 20.2% efficiency. Joule 6 (1), 171–184. doi:10.1016/j.joule.2021.12.017
Zhou, K. K., Xian, K. H., and Ye, L. (2022a). Morphology control in high-efficiency all-polymer solar cells. InfoMat 4 (4), e12270. doi:10.1002/inf2.12270
Zhou, X. M., Dong, X. Y., Liu, Y., Wang, W., Wei, W. X., Chen, J. P., et al. (2022b). Effect of wetting surfactants on the work function of PEDOT:PSS for organic solar cells. ACS Appl. Energ Mater 5 (3), 3766–3772. doi:10.1021/acsaem.2c00217
Zhu, L., Zhang, M., Xu, J. Q., Li, C., Yan, J., Zhou, G. Q., et al. (2022). Single-junction organic solar cells with over 19% efficiency enabled by a refined double-fibril network morphology. Nat. Mater 21 (6), 656–663. doi:10.1038/s41563-022-01244-y
Zhu, L., Zhang, M., Zhong, W. K., Leng, S. F., Zhou, G. Q., Zou, Y. C., et al. (2021). Progress and prospects of the morphology of non-fullerene acceptor based high-efficiency organic solar cells. Energy Environ. Sci. 14 (8), 4341–4357. doi:10.1039/d1ee01220g
Keywords: organic solar cell, PEDOT:PSS, alcoholic solvent, phase component, morphology
Citation: Han T, Gao C, Sun K, Zhu L, Wang L, Liang W, Miao Y, Wang Y, Qiu M, Li S and Zhang G (2024) Efficiency enhancement of non-fullerene organic solar cells using PEDOT:PSS diluted with alcohol solvents as the hole transport layer. Front. Mater. 11:1383816. doi: 10.3389/fmats.2024.1383816
Received: 08 February 2024; Accepted: 19 April 2024;
Published: 16 May 2024.
Edited by:
Lulu Ren, University of California, Irvine, United StatesCopyright © 2024 Han, Gao, Sun, Zhu, Wang, Liang, Miao, Wang, Qiu, Li and Zhang. This is an open-access article distributed under the terms of the Creative Commons Attribution License (CC BY). The use, distribution or reproduction in other forums is permitted, provided the original author(s) and the copyright owner(s) are credited and that the original publication in this journal is cited, in accordance with accepted academic practice. No use, distribution or reproduction is permitted which does not comply with these terms.
*Correspondence: Yanqin Miao, miaoyanqin@tyut.edu.cn; Yufei Wang, wangyufei@sztu.edu.cn; Mingxia Qiu, qiumingxia@sztu.edu.cn; Shunpu Li, lishunpu@sztu.edu.cn; Guangye Zhang, zhangguangye@sztu.edu.cn