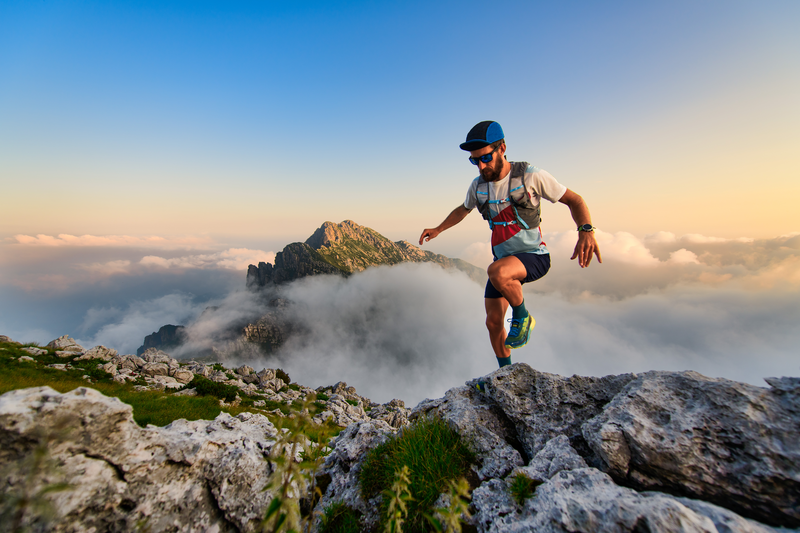
94% of researchers rate our articles as excellent or good
Learn more about the work of our research integrity team to safeguard the quality of each article we publish.
Find out more
ORIGINAL RESEARCH article
Front. Mater. , 29 February 2024
Sec. Polymeric and Composite Materials
Volume 11 - 2024 | https://doi.org/10.3389/fmats.2024.1380472
This article is part of the Research Topic Women In Science: Materials 2023 View all 10 articles
Carbon nanotubes (CNTs), which have a diameter on the nanometer scale, are tube-like materials composed of carbon and graphite sheets. Carbon nanotubes play an important role in many fields such as nanotechnology, electronics, optics and material science due to their superior properties such as high elasticity, high thermal conductivity, low density and being more chemically inert. In this study, the use of carbon nanotubes in radar absorbing materials (RAM) to reduce the reflection of electromagnetic waves was investigated. In this sense, by using a polymeric composite structure reinforced with carbon nanotubes, a material with unique properties such as solid absorption, low weight/thickness and cost-effectiveness has been produced, minimizing the reflection of electromagnetic waves. For this purpose, carbon nanotubes with different particle sizes of 8 nm, 30–50 nm and 48–78 nm were blended with polyester at the ratio of 1%. The resulting composites were characterized using a Network analyzer in the frequency range of 8–12 GHz. According to the results obtained, it was observed that radar absorption increased with increasing particle size. It has been determined that by changing the particle sizes of carbon nanotubes in composites, the absorption bands can be adjusted to suit different applications in different frequency bands.
Recently, we encounter many different materials brought about by technological developments and the constant search for innovation. In this regard, the field of materials science is at the forefront of shaping revolutionary changes in various sectors. Thanks to nanotechnological studies, the synthesis and application of carbon nanotubes (CNTs) have emerged as a ray of hope, especially in the field of radar absorbing materials. These nanostructures contribute to the development of cutting-edge materials with extraordinary capabilities in the fields of stealth technology, aerospace and defense. These cylindrical structures, called carbon nanotubes, represent a wrapped manifestation of a two-dimensional (2D) carbon structure known as graphene, intricately woven with carbon atoms arranged in hexagonal patterns. Both ends of this tube can be encapsulated with spherical cylindrical structures (Zhao et al., 2019; Ruiz-Perez et al., 2022). The remarkable physical and electronic properties inherent in carbon nanotubes have made them the focus of extensive scientific research. When strategically incorporated into polymer matrices, these nanotubes give rise to composites capable of absorbing and scattering electromagnetic radiation, including radar signals. In some studies, the electromagnetic or conductivity values of additives can be manipulated by modifying carbon nanotubes or various functionalization processes. As long as the ratio of hybrid filler components within the composite can be optimally adjusted, higher aspect ratio creates more filler junctions to create denser and more interconnected channels, which has ultimately been shown to increase the electrical and EMI shielding performance of composite films (RenaLia et al., 2020; Ghosh et al., 2023). The implications of such materials extend far beyond traditional applications and offer a promising glimpse into the future landscape of advanced materials engineering (QiYangZhong et al., 2009; Hong MelvinNi and Natsuki, 2014; Luo et al., 2014).
It can be said that the working principle of the radar is based on the same principle as the sound echoing from the wall. Because in both cases, a signal is transmitted, reflected from an object and returned. While the signal sent in sound reverberation is sound, electromagnetic wave types such as radio waves and microwaves are used as the signal sent in the radar system (National Imagery and Mapping Agency, 2001; Ting et al., 2008). The amount of reflection and refraction of these waves depends on the properties and surface of the material through which the signal is sent. When the signal hits a completely flat surface, the signal is reflected in one direction. When it hits a rough surface, it is reflected in various directions and only a very small portion of the original signal is transmitted back to the receiver (Varshney, 2002; Trujillo and Thurman, 2004). Another way to reduce the signal is to impregnate the signal by the material it passes through. At this point, the radar absorbing materials we encounter have a mechanism that traps incoming radar signals and prevents their reflection (Ghasemi et al., 2006; Mozaffari et al., 2009). These materials can be produced by changing the dielectric and magnetic properties of the materials. Magnetic materials such as ferrite, iron, and cobalt-nickel alloys are used to change the permittivity of base materials, while high dielectric materials such as carbon, graphite, and metal flakes are used to change the dielectric properties. Dielectric materials used in radar absorption do not have magnetic properties. Examples of these are commonly used materials such as foams, plastics and elastomers (Kojima et al., 1982; Lalrdtech, 2012; Atay and Çelik, 2014).
The main aim of this study is to obtain a radar-absorbing composite material by grafting carbon nanotubes into an epoxy resin matrix. Utilizing three different sizes of carbon nanotubes, the article aims to examine the effect of these size differences on radar absorption properties. Research and development in this field is pushing the boundaries of what is considered possible, and our understanding of the complex interaction between nanotechnology and radar-absorbing materials is constantly expanding. For this purpose, carbon nanotubes with different particle sizes of 8 nm, 30–50 nm and 48–78 nm were blended with polyester at the ratio of 1%. The structural and morphological properties of these composites were evaluated by X-ray Diffraction (XRD) and Scanning Electron Microscope (SEM) analyses. Magnetic properties were analyzed by vibrating sample magnetometer (VSM). Additionally, the composites were subjected to characterization through a Network Analyzer covering the frequency range from 8 to 12 GHz.
The carbon nanotubes used as reinforcement materials in this research are multi-walled carbon nanotubes. These carbon nanotubes, which were used without being functionalized in any way, were purchased from Nanografi. Nanotubes have a high purity level of 96% and are available in three different particle size ranges: <8nm, 30–50nm and 48–78 nm. Polyester resin was used as matrix material. Carbon nanotubes and polyester resin were first mixed with 3% hardener (methyl ethyl ketone peroxide) and left to cure for 2 days at room temperature, then mixed with polyester resin at 1% loading level. Mixing was done by mechanical mixing for 15 min. After this, the resulting mixture was poured into a special holder. The samples produced with this mold were used in Network analyzer radar absorption measurement.
Electromagnetic parameters of the composites were measured by the transmission/reflection method in the 8–12 GHz region with the 8720D Network Analyzer device (Agilent Technologies) shown in Figure 1A. The network analyzer and coaxial line armature were calibrated to perform microwave absorption measurement. The reason for using the mold in Figure 1B is to ensure that the sample to be analyzed according to the transition line method is placed between the waveguides of the analyzer without any gaps. Accordingly, reliable and healthy results were obtained by ensuring full overlap with the holder. The control sample of pure polyester resin was cast in the same way and measurements were made. Accordingly, a signal is sent from a source to the material. Signals reflected and transmitted from the material are detected by sensors. Performance calculation is based on the principle that the sum of the reflected, transmitted and absorbed signals in the material is exactly. The amount absorbed is calculated in this way (Atay, 2016). In this study, radar absorption value was evaluated with reflection loss.
FIGURE 1. Schematic presentation of; (A) S-parameter measurement setup using 8720D network analyser, and (B) prepared specimen.
Phase analysis of the powders was performed using an Empyrean X-ray diffractometer using Cu Kα radiation (λ = 1.540 Å) in the 2θ range ranging from 20 to 80°. A Carl Zeiss Sigma 300 VP scanning electron microscope (SEM) was used to obtain morphological images of the composites. The magnetic behavior of the materials at room temperature was evaluated using a VSM 550–100 vibrating sample magnetometer. Polytetrafluoroethylene (PTFE) Teflon tape was used during these measurements.
As shown in Figure 2, XRD (X-ray Diffraction) patterns were obtained using the Panalytical Empyrean XRD device. Monochromatic CuKα beam was used as the X-ray source. Scanning was done in the range of 5°–90°, and the patterns were obtained at 45 kV and 40 mA conditions.
SEM images of 96% pure multi-walled carbon nanotubes (MWCNTs) with particle sizes of <8nm, 30–50nm and 48–78nm, without any additional preparation or processing, are shown in Figure 3. The results obtained from the images confirm that the dimensional consistency of the received carbon nanotubes is at the specified level. In addition, it can be seen that the nanotubes have a homogeneous and stable structure.
Table 1 shows the coercivity and saturation magnetization values obtained from the VSM analysis. It is clearly seen that both saturation magnetization and coercivity values decrease with increasing particle sizes. The reason for this is that increasing the particle size causes a decrease in the surface/volume ratio. In this case, dielectric loss is expected to decrease as a result of increased agglomeration.
The properties of the composites were evaluated using a Network Analyzer in the frequency spectrum from 8 to 12 GHz. Initially, composites were produced as single-layer configurations at 1% loading level with different particle size. The data obtained from these measurements were analyzed by creating graphical representations demonstrated in Figure 4. According to these results, the best value was observed in composites with dimensions in the range of 48–78 nm, the minimum reflection loss value was determined as −9.38 dB and the effective bandwidth was determined as 10.27 GHz.
Similar results have been obtained in many studies investigating the radar absorption performance of composites prepared using carbon nanotubes. In addition, materials prepared using other additives in addition to carbon nanotubes have also taken their place in the literature. In a study, the electromagnetic wave absorption abilities of carbon nanotubes and composite materials using Fe3O4 were investigated and very good results were obtained (Ghosh et al., 2023). A significant improvement in impedance matching was observed in CNT-loaded Fe3O4 composites compared to pure CNTs or Fe3O4. The absorption mechanism is primarily attributed to improved impedance matching, interfacial scattering, and dielectric and magnetic losses. The outstanding electromagnetic wave absorption performance of CNT-loaded Fe3O4 composites has been found to contribute to the development of lightweight and highly efficient electromagnetic wave absorbing materials (RenaLia et al., 2020). In another study, the meticulous preparation of barium titanate (BTO) nanoparticles and their subsequent integration onto the surface of carbon nanotubes (CNTs) was achieved using the sol–gel method. The study systematically evaluates the capacity of these materials to absorb electromagnetic (EM) waves by measuring their dielectric and magnetic losses when subjected to EM wave penetration (Hong MelvinNi and Natsuki, 2014). There are other studies on the design of CNT/G hybrids, which can be considered as an effective way to design lightweight and high-performance EM absorber materials by combining CNTs and graphene sheets into three-dimensional structures (Luo et al., 2014). This study introduces an innovative and efficient approach to fabricate a promising EM absorption material with characteristics such as light weight, robust absorption capability, and a broad absorption bandwidth. The results of the research conducted according to the size range will serve as a guide for future studies.
In this study, the production of radar absorbing material using carbon nanotubes was investigated. In this sense, it has been determined that the electromagnetic wave absorption abilities of the composite materials produced may vary depending on factors such as particle size. Looking at the results, it was seen that the reflection loss values increased with increasing particle size. The reason for this is that the resulting dielectric loss causes the composite materials to interact with electromagnetic waves and weaken these waves. Accordingly, the microwave absorption ability increased. Since it is a study in which additives are evaluated dimensionally, this study will be a guide for optimizing microwave absorption properties and using them in various applications in the future.
The original contributions presented in the study are included in the article/Supplementary material, further inquiries can be directed to the corresponding author.
GY: Writing–original draft, Writing–review and editing. NB: Writing–original draft.
The author(s) declare that no financial support was received for the research, authorship, and/or publication of this article.
The authors declare that the research was conducted in the absence of any commercial or financial relationships that could be construed as a potential conflict of interest.
All claims expressed in this article are solely those of the authors and do not necessarily represent those of their affiliated organizations, or those of the publisher, the editors and the reviewers. Any product that may be evaluated in this article, or claim that may be made by its manufacturer, is not guaranteed or endorsed by the publisher.
Atay, H. Y. (2016). MIKRO VE NANO-BOYUTLU BARYUM HEKZAFERRIT TOZLARI ILE TAKVIYE EDILMIŞ POLIMER KOMPOZITLERIN RADAR ABZORBLAYICI ÖZELLIKLERI ÜZERINE BIR KARŞILAŞTIRMA. Mugla J. Sci. Technol. 2, 88–92. doi:10.22531/muglajsci.269979
Atay, H. Y., and Çelik, E. (2014). Barium hexaferrite reinforced polymeric dye composite coatings for radar absorbing applications. Polym. Compos. 35, 602–61010. doi:10.1002/pc.22701
Ghasemi, A., Hossienpour, A., Morisako, A., Saatchi, A., and Salehi, M. (2006). Electromagnetic properties and microwave absorbing characteristics of doped barium hexaferrite. J. Magn. Magn. Mater. 302 (2), 429–435. doi:10.1016/j.jmmm.2005.10.006
Ghosh, S. K., Nath, K., Paul, S., Ghosh, T., Katheria, A., Das, P., et al. (2023). Combination effect of functionalized high aspect ratio carbonaceous nanofillers and carbon black on electrical, thermal conductivity, dielectric and EMI shielding behavior of co-continuous thermoplastic elastomeric blend composite films. Chem. Eng. J. Adv. 15, 100505. doi:10.1016/j.ceja.2023.100505
Hong MelvinNi, G. J.Q.-Q., and Natsuki, T. (2014). Electromagnetic wave absorption properties of barium titanate/carbon nanotube hybrid nanocomposites. J. Alloys Compd. 615, 84–90. doi:10.1016/j.jallcom.2014.06.191
Kojima, H., and Wohlfarth, E. P. 1982 Ferromagnetic materials: a handbook on the properties of magnetically ordered substances(Ed.), vol. 3, ch 5. North Holland, Amsterdam
Luo, K., YinYuan, X. X., Zhang, Y., Liu, X., Cheng, L., Litong, Z., et al. (2014). Electromagnetic wave absorption properties of graphene modified with carbon nanotube/poly(dimethyl siloxane) composites. Carbon 73, 185–193. doi:10.1016/j.carbon.2014.02.054
Mozaffari, M., Taheri, M., and Amighian, J. (2009). Preparation of barium hexaferrite nanopowders by the sol–gel method, using goethite. J. Magn. Magn. Mater. 321, 1285–1289. doi:10.1016/j.jmmm.2008.11.106
National Imagery and Mapping Agency (2001). “Radar Navigation and maneuvering board manual,”. NTM 38/08 in Basic radar principles and general characteristics. Seventh Edition (ProStar Publications). Chapter 1.
QiYangZhong, X. Y. W., DengAu, Y. C., and Du, Y. (2009). Large-scale synthesis, characterization and microwave absorption properties of carbon nanotubes of different helicities. J. Solid State Chem. 182 (10), 2691–2697. doi:10.1016/j.jssc.2009.07.036
RenaLia, M. F., WangaWeia, B. J., and Yu, Q. (2020). Preparation and electromagnetic wave absorption properties of carbon nanotubes loaded Fe3O4 composites. J. Magnetism Magnetic Mater. 513, 167259. doi:10.1016/j.jmmm.2020.167259
Ruiz-Perez, F., López-Estrada, S. M., Tolentino-Hernández, R. V., and Caballero-Briones, F. (2022). Carbon-based radar absorbing materials: a critical review. J. Sci. Adv. Mater. Devices 7 (Issue 3), 100454. doi:10.1016/j.jsamd.2022.100454
Ting, T. H., Wu, K. H., Hsu, J. S., Chuang, M. H., and Yang, C. C. (2008). Microwave absorption and infrared stealth characteristics of bamboo charcoal/silver composites prepared by chemical reduction method. J. Chin. Chem. Soc. 55, 724–731. doi:10.1002/jccs.200800109
Trujillo, A. P., and Thurman, H. V. (2004). “The black arts: materials and process selection and stealth technology,” in Essentials of oceanography.
Varshney, L. (2002). Technical report radar principles october 29, 2002 revision No. 3 syracuse research corporation. NY, 295–326.
Keywords: radar absorbing materials, carbon nanotubes, polymer composites, particle size effect, network analysis
Citation: Yilmaz Atay G and Bilgiç N (2024) Radar absorbing properties of different size carbon nanotube reinforced polymer composites. Front. Mater. 11:1380472. doi: 10.3389/fmats.2024.1380472
Received: 01 February 2024; Accepted: 14 February 2024;
Published: 29 February 2024.
Edited by:
Tushar Kanti Das, Silesian University of Technology, PolandReviewed by:
Narayan Das, Indian Institute of Technology Kharagpur, IndiaCopyright © 2024 Yilmaz Atay and Bilgiç. This is an open-access article distributed under the terms of the Creative Commons Attribution License (CC BY). The use, distribution or reproduction in other forums is permitted, provided the original author(s) and the copyright owner(s) are credited and that the original publication in this journal is cited, in accordance with accepted academic practice. No use, distribution or reproduction is permitted which does not comply with these terms.
*Correspondence: Gül Yilmaz Atay, aGd1bHlpbG1hekBnbWFpbC5jb20=
Disclaimer: All claims expressed in this article are solely those of the authors and do not necessarily represent those of their affiliated organizations, or those of the publisher, the editors and the reviewers. Any product that may be evaluated in this article or claim that may be made by its manufacturer is not guaranteed or endorsed by the publisher.
Research integrity at Frontiers
Learn more about the work of our research integrity team to safeguard the quality of each article we publish.