- Zhenjiang Port Group Co., Ltd., Zhenjiang, Jiangsu, China
To enhance the mechanical properties and cost-effectiveness of conventional polyvinyl alcohol fiber reinforced strain hardening cementitious composite (PVA-SHCC), a modified version called multiscale hybrid fiber reinforced SHCC (MsHySHCC) was developed. This new composite incorporates a combination of steel fiber, PVA fiber and calcium carbonate (CaCO3) whisker. Uniaxial direct tensile behaviors (stress-strain relationship, tensile strength, tensile deformation capacity and tensile toughness) of designed MsHySHCCs were investigated and evaluated. The results show that the PVA fibers dominate the ductile behavior and the steel fibers and CaCO3 whiskers effectively affect the strength of MsHySHCCs. The PVA fibers can be partially replaced by CaCO3 whisker and steel fiber, along with an increase in tensile strength and ductility of designed composites. The findings suggest that the configuration of MsHySHCC proves to be a viable approach in simultaneously enhancing the strength and ductility of PVA-SHCC. A semi-theoretical prediction model for tensile constitutive relationship was derived. The comparison of the theoretical results with the experimental data shows that this semi-theoretical model is applicable for determining the tensile constitutive relationship of PVA-SHCCs and MsHySHCCs.
1 Introduction
Strain hardening cementitious composite (SHCC) is a kind of cementitious composite with high ductility (Liu et al., 2020; Arain et al., 2023). In SHCC preparation, PVA fiber and PE fiber are commonly utilized (Zhang et al., 2021; Zhang et al., 2023a; Khalil and Atta, 2023; Tran and Nguyen, 2023). Out of all these, PVA-SHCC stands as the extensively researched and utilized fiber reinforced material. Nevertheless, the high expense remains a significant obstacle for PVA-SHCC to be implemented in extensive engineering projects (Zhang et al., 2020).
Hybrid use of low cost fibers is one of the effective ways to decrease the material cost of PVA-SHCC (Maalej et al., 2012; Liu et al., 2020; Rawat et al., 2022; Qasim et al., 2023), especially for the hybridization of PVA fiber and micro steel fiber (SF/PVA-SHCC). A lot of previous literatures have reported the tensile performance of SF/PVA-SHCCs. Ramasamy and Shanmughasundaram, (2018) reported the mechanical properties of SF/PVA-SHCC containing 0.65% PVA fibers and 1.35% steel fibers. The experimental tensile strength and ultimate tensile strain are 6.78 MPa and 0.98%, respectively. Ramasamy’s research found that the steel fiber content exceeded that of PVA fiber, resulting in a comparatively elevated tensile strength but relatively limited tensile ductility. Similar results were also noted in other studies (Hermes et al., 2012; Feng, 2019). It seems that the tensile ductility of SF/PVA-SHCC isn’t mainly dominated by steel fibers. Wang et al. (2014) studied the tensile behavior of SF/PVA-SHCCs made by 0.3% steel+1.7% PVA fibers and 0.6% steel+1.7% PVA fibers. The tensile strength of these two kinds of SF/PVA-SHCCs is 3.72 and 4.02 MPa, respectively, while the ultimate tensile strain is 2.39% and 1.97%, respectively. It seems that the combination of high content of PVA fiber and low content of steel fiber can obtain a relatively high tensile ductility, but can’t achieve a relatively high tensile strength. Similar experimental results were also pointed out by Liu and Tan. (2017a); Pourfalah (2018); Zhao et al. (2020); Tinoco and Silva (2021).
However, the influence of the content of steel fiber and PVA fiber on the tensile behaviors of SHCC is not completely consistent with the change laws discussed above. A lot of studies have attempted to achieve both high tensile strength and ductility via further adjusting fiber content, introducing highly reactive mineral admixtures, lowing water-binder ratio and optimizing the sand-binder ratio (Zhang et al., 2016; Liu et al., 2017b; Deshpande et al., 2019). However, it is regrettable that we are still far from this idea. Despite the high concentration of steel fibers and PVA fibers in certain research studies, the obtained experimental outcomes remain dissatisfactory (Zhang et al., 2016; Deshpande et al., 2019). As has been reported in Zhang’s study, the combination of 1% steel fiber and 2% PVA fiber obtained a very high tensile strength (7.22 MPa), while the ultimate tensile strain was relatively low (0.8%) (Zhang et al., 2016). Therefore, it still needs to find an effective way to optimize the tensile strength and ductility of SF/PVA-SHCC.
It is widely recognized that cementitious materials possess distinct structural characteristics at multiple levels, including the levels of cement hydration products, cement paste, mortar, and concrete (Koichi et al., 2003; Kang and Bolander, 2016). Theoretically, the performance of cementitious materials can be significantly improved by incorporating multi-scale hybrid fibers, spanning from the microscopic to the macroscopic level (Parant and Rossi, 2007a; Parant and Rossi, 2007b; Pierre and Edouard, 2008; Rossi and Parant, 2008; Zhang and Cao, 2014; Cao et al., 2015). However, it is clear that achieving this task solely with steel fiber and PVA fiber is challenging. According to reports, it is believed that the size of fibers, particularly their diameter, has an inverse relationship with their ability to delay the development of microscopic cracks (Betterman et al., 1995; Cao et al., 2013). The dimensions of commonly used PVA fiber and steel fiber do not align well with the microscopic scale of cement hydration product and microscopic cracks, consequently failing to effectively improve the microscopic characteristics of cementitious materials. Hence, it is imperative to discover a viable approach to further augment the microscopic properties of SF/PVA-SHCC.
In recent times, SHCC’s mechanical properties were improved using calcium carbonate (CaCO3) whiskers with dimensions of about 30 μm in length and 1 um in diameter. Meanwhile, the cost of CaCO3 whiskers is only about 230 $/t. The enhancement is achieved through microscopic mechanisms such as whisker pull-out, bridging, and crack deflection (Ma et al., 2017; Pan et al., 2018; Cao et al., 2019; Xie et al., 2020). As a result, a composite material named MsHySHCC, which consists of multiple scales of hybrid fibers and exhibits strain hardening properties, was developed. There are two main kinds of MsHySHCC now. The initial type of MsHySHCC solely comprises of PVA fiber and CaCO3 whisker, whereas the second type of MsHySHCC is created by simultaneously incorporating two macro fibers (steel fiber and PVA fiber) and one microscopic fiber (CaCO3 whisker). Mechanical performance and reinforcing mechanism of these two kinds of MsHySHCC have been widely studied. Ma et al. (2017) tested the tensile properties of the aforementioned first kind of MsHySHCC. The experimental findings indicated that CaCO3 whisker increased the tensile strain capacity of PVA-SHCC. However, the strength of the MsHySHCC is comparatively weak, with a compressive strength of about 25 MPa and a tensile strength of about 3 MPa. Consequently, this aspect hinders the practical implementation of the designed MsHySHCC. Mechanical properties of PVA-SHCC, which was modified by CaCO3 whiskers (the aforementioned initial type of MsHySHCC), were also documented in Pan’s study. It was discovered that the CaCO3 whiskers can be used as a partial replacement for the PVA fibers, resulting in further improvement of the tensile and flexural characteristics of the designed MsHySHCC. However, the concentration of CaCO3 whisker in their research is exceedingly high, consequently elevating the likelihood of whisker agglomeration (Cao et al., 2013). Cao et al. (2019) and Xie et al. (2020) studied the rheological behaviors, mechanical properties and shrinkage performances of MsHySHCC containing steel fiber, PVA fiber and CaCO3 whisker (the aforementioned second kind of MsHySHCC). The researchers discovered that the engineered MsHySHCCs improved the abiligy of the mortar matrix against cracking at multiple scales. However, despite their initial intention to create the SHCC substance through the incorporation of fibers at various scales, the strain hardening characteristics of the developed MsHySHCCs did not meet expectations. Due to the improper consideration of the steel fiber’s role in controlling the hardening performance of cementitious materials in their study, the content of steel fiber is higher than that of PVA fiber, resulting in the inability to achieve a high ductility for MsHySHCCs.
From the above previous literatures, it can be noticed that although previous studies focused on MsHySHCC have obtained some relatively rich achievements, there are three basic questions still need to be well responded. (a) What methods can be used to simultaneously enhance the strength and ductility of existing MsHySHCCs? (b) Most of the literatures are experimental studies focus on mechanical properties of MsHySHCC, it still lacking an effective constitutive model to describe the tensile behavior of MsHySHCC, although some micromechanical models and fractural models have been proposed for single fiber type SHCC. To this question, the main difficulties are how to consider the effect of hybrid fibers and most importantly how to take the action of CaCO3 whisker into consideration.
In order to answer the above questions, the following two main works were carried out in this paper. By reasonably adjusting the ratio of matrix, steel fiber, PVA fiber, and CaCO3 whisker, a novel form of MsHySHCC was achieved. Direct tensile performance of this kind of MsHySHCC was characterized, and the balance of strength and ductility of the designed composites was verified. By considering the impact of hybrid fibers and CaCO3 whisker, a semi-theoretical prediction model was given to describe the tensile constitutive relationship of the designed MsHySHCC.
2 Material and experiment
2.1 Raw materials and mix proportion
The components utilized in the matrix included P୰O 42.5 cement, fly ash, and fine quartz sand with a particle size ranging from 100 to 210 μm and an average size of 150 μm. Figure 1 displays the particle size distribution curves for fly ash, sand and cement. The particle size of fly ash is finer compared to cement. It can be used to make the particles of the raw material pack more closely, meanwhile, it has higher hydration activity than low grade fly ash, thus improving the strength of the composite material to a certain extent. Referred to the previous research results (Ma et al., 2017; Zhang et ak., 2020a), the mass ratio of fly ash and cement was set as 4:1 to guarantee a substantial ductility for the designed composite material.
Previous literatures suggested that the optimal proportion of cement to fine sand should be between 0.6 and 1.8 (Liu and Tan, 2017a; Liu et al., 2017; Ramasamy and Shanmughasundaram, 2018; Tinoco and Silva, 2021; Arain et al., 2023). This study set the mass ratio of sand and cement as 1.8:1 in order to increase the density of the particles, bringing them closer to the theoretical curve, as depicted in Figure 2. More details about the modified A&A model can be obtained in previous literatures (Andreasen and Andersen, 1930; Funk and Dinger, 1994; Hunger, 2010; Karim et al., 2019).
The water to binder ratio was set as 0.34. The fresh MsHySHCC’s workability was modified using superplasticizer at 0.5 wt% of binder. Three kinds of reinforcing fibrous materials were employed in this study, as shown in Figure 3. Table 1 displays the composition of various fibers in each designed group. Meanwhile, the 28 days compressive strength (70.7 mm3 cube) of each group is also give in Table 1. It can be seen that the designed composites had a relative high compressive strength.
2.2 Specimen preparation and testing method
The process of preparing the specimen is illustrated in Figure 4. Dog-bone-shaped specimen was prepared. The tension specimen size is shown in Figures 4, 5. To ensure the precision of the experimental findings, three samples were prepared for every design mixture.
Tensile test set-ups are shown in Figure 5. A 15 mm range extensometer was used to monitor the tensile deformation of the specimen. The tensile loading rate is 0.1 mm/min.
3 Results and discussion
3.1 Tensile stress-strain relationships
Figure 6 shows the tensile stress-strain relationships of control groups with or without CaCO3 whiskers. Table 2 summarizes the average tensile values of strength, ultimate strain and toughness of the three groups of materials. This paper quantifies the tensile toughness by calculating the enclosed region beneath the tensile stress-strain graph, which is employed to assess the specimen’s ability to absorb energy under tensile force. By observing Figure 6; Table 2, it becomes evident that CaCO3 whiskers can enhance both the tensile strength and tensile strain capacity of the mortar matrix. And the tensile strength and ultimate strain are further improved with increasing the content of CaCO3 whiskers from 1% to 2%. The enhancements can be explained by the micro-mechanisms of CaCO3 whiskers as illustrated in Figure 7. However, it is worth mentioning that despite the enhancement of tensile properties in the mortar matrix due to the utilization of CaCO3 whisker, the failure process still exhibited evident brittleness and lacked post peak toughness (Cao et al., 2013; Cao et al., 2019). Because the particle size of CaCO3 whisker is small, it can only strengthen and toughen the mortar matrix at the microscopic level, thus can’t improve the post peak toughness of the matrix like other macro fibers.
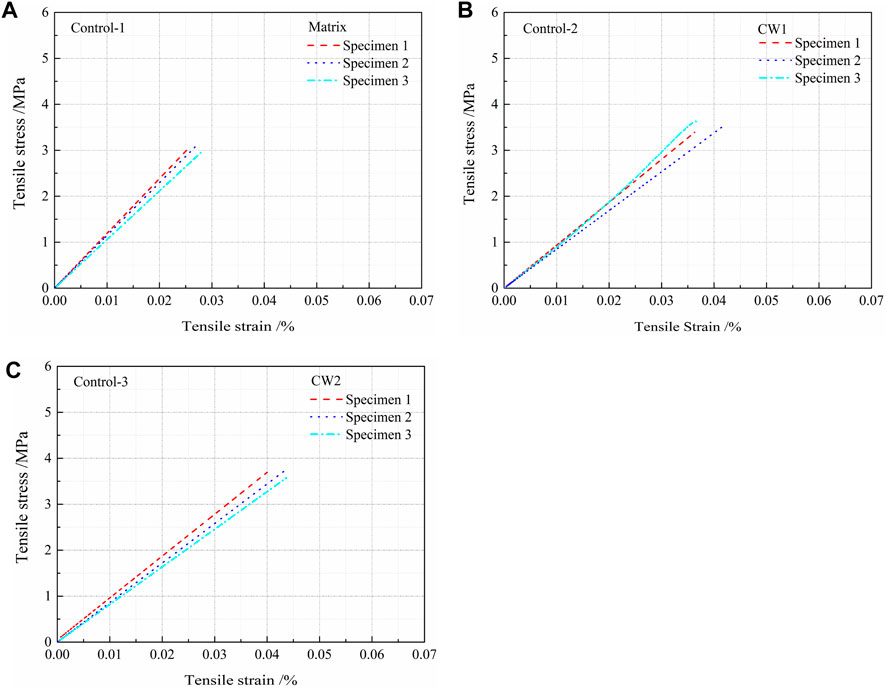
Figure 6. Tensile stress-strain relationships of (A) matrix; (B) 1% CaCO3 whisker and (C) 2% CaCO3 whiskers.
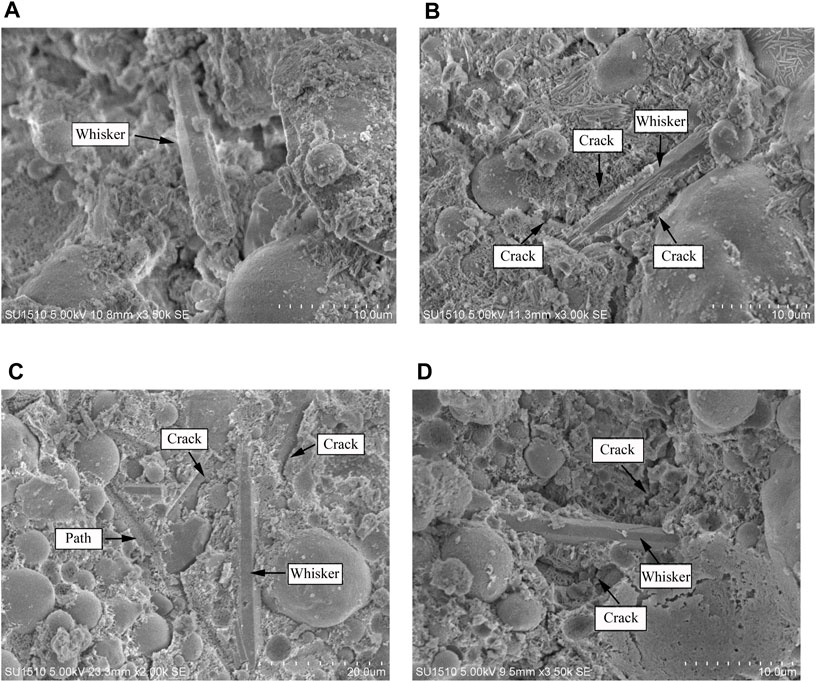
Figure 7. Micro-mechanisms of calcium carbonate whisker (A) pull-out; (B) crack deflection; (C) crack deflection and (D) bridging.
The relationship between tensile stress and strain is depicted in Figure 8. Experimental tensile results of strength, ultimate strain and toughness for each group are provided in Figure 9. Experimental data of tensile parameters in previous available literatures are summarized in Table 3. Figure 10 illustrates the comparisons of the experimental findings between previous literature and the current study. From Figures 8–10; Table 3, the following findings can be addressed.
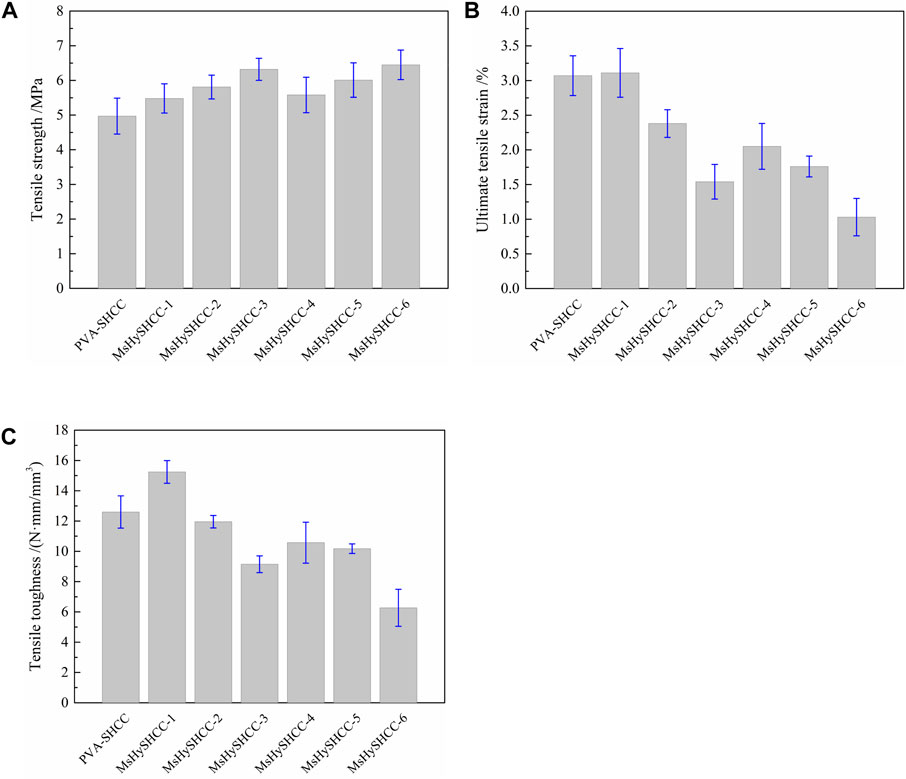
Figure 9. Experimental tensile results of (A) strength; (B) ultimate strain and (C) toughness for PVA-SHCC and MsHySHCCs.
The experimental curves exhibited a slight improvement in robustness when PVA fibers were partially replaced with CaCO3 whiskers and micro hooked steel fibers, as depicted in Figures 8A–G. The microscopic mechanisms of CaCO3 whisker shown in Figure 7 have the potential to enhance the quantity and evenness of micro-cracks within the mortar matrix (Cao et al., 2015; Ma et al., 2017; Pan et al., 2018). Ma et al. (2017) discovered that additional crack sources could potentially contribute to the multiple cracking performance of SHCC (Pan et al., 2018). As a result, it is possible to improve the robustness of the tensile stress-strain curves.
The addition of steel fibers and CaCO3 whiskers to replace some PVA fibers increased the tensile strength of PVA-SHCC. Compared to PVA-SHCC, when the whisker content maintained at 1%, the tensile strength exhibited an increase from 5.48 MPa to 6.32 MPa with the steel fiber content ranging from 0.25% to 0.75%. Increasing the amount of whiskers further enhanced the tensile strength to a small extent, despite the further reduction in PVA fiber content. The enhancements could be credited to the higher peak stress for crack bridging offered by steel fibers with hooks and the reinforcing effect at a microscopic level provided by CaCO3 whiskers. The whiskers and steel fibers have greater modulus of elasticity and stiffness compared to PVA fibers. By limiting the development of cracks, these rigid fibers can effectively improve the principal tensile stress of the material, thus increasing its tensile strength (Rawat et al., 2022; Qasim et al., 2023). On the contrary, PVA fiber is a kind of flexible synthetic fiber that has a lesser impact on the tensile strength compared to steel fiber and CaCO3 whisker.
As depicted in Figure 8; Table 3, MsHySHCC-1 exhibited greater tensile strength and ultimate tensile strain in comparison to PVA-SHCC, with 1% CaCO3 whiskers hybrid 0.25% steel fibers partially replacing 0.25% PVA fibers. Nevertheless, a gradual deterioration in strain hardening behavior of SHCCs was observed when the PVA fiber content was reduced while the steel fiber and CaCO3 whisker amounts were increased. It implies the strain hardening behavior dominates by the PVA fibers. The discrepancy in total quantity between PVA fiber and hooked steel fiber arises due to the constant volume content condition. The main determinant for enhancing hardening performance primarily relies on the quantity of fibers rather than just the fiber content. A decrease in the number of fiber pieces results in a reduction in the effective stress caused by fibers, consequently resulting in an unsaturated cracking performance and an unstable hardening process. Meanwhile, although the number of whiskers is very high, the size of the whiskers is tens of times smaller than that of the PVA fibers, so the whiskers can only have a positive effect on microscopic cracks in the matrix. Consequently, the SHCCs don’t exhibit significant ductility due to the excessive replacement of PVA fibers with whiskers and hooked steel fibers.
As shown in Table 3; Figure 10, based on the findings, it can be inferred that incorporating PVA fiber, steel fiber, and CaCO3 whisker in the design process of multi-scale fibers is a viable approach to simultaneously enhance the strength and ductility in SHCC.
3.2 Semi-theoretical prediction model
Available literatures have confirmed that double-line model can be used to describe the tensile constitutive relationship of PVA-SHCC, as illustrated in Figure 11. To the SHCC reinforced by steel and PVA hybrid fibers, it may have obvious post-peak softening stage due to the introduction of hooked steel fibers. Generally, the parameter of fiber reinforcing factor
The triple-line model needs to determine three key parameters, namely, stable cracking stress
Where
The interfacial bonding parameter
The ultimate tensile stress
The hooked steel fiber characteristic parameter
To characterize the ductility of SHCC, it is necessary to theoretically calculate the ultimate tensile strain
Based on earlier research (Li and Stang, 1997; Lin and Li, 1997; Kanda and Li, 2000), the ultimate crack opening displacement
Where
According to previous literature (Wu and Li, 1992; Alwan, 1994; Kanda and Li, 1998; Wu and Li, 1995), the crack spacing value
Where
Figures 12, 13 showcase the theoretical findings related to the tensile parameters and stress-strain curves. It can be seen that the proposed model adequately characterizes the tensile stress-strain correlation of MsHySHCC. Nevertheless, to a certain degree, the proposed model overestimates the cracking saturation degree of MsHySHCC and traditional PVA-SHCC during the experiment. Consequently, the calculated ultimate tensile strain values surpass the corresponding experimental measurements.
4 Conclusion
The aim of this study was to examine the tensile characteristics of SHCC reinforced with hybrid fibers at multiple scales (MsHySHCC). Two main questions were responded in this study. The initial step involves achieving a kind of MsHySHCC with both high strength and ductility. The second one is how to predict the tensile behaviors of MsHySHCC. This study yields the subsequent fundamental findings.
(1) PVA fibers control the tensile ductility of MsHySHCC. Steel fibers have a more pronounced effect on the tensile strength, but do not improve the tensile ductility of MsHySHCC. The tensile strength and tensile deformation capacity of the SHCC matrix can be significantly enhanced due to the micro-mechanisms involving CaCO3 whiskers. The improved effect of whiskers on the mechanical properties of the matrix can further strengthen the pull-out mechanism of PVA fibers and steel fibers.
(2) Designing MsHySHCC is one of the effective ways to simultaneously enhance the strength and ductility of PVA-SHCC. The designed MsHySHCC shows higher tensile strength than that of traditional PVA-SHCC. Replacing some of the PVA fibers with CaCO3 whiskers and hooked steel fibers at a volume fraction of 0.25% can result in increased tensile strength and ultimate tensile strain. However, a substantial decrease in the PVA fiber amount will greatly diminish the tensile ductility.
(3) By considering the impact of hybrid fibers and CaCO3 whiskers, a semi-theoretical model was developed to describe the tensile constitutive relationship of the designed MsHySHCC. The comparison between the theoretical and experimental findings leads to the conclusion that this semi-theoretical model is capable of determining the tensile stress-strain relationships of MsHySHCC.
Data availability statement
The original contributions presented in the study are included in the article/Supplementary material, further inquiries can be directed to the corresponding author.
Author contributions
JH: Investigation, Writing–original draft, Writing–review and editing. JB: Investigation, Writing–review and editing. HM: Investigation, Writing–review and editing. ZX: Investigation, Writing–review and editing.
Funding
The author(s) declare that no financial support was received for the research, authorship, and/or publication of this article.
Conflict of interest
Authors JH, JB, HM, and ZX were employed by Zhenjiang Port Group Co., Ltd.
Publisher’s note
All claims expressed in this article are solely those of the authors and do not necessarily represent those of their affiliated organizations, or those of the publisher, the editors and the reviewers. Any product that may be evaluated in this article, or claim that may be made by its manufacturer, is not guaranteed or endorsed by the publisher.
References
Ahmed, S. F., Maalej, M., and Paramasivam, P. (2007). Analytical model for tensile strain hardening and multiple cracking behavior of hybrid fiber-engineered cementitious composites. J. Mater. Civ. Eng. 19 (7), 527–539. doi:10.1061/(asce)0899-1561(2007)19:7(527)
Alwan, J. M. (1994). Modeling of the mechanical behavior of fiber reinforced cement based composites under tensile loads. PH.D. Dissertation. Michigan: University of Michigan.
Andreasen, A., and Andersen, J. (1930). About the relationship between grain and gradation gap in products of loose grains (with a few experiments). Kolloid-Zeitschrift 50 (3), 217–228. doi:10.1007/bf01422986
Arain, M. F., Memon, H., Wang, M., Ahmed, A., Chen, J., and Zhang, H. (2023). Matrix tailoring for polyvinyl alcohol (PVA) fiber-reinforced ductile cementitious composites. AATCC J. Res. 10 (2), 63–72. doi:10.1177/24723444221147982
ASTM (2010). Standard test method for flexural performance of fiber-reinforced concrete (using beam with third-point loading). C1609/C1609M. West Conshohoken, PA: ASTM.
Betterman, L., Ouyang, C., and Shah, S. (1995). Fiber-matrix interaction in microfiber reinforced mortar. Adv. Cem. Based Mater. 2, 53–61. doi:10.1016/1065-7355(94)00027-b
Cao, M., Xie, C., Li, L., and Khan, M. (2019). Effect of different PVA and steel fiber length and content on mechanical properties of CaCO3 whisker reinforced cementitious composites. Mater. Construcc. 69 (336), 200. doi:10.3989/mc.2019.12918
Cao, M., Zhang, C., Li, Y., and Wei, J. (2015). Using calcium carbonate whisker in hybrid fiber-reinforced cementitious composites. J. Mater. Civ. Eng. 27 (4), 1–13. doi:10.1061/(asce)mt.1943-5533.0001041
Deshpande, A., Kumar, D., and Ranade, R. (2019). Influence of high temperatures on the residual mechanical properties of a hybrid fiber-reinforced strain-hardening cementitious composite. Constr. Build. Mater. 208 (30), 283–295. doi:10.1016/j.conbuildmat.2019.02.129
Ding, Y., Yu, J., Yu, K., and Xu, S. l. (2018). Basic mechanical properties of ultra-high ductility cementitious composites: from 40 MPa to 120 MPa. Compo. Struct. 185, 634–645. doi:10.1016/j.compstruct.2017.11.034
Feng, J. Q. (2019). Tensile properties of new hybrid fiber reinforced cementitious composites (NHyFRCC). Ph.D. Dissertation. Dalian: Dalian University of Technology.
Funk, J., and Dinger, D. (1994). Predictive process control of crowded particulate suspensions. New York, NY, USA: Springer.
Hermes, P., Zhang, Y., Soe, K., and Bell, J. (2012). Material properties of a new hybrid-fiber engineered cementitious composite. Adv. Mater. Res. 450-451, 433–438. 10.4028/www.scientific.net/amr.450-451.433.
Huang, T., Zhang, Y. X., and Yang, C. H. (2016). Multiscale modelling of multiple-cracking tensile fracture behaviour of engineered cementitious composites. Eng. Fract. Mech. 160, 52–66. doi:10.1016/j.engfracmech.2016.04.006
Hunger, M. (2010). An integral design concept for ecological self-compacting concrete. Ph.D. Dissertation. Eindhoven: Eindhoven University of Technology.
Kabele, P. (2007). Multiscale framework for modeling of fracture in high performance fiber reinforced cementitious composites. Eng. Fract. Mech. 74, 194–209. doi:10.1016/j.engfracmech.2006.01.020
Kanda, T., and Li, V. C. (1998). Multiple cracking sequence and saturation in fiber reinforced cementitious composites. Concr. Res. Tech. 9 (2), 19–32. doi:10.3151/crt1990.9.2_19
Kanda, T., and Li, V. C. (2000). Tensile stress-strain modeling of pseudo-strain hardening cementitious composites. J. Mater. Civ. Eng. 12 (2), 147–156. doi:10.1061/(asce)0899-1561(2000)12:2(147)
Kang, J., and Bolander, J. E. (2016). Multiscale modeling of strain-hardening cementitious composites. Mech. Res. Commun. 78, 47–54. doi:10.1016/j.mechrescom.2015.08.004
Karim, R., Najimi, M., and Shafei, B. (2019). Assessment of transport properties, volume stability, and frost resistance of non-proprietary ultra-high performance concrete. Constr. Build. Mater. 227, 117031. doi:10.1016/j.conbuildmat.2019.117031
Khalil, A. A., Atta, A. M., Baraghith, A. T., Behiry, R. N., and Soliman, O. E. (2023). Shear strengthening of concrete deep beams using pre-fabricated strain-hardening cementitious composite plates. Eng. Struct. 278, 115548. doi:10.1016/j.engstruct.2022.115548
Koichi, M., Tetsuya, I., and Toshiharu, K. (2003). Multi-scale modeling of concrete performance. J. Adv. Concr. Technol. 1, 91–126.
Li, V. C., and Leung, C. K. Y. (1992). Steady-state and multiple cracking of short random fiber composites. J. Eng. Mech. 11 (118), 2246–2264. doi:10.1061/(asce)0733-9399(1992)118:11(2246)
Li, V. C., and Stang, H. (1997). Interface property characterization and strengthening mechanisms in fiber reinforced cement based composites. Adv. Cem. Based Mater. 6 (1), 1–20. doi:10.1016/s1065-7355(97)90001-8
Lin, Z., and Li, V. C. (1997). Crack bridging in fiber reinforced cementitious composites with slip-hardening interfaces. J. Mech. Phys. Solids 45 (5), 763–787. doi:10.1016/s0022-5096(96)00095-6
Liu, J. C., and Tan, K. H. (2017a). Fire resistance of strain hardening cementitious composite with hybrid PVA and steel fibers. Constr. Build. Mater. 135, 600–611. doi:10.1016/j.conbuildmat.2016.12.204
Liu, J. C., Tan, K. H., and Fan, S. (2018). Residual mechanical properties and spalling resistance of strain-hardening cementitious composite with Class C fly ash. Constr. Build. Mater. 181 (30), 253–265. doi:10.1016/j.conbuildmat.2018.06.009
Liu, J. C., Tan, K. H., and Zhang, D. (2017b). Multi-response optimization of post-fire performance of strain hardening cementitious composite. Cem. Concr. Compos. 80, 80–90. doi:10.1016/j.cemconcomp.2017.03.001
Liu, T., Yang, Y., Chen, Z., Li, Y., and Bai, R. (2020). Optimization of fiber volume fraction to enhance reinforcing efficiency in hybrid fiber reinforced strain hardening cementitious composite. Cem. Concr. Compos. 113, 103704. doi:10.1016/j.cemconcomp.2020.103704
Ma, H., Cai, J., Lin, Z., Qian, S., and Li, V. C. (2017). CaCO3 whisker modified engineered cementitious composite with local ingredients. Constr. Build. Mater. 151, 1–8. doi:10.1016/j.conbuildmat.2017.06.057
Maalej, M., Quek, S. T., Ahmed, S. F. U., Zhang, J., Lin, V., and Leong, K. (2012). Review of potential structural applications of hybrid fiber engineered cementitious composites. Constr. Build. Mater. 36, 216–227. doi:10.1016/j.conbuildmat.2012.04.010
Ning, X., Ding, Y., and Zhang, F. (2015). Experimental study and prediction model for flexural behavior of reinforced SCC beam containing steel fibers. Constr. Build. Mater. 93 (0), 644–653. doi:10.1016/j.conbuildmat.2015.06.024
Ou, Y., Tsai, M., Liu, K., and Chang, K. C. (2011). Compressive behavior of steel-fiber-reinforced concrete with a high reinforcing index. J. Mater. Civ. Eng. 24 (2), 207–215. doi:10.1061/(asce)mt.1943-5533.0000372
Pan, J., Cai, J., Ma, H., and Leung, C. K. Y. (2018). Development of multiscale fiber-reinforced engineered cementitious composites with PVA fiber and CaCO3 whisker. J. Mater. Civ. Eng. 30 (6), 04018106. doi:10.1061/(asce)mt.1943-5533.0002305
Pan, Z., Wu, C., Liu, J., and Wang, W. (2015). Study on mechanical properties of cost-effective polyvinyl alcohol engineered cementitious composites (PVA-ECC). Constr. Build. Mater. 78, 397–404. doi:10.1016/j.conbuildmat.2014.12.071
Parant, E., Rossi, P., and Boulay, C. (2007a). Fatigue behavior of a multi-scale cement composite. Cem. Concr. Res. 37, 264–269. doi:10.1016/j.cemconres.2006.04.006
Parant, E., Rossi, P., and Maou, F. L. (2007b). Durability of a multiscale fibre reinforced cement composite in aggressive environment under service load. Cem. Concr. Res. 37, 1106–1114. doi:10.1016/j.cemconres.2006.02.021
Pierre, R., and Edouard, P. (2008). Damage mechanisms analysis of a multi-scale fibre reinforced cement-based composite subjected to impact and fatigue loading conditions. Cem. Concr. Res. 38, 413–421. doi:10.1016/j.cemconres.2007.09.002
Pourfalah, S. (2018). Behaviour of engineered cementitious composites and hybrid engineered cementitious composites at high temperatures. Constr. Build. Mater. 158, 921–937. doi:10.1016/j.conbuildmat.2017.10.077
Qasim, M., Lee, C. K., and Zhang, Y. X. (2023). Flexural strengthening of reinforced concrete beams using hybrid fibre reinforced engineered cementitious composite. Eng. Struct. 284, 115992. doi:10.1016/j.engstruct.2023.115992
Ramasamy, K. A., and Shanmughasundaram, K. (2018). Flexural performance of hybrid engineered cementitious composite layered reinforced concrete beams. Period. Polytech. – Civ. 62 (4), 921–929. doi:10.3311/ppci.11748
Rawat, S., Zhang, Y. C., and Lee, C. K. (2022). Multi-response optimization of hybrid fibre engineered cementitious composite using Grey-Taguchi method and utility concept. Constr. Build. Mater. 319, 126040. doi:10.1016/j.conbuildmat.2021.126040
Rossi, P., and Parant, E. (2008). Damage mechanisms analysis of a multi-scale fibre reinforced cement-based composite subjected to impact and fatigue loading conditions. Cem. Concr. Res. 38, 413–421. doi:10.1016/j.cemconres.2007.09.002
Shao, Y., Li, Z., and Shah, S. P. (1993). Matrix cracking and interface debonding in fiber-reinforced cement-matrix composites. Adv. Cem. Based Mater. 1 (2), 55–66. doi:10.1016/1065-7355(93)90010-l
Soe, K., Zhang, Y., and Zhang, L. (2013). Material properties of a new hybrid fibre-reinforced engineered cementitious composite. Constr. Build. Mater. 43, 399–407. doi:10.1016/j.conbuildmat.2013.02.021
Tinoco, M. P., and Silva, F. D. A. (2021). On the mechanical behavior of hybrid fiber reinforced strain hardening cementitious composites subjected to monotonic and cyclic loading. J. Mater. Res. Tech. 11, 754–768. doi:10.1016/j.jmrt.2021.01.053
Tran, N. T., Nguyen, T. K., Nguyen, D., and Le, Q. H. (2023). Assessment of fracture energy of strain-hardening fiber-reinforced cementitious composite using experiment and machine learning technique. Struct. Concr. 24 (3), 4185–4198. doi:10.1002/suco.202200332
Voo, J., and Foster, S. (2003). Variable engagement model for fiber reinforced concretein tension UNICIV Report No. R-420. Sydney: The University of New South Wales.
Wang, Y., Li, V. C., and Backer, S. (1988). Modelling of fibre pull-out from a cement matrix. Int. J. Cem. Compos. Lightweight Concr. 10 (3), 143–149. doi:10.1016/0262-5075(88)90002-4
Wang, Z., Zhang, J., and Wang, J. (2014). Tensile performance of polyvinyl alcohol–steel hybrid fiber reinforced cementitious composite with impact of water to binder ratio. J. Compos. Mater. 49 (18), 1–19. doi:10.1177/0021998314542450
Wu, H., and Li, V. C. (1992). Snubbing and bundling effects on multiple crack spacing of discontinuous random fiber-reinforced brittle matrix composites. J. Am. Ceram. Soc. 75 (12), 3487–3489. doi:10.1111/j.1151-2916.1992.tb04457.x
Wu, H., and Li, V. C. (1995). Stochastic process of multiple cracking in discontinuous random fiber reinforced brittle matrix composites. Int. J. Damage Mech. 4 (4), 83–102. doi:10.1177/105678959500400105
Wu, H., and Li, V. C. (1999). Fiber/cement interface tailoring with plasma treatment. Cem. Concr. Compos. 21 (3), 205–212. doi:10.1016/s0958-9465(98)00053-5
Xie, C., Cao, M., Si, W., and Khan, M. (2020). Experimental evaluation on fiber distribution characteristics and mechanical properties of calcium carbonate whisker modified hybrid fibers reinforced cementitious composites. Constr. Build. Mater. 265, 120292. doi:10.1016/j.conbuildmat.2020.120292
Zhang, C., and Cao, M. (2014). Fiber synergy in multi-scale fiber-reinforced cementitious composites. J. Reinf. Plast. Comp. 33 (9), 862–874. doi:10.1177/0731684413514785
Zhang, J., Wang, Q., and Wang, Z. (2016). Properties of polyvinyl alcohol-steel hybrid fiber reinforced composite with high-strength cement matrix. J. Mater. Civ. Eng. 29 (7), 1–9. doi:10.1061/(asce)mt.1943-5533.0001868
Zhang, Y., Deng, M., and Dong, Z. (2019). Seismic response and shear mechanism of engineered cementitious composite (ECC) short columns. Eng. Struct. 192, 296–304. doi:10.1016/j.engstruct.2019.05.019
Zhang, Z. G., Li, Z., He, J., and Shi, X. (2023b). High-strength engineered cementitious composites with nanosilica incorporated: mechanical performance and autogenous self-healing behavior. Cem. Concr. Compos. 135, 104837. doi:10.1016/j.cemconcomp.2022.104837
Zhang, Z. G., Liu, J., Li, J., Qin, F., and Di, J. (2023a). Micromechanics-based analysis of PVA-ECC after thermal exposure. Arch. Civ. Mech. Eng. 23, 213. doi:10.1007/s43452-023-00736-1
Zhang, Z. G., Liu, S., Yang, F., Weng, Y., and Qian, S. (2021). Sustainable high strength, high ductility engineered cementitious composites (ECC) with substitution of cement by rice husk ash. J. Clean. Prod. 317, 128379. doi:10.1016/j.jclepro.2021.128379
Zhang, Z. G., Qin, F., Ma, H., and Xu, L. (2020a). Tailoring an impact resistant engineered cementitious composite (ECC) by incorporation of crumb rubber. Constr. Build. Mater. 262, 120116. doi:10.1016/j.conbuildmat.2020.120116
Zhang, Z. G., Yang, F., Liu, J., and Wang, S. (2020b). Eco-friendly high strength, high ductility engineered cementitious composites (ECC) with substitution of fly ash by rice husk ash. Cem. Concr. Res. 137, 106200. doi:10.1016/j.cemconres.2020.106200
Zhao, X., Li, Q., and Xu, S. (2020). Contribution of steel fiber on the dynamic tensile properties of hybrid fiber ultra-high toughness cementitious composites using Brazilian test. Constr. Build. Mater. 246, 118416. doi:10.1016/j.conbuildmat.2020.118416
Keywords: strain hardening cementitious composite, tensile behavior, hybrid fiber, calcium carbonate whisker, constitutive relationship
Citation: Hou J, Bai J, Mou H and Xiang Z (2024) Tensile properties and constitutive model of cost-effective multiscale hybrid fiber reinforced strain hardening cementitious composites. Front. Mater. 11:1378089. doi: 10.3389/fmats.2024.1378089
Received: 29 January 2024; Accepted: 18 March 2024;
Published: 05 April 2024.
Edited by:
Zhigang Zhang, Chongqing University, ChinaReviewed by:
Hui Li, Hebei University of Technology, ChinaMingfeng Xu, State Key Laboratory of Building Safety and Environment, China
Copyright © 2024 Hou, Bai, Mou and Xiang. This is an open-access article distributed under the terms of the Creative Commons Attribution License (CC BY). The use, distribution or reproduction in other forums is permitted, provided the original author(s) and the copyright owner(s) are credited and that the original publication in this journal is cited, in accordance with accepted academic practice. No use, distribution or reproduction is permitted which does not comply with these terms.
*Correspondence: Jin Hou, aG91amluMTk3NkBob3RtYWlsLmNvbQ==