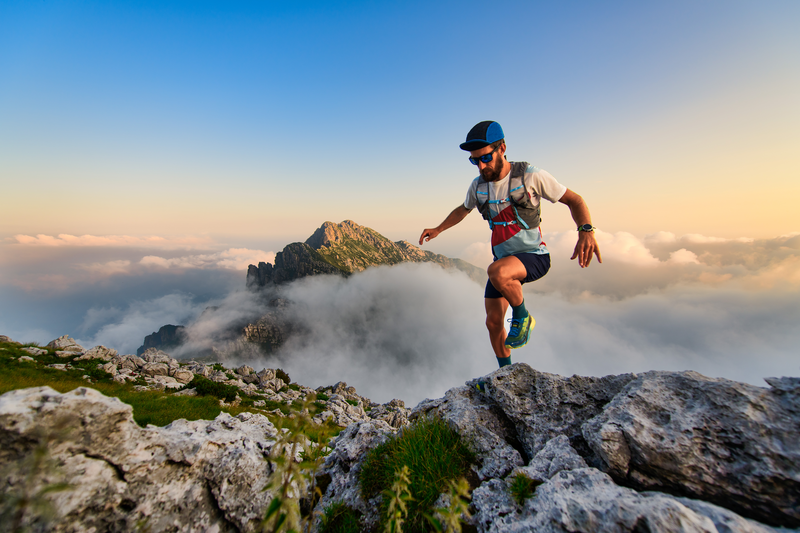
95% of researchers rate our articles as excellent or good
Learn more about the work of our research integrity team to safeguard the quality of each article we publish.
Find out more
ORIGINAL RESEARCH article
Front. Mater. , 21 March 2024
Sec. Mechanics of Materials
Volume 11 - 2024 | https://doi.org/10.3389/fmats.2024.1370921
This article is part of the Research Topic Women In Science: Materials 2023 View all 10 articles
The conservation and repair of XX century architectural heritage built with reinforced concrete is becoming more and more important and requires suitable materials and technical solutions. In particular, effectiveness, compatibility and durability must be ensured, in spite of the limited extent of demolition allowed by the local authorities, and the literature in this field is still limited. In this paper, an experimental campaign was carried out in an historic reinforced concrete floor slab, where different repair mortars were used. After some on-site testing on the corrosion potential, the concrete beams of the slab were integrally cut and transported to the laboratory for a series of systematic tests, aimed at investigating the performance and compatibility of the repair materials, as well as any possible issues hindering the success of this structural intervention. The filling ability, physical compatibility, chemical compatibility and mechanical compatibility of the repair mortars and the corrosion behavior of the steel reinforcement were investigated, deriving some results of general interest, which may contribute to a better insight about the repair of heritage concrete floor slabs.
Reinforced concrete is routinely subjected to periodic repair interventions all over the world (Brindha et al., 2023) because it is affected by different deterioration processes in both concrete (freeze-thaw cycles, chemical attack, lime washout, salt scaling) and steel (corrosion induced by carbonation and chlorides). The rehabilitation may or may not include strengthening (Conde Silva and Serra, 2022) and it is generally carried out by removing deteriorated concrete and rust, applying additional steel reinforcement if necessary, and reconstructing the lost concrete with cement-based mortars, according to the principles described in the European standard EN 1504-3 (European standard EN 1504-3:2005, 2005). These reconstructions may be called patches or overlays, according to the fact that small or large volumes of mortar are applied (Conde Silva and Serra, 2022). Additional strengthening solutions may be adopted, such as the application of fiber-reinforced composites (among the others (Zanotti et al., 2018; Jahangir et al., 2023; Ortiz et al., 2023)).
Repair mortars used for concrete reprofiling/reconstruction are usually dry ready-mix mortars complying with the requirement of the European standard EN 1504-3, which classifies these mortars into four distinct classes, i.e., R1-R2 (mortars for non-structural and “cosmetic” repair in mild environment) and R3-R4 (mortars for structural repairs in severe and harsh environment). Due to the obsolescence of reinforced concrete, the market for these certified dry ready-mix repair mortars is expected to grow at a compound annual growth rate (CAGR) of more than 6% globally from 2023 to 2028 (Mordor Intelligence, 2024). Besides the requirements posed by EN 1504-3, some authors provided additional recommendations for repair mortars (Miranda et al., 2021; Conde Silva and Serra, 2022; Miranda et al., 2022), ranging from compatibility to effectiveness to durability, which points out how challenging the concrete repair by mortars is.
Moreover, when heritage concrete structures are concerned (an increasing issue in society (Miranda et al., 2021)) additional challenges arise (Gaudette, 2000; McDonald and Gonçalves, 2020). In fact:
a) historic concrete is often characterized by low quality, due to high water-to-cement ratio, unsuitable local aggregates (Sotiriadis et al., 2022), etc., possibly causing problems of adhesion and compatibility when repair mortars are applied;
b) local conservation authorities usually allow the demolition and reconstruction of a limited amount of concrete. When concrete exhibits some surface texture or aesthetical finishing, its demolition may even be not allowed (Miranda et al., 2021);
c) the profile of the concrete elements cannot be altered or increased, not to change the building’s aesthetics, so the concrete cover cannot be increased, even in aggressive environment. This is particularly critical considering that historic structures usually have undersized concrete cover.
All these issues make the repair of heritage concrete structures challenging (Courard et al., 2013; Naldini et al., 2023), and new approaches and criteria are often required, as highlighted by the Madrid—New Delhi Document (ISC20C ICOMOS, 2017).
A recent paper focused on the methods to achieve chromatic, texture and finishing compatibility between repair mortars designed case-by-case and concrete surfaces, in the case of exposed concrete (Miranda et al., 2022), while another study investigated the impact of concrete removal techniques on the bond with repair mortar (Yazdi et al., 2023). The use of high-strength textile-reinforced mortar was found to allow the application of a durable and very thin layer (Beßling et al., 2022), while the effectiveness of repair was improved by adding polymers and/or fibers to the formulation of cement-based repair mortars (Kaish et al., 2020; Putri, 2021; Li and Li, 2022). However, the literature about the repair of concrete floor slabs is still limited (Yamamoto, 2000; Thanoon W. A. et al., 2005; Bissonnette et al., 2013) and it does not consider heritage structures. Moreover, it is often reported that current cementitious repair materials used for concrete floor slabs have insufficient adhesion strength and are vulnerable to exfoliation and early cracking (Kim et al., 2020). A recent study focused on the corrosion rate around a repair area caused by the formation of a galvanic cell between the substrate concrete slab and the patch repair mortar, and found that a considerable improvement can be obtained adding microsilica to the mortar (Ghoddousi et al., 2021). Chemically bonded cements, i.e., cements containing magnesium potassium phosphate ceramics (MKPC), were also investigated to obtain ultra-rapid hardening and to hinder crack propagation (Kim et al., 2020), the latter being the most common defect observed in reinforced concrete floor slabs (Thanoon WaleedA. et al., 2005). Another study was addressed to repair and strengthening of reinforced concrete slabs using advanced techniques, including Carbon Fiber Reinforced Polymer (CFRP), ferrocement mix with expanded wire mesh, and steel strips (Tayel et al., 2004). The solutions proposed in the above-mentioned studies were developed for the repair of deteriorated reinforced concrete floor slabs of ordinary nature, hence their applicability to heritage floor slabs should be evaluated for the specific structures, considering also the existing restrains.
In the present paper, the use of different mortars for the repair of a reinforced concrete floor slab built in the 1930s was investigated, in terms of effectiveness and compatibility. The floor slab under testing is composed by a ribbed reinforced slab and hollow bricks, and it covered on the lower side by a layer of hollow brick and plaster, which was removed to investigate the state of the reinforced concrete beams, in view of the assessment of their load bearing capacity. Then, three concrete beams were reprofiled using three different commercial products, which were applied with procedures currently used in real practice, to highlight the performance of these solutions in real conditions. After some onsite inspection and testing, the three reinforced concrete beams were entirely cut and transported to the laboratory, where different characterization tests were carried out.
The experimental campaign was carried out in a public building built in 1934-37 in Predappio (Forlì, Italy) and called “Casa del Fascio” for its use for organizational and entertaining activities of the Fascist National Party (Figure 1). The building has an L-shape in plan and was constructed with a mixed masonry and reinforced concrete structure, while the external finishing is constituted by bricks and travertine slabs cladding. Being in the town where Mussolini was born, this rationalist building is huge, monumental, and oversized for the small town of Predappio, as it had a celebratory character. Soon after the fall of the fascist regime, it was temporarily used in some limited parts and then completely abandoned in the 1990s, being the target of strong “damnatio memoriae” for its ideologic significance. The building was not subjected to any repair and/or maintenance work, and this caused a progressive deterioration, but also ensured the survival of all the original building materials. In the 2010s the Superintendence set a safeguard restriction on this rationalist building for its historic and artistic value (Delizia et al., 2015), hence the municipality, which is the owner, recently launched the call for a restoration project, which is presently in progress. This experimental study was carried out in the frame of the preliminary survey of the building, in view of its conservation and repair.
Figure 1. The former “Casa del Fascio”: (A) front view facing the central square; (B) rear view (the arrow indicates the location of the slab under testing).
Three types of ribbed concrete floor slabs are present in this building, depending on the level: STIMIP A, STIMIP B, i.e., two kinds of floors having extremely low weight which were patented in 1930 in Italy by the Company RDB in Piacenza (Guidi, 1937; Studio Valle Progettazioni s.r.l, 2020), and a mixed floor with ribbed reinforced concrete slab and hollow brick tiles. The restrictions set by the authorities imposed to conserve the original materials as much as possible, but the preservation of the floor slabs is challenging for three main reasons. Firstly, they are affected by severe deterioration due to the prolonged rain infiltration from the roof. Secondly, the floor slabs must ensure the structural performance requested for the new uses of the building, i.e., exhibitions, conferences and cultural activities, according to national laws. Thirdly, any repair and strengthening intervention must be necessarily performed at the intrados, not to damage the upper original floorings. For these reasons, a preliminary testing campaign was considered necessary, to investigate the state of the existing concrete floor slab and the feasibility of the repair solutions. A portion of floor slab was selected for a destructive trial testing, in collaboration with the engineers and architects in charge of the conservation intervention. The selected floor slab is located between the basement and the raised ground floor (Figure 1B) and is constituted by a ribbed reinforced concrete slab and hollow bricks (Figure 2A). The painted plaster at the intrados appeared in good state of conservation, but prevented the examination of the slab materials, hence the layers of thin hollow brick and plaster were demolished and four reinforced concrete ribs (beams) were entirely exposed (Figure 2A). These beams were characterized by exceptionally large aggregates (size up to 8 cm, Figure 2A), totally unfitting to the cross section of the beam, which is 8 cm large. In fact, visible gaps and voids were observed in the beams, confirming that casting the fresh concrete into the formworks was difficult. Likely, at the age of construction the provincial workers were not familiar with concrete yet. At the bottom of each beam, two plain rebars having diameter 10 mm were present (Figures 2B, C). These rebars were placed in a very inaccurate way, i.e., not symmetrically and directly on the formworks, resulting in an almost nonexistent concrete cover. Rust was observed over the rebars’ surface.
Figure 2. (A) Detail of a beam after the demolition of the layers underneath: it is possible to see the large size of the gravel and the irregular position of the longitudinal steel bars; (B) sketch of the reinforced concrete and hollow tiles mixed floor; (C) size of the reinforced concrete beams (sizes in cm, diameter of the rebars in mm).
Given the low quality of concrete and the presence of rust over the steel reinforcement, it was decided to clean the rebars and apply repair mortar at the intrados, reprofiling the beams without any change of size, as requested by the authorities. The application of additional strengthening solutions at the beams’ intrados, such as fiber-reinforced composites, will be evaluated in the future, after the modelling of the seismic behaviour of the building. Three different repair mortars were tested, in the beams A, B and C, respectively (Figure 3). Each beam was ideally divided into three parts having length 80 cm (parts I-III), which were subjected to different treatments:
- In Part I, concrete was removed all around the reinforcing bars, and rust was manually cleaned by steel brush (Figure 3B). Then, the original profile of the beam was recreated by applying a repair mortar.
- In Part II, concrete was removed only in the cover part, i.e., below the steel rebars, and rust was manually cleaned by steel brush only from the exposed surface of the bars (Figure 3B). This condition was investigated to mimic what could happen in practice, if a less radical demolition is carried out. Then, the original profile of the beam was recreated by applying a repair mortar.
- Part III was left without any mortar application, for reference.
Figure 3. Reinforced concrete beams under testing in the selected slab: (A) plan of the beams, with the different parts; (B) schematic representation of the cross-sections in parts I and II; (C,D) the beams after the curing of the different repair mortars.
The application of the repair mortars in Parts I and II was carried out under the direct supervision of the relevant manufacturers.
Beam A was repaired as follows:
- after wetting the original concrete substrate with water, a liquid migrating corrosion inhibitor was applied by brushing on the steel reinforcements. The inhibitor is an aqueous solution whose composition was not disclosed by the manufacturer;
- a thixotropic repair mortar (mortar A) was prepared by adding the dry-mix with an amount of water suitable to obtain the desired workability, according to the common use in the working site. The mortar was applied by trowel at the beam’s intrados, with the help of wood boards to reconstruct the profile of the beam. According to the datasheet provided by the manufacturer, mortar A is a cement-based ready-mix mortar classified as R4 according to EN 1504-3 (mortar suitable for structural repair) and exhibits the following characteristics: bulk density 2,150 kg/m3, adhesion to concrete (according to EN 1504-3) > 2MPa, 28-day compressive strength 50 MPa;
- after removing the boards (about 2 h after the application of the mortar), the same migrating corrosion inhibitor was brushed over the surface of the reprofiled beam.
Beam B was repaired as follows:
- after wetting the original concrete substrate with water, a passivating paste was applied by brushing on both concrete and reinforcement. This paste is composed of Portland cement, fine quartz sand and chemical admixtures;
- a thixotropic repair mortar (mortar B) was prepared by adding the dry-mix with an amount of water suitable to obtain the desired workability, as in the previous case. The mortar was applied by trowel, using wood boards as above. According to the datasheet provided by the manufacturer, mortar B is a cement-based ready-mix mortar classified as R3 according to EN 1504-3 (mortar suitable for structural repair) and exhibits the following characteristics: bulk density 1,500 kg/m3, adhesion to concrete (according to EN 1504-3) > 0.8 MPa, 28-day compressive strength 20 MPa.
Beam C was repaired as follows:
- after wetting the original concrete substrate with water, a thixotropic repair mortar (mortar C) was prepared by adding the dry-mix with an amount of water suitable to obtain the desired workability, as in the previous cases. The mortar was applied by trowel, using wood boards as above. According to the datasheet provided by the manufacturer, mortar C is a mineral ready-mix mortar with low amount of chemical admixtures, classified as R4 according to EN 1504-3 (mortar suitable for structural repair) and exhibits the following characteristics: bulk density 2,050 kg/m3, adhesion to concrete (according to EN 1504-3) > 2 MPa, 28-day compressive strength 45 MPa.
The different parts of the beams were labelled with the letter of the beam (A, B, C) and the part (I, II, III).
The repair materials were repeatedly wetted for the first day after the application, then they were let cure at room condition up to 28 days (Figures 3C, D). After curing, the bar corrosion potential was measured onsite (Section 2.2). Then, the vertical portions of the three T-shaped concrete beams were cut by an angle grinder (Figure 4) and transported to the laboratory for further testing. The remaining floor slab was sustained by a purposely designed steel profiles structure.
Figure 4. The ribs (beams) of the concrete floor slab after cutting (side view) (from right to left: parts I, II and III).
Although the concrete beams are located indoor, in an environment characterized by low humidity, they were subject for about 70 years to significant water infiltration and the observation of the steel reinforcement during the demolition operations highlighted the widespread presence of rust. Hence, some tests were carried out to investigate the behavior of the existing reinforcement with respect to corrosion.
After 28-day curing of the repair mortars, the corrosion potential of the steel reinforcement was measured onsite, to investigate the effectiveness of the treatments in inhibiting the corrosion process. The test was performed according to the Italian standard UNI 10174:2020 (Italian standard UNI 10174:2020, 2020), using a Tecnotest AT 410 (reference electrode Cu/CuSO4, direct current measurement, voltage2 V, input resistance 25 MΩ). For each beam, the electrode was connected to a portion of the uncovered reinforcement in part III, after locally cleaning it from rust, while the reference electrode was placed at the intrados of the beam, along its axis, at points located 20 cm apart. The same measurement was carried out also in the limited portions of the original concrete offering a plain surface (Part III). The electrical continuity of the bars was assumed, based on the observation that clamps are present in the beams.
After transporting the cut beams to the laboratory, characterization tests were carried out on slices that were obtained by sawing, to investigate the different repair solutions.
The carbonated zones of the beams were assessed both in the original concrete and in the repair mortars, by cutting 5 cm thick slices (one from part I and one from part II) and immediately spraying a thymolphthalein solution (0.1 g in a liquid phase consisting of 50 mL ethanol and 50 mL deionized water), which turns from colorless to blue at pH > 10.5. Moreover, fragments of mortars were collected by chisel in three points of other slices, immediately after the cut, to assess the pH values in both the new and the original materials, in the positions shown in Figure 5. In the positions 2 and 3, which are in the original concrete, fragments of the mortar only were collected (without coarse aggregates). The sampling point 2 in Figure 5 was selected to investigate the possible realkalization induced in the original concrete substrate by the application of the different products, some of which are intended to provide this additional effect. The procedure adopted for the pH measurement, called “ex-situ leaching method,” is described in (Behnood et al., 2016). In brief, each mortar fragment was finely ground and then 5 g of the relevant powder were added to 50 mL of deionized water, keeping it under stirring for 24 h in a sealed vessel and finally measuring the pH by a CyberScan pH310 pH-meter (Eutech Instruments). Afterwards, the dispersion was kept at rest for 24 h in sealed conditions and a second measurement was carried out.
Finally, after the end of the other tests (Section 2.2.2), the steel reinforcements were extracted by chisel from the slices of concrete, to allow a direct observation of their surface.
The effectiveness of the different repair mortars was investigated by cutting transversal slices of the beams and observing the cross sections both visually and in an Olympus SZX10 stereo-optical microscope (SOM), to qualitatively evaluate the adhesion between the repair mortars and the substrate (concrete and steel reinforcement).
The compatibility between new and original materials was firstly evaluated by determining the total open porosity (OP%), water absorption (WA%) and bulk density (ρbulk) of the repair mortars and the original concrete. For this purpose, regular prismatic samples were obtained by sawing from the parts I and II of each beam. At least 2 samples per investigated condition were used. The porosity, total water absorption and bulk density were calculated by measuring the dry, saturated and hydrostatic masses of the samples, according to EN 1936:2006 (European standard EN, 1936:2006, 2006). The same prismatic samples, after drying in ventilated oven at 70°C, were tested by a PUNDIT (Portable Ultrasonic Non-destructive Digital Indicating Test, C.N.S. Electronics, frequency 55 kHz, thin rubber sheet among the probes and the material’s surface), to determine the Ultrasonic Pulse Velocity (UPV) of the repair mortars and the original concrete. The test was carried out according to EN 12504-4:2021 (European standard EN 12504-4:2021, 2021). Then, the dynamic elastic modulus (Ed) was calculated through the formula:
Mercury intrusion porosimetry (MIP) was carried out on mortars fragments collected by chisel from the beams, through Thermo Scientific Pascal 140 Series and 240 Series, allowing to measure pores of diameter 116–0.0075 μm. For each beam, the following samples were analyzed:
- a sample of repair mortars, collected from the internal part of the reconstructed volume (likewise the position 1 in Figure 5).
- a sample of repair mortars, collected in adjacence to the interface with the original concrete. This position was selected to investigate if the properties of the repair mortar change at the interface with concrete (e.g., due to mixing water depletion in the mortar), possibly affecting the adhesion (Müller et al., 2006).
- a sample of mortar fraction from the original concrete, collected paying attention to skip the coarse aggregate (likewise the position 3 in Figure 5).
The real density (ρreal) of the three repair mortars was investigated collecting samples of about 10 g, grinding them to powder (<0.063 mm) and testing them in a water pycnometer.
Samples of the repair mortars were collected and characterized in terms of chemical-mineralogical composition by X-ray diffraction (XRD) in a Panalytical Empyrean equipped with a CuKα tube operating at 40 kV and 30 mA. Analyses were performed on dried powder (milled samples) using a 2θ range from 4° to 80° and a step size of 0.026°.
Finally, the presence of soluble salts in the repair mortars and concrete was investigated by ion chromatography in a Dionex ICS 1000. The samples were previously milled to powder, put in boiling deionized water for 10 min and subsequently filtered by blue ribbon filter to obtain the solution to test.
The corrosion potential values measured along the beams are reported in Figure 6. It was not possible to find reliable values for the untreated original concrete, as the values resulted very scattered, likely due to the uneven surface of the material and the presence of void and large aggregates adjoining the steel reinforcements. According to UNI 10174, potential values > −200 mV indicate that corrosion is probably absent (probability >90%), whereas potential values < −350 mV indicate that corrosion is probably ongoing (probability >90%). None of the repair mortars could restore a potential > −200 mV, so according to the mentioned standard the corrosion process was not totally stopped in any case by the mortars’ application. However, the potential values significantly improved passing from part II to part I, confirming the importance of cleaning the whole steel surface. Indeed, an only partial removal of the rust (part II) strongly jeopardizes the treatment’s success, notwithstanding their alleged inhibition effect. The fact that the potential, even in the parts where the repair mortar was applied all around the reinforcement, was not restored to values > −200 mV may be ascribed to an incomplete removal of the rust by manual cleaning and/or to the fact that the material surrounding the rebars was mortar rather than concrete, hence the reference values reported in the standard might be not entirely fitting and specific values should be fixed. Moreover, it is known that potential measurements taken from the concrete surface are influenced not only by the corrosion state of the rebars (active or passive) but also by the concrete cover and resistivity, which varies at least with temperature, moisture, carbonation, thus the reference values of potential should not be used as absolute criteria to determine the condition of steel in concrete (Elsener et al., 2003). However, the comparison of the potential values across different beam sections offers valuable insights into corrosion susceptibility and, although the results could change over time, the measurement performed at 28-day seems adequate for an initial assessment.
Figure 6. Potential measurement along the repaired parts of the beams (parts I and II), together with the location of the measurement points (bottom view).
In terms of comparison among the different repair solutions in Part I of the beams, the measured potential values seem quite comparable, but beam A exhibited a slightly higher potential, likely due to the corrosion inhibitor, although this effect might be ascribed also to some heterogeneity in the beams’ cleaning.
The colorimetric test by thymolphthalein (Figure 7) highlighted that the original concrete was entirely carbonated, while all the repair mortars were highly alkaline, as expected. The pH values measured in the positions of Figure 5 are reported in Table 1. The two set of measurements taken after 24 and 48 h in water (see Section 2.2.1) were basically identical, hence the pH values reported in Table 1 are their average. The original concrete exhibited an average pH equal to 9.5, while the new mortars resulted quite similar each other and with an average pH of 12.4. It is difficult to say if some re-alkalization of the original concrete occurred as a consequence of the application of the new products, because the pH of concrete close to the interface (sampling position 2 in Figure 5) was in some cases unaltered and in some cases slightly higher, so drawing conclusive remarks was not possible. However, even when improved, the pH or the original concrete adjacent to the repair mortars only reached values equal to 10.6, 11.8, and 10.1 (in parts I of beams A, B and C, respectively), so not enough for protection conditions.
Figure 7. Colorimetric tests performed by thymolphthalein on the cross sections of beams (A–C) (some circular blue traces on the concrete are due to the powder of repair mortars spread by the angle grinder during the cut).
Table 1. pH values of mortars in the locations of Figure 5.
The appearance of the reinforcing bars extracted from the slices of the beams is shown in Figure 8. Although the photos provide no insight into the corrosion depth, they show at a glance how rust is present almost everywhere, despite the cleaning of the rebars before the application of the repair products, thus steel brushing was not effective enough in removing the corrosion products. This observation is consistent with the data of the corrosion potential described above. The shiny patina in A I is due to the corrosion inhibitor, while the white layer covering the reinforcement in B I is due to the passivating paste. In C I, the light brown color of the steel is likely due to some mixing between the rust powder and the repair mortar.
Based on microscopic observation of the interface between concrete and repair mortars, all the new products seem able to provide a good adhesion to concrete (Figure 9, above) and steel (Figure 9, below). However, from a macroscopic point of view, several gaps are visible between the new and the original materials (Figure 10). The insufficient filling ability of the repair mortars seems due to their low flowability, necessary for ceiling application but counterproductive for the filling ability. An alternative application technology should be developed, involving the casting of the mortar (Ana Bras et al., 2013). Figure 10 shows that the original concrete exhibits very coarse aggregates, large voids and an asymmetric arrangement of the steel rebars, as observed onsite.
Figure 9. SOM images. For each beam: interface between repair mortar and original concrete (above) and surface of the steel rebars after their extraction (below). Marker: 1 mm.
Concerning microstructural compatibility, the results are reported in Table 2. The original concrete exhibits a bulk density equal to 2.3 kg/dm3, which is in line with the typical values for this kind of material, while all the three repair mortars exhibit a much lower bulk density (in the range 1.8–1.9 kg/dm3). The use of lightweight materials is generally considered a positive feature in structural strengthening of existing buildings. The bulk densities of the repair mortars are different from the values reported in their technical datasheets, due to the presence of air entrapped during the hand mixing (Figure 12) and/or to the fact that the water was added to the dry mixes onsite just to reach a suitable workability, as in real practice, without considering the amount reported in the datasheets. Consequently, the mortars exhibit a higher open porosity and water absorption than concrete. However, mortar B, despite having a bulk density equal to mortars A and C, exhibits a much lower open porosity and water absorption. Considering that the real density of the mortars is the same, the low open porosity of mortar B suggests the presence in the mix of some lightweight aggregates having close porosity. This was confirmed through optical microscopy, as small spheres having a foam appearance were observed (Figure 11).
Table 2. Values of bulk density (ρbulk), open porosity (OP) and water absorption (WA) and real density (ρreal).
The pore size distribution of the mortars in terms of differential curves obtained by MIP is reported in Figure 12. Pores are generally larger in the three mortars than in the mortar fraction collected from the original concrete, which is consistent with their light weight. However, as large air voids are not properly detected by MIP analysis (Franzoni et al., 2017), SOM observations were performed too. Large and round-shaped air bubbles were found in all the repair mortars (Figure 13), suggesting the use of air-entraining agents for lightweight purposes. The MIP analysis carried out on the repair mortars’ samples adjacent to the original concrete are not reported for brevity’s sake, as they showed no significant difference. Thus, the wetting of the original concrete was sufficient and no water depletion occurred in the repair mortars close to the interface.
Figure 12. Differential MIP curves of the three repair mortars and the original concrete (mortar fraction only).
Mechanical properties determined via ultrasonic testing are reported in Table 3. The original concrete, being less porous than mortars, exhibits a higher UPV value, as expected. However, when Ed is calculated, the materials’ density plays a strong role and the dynamic elastic modulus of all the mortars is much lower compared to concrete (−49%, −32%, −46% for mortars A, B and C, respectively). This mismatch between the stiffness of the original and repair materials might decrease the structural effectiveness of the intervention, as the material with higher elastic modulus will proportionately be more overloaded (Conde Silva and Serra, 2022). This effect may be particularly significant when a large portion of concrete is demolished and repointed, to improve the corrosion behavior of the rebars.
The results of XRD carried out on the repair mortars are shown in Table 4. All the samples contain a dominant fraction of quartz, due to the aggregate. In mortar A, a significant presence of feldspar (albite) was found, ascribed to the aggregate, and a minor amount of calcite, possibly present in the aggregate and/or in the binder. The phases of the hydrated binder are not visible, likely due to their low crystallinity, but a minor amount of ettringite was detected, which suggests some sulphate presence in the mortar and a binder essentially based on Portland cement, consistently with the class R4 of the mortar. Notably, no requirement on sulphate ion content is given for repair mortars in EN 1504-3. In mortar B, besides quartz, a significant amount of calcite is present, and also a minor amount of C2S (larnite), which was still not hydrated after 28 days of curing, hence some hydraulic lime seems present besides cement, resulting in a slightly lower strength class for this mortar compared to the other two (C3 instead of R4). In mortar C, quartz and calcite were dominantly present, with minor amounts of albite, i.e., a feldspar fraction in the aggregate, and ettringite, resulting from the reaction of Portland cement with sulphates. Some presence of gismondine was found too. Microcrystalline zeolites of the gismondine family are often reported in alkali-activated and blended cement systems (Okoronkwo et al., 2021).
Table 4. Results of XRD carried out on repair mortars: +++ = dominantly present, ++ = present, + = present in minor amount, – = not present. Q = quartz, C = calcite, A = albite (plagioclase feldspar group), E = ettringite, L = larnite (C2S), G = gismondine (zeolite group).
The presence of salts in the concrete and repair mortars was measured by ion chromatography, and the results are reported in Table 5 for the sulphate anions only, as the amounts of chloride and nitrate were negligible (<0.06%). Mortars A and C contain a high amount of sulphate, probably responsible for the ettringite found by XRD and potentially negative on long term.
The repair solution investigated in this study allowed to work at the intrados of the slab, conserving the original floors and limiting the demolition of the existing concrete, as requested due to the heritage character of this building. The results obtained in this study allowed to derive the following conclusions:
- The existing concrete, which dates back the 1930s, has distinctive features, i.e., unsuitably high maximum diameter, air voids, extremely thin cover and an irregular positioning of the steel rebars. These characteristics have an impact on the applicability of some of the currently used characterization techniques. For example, it was impossible to determine the corrosion potential. Moreover, considering that a sample of concrete is generally considered representative if its size is higher than three times the maximum aggregate size, in this case the size of the sample should have been higher than the size of the beam.
- The technology used for the partial reconstruction of reinforced concrete slabs dramatically influences the success of the intervention, having a role which seems equally important than the materials’ properties. An incomplete removal of rust from the steel rebars and/or an incomplete removal of concrete around the rebars hinder the reestablishment of potentials that guarantee the absence of corrosion. The ceiling application of repair mortars by trowel does not ensure a proper filling of the volume, and the presence of air gaps may jeopardize the adhesion of mortars to the substrate. The flowability of the mortar and the application technique should be developed together, to ensure the best outcome of the reconstruction.
- The potential measurement was successfully carried out onsite according to a UNI standard procedure, but the threshold values proposed in the standard are probably suitable neither for repair mortars nor for this kind of concrete with extraordinary coarse aggregate. Further collection of data in real structures would help in obtaining a more in-depth insight about this aspect. However, the comparison of corrosion potential values across different beam sections offers valuable insights into corrosion susceptibility and may constitute a useful tool especially on a comparative basis.
- None of the investigated treatments/materials was able to reestablish the original highly alkaline conditions in the concrete substrate, not even close to the interface.
- All the three investigated mortars exhibit a good adhesion capability, although the presence of macroscopic voids at the interface between mortar and concrete may obviously jeopardize the structural continuity.
- The three investigated mortars exhibit a much higher porosity, a much lower density and larger pores than the original concrete, also due to the presence of air bubbles likely due to air-entraining agents. On the one hand, the repair intervention benefits from this weight decrease, while on the other hand the mechanical properties of the reconstructed part in terms of dynamic elastic modulus and hence stiffness result very different from those of the concrete. This aspect should be evaluated in terms of structural behavior of the entire element, to elucidate if this mismatch is significant.
- A very high amount of sulphate ions was found in two of the investigated ready-mix mortars, which may cause potential damage on long term, due to the formation of expansive ettringite upon reaction with the aluminates contained in Portland cement. Indeed, some ettringite was found in the two relevant mortars.
Further analysis will be necessary to understand if the repaired concrete slab ensures the requested load capacity for the new uses of the buildings, or additional strengthening solutions are necessary, such as the application of fiber-reinforced polymer (FRP) or fiber-reinforced cementitious matrix (FRCM) composites at the intrados. The applicability of externally bonded fiber-reinforced composites is often allowed in heritage concrete structures thanks to their ability to provide a significant strengthening with an extremely limited weight and volume increase of the original structure. In this case, the preliminary application of a repair mortar over the original (and defective) concrete, besides giving the benefits shown above, may also provide a suitable substrate for composites’ application.
The raw data supporting the conclusion of this article will be made available by the authors, without undue reservation.
EF: Conceptualization, Formal Analysis, Investigation, Methodology, Supervision, Writing–original draft, Writing–review and editing. CP: Conceptualization, Data curation, Formal Analysis, Investigation, Methodology, Writing–review and editing.
The author(s) declare that no financial support was received for the research, authorship, and/or publication of this article.
Veronica Manzini and Beatrice Verasani are gratefully acknowledged for their contribution in the tests. Ing. Alberto Gentili, Arch. Giancarlo Gatta and Arch. Cesare Valle, members of the restoration project team, are gratefully acknowledged for giving the authors this opportunity of collaboration.
The authors declare that the research was conducted in the absence of any commercial or financial relationships that could be construed as a potential conflict of interest.
All claims expressed in this article are solely those of the authors and do not necessarily represent those of their affiliated organizations, or those of the publisher, the editors and the reviewers. Any product that may be evaluated in this article, or claim that may be made by its manufacturer, is not guaranteed or endorsed by the publisher.
Ana Bras, , Gião, R., Lúcio, V., and Chastre, C. (2013). Development of an injectable grout for concrete repair and strengthening. Cem. Concr. Compos. 37, 185–195. doi:10.1016/j.cemconcomp.2012.10.006
Behnood, A., Van Tittelboom, K., and De Biele, N. (2016). Methods for measuring pH in concrete: a review. Constr. Build. Mater. 105, 176–188. doi:10.1016/j.conbuildmat.2015.12.032
Beßling, M., Groh, M., Koch, V., Auras, M., Orlowsky, J., and Middendorf, B. (2022). Repair and protection of existing steel-reinforced concrete structures with high-strength, textile-reinforced mortars. Buildings 12 (10), 1615. doi:10.3390/buildings12101615
Bissonnette, B., Courard, L., Beushausen, H., Fowler, D., Trevino, M., and Vaysburd, A. (2013). Recommendations for the repair, the lining or the strengthening of concrete slabs or pavements with bonded cement-based material overlays. Mater. Structures/Materiaux Constr. 46 (3), 481–494. doi:10.1617/s11527-012-9929-8
Brindha, U., Maheswaran, J., Chellapandian, M., and Arunachelam, N. (2023). Quantitative assessment of strengthening strategies and design recommendations for the repair of corrosion-damaged reinforced concrete members. Buildings 13 (4), 1080. doi:10.3390/buildings13041080
Conde Silva, J., and Serra, C. (2022). A review on cement-based materials and practices for rehabilitation, retrofitting, and strengthening of hydraulic structures. Pract. Periodical Struct. Des. Constr. 27 (3), 03122002. doi:10.1061/(asce)sc.1943-5576.0000692
Courard, L., Bissonnette, B., Beushausen, H., Fowler, D., Trevino, M., Alex, V., et al. (2013). Recommendations for the repair, the lining or the strengthening of concrete slabs or pavements with bonded cement-based material overlays. Mater. Struct. 46, 481–494. doi:10.1617/s11527-012-9929-8
Delizia, F., Di Francesco, C., Di Resta, S., and Pretelli, M. (2015). “La Casa del Fascio di Predappio nel panorama del restauro dell’architettura contemporanea,” in Contributi per aiutare a scegliere (Bologna, Italy: Bononia University Press).
Elsener, B., Andrade, C., Gulikers, J., Polder, R., and Raupach, M. (2003). Half-cell potential measurements - potential mapping on reinforced concrete structures. Mater. Structures/Materiaux Constr. 36 (261), 461–471. doi:10.1007/bf02481526
European standard EN 12504-4:2021, 2021 Testing concrete in structures Determination of ultrasonic pulse velocity, https://www.en-standard.eu/bs-en-12504-4-2021-testing-concrete-in-structures-determination-of-ultrasonic-pulse-velocity/.
European standard EN 1504-3:2005. 2005, Products and systems for the protection and repair of concrete structures — definitions, requirements, quality control and evaluation of conformity — Part 3: structural and non-structural repair, https://knowledge.bsigroup.com/products/products-and-systems-for-the-protection-and-repair-of-concrete-structures-definitions-requirements-quality-control-and-evaluation-of-conformity-structural-and-non-structural-repair?version=standard.
European standard EN 1936:2006, 2006, Natural stone test methods- Determination of real density and apparent density, and of total and open porosity, https://standards.iteh.ai/catalog/standards/cen/92668a0a-2aa1-417a-b996-7cd0bf0fc396/en-1936-2006.
Franzoni, E., Leemann, A., Griffa, M., and Lura, P. (2017). The “Terranova” render of the Engineering Faculty in Bologna (1931–1935): reasons for an outstanding durability. Mater. Struct. 50 (5), 221. doi:10.1617/s11527-017-1083-x
Gaudette, P. (2000). “Special considerations in repair of historic concrete,” in Concrete repair bulletin (St. Paul, Minnesota, USA: International Concrete Repair Institute Inc).
Ghoddousi, P., Haghtalab, M., and Shirzadi Javid, A. A. (2021). Experimental and numerical investigation of repair dimensions effect on macro-cell corrosion induced by concrete slabs patch repair. Int. J. Civ. Eng. 19 (9), 1091–1110. doi:10.1007/s40999-021-00620-0
Guidi, G. (1937). Solai in cemento armato con soletta in laterizio ed autarchia economica, L’architettura Italiana. Period. Mens. Di Archit. Tec. 2, 61–67.
ISC20C ICOMOS, Madrid – New Delhi document. Approaches to the conservation of twentieth-century cultural heritage, https://openarchive.icomos.org/id/eprint/2677/, 2017
Italian standard UNI 10174:2020, 2020, “Istruzioni per l'ispezione delle strutture di calcestruzzo armato esposte ad ambienti aggressivi mediante mappatura di potenziale”, https://store.uni.com/uni-10174-2020.
Jahangir, H., Nikkhah, Z., Eidgahee, D. R., and Esfahani, M. R. (2023). Performance based review and fine-tuning of TRM-concrete bond strength existing models. J. Soft Comput. Civ. Eng. 7 (1), 43–55.
Kaish, A. B. M. A., Sahari, E. I., and Fong, N. L. (2020). Development of self-compacting fibre reinforced structural mortar for concrete repair. IOP Conf. Ser. Mater. Sci. Eng. 713 (1), 012030. doi:10.1088/1757-899x/713/1/012030
Kim, H., Han, D., Kim, K., and Romero, P. (2020). Performance assessment of repair material for deteriorated concrete slabs using chemically bonded cement. Constr. Build. Mater. 237, 117468. doi:10.1016/j.conbuildmat.2019.117468
Li, W., and Li, Z. (2022). Bonding and repairing properties of fiber-polymer mortar for concrete repair. Adv. Mater. Sci. Eng. 2022, 1–8. doi:10.1155/2022/7663729
McDonald, S., and Gonçalves, A. (2020). Conservation principles for concrete of cultural significance, principles. Los Angeles, CA, USA: Getty Conservation Institute.
Miranda, J., Costa, H., Valença, J., Do Carmo, R., and Júlio, E. (2021). Design and durability assessment of restoring mortar for concrete heritage. Materials 14 (16), 4508. doi:10.3390/ma14164508
Miranda, J., Valença, J., Costa, H., and Júlio, E. (2022). Methodology for the restoration of heritage built in exposed concrete. The case study of ‘Piscina das Marés’, Portugal. case study ‘Piscina das Marés’, Portugal, Constr. Build. Mater. 328, 127040. doi:10.1016/j.conbuildmat.2022.127040
Mordor Intelligence, (2024). Concrete repair mortar market size & share analysis - growth trends & forecasts (2023 - 2028). India (accessed online on June 2023 at https://www.mordorintelligence.com/industry-reports/concrete-repair-mortar-market.
Müller, A., and Zilch, K. (2006). “Examination and evaluation of factors influencing the bond strength between old and new concrete,” in 2nd international RILEM symposium on advances in concrete through science and engineering. Editor J. Marchand, (RILEM Publications SARL), 1–13.
Naldini, S., Ioannou, I., Hadjimichael, M., Musso, S. F., Pompejano, F., and Dušek, O. (2023). Legislation and practice: the case of historic concrete buildings. J. Cult. Herit. Manag. Sustain. Dev., 1–16. doi:10.1108/jchmsd-03-2022-0048
Okoronkwo, M. U., Mondal, S. K., Wang, B., Ma, H., and Kumar, A. (2021). Formation and stability of gismondine-type zeolite in cementitious systems. J. Am. Ceram. Soc. 104 (3), 1513–1525. doi:10.1111/jace.17572
Ortiz, J. D., Khedmatgozar Dolati, S. S., Malla, P., Nanni, A., and Mehrabi, A. (2023). FRP-Reinforced/Strengthened concrete: state-of-the-art review on durability and mechanical effects. Materials 16 (5), 1990. doi:10.3390/ma16051990
Putri, P. M. (2021). Study of mortar creep with additional polymer materials for concrete repair. J. Phys. Conf. Ser. 1912 (1), 012061. doi:10.1088/1742-6596/1912/1/012061
Sotiriadis, K., Aspiotis, K., Mazur, A., Tolstoy, P., Badogiannis, E., and Tsivilis, S. (2022). Characterization of old concrete from a heritage structure of inousses cluster of islands. Lect. Notes Civ. Eng. (LNCE) 209, 80–89.
Studio Valle Progettazioni s.r.l, . and others, “Lavori di restauro e rifunzionalizzazione della Ex Casa del Fascio e dell’Ospitalità di Predappio - linee guida per lo sviluppo della progettazione. Roma, Italy: Predappio Municipality to the Architectural firm, 2020
Tayel, M. A., Meleka, N. N., and Ramadan, A. M. (2004). Experimental evaluation of advanced techniques for repair and strengthening of reinforced concrete slabs. AEJ - Alexandria Eng. J. 43 (3), 381–389.
Thanoon, W. A., Jaafar, M. S., Kadir, M. R. A., and Noorzaei, J. (2005a). Repair and structural performance of initially cracked reinforced concrete slabs. Constr. Build. Mater. 19 (8), 595–603. doi:10.1016/j.conbuildmat.2005.01.011
Thanoon, W. A., Jaafar, M. S., Kadir, M. R. A., and Noorzaei, J. (2005b). Repair and structural performance of initially cracked reinforced concrete slabs. Constr. Build. Mater. 19, 595–603. doi:10.1016/j.conbuildmat.2005.01.011
Yamamoto, T. (2000). Repair of deteriorated reinforced concrete slabs. American Concrete Institute, ACI Special Publication, SP-193, San Francisco, CA, USA, 151–162.
Yazdi, M. A., Gruyaert, E., Van Tittelboom, K., and De Belie, N. (2023). A new research strategy in studying and improving the bond between concrete and repair mortar. RILEM Bookseries 40, 781–788. doi:10.1007/978-3-031-21735-7_83
Keywords: heritage concrete, corrosion potential, compatibility, adhesion, porosity, dynamic elastic modulus
Citation: Franzoni E and Pizzigatti C (2024) Onsite and laboratory assessment of repair mortars for reinforced concrete floor slabs in heritage buildings. Front. Mater. 11:1370921. doi: 10.3389/fmats.2024.1370921
Received: 15 January 2024; Accepted: 21 February 2024;
Published: 21 March 2024.
Edited by:
Tadeja Kosec, Slovenian National Building and Civil Engineering Institute, SloveniaReviewed by:
Nina Gartner, Slovenian National Building and Civil Engineering Institute, SloveniaCopyright © 2024 Franzoni and Pizzigatti. This is an open-access article distributed under the terms of the Creative Commons Attribution License (CC BY). The use, distribution or reproduction in other forums is permitted, provided the original author(s) and the copyright owner(s) are credited and that the original publication in this journal is cited, in accordance with accepted academic practice. No use, distribution or reproduction is permitted which does not comply with these terms.
*Correspondence: Elisa Franzoni, ZWxpc2EuZnJhbnpvbmlAdW5pYm8uaXQ=
Disclaimer: All claims expressed in this article are solely those of the authors and do not necessarily represent those of their affiliated organizations, or those of the publisher, the editors and the reviewers. Any product that may be evaluated in this article or claim that may be made by its manufacturer is not guaranteed or endorsed by the publisher.
Research integrity at Frontiers
Learn more about the work of our research integrity team to safeguard the quality of each article we publish.