- Analytical Chemistry, Nutrition and Food Sciences Department, University of Alicante, Alicante, Spain
Antioxidant/antifungal active films based on bio-polyethylene (BPE) added with Nutrabiol®T90 and Tocobiol®Plus natural extracts rich in tocopherols at different concentrations (1 and 3 wt%) were developed by melt-blending followed by compression-molding. Thermal, barrier, mechanical, optical and functional properties of the developed active films were evaluated. The addition of active extracts did not cause important changes in thermal and barrier properties of active films whereas a significant increase in oxidative stability was found. Release kinetics of active additives migration showed higher tocopherol diffusion coefficient and antioxidant capacity, determined by the DPPH and ABTS methods, for films containing 3 wt% of Nutrabiol®T90. This film also delayed polymer oxidation in 260 min and extended strawberries shelf-life during storage for 7 days compared to neat BPE, showing a significant antifungal effect. The studied biomaterials offer great potential to substitute conventional petrol-derived materials at the industrial scale as active food packaging systems to increase the shelf-life of perishable foodstuff.
1 Introduction
In recent years, there has been growing concern about the increasing amount of plastic waste from everyday life, as indicated by United Nations (UN) in their 2030 Agenda for Sustainable Development (UN, 2023). Different types of synthetic plastics are currently being used for a wide range of applications, but considerable attention has been given to “green” bioplastics in order to reduce the impact of petroleum-based plastics and waste materials in the environment (Siracusa and Blanco, 2020; Findrik and Meixner, 2023). Low density polyethylene (LDPE) and high density polyethylene (HDPE) are polymers with many industrial applications, including food packaging, by their good properties, such as low permeability, odorless character, transparency, low cost and no toxicity (Barros et al., 2023). However, LDPE and HDPE are derived from petroleum and show high environmental impact, reducing the sustainability in their applications. Bio-polyethylene (BPE) has been proposed as a good and “green” alternative since it is produced from renewable, such as ethanol obtained from sugarcane, and it is polymerized by using the same method than conventional polyethylene sources, with no use of hazardous chemicals and reagents (Mendieta et al., 2020). BPE has gained special attention since its fossil-based counterpart is one of the most used plastics worldwide (Siracusa and Blanco, 2020) and one of the most significant contributors to global greenhouse gases (GHG) among conventional plastics. Therefore, the use of BPE, offering similar structure and operating properties to HDPE, has clearly increased to reach 12.3% of the bioplastics market in 2023 (https://www.european-bioplastics.org/market/).
The addition of active compounds has been widely proposed as a way to improve polymer properties. Particularly, different compounds were used in the field of active food packaging to improve antioxidant (Roy et al., 2021; Gengatharan and Hafiz, 2023) and antimicrobial properties (Severo et al., 2021; Manzoor et al., 2023), obtaining films able to extend the food shelf-life while reducing the amount of synthetic additives in food products. In the case of BPE, carbon fibers were used to reinforce mechanical properties (Bajwa et al., 2019; Bazan et al., 2020); but biocomposites based on the use of synthetic fibers and biopolymers do not completely solve the environmental problems associated with the use of materials of fossil origin. Other studies focused on the formulation of fully bio-based composites between BPE and agricultural fillers, such as wood flour, flax fibers and walnut shell flour (Mazur et al., 2020), which were directly incorporated into the biopolymer matrix without any pre-treatment to increase the sustainability of the biocomposite. But these reinforcing capabilities are not linked to other important functionalities in food packaging, such as antioxidant/antimicrobial properties.
The most usual way to get these functionalities is by the addition of synthetic compounds, such as butylated hydroxytoluene (BHT) and butylated hydroxyanisole (BHA), two efficient antioxidants which traditionally have shown good results with many polymer matrices. However, their high migration rates make them potentially harmful in food packaging materials (Lu et al., 2022). Indeed, the European Food Safety Authority (EFSA) has published studies showing evidence of some toxicity by synthetic antioxidants, such BHT and BHA, which possess carcinogenic effects at high levels (EFSA Panel on Food Additives and Nutrient Sources added to Food (ANS), 2011; EFSA Panel on Food Additives and Nutrient Sources added to Food (ANS), 2012). This is the reason why the substitution of synthetic additives by natural ones, such as essential oils or plant extracts, is a developing topic in the last few years, especially in food products and packaging (Othman et al., 2023). Active food packaging films based on LDPE were developed with the incorporation of curcumin (Zia et al., 2019), resveratrol (Glaser et al., 2019), thymol and linalool (Chang et al., 2022) among others, concluding that LDPE-based films have a broad spectrum of application in active food packaging with specific functionalities. This is a highly promising possibility, but the current legislation still represents a drawback, since just a short list of natural compounds for food packaging have been permitted in their use as food additives. In this regard, not only essential oils extracted from natural sources, but also commercial natural additives show great advantages to develop new functional materials. Nutrabiol®T90 and Tocobiol®Plus are commercial natural extracts rich in tocopherols with antioxidant/antimicrobial performance. Tocopherols have been used as an efficient antioxidant compound with good results in food packaging applications (Mellinas et al., 2020). In addition, polypropylene (PP) films modified with different chain extenders were developed to control the release rate of low molecular weight antioxidants, including tocopherols (López De Dicastillo et al., 2013). For instance, polypropylene glycol (PPG)-polyethylene glycol (PEG) triblock copolymers were used, resulting in the separation of polymer chains, facilitating the molecular movement and increasing the release rate of tocopherols (Castro López et al., 2012). Commercial tocopherols were already used to develop antioxidant films based on LDPE to extend the shelf-life of fish (Barbosa-Pereira et al., 2013), being films with 5 wt% of Nutrabiol®T90 the most effective for salmon preservation under long-term storage. Tocopherols are employed in different fields, including dietary supplements for humans and animals, and antioxidant and preservative additives in food and beverages (Delgado et al., 2020).
However, the use of natural or synthetic antioxidants could have a significant effect on mechanical, barrier and structural properties of the polymer matrix. For instance, the incorporation of curcumin (7 wt%) to LDPE causes the enhancement of the elastic modulus, and the decrease of elongation at break, reaching 47% and 68%, respectively, respect to the neat polymer (Zia et al., 2019). Different changes into morphology and microstructure of neat polymers have been reported due to the incorporation of bioactive compounds. Cinnamon essential oil produces a rougher structure together with holes in zein films due to evaporation. In another study, SEM images revealed that murta leaf extract showed adverse effects on surface morphology owing to the formation of new cross-link bonds, although caffeic acid improved the structure of chitosan-starch film (Sabaghi et al., 2022). Therefore, it is necessary to evaluate the functional properties of the bioactive compounds, not only on the packaged food but also on the inherent properties of the polymer matrix.
The aim of this work is to study the effect of the addition of two different natural extracts rich in tocopherols (Nutrabiol®T90 and Tocobiol®Plus) on the intrinsic properties of commercial BPE (thermal stability, mechanical, color and barrier) as well as the functional properties (antifungal and antioxidant activity) considering the intended application as food active packaging materials. Commercially available additives rich in tocopherols were added to BPE. The effects of type and amount of natural extracts on the release of tocopherol to 50% (v/v) ethanol food simulant with time were studied. Moreover, kinetics and mathematical modelling of tocopherol release from the polymer matrix were also evaluated. Additionally, the effect of these materials in highly perishable food preservation, such as strawberries, was also evaluated.
2 Materials
Green high-density polyethylene (BPE) BS002W, obtained from sugar cane (density 0.965 g cm−3, melt flow rate (190°C) 0.33 g/10 min) was supplied by Braskem (Braskem Ideasa, Sao Paulo, Brazil) and it was used as polymer matrix. Natural extracts rich in tocopherols (Nutrabiol®T90 and Tocobiol®Plus) were kindly provided by Biotecnologías Aplicadas S.L. (BTSA, Madrid, Spain). Nutrabiol®T90 was composed of a mix of tocopherols (minimum 90 wt%) in soybean oil, whereas Tocobiol®Plus composition was 4 wt% of squalene, 9.2 wt% of sterols, 19 wt% of monoglycerides, 20 wt% ascorbyl palmitate and a remaining mixture of tocopherols in soybean oil. Ethanol absolute 99.9%, methanol HPLC grade, acetonitrile HPLC grade, 2,2-diphenyl-1-picrylhydrazyl (DPPH), 2,2′ azino-bis (3-ethylbenzothiazoline)-6-sulfonic acid (ABTS), potassium persulfate, and calcium chloride were purchased from Sigma–Aldrich (Madrid, Spain). Strawberries of the “Sabrina” variety were purchased in a local supermarket (Alicante, Spain).
3 Methods
3.1 Films preparation
Polymer mixtures were obtained by melt-blending in a Haake Polylab QC mixer (ThermoFischer Scientific, Walham, MA, United States) with a mixing time of 5.5 min at 180°C with a rotor speed of 50 rpm. Films were obtained by compression-molding at 180°C in a hot-plates press (Carver Inc 3850, Wabash, IN, United States) and kept at atmospheric pressure for 5 min until melted and pressed at 2 MPa for 1 min, 3.5 MPa for 1 min and finally 5 MPa for 5 min to eliminate the trapped air bubbles. Different active formulations up to four (Table 1) were produced containing 1 and 3 wt% of Nutrabiol®T90 (M1 and M3, respectively) and 1 and 3 wt% of Tocobiol®Plus (T1 and T3, respectively). A control film using neat BPE was also obtained and characterized. The average thickness of these films, considering all formulations, was 0.28 ± 0.02 mm (n = 15, mean ± SD).
3.2 Films characterization
3.2.1 Thermal analysis
Thermogravimetric analysis (TGA) tests were performed, in triplicate, with a TGA/SDTA 851 thermal analyzer (Mettler Toledo, Schwarzenbach, Switzerland). Approximately 6 mg of samples were heated from 25ºC to 700°C at 10°C min−1 under nitrogen atmosphere (50 mL min−1) (Busolo and Lagaron, 2015). Initial degradation temperatures were evaluated at 5 wt% of the initial sample weight.
Differential scanning calorimetry (DSC) triplicate tests were used to determine crystallization and melting temperatures and enthalpies using a TA DSC Q-2000 instrument (New Castle, DE, USA) under nitrogen atmosphere (50 mL min−1). Samples (around 4 g) were equilibrated to −90°C and maintained in isothermal mode for 3 min, heated from −90ºC to 200°C, then cooled to −90°C and further heated to 200°C (all steps at 10°C min−1). Eq. 1 was used to calculate the percentage of crystallinity (
where
DSC was also used to evaluate the oxidative stability of BPE-based films by determining oxidation onset temperature (OOT) and oxidation induction time (OIT). For OOT, samples were heated at 10°C min−1 under a pure oxygen atmosphere (50 mL min−1) from 25°C until observation of the exothermic oxidation peak. OOT was calculated as the temperature corresponding to the intersection between the baseline and the slope of the exothermic peak in each case. Then, OOT values obtained for the control sample (neat BPE) were used to fix the adequate temperature to obtain the OIT values. OIT test was carried out by heating samples at 100°C min−1 under nitrogen (50 mL min−1) to the set temperature (200°C). After 5 min, the atmosphere was switched to pure oxygen or air (50 mL min−1). The heat flow was then recorded under isothermal conditions up to the detection of the exothermic peak, indicating the beginning of the oxidation reaction. OIT value was determined as the difference between the time at the intersection between the baseline and the tangent of the exothermic oxidation peak and the time for the gas switching in two different atmospheres: pure oxygen and nitrogen.
3.2.2 Barrier properties
Water vapor permeability (WVP, kg·m·Pa−1·s–1·m–2) was determined, in triplicate, gravimetrically using UNE 53097:20002 standard and it was calculated according to Eq. 2:
where WVT (kg·s–1·m–2) is the water vapor transmission rate, e (m) is the average film thickness and ΔP is the vapor pressure difference between the two sides of the specimens (Pa). Samples (90 mm diameter circles) were sealed with paraffin to the test stainless steel dishes containing anhydrous calcium chloride (pre-dried at 200°C for 2 h) as desiccant agent. Dishes were placed in a climate chamber at 23°C ± 1°C and RH 50% ± 2% and they were weighed periodically until the steady state was reached.
An oxygen permeation analyzer (8500 model Systech, Metrotec S.A, Spain) was used for OTR (oxygen transmission rate) tests, in triplicate based on ASTM D1434-23. Pure oxygen (99.9%) was introduced into the upper half of the diffusion chamber while nitrogen was injected into the lower half, where an oxygen sensor was located. Films were cut into 14 cm diameter circles and they were clamped in the diffusion chamber at 25°C before testing. Results were expressed as oxygen transmission rate per film thickness.
3.2.3 Mechanical properties
Tensile properties of films were determined with a 3340 Series Single Column System Instron Instrument, LR30K model (Fareham Hants, United Kingdom) equipped with a 2 kN load cell. Tensile parameters were calculated from stress-strain curves according to ASTM D882-09 standard. Before testing, samples were conditioned for 24 h at 23°C and 50% RH. Tests were performed with 100 × 10 mm2 rectangular probes and initial grip separation of 60 mm. The specimens were stretched at 10 mm min−1. Results were the average of five measurements.
Hardness tests were conducted using a Shore A durometer (J Bot Instruments S.A, Barcelona, Spain), according to UNE-EN-ISO 868:2003 and a measuring time of 15 s, and five hardness measurements were obtained.
3.2.4 Color and optical properties
Color modifications on BPE-based films caused by the addition of the active extracts were studied by using a Konica CM-3600d COLORFLEX-DIFF2 colorimeter (HunterLab, Reston, VA, USA). Color values were expressed as L* (lightness), a* (red/green) and b* (yellow/blue) coordinates in the CIELab color space, and they were determined at five different locations in film surfaces. Average values were calculated and total color difference (ΔE) was determined according to Eq. 3:
where L, a, and b are color coordinates of the control sample (BPE).
Transparency of BPE-based films was determined, in triplicate, using a Biomate-3 UV-VIS Spectrophotometer (Thermospectronic, Mobile, AL, USA). Film pieces, 10 mm × 30 mm, were placed on the internal side of the spectrophotometer cells and transparency was calculated following Eq. 4:
where A600 is the absorbance measured at 600 nm and e is the film thickness (mm).
3.3 Release test
Migration of active compounds from the polymer matrix was carried out by direct contact of films with ethanol 50% (v/v) as food simulant under standard test conditions according to UNE-EN 13130-1:2005 referring to materials and articles in contact with food and EU Regulation N10/2011 (European Union, 2011). The release kinetics study was performed at 40°C in an oven (J.P. Selecta, Barcelona, Spain) for 2, 8, 14, 24, 48 h and 5, 10, and 15 days, in triplicate. Samples were immersed in 20 mL of ethanol 50% (v/v) using an area-to-volume ratio of 6 dm2 L−1.
3.3.1 Quantification of tocopherol in food simulant by HPLC-UV/VIS
β+γ tocopherols were monitored after migration tests. Tocopherols concentration present in the food simulant was determined with an Agilent 1260 infinity liquid chromatograph with UV-VIS detector and BRISA LC2 C18 column (250 mm × 4.6 mm x 5 µm, Teknokroma, Barcelona, Spain). Methanol:acetonitrile 50% (v:v) was used as mobile phase at 1 mL min−1 20 μL of sample were injected and detection was performed at 292 nm (Gliszczyńska-Świgło and Sikorska, 2004). Standard solutions of the studied compounds were prepared in ethanol 50% (v/v).
3.3.2 Release kinetics
Migration of an active compound from a polymer matrix is often described by Fick’s second law in one dimension (x) using Eq. 5 (Ortega et al., 2022; Cui et al., 2023; Laroque et al., 2023):
where C is the concentration of the active compound at time t and position x, and D is the diffusion coefficient, which is considered constant during migration and depends only on temperature. Considering a flat surface and uniform initial distribution of concentration, the migrant concentration transferred from the polymer matrix to the food (or simulant) can be estimated using Eq. 6:
which is the general equation that can be simplified depending on the system, where L is the film thickness and MF,L is the migrant quantity at the polymer-food interface. When the initial concentration of the analyte in the food or simulant is 0, the analyte concentration will change from 0 to its equilibrium value all along the experiment. Eq. 7 is the most rigorous equation to explain its behavior:
where MF,t and MF,∞ are the total amount of diffusing substance released by the film at time t and after infinite time, respectively; L is the thickness; qn are the non-zero positive roots of tanqn = -αqn, and α can be calculated by using the following expression:
where VF and VP are the volumes of the simulant and polymer, respectively, and KFP is the active partition constant between the simulant and the polymer.
3.4 Antioxidant capacity
The antioxidant activity of migration extracts was analyzed using the stable radical 2,2-diphenyl-1-picrylhydrazyl (DPPH) assay, as previously reported (Byun et al., 2010). Extracts (500 μL) were mixed with 2 mL of an ethanolic solution of DPPH (0.06 mM) in a capped cuvette. The mixture was shaken vigorously at room temperature and the absorbance of the solution was measured at 517 nm with a Biomate-3 UV-VIS spectrophotometer (Thermospectronic, Mobile, AL, USA). All analyses were performed in triplicate.
ABTS (2, 2′ azino-bis (3-ethylbenzothiazoline)-6-sulfonic acid) free radical decolorization assay was performed according to Re et al., 1999 with some modifications. Samples (150 μL) were mixed with 2850 μL of the ABTS solution. The absorbance was measured at 5 min and analyses were performed in triplicate.
Antioxidant activity was calculated as percentage of inhibition (I %) following Eq. 9 in both cases:
where A0 is the absorbance of the blank sample at t = 0 min and ASample is the absorbance of the tested sample at t = 60 min.
3.5 Strawberry preservation test
Antifungal properties and food preservation effect of the studied films were evaluated by studying strawberries shelf-life under aggressive conditions in a climate chamber at 23°C ± 1°C and RH 50% ± 2% for 7 days. Fruit samples were located in the middle of two films (one at the top and one at the bottom) and placed in a Petri dish to simulate packaging conditions. The package was sealed with parafilm to avoid undesirable humidity or oxygen penetration and samples were maintained under storage conditions until mold development.
3.6 Statistical analysis
Tests were expressed as mean values and statistical analysis of results was performed with Statgraphics Centurion XVI statistical software. An analysis of variance (ANOVA) was carried out. Differences between values were analyzed using the Tukey test at a p ≤ 0.05 significance level.
4 Results and discussion
4.1 Thermal properties
The incorporation of active extracts can lead to some changes in thermal properties compared to the neat polymer. In this study, TGA and DSC tests were carried out to evaluate these modifications. The initial degradation temperatures of Tocobiol®Plus and Nutrabiol®T90 (191°C ± 1°C and 297°C ± 2°C, respectively) were higher than the processing temperature of active films (180°C), thus avoiding losses caused by extracts degradation during thermal processing. Moreover, the absence of characteristic peaks of the studied extracts in the thermograms obtained for active films (data not shown) suggested a good incorporation of the active additives to the biopolymer matrix. These results agree with others previously reported in polymer formulations with these commercial additives (López De Dicastillo et al., 2013).
Pure BPE showed a one-step degradation process, presenting a maximum degradation temperature (Tmax) of 480°C (Table 2), in agreement with previous studies (Garcia-Garcia et al., 2016; Montanes et al., 2018). No significant differences (p > 0.05) on Tmax values were observed in active films indicating that the presence of tocopherol-based extracts did not modify the thermal degradation behavior of the BPE matrix under nitrogen atmosphere. The incorporation of natural extracts caused a slight decrease (p < 0.05) of around 10°C in the initial degradation temperature compared to neat BPE, regardless of the extract’s concentration. This behavior was attributed to the plasticizing effect of the studied additives also referred by some authors to the antioxidant effects in different polymer matrices (Barczewski et al., 2023; Safakas et al., 2023; Stoyanova et al., 2023).
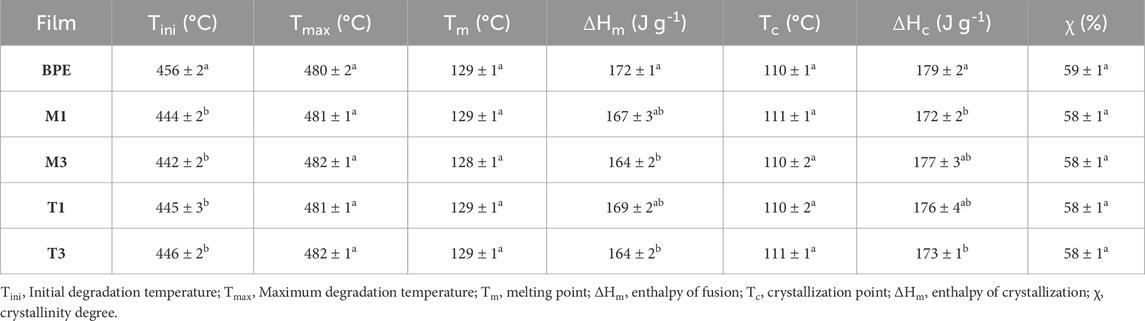
Table 2. Thermal properties of BPE-based films. Mean ± SD, n = 3. Different superscripts within the same column indicate significant differences between formulations (p < 0.05).
The addition of the studied additives did not show significant differences (p > 0.05) in melting and crystallization temperatures (Table 2). Some significant differences (p < 0.05) were found in the corresponding enthalpy values, showing active films slightly lower melting and crystallization values compared to neat BPE, although these changes were not enough to produce significant modifications (p > 0.05) in crystallinity values of BPE-based active films, being approximately 59%, in agreement with previous reported values (Mazur et al., 2020). These results are in line with those found by Lopez de Dicastillo et al. who concluded that the addition of Nutrabiol®T90 to a PP matrix did not produce significant changes in crystallinity (López De Dicastillo et al., 2013).
4.2 Oxidative stability
OOT can be defined as the minimum temperature where oxidation takes place in pure oxygen atmosphere whereas OIT represents the time to the onset of an exothermic oxidation peak under oxygen atmosphere. These parameters are commonly used as fast screening indicators of polyolefin’s stability under oxidative atmosphere (Agustin-Salazar et al., 2017; Rojas-Lema et al., 2020; Xia et al., 2020). The results obtained for OOT and OIT are summarized in Table 3. The incorporation of tocopherol-based additives into the BPE matrix produced a significant increase (p < 0.05) in oxidative stability, showing active films containing Nutrabiol®T-90 higher OOT and OIT values. In particular, the M3 film showed values of 280°C and 261 min, respectively, significantly delaying polymer oxidation compared to neat BPE. Moreover, an increase in the ability to stabilize the polymer matrix was found with increasing additives concentration from 1 wt% to 3 wt%. The oxidation-degradation of polyolefins is dominated by chain reactions of free radicals, light energy or heat which can cause polyolefin chain breaking, thus promoting the formation of free radicals which generally react with oxygen to form peroxides or peroxyl radicals (Schweighuber et al., 2021). The addition of antioxidants can prevent the propagation of these chain radicals by donating hydrogen atoms to free radicals, delaying the oxidation of polymer chains (Xia et al., 2021). The antioxidant effect of tocopherol has been previously demonstrated in the stabilization of polymer matrices in previous studies (Barbosa-Pereira et al., 2013; López De Dicastillo et al., 2013; Šlouf et al., 2019).
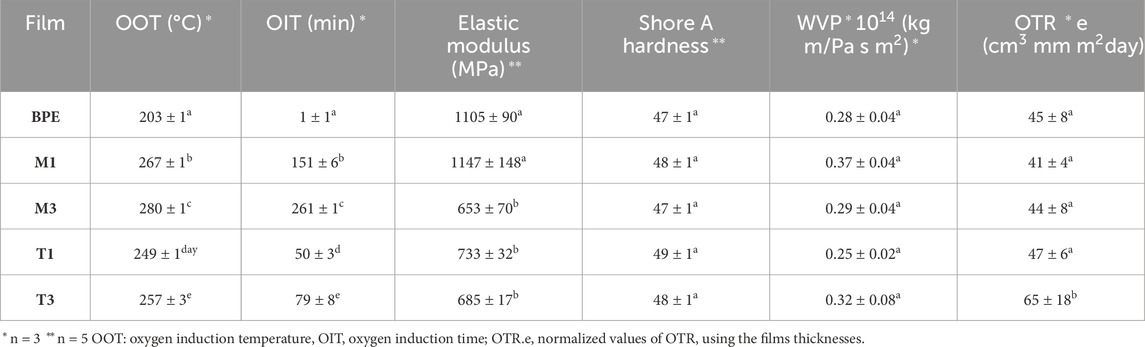
Table 3. OOT, OIT, barrier and mechanical properties of BPE-based films. Mean ± SD. Different superscripts within the same column indicate significant differences between formulations (p < 0.05).
4.3 Barrier properties
Barrier properties are key for the development of materials intended for food packaging applications. High barrier properties allow to extend the food shelf-life avoiding that humidity or other substances such as oxygen, ethylene, aroma, or unusual flavors could interact with food. Reducing the contact between these substances and food decreases the risk of adverse reactions that could reduce organoleptic properties and/or safety and quality of the final product (Ambrosio-Martín et al., 2014; Asgher et al., 2020; Elhamnia et al., 2023). Polyethylene is well known and widely used in packaging due to its quite low water vapor permeability (WVP). OTR values were normalized using film thickness values (OTR.e) to compare these results with previous literature. In general terms, the addition of tocopherol-based additives did not cause significant changes (p > 0.05) in WVP and OTR.e values (Table 3). However, T3 film showed significant differences (p < 0.05) in OTR.e values compared to the other BPE-based films, showing values of 65 ± 18 and 45 ± 8 cm3mm m2day for T3 and neat BPE, respectively. This behavior may be due to the presence of other compounds in Tocobiol®Plus, such as squalene (4 wt%) or ascorbyl palmitate (20 wt%), increasing the mobility of polymer chains and resulting in higher diffusion of oxygen through the polymer matrix. The increase in OTR.e values with some antioxidants addition was reported in previous studies as a consequence of a plasticizer effect in the polymer matrix (Luzi et al., 2019; Jiang et al., 2021; Dong et al., 2022).
4.4 Mechanical properties
The addition of 1 wt% of Nutrabiol®T90 (M1 film) did not produce significant differences (p > 0.05) in the elastic modulus (Table 3) compared to neat BPE with values of 1147 ± 148 and 1105 ± 90 MPa, respectively. However, significant differences (p < 0.05) were found for M3, T1 and T3 films compared to the control film. The addition of 3 wt% of Nutrabiol®T90 decreased elastic modulus to 653 ± 70 MPa as a consequence of an increase in the tocopherol concentration and plasticizing effect, in line with previous works (Noronha et al., 2014). In the case of Tocobiol®Plus addition, a significant decrease (p < 0.05) in the elastic modulus was observed in all formulations, which may be due to the presence of other antioxidant compounds, although no significant differences (p > 0.05) were found between T1 and T3 films. The influence of the addition of tocopherol in mechanical properties for different polymer matrices was studied by other authors and it was concluded that this additive can modify the film structure, reducing macromolecules motion in the active formulation (Agudelo-Cuartas et al., 2020; Zhang et al., 2020b; Dias et al., 2021; Jiang et al., 2021).
Hardness was also studied as a measure of the resistance of the material to plastic deformation in a localized region of the effect of a compressing object. The incorporation of both additives did not produce significant (p > 0.05) variations in Shore A hardness values (Table 3) compared to BPE, with values around 47. Hardness is directly related to crystallinity and molecular weight of the polymer (Šlouf et al., 2019; Aydoğmuş et al., 2022; Bello et al., 2023). So, the absence of changes in Shore A hardness is in accordance with crystallinity values obtained for BPE-based films, where no significant differences (p > 0.05) were found.
4.5 Color and optical properties
Visual appearance is very important for the development of food packaging materials since this property can determine their final acceptance by consumers. In this work, due to the intrinsic slight brown color of the incorporated additives, possible variations in optical properties such as color and transparency were evaluated. Table 4 shows color parameters (L*, a*, b*) and total color differences (ΔE) obtained for BPE-based films. Lightness was affected by the incorporation of both additives, causing a significant decrease (p < 0.05) in L* values in all active formulations compared to neat BPE, showing T3 film the lowest value (38.88 ± 0.54). The most significantly affected color parameter with additives addition was b*, presenting a shift to more positive (yellow) values with increasing additives concentration, showing T3 film the highest value (4.71 ± 0.92). This coloring effect can be attributed partially to the thermal treatment that increased the brown color of additives themselves, while it can be also associated to caramelization reactions of sugars remaining in the antioxidant as impurities as well as to partial polymerization of phenolic compounds (Wessling et al., 2000; Busolo and Lagaron, 2015; Agudelo-Cuartas et al., 2020). No significant changes (p > 0.05) in transparency were found in active films with the incorporation of tocopherol-based additives with values in agreement with previous reported literature for neat PE (Guzman-Puyol et al., 2022).
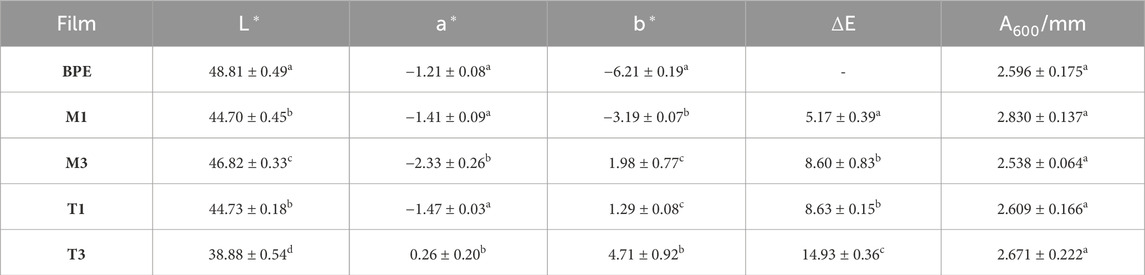
Table 4. Optical properties of BPE-based films. Mean ± SD, n = 3. Different superscripts within the same column indicate significant differences between formulations (p < 0.05).
4.6 Quantification of tocopherol in food simulant by HPLC-UV/VIS
The amount of tocopherol released in the food simulant after migration tests was analyzed by following the method proposed by Gliszczynska-Swigło et al. with some modifications (Gliszczyńska-Świgło and Sikorska, 2004). A good reproducibility was observed with RSD values ranging between 0.1%–3%. A high linearity was obtained at the studied calibration range (0.15–0.97 ppm in ethanol 50% (v/v) at five calibration points) showing a R2 value of 0.9998, with values found for the slope and intercept of 17.96 ± 0.13 and −0.463 ± 0.077 (n = 3), respectively. LOD and LOQ values were 0.014 and 0.047 ppm, respectively, and they were determined using Eqs 11, 12, where Sxy is the standard deviation of the residues and a is the slope.
Migration tests in 50% (v/v) ethanol as fatty food simulant (D1) were conducted to determine the total amount of tocopherol that could migrate from the active films into food. Experimental migration data of tocopherol-based additives from BPE active films immersed in the D1 food simulant are summarized in Figure 1. M1 and M3 films showed the highest (p < 0.05) concentration of tocopherol at any studied time, enhancing release with increasing concentration of additives, as expected. The released concentration of tocopherol for T1 and T3 films was lower, probably due to the lower amount of tocopherol present in this extract and lower affinity for the selected medium (Dumitriu et al., 2021; López De Dicastillo et al., 2013). At short exposition times (2–14 h), the concentration of tocopherol released was 0.131 ± 0.011 and 0.24 ± 0.05 mg kg−1 for T1 and T3, respectively. After 24 h, the concentration significantly increased (p < 0.05) up to 0.26 ± 0.06 and 0.48 ± 0.02 mg kg−1, respectively, but after 360 h these formulations did not arrive to the stationary state. Regarding M1 and M3, the amount of released tocopherol increased gradually with time and reached steady-state after 120 h, not showing significant differences (p > 0.05) for each film from 120 h onwards, with concentration values of 2.32 ± 0.10 and 4.69 ± 0.16 mg kg−1, respectively. The steady-state phenomenon reached in these films could be attributed to the low wettability, absorption, and penetration of water molecules into non-polar films such as BPE (Kim et al., 2019).
4.7 Release kinetics
The efficiency of using antioxidant films is normally based on the release of active components into food, being diffusion of these components from films a key factor for the development of antioxidant food packaging systems. The amount of β+γ-tocopherol released (Mt) to the food simulant was monitored as a function of time over 360 h for films containing Nutrabiol®T90 (M1, M3), which reached equilibrium state after 120 h, as it is shown in Figure 1. Considering this value, α and D parameters were calculated according to the mathematical model presented in Eq. 7 in order to study the kinetics of tocopherol release from BPE. Figure 2 shows release profiles for M1 and M3 films using experimental and modulated data. The experimental release of these two formulations started with a rapid migration followed by a slow release, fitting well the proposed model with R2 values higher than 0.95.
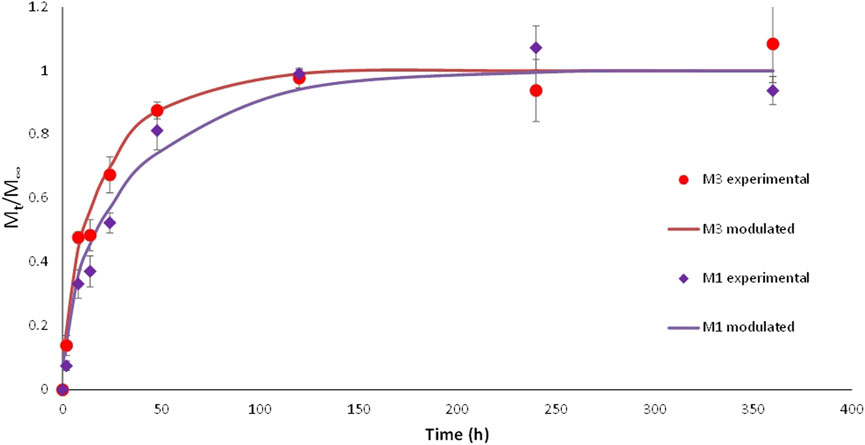
Figure 2. Tocopherol release experimental profiles and kinetics modeling from BPE-based films containing Nutrabiol®T-90 (mean ± SD, n = 3).
The α-values obtained were 1.58 ± 0.04 and 2.90 ± 0.05 for M1 and M3 films, respectively. These values indicated that Kp (active partition constant between the food simulant and the polymer) was lower than 1 in both cases, showing lower affinity of the migrant towards the polymer matrix and so faster release in the simulant medium (Dumitriu et al., 2021). These results are in line with the high diffusion coefficients (D) obtained in this study (1.12 ± 0.03 × 10−9 and 2.03 ± 0.02 × 10−9 cm2s−1 for M1 and M3, respectively), suggesting low interaction between the polymer and the migrant, resulting in a fast release of tocopherol-based additives. Therefore, active materials developed in this work could be recommended as potential alternatives to traditional packaging for foods with short shelf-life, such as fruit or meat, among others. D values obtained in this work are higher than those previously reported by other authors using tocopherol as active agent in similar polymer matrices (Table 5). Diffusion coefficients of active compounds do not only depend on the polymer matrix as they are also influenced by other factors, such as temperature and type of food simulant. High temperatures usually result in an increase in the diffusion coefficient, so it is expected that the use of active materials under refrigerated conditions would produce a delayed release of active compounds (Assis et al., 2021). Furthermore, if the affinity for the food is decreased, considering that tocopherol has low affinity for aqueous foods, this release would be also delayed (López de Dicastillo et al., 2013).
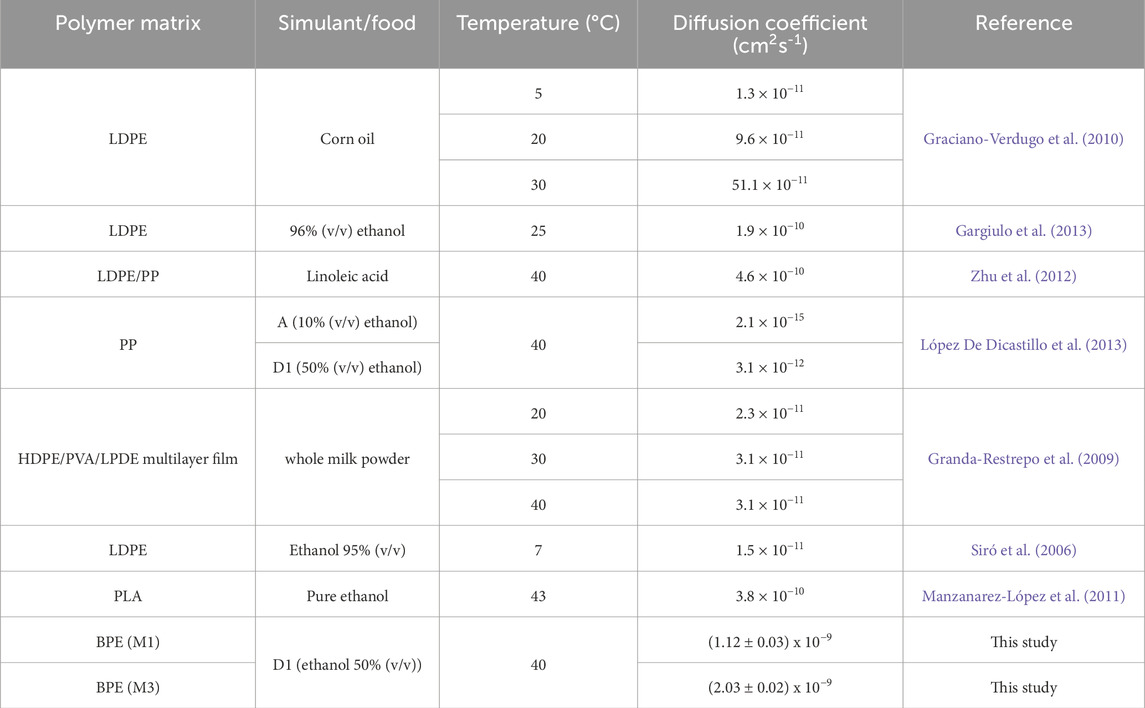
Table 5. Diffusion coefficient of tocopherol in different polymer matrices and conditions from literature.
4.8 Antioxidant capacity
Antioxidant packaging is a very promising approach for extending shelf-life of food products. In this work, antioxidant activity in migration extracts was studied by the DPPH and ABTS assays in order to evaluate the potential use of the developed BPE-films as antioxidant systems to preserve fatty foods. The antioxidant capacity of natural tocopherol-based extracts was previously studied by Barbosa-Pereira et al., reporting higher antioxidant activity compared to butylated hydroxytoluene (BHT), a synthetic antioxidant commonly used by the food industry (Barbosa-Pereira et al., 2013).
BPE control films did not show any antioxidant activity while films with tocopherol-based additives showed high antioxidant activity in both tested methods. These results confirmed the antioxidant stability after film processing as well as in vitro antioxidant capacity in active films (Figure 3). A similar trend was observed in DPPH and ABTS assays, showing M3 film a significantly higher antioxidant capacity followed by M1 > T3 > T1 (p < 0.05). These results are in line with tocopherol concentrations released and results found by other authors reporting an increase in antioxidant activity with increasing the amount of antioxidant added to the film, although it did not always follow a linear trend. The antioxidant activity depends not only on the structure of the active compound, but also on many other factors, such as light, physical state of the system, concentration, temperature and type of simulant (Agudelo-Cuartas et al., 2020; Petchsomrit et al., 2020; Dias et al., 2021; Jiang et al., 2021). The results obtained in this work highlighted the great potential of BPE-based active films to be used in food packaging; in particular the M3 film.
4.9 Fruit preservation test
The antifungal capacity of films for food preservation was evaluated on strawberries as a highly perishable food model. Tests were performed using experimental conditions indicated in Section 3.4. Fruits and vegetables are characterized by a short shelf-life due to the rapid proliferation of yeasts and molds which can be associated to their low pH, high water activity and high respiration rate. Molds contaminate the plant and proliferate during flowering, contaminating the future fruit with spores, which remain dormant awaiting optimal conditions for their development (Finnegan and O’Beirne, 2015). Strawberry is known for its high levels of bioactive compounds, such as polyphenols, minerals and vitamins. However, strawberry is prone to spoilage caused by fungi and/or pathogens which can lead to losses in quality and economic value during storage. Under normal conditions, the shelf-life of strawberries at room temperature is approximately 1–2 days (Fu et al., 2024). Figure 4 shows the effect of BPE-based active films on strawberries deterioration. All active formulations were able to prolong the shelf-life of strawberries compared to neat BPE retarding the growth of molds and yeasts.
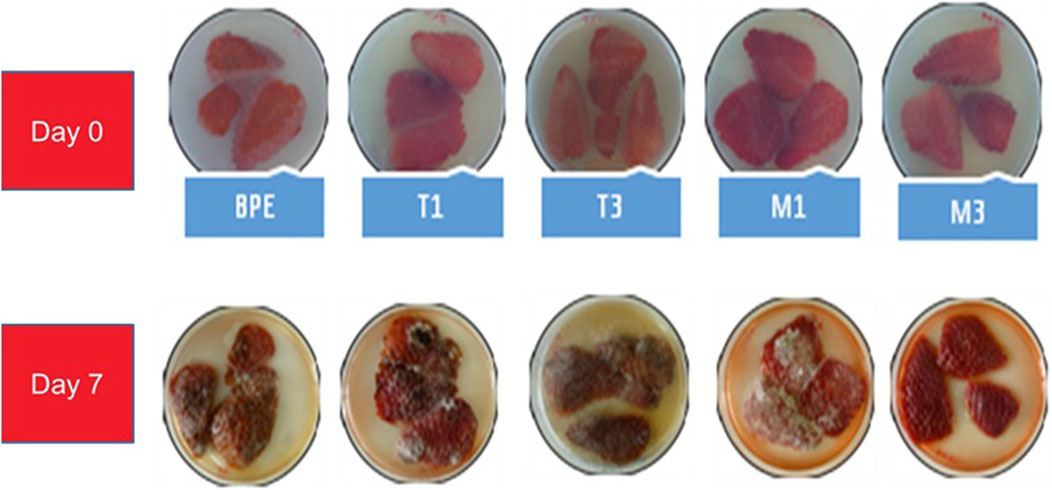
Figure 4. Antifungal properties and preservation effects of BPE-based films on strawberries after 7 days.
The main disorders found on strawberries are grey mold disease and black mold of bread, caused by Botrytis cinerea and Rhizopus stolonifer, respectively (Singh et al., 2023; Srivastava et al., 2023; Fu et al., 2024). Films containing Tocobiol®Plus extract (T1, T3) were able to retard fungal growth for 3 days followed by the M1 film, showing the M3 film the best effect. These results agree with those obtained in migration tests, where films added with Nutrabiol®T90 extract showed faster tocopherol release compared to films incorporated with Tocobiol®Plus extract, taking 5 days to reach stability. This factor is important in food protection at short periods of time before the appearance of microorganism’s growth.
Fungal growth is enhanced at poor refrigerated conditions. After 6 days of storage at 25°C, all packed fruits, except for M3 film, showed advanced mold growth, covering most of the strawberry surface and showing areas with high spore densities. On day 7, ripe strawberries were observed when being packed with the M3 film, showing exuded juice without enzymatic browning. The first changes observed in fruit deterioration affected texture and appearance, being caused by membrane degradation with the release of nutrients and enzymes. Within a few days, the higher permeability of the cell wall results in increasing the exuded juice (Finnegan and O’Beirne, 2015). Polyphenol oxidase enzyme causes browning of anthocyanins, tannins and lignin which are polyphenol-based polymers providing rigidity to the plant (Vinagre-Marques and Alifdalino, 2022). The brown color of strawberries found in packed formulations, except for M3, was attributed to the presence of quinones, a product of enzymatic browning of polyphenols. These conditions favor the proliferation of microorganisms, especially molds, yeasts and lactic acid bacteria in the case of strawberries (Teribia et al., 2021).
The incorporation of 3 wt% Nutrabiol®T90 showed great potential for the development of new packaging materials for food preservation. The extension of strawberries shelf-life was attributed to the antioxidant capacity of the tocopherol-based additive, protecting fruit against early deterioration, preventing phenolic compound oxidation and delaying the germination of spores. The incorporation of α-tocopherol into PLA-based films to increase the shelf-life of peach was previously reported, showing less oxidative spoilage during storage (Jiang et al., 2021). The effectiveness of chitosan/zein active films containing α-tocopherol on mushrooms storage was also demonstrated showing mushrooms high firmness and total phenolic content by using the active formulations (Zhang et al., 2020a).
5 Conclusion
Tocopherol-based natural extracts, such as Nutrabiol®T90 and Tocobiol®Plus, have demonstrated their potential to be used as active additives in BPE-based films for food packaging applications, substituting common synthetic and potentially toxic antioxidants and petrol-based polyolefins. The addition of the active extracts did not cause important changes in thermal, mechanical and barrier properties, but significantly increased OOT and OIT values, stabilizing the polymer matrix against thermo-oxidative degradation. These additives were quickly released from the polymer matrix, playing their active role, showing films containing 3 wt% of Nutrabiol®T90 (M3) the highest tocopherol release in 50% (v/v) ethanol and consequently higher diffusion coefficient and antioxidant capacity. Moreover, this formulation successfully delayed fungal growth in packed strawberries for 7 days. Therefore, the obtained BPE-based film incorporating Nutrabiol®T90 at 3 wt% showed improved functional properties and great potential to enhance food quality and safety for food packaging applications. The obtained formulation can be easily scaled-up to obtain a potential interesting commercial material for active packaging applications within the food manufacturing and agricultural sectors. Further work is currently on-going to evaluate organoleptic behavior to ensure industrial transfer for food packaging applications.
Data availability statement
The original contributions presented in the study are included in the article/Supplementary Material, further inquiries can be directed to the corresponding author.
Author contributions
AM: Formal Analysis, Investigation, Visualization, Writing–original draft. EG-S: Formal Analysis, Investigation, Writing–original draft. AJ: Funding acquisition, Supervision, Validation, Writing–review and editing. MG: Funding acquisition, Supervision, Validation, Writing–review and editing.
Funding
The author(s) declare that financial support was received for the research, authorship, and/or publication of this article. Authors acknowledge the financial support from the Spanish Ministry of Science and Innovation (PID 2020-116496RB-C21).
Conflict of interest
The authors declare that the research was conducted in the absence of any commercial or financial relationships that could be construed as a potential conflict of interest.
The author(s) declared that they were an editorial board member of Frontiers, at the time of submission. This had no impact on the peer review process and the final decision.
Publisher’s note
All claims expressed in this article are solely those of the authors and do not necessarily represent those of their affiliated organizations, or those of the publisher, the editors and the reviewers. Any product that may be evaluated in this article, or claim that may be made by its manufacturer, is not guaranteed or endorsed by the publisher.
References
Agudelo-Cuartas, C., Granda-Restrepo, D., Sobral, P. J. A., Hernandez, H., and Castro, W. (2020). Characterization of whey protein-based films incorporated with natamycin and nanoemulsion of α-tocopherol. Heliyon 6 (4), e03809. doi:10.1016/j.heliyon.2020.e03809
Agustin-Salazar, S., Gamez-Meza, N., Medina-Juárez, L. A., Malinconico, M., and Cerruti, P. (2017). Stabilization of polylactic acid and polyethylene with nutshell extract: efficiency assessment and economic evaluation. ACS Sustain. Chem. Eng. 5 (6), 4607–4618. doi:10.1021/acssuschemeng.6b03124
Ambrosio-Martín, J., Fabra, M. J., Lopez-Rubio, A., and Lagaron, J. M. (2014). An effect of lactic acid oligomers on the barrier properties of polylactide. J. Mater. Sci. 49 (8), 2975–2986. doi:10.1007/s10853-013-7929-x
Asgher, M., Sarmad, A. Q., Bilal, M., and Iqbal, H. M. N. (2020). Bio-based active food packaging materials: sustainable alternative to conventional petrochemical-based packaging materials. Food Res. Int. 137, 109625. doi:10.1016/j.foodres.2020.109625
Assis, R. Q., Pagno, C. H., Stoll, L., Rios, P. D., Rios, A., and Olivera, F. C. (2021). Active food packaging of cellulose acetate: storage stability, protective effect on oxidation of riboflavin and release in food simulants. Food Chem. 349, 129140. doi:10.1016/j.foodchem.2021.129140
Aydoğmuş, E., Dağ, M., Yalçın, Z. G., and Arslanoğlu, H. (2022). Synthesis and characterization of waste polyethylene reinforced modified castor oil-based polyester biocomposite. J. Appl. Polym. Sci. 139 (27). doi:10.1002/app.52526
Bajwa, D. S., Adhikari, S., Shojaeiarani, J., Bajwa, S. G., Pandey, P., and Shanmugam, S. R. (2019). Characterization of bio-carbon and ligno-cellulosic fiber reinforced bio-composites with compatibilizer. Constr. Build. Mater. 204 (April), 193–202. doi:10.1016/j.conbuildmat.2019.01.068
Barbosa-Pereira, L., Cruz, J. M., Sendón, R., Rodríguez Bernaldo de Quirós, A., Ares, A., Castro-López, M., et al. (2013). Development of antioxidant active films containing tocopherols to extend the shelf life of fish. Food control. 31 (1), 236–243. doi:10.1016/j.foodcont.2012.09.036
Barczewski, M., Aniśko, J., Hejna, A., Mysiukiewicz, O., Kosmela, P., Sałasińska, K., et al. (2023). Ground lemon and stevia leaves as renewable functional fillers with antioxidant activity for high-density polyethylene composites. Clean Technol. Environ. Policy 25 (10), 3345–3361. doi:10.1007/s10098-023-02565-5
Barros, C., Miranda, S., Castro, O., Carneiro, O. S., and Machado, A. V. (2023). LDPE-nanoclay films for food packaging with improved barrier properties. J. Plastic Film Sheeting 39 (3), 304–320. doi:10.1177/87560879221151190
Bazan, P., Mierzwiński, D., Bogucki, R., and Kuciel, S. (2020). Bio-based polyethylene composites with natural fiber: mechanical, thermal, and ageing properties. Materials 13 (11), 2595. doi:10.3390/ma13112595
Bello, S. A., Kolawole, N., Yinka, M., Kayode, M., Adekunle, J., Adekunle, K., et al. (2023). Eggshell nanoparticle reinforced recycled low-density polyethylene: a new material for automobile application. J. King Saud Univ. - Eng. Sci. 35 (6), 406–414. doi:10.1016/j.jksues.2021.04.008
Busolo, M. A., and Lagaron, J. M. (2015). Antioxidant polyethylene films based on a resveratrol containing clay of interest in food packaging applications. Food Packag. Shelf Life 6, 30–41. doi:10.1016/j.fpsl.2015.08.004
Byun, Y., Teck, Y., and Whiteside, S. (2010). Characterization of an antioxidant polylactic acid (PLA) film prepared with α-tocopherol, BHT and polyethylene glycol using film cast extruder. J. Food Eng. 100 (2), 239–244. doi:10.1016/j.jfoodeng.2010.04.005
Castro López, M., Dopico, S., Ares, A., López, J. M., and González, M. V. (2012). Effect of PPG-PEG-PPG on the tocopherol-controlled release from films intended for food-packaging applications. J. Agric. Food Chem. 60 (33), 8163–8170. doi:10.1021/jf301442p
Chang, S., Mohammadi, A., and Baghaei, H. (2022). Characterization and application of active polyethylene films incorporated with thymol and linalool on mozzarella cheese preservation. Mdrsjrns 19 (123), 369–380. doi:10.52547/fsct.19.123.369
Cui, Y., Zhang, R., Wang, L., Cheng, M., Guo, Y., and Wang, X. (2023). Quantitative study on release kinetics of thymol in food packaging films. J. Food Eng. 340, 111307. doi:10.1016/j.jfoodeng.2022.111307
Delgado, A., Al-Hamimi, S., Fawzy, M., De Wit, M., Durazzo, A., Lin, K., et al. (2020). Contribution of tocols to food sensorial properties, stability, and overall quality. J. Food Qual. 2020, 8885865. doi:10.1155/2020/8885865
Dias, M. V., Machado, V., Fonseca, L., Salgado, A. C., Vilela, S., Ferreira, N. F., et al. (2021). Chitosan-nanocomposites as a food active packaging: effect of addition of tocopherol and modified montmorillonite. J. Food Process Eng. 44 (11). doi:10.1111/jfpe.13843
Dong, Q., Zhang, Y., Yang, L., Bian, J., and Li, Li (2022). Development of gelatin film deposited with carvacrol-loaded fibers and its application to sea bass fillet preservation. J. Food Process. Preserv. 46 (5), e16570. doi:10.1111/JFPP.16570
Dumitriu, R. P., StoleruMitchell, E. R. G. R., Vasile, C., and Brebu, M. (2021). Bioactive electrospun fibers of poly(ε-caprolactone) incorporating α-tocopherol for food packaging applications. Molecules 26 (18), 5498. doi:10.3390/molecules26185498
EFSA panel on food additives and nutrient sources added to food (ANS) (2011). Scientific opinion on the re-evaluation of butylated hydroxyanisole – bha (e 320) as a food additive. EFSA J. 9 (10), 2392. doi:10.2903/j.efsa.2011.2392
EFSA panel on food additives and nutrient sources added to food (ANS) (2012). Scientific opinion on the re-evaluation of butylated hydroxytoluene bht (e 321) as a food additive. EFSA J. 10 (3), 2588. doi:10.2903/j.efsa.2012.2588
Elhamnia, M., Hashemi, G., and Hassan, S. (2023). Improved barrier properties of biodegradable pbat films for packaging applications using EVOH: morphology, permeability, biodegradation, and mechanical properties. J. Appl. Polym. Sci. 16, 53855. doi:10.1002/app.53855
European_Union (2011). Commission regulation (EU) No 10/2011 of 14 january 2011 on plastic materials and articles intended to come into contact with food. Official J. Eur. Union 12.
Finnegan, E., and O’Beirne, D. (2015). Characterising and tracking deterioration patterns of fresh-cut fruit using principal component analysis - Part I. Postharvest Biol. Technol. 100, 73–80. doi:10.1016/j.postharvbio.2014.09.008
Findrik, E., and Meixner, O. (2023). Drivers and barriers for consumers purchasing bioplastics-a systematic literature review. Journal of Cleaner Production 410. doi:10.1016/j.jclepro.2023.137311
Fu, X., Chang, X., Xu, S., Xu, H., Ge, S., Xie, Y., et al. (2024). Development of a chitosan/pectin-based multi-active food packaging with both uv and microbial defense functions for effectively preserving of strawberry. Int. J. Biol. Macromol. 254 (P2), 127968. doi:10.1016/j.ijbiomac.2023.127968
Garcia-Garcia, D., Carbonell-Verdu, A., Jordá-Vilaplana, A., Balart, R., and Garcia-Sanoguera, D. (2016). Development and characterization of green composites from bio-based polyethylene and peanut shell. J. Appl. Polym. Sci. 133 (37), 12–17. doi:10.1002/app.43940
Gargiulo, N., Ilaria Attianese, I., Buonocore, G., Caputo, D., Lavorgna, M., Mensitieri, G., et al. (2013). α-Tocopherol release from active polymer films loaded with functionalized sba-15 mesoporous silica. Microporous Mesoporous Mater. 167, 10–15. doi:10.1016/j.micromeso.2012.07.037
Gengatharan, A., and Hafiz, M. (2023). The application of clove extracts as a potential functional component in active food packaging materials and model food systems: a mini-review. Appl. Food Res. 3 (1), 100283. doi:10.1016/j.afres.2023.100283
Glaser, T. K., Plohl, O., Vesel, A., Ajdnik, U., Poklar, N., Hrnčič, M. K., et al. (2019). Functionalization of polyethylene (PE) and polypropylene (PP) material using chitosan nanoparticles with incorporated resveratrol as potential active packaging. Materials 12 (13), 2118. doi:10.3390/ma12132118
Gliszczyńska-Świgło, A., and Sikorska, E. (2004). Simple reversed-phase liquid chromatography method for determination of tocopherols in edible plant oils. J. Chromatogr. A 1048 (2), 195–198. doi:10.1016/j.chroma.2004.07.051
Graciano-Verdugo, A. Z., Soto-Valdez, H., Peralta, E., Cruz-Zárate, P., Islas-Rubio, A. R., Sánchez-Valdes, S., et al. (2010). Migration of α-tocopherol from LDPE films to corn oil and its effect on the oxidative stability. Food Res. Int. 43 (4), 1073–1078. doi:10.1016/j.foodres.2010.01.019
Granda-Restrepo, D. M., Soto-Valdez, H., Peralta, E., Troncoso-Rojas, R., Vallejo-Córdoba, B., Gámez-Meza, N., et al. (2009). Migration of α-tocopherol from an active multilayer film into whole milk powder. Food Res. Int. 42 (10), 1396–1402. doi:10.1016/j.foodres.2009.07.007
Guzman-Puyol, S., Benítez, J. J., and Heredia-Guerrero, J. A. (2022). Transparency of polymeric food packaging materials. Food Res. Int. 161, 111792. doi:10.1016/j.foodres.2022.111792
Jiang, J., Dong, Q., Gao, H., Han, Y., and Li, L. (2021). Enhanced mechanical and antioxidant properties of biodegradable poly (lactic) acid-poly(3-hydroxybutyrate-co-4-hydroxybutyrate) film utilizing α-tocopherol for peach storage. Packag. Technol. Sci. 34 (3), 187–199. doi:10.1002/pts.2553
Kim, M. H., Kim, T. H., Ko, J. A., Ko, S., Min, J., and Jin, H. (2019). Kinetic and thermodynamic studies of silver migration from nanocomposites. J. Food Eng. 243, 1–8. doi:10.1016/j.jfoodeng.2018.08.028
Laroque, D. A., Jong, N., Müller, L., Paganini, C. C., Araújo, P. H. H., Aragão, G. M. F., et al. (2023). Carvacrol release kinetics from cellulose acetate films and its antibacterial effect on the shelf life of cooked ham. J. Food Eng. 358, 111681. doi:10.1016/j.jfoodeng.2023.111681
López De Dicastillo, C., Ares, A., Castro-López, M. M., López, J. M., and González, M. V. (2013). Enhancing the release of the antioxidant tocopherol from polypropylene films by incorporating the natural plasticizers lecithin, olive oil, or sunflower oil. J. Agric. Food Chem. 61 (48), 11848–11857. doi:10.1021/jf404283q
Lu, L., Cheng, C., Li Xu, L., Pan, L., Feng, H., and Lu, L. (2022). Migration of antioxidants from food-contact rubber materials to food simulants. J. Food Eng. 318, 110904. doi:10.1016/j.jfoodeng.2021.110904
Luzi, F., Dominici, F., Armentano, I., Fortunati, E., Burgos, N., Fiori, S., et al. (2019). Combined effect of cellulose nanocrystals, carvacrol and oligomeric lactic acid in PLA_PHB polymeric films. Carbohydr. Polym. 223, 115131. doi:10.1016/j.carbpol.2019.115131
Manzanarez-López, F., Soto-Valdez, H., Auras, R., and Peralta, E. (2011). Release of α-tocopherol from poly(lactic acid) films, and its effect on the oxidative stability of soybean oil. J. Food Eng. 104 (4), 508–517. doi:10.1016/j.jfoodeng.2010.12.029
Manzoor, A., Yousuf, B., Pandith, J. A., and Ahmad, S. (2023). Plant-derived active substances incorporated as antioxidant, antibacterial or antifungal components in coatings/films for food packaging applications. Food Biosci. 53, 102717. doi:10.1016/j.fbio.2023.102717
Mazur, K., Jakubowska, P., Romańska, P., and Kuciel, S. (2020). Green high density polyethylene (HDPE) reinforced with basalt fiber and agricultural fillers for technical applications. Compos. Part B Eng. 202, 108399. doi:10.1016/j.compositesb.2020.108399
Mellinas, C., Ramos, M., Grau-Atienza, A., Jordà, A., Burgos, N., Jiménez, A., et al. (2020). Biodegradable Poly(ε-Caprolactone) active films loaded with MSU-X mesoporous silica for the release of α-tocopherol. Polymers 12 (1), 137. doi:10.3390/polym12010137
Mendieta, C. M., Vallejos, M. E., Felissia, F. E., Chinga-Carrasco, G., and Area, M. C. (2020). Review: bio-polyethylene from wood wastes. J. Polym. Environ. 28 (1), 1–16. doi:10.1007/s10924-019-01582-0
Montanes, N., Garcia-Sanoguera, D., Segui, V. J., Fenollar, O., and Boronat, T. (2018). Processing and characterization of environmentally friendly composites from biobased polyethylene and natural fillers from thyme herbs. J. Polym. Environ. 26 (3), 1218–1230. doi:10.1007/S10924-017-1025-2
Noronha, C. M., Matos, S., Calegari, R., and Manique, P. L. (2014). Characterization of antioxidant methylcellulose film incorporated with α-tocopherol nanocapsules. Food Chem. 159, 529–535. doi:10.1016/j.foodchem.2014.02.159
Ortega, F., Sobral, P., Jios, J. L., Arce, V. B., and García, M. A. (2022). Starch nanocomposite films: migration studies of nanoparticles to food simulants and bio-disintegration in soil. Polymers 14 (9), 1636. doi:10.3390/polym14091636
Othman, S. H., Kahar, N. S., Nordin, N., and Shapi’i, R. A. (2023). Properties and food packaging applications of solvent casting-made starch-based films incorporated with essential oils: a review. Starch - Stärke 75 (5–6), 2200152. doi:10.1002/star.202200152
Petchsomrit, A., McDermott, M. I., Chanroj, S., and Choksawangkarn, W. (2020). Watermelon seeds and peels: fatty acid composition and cosmeceutical potential. OCL 27, 54. doi:10.1051/ocl/2020051
Re, R., Pellegrini, N., Proteggente, A., Pannala, A., Yang, M., and Rice-Evans, C. (1999). Antioxidant activity applying an improved abts radical cation decolorization assay. Free Radic. Biol. Med. 26 (9–10), 1231–1237. doi:10.1016/S0891-5849(98)00315-3
Rojas-Lema, S., Torres-Giner, S., Quiles-Carrillo, L., Gomez-Caturla, J., Garcia-Garcia, D., and Balart, R. (2020). On the use of phenolic compounds present in citrus fruits and grapes as natural antioxidants for thermo-compressed bio-based high-density polyethylene films. Antioxidants 10 (1), 14. doi:10.3390/antiox10010014
Roy, S., Kim, H. C., Panicker, P. S., Rhim, J. :W., and Kim, J. (2021). Cellulose nanofiber-based nanocomposite films reinforced with zinc oxide nanorods and grapefruit seed extract. Nanomaterials 11 (4), 877. doi:10.3390/nano11040877
Sabaghi, M., Tavasoli, S., Jamali, S. N., Katouzian, I., and Faridi Esfanjani, A. (2022). The pros and cons of incorporating bioactive compounds within food networks and food contact materials: a Review. Food Bioprocess Technol. 15, 2422–2455. doi:10.1007/s11947-022-02837-w
Safakas, K., Giotopoulou, I., Giannakopoulou, A., Katerinopoulou, K., Lainioti, G. C., Stamatis, H., et al. (2023). Designing antioxidant and antimicrobial polyethylene films with bioactive compounds/clay nanohybrids for potential packaging applications. Molecules 28 (7), 2945. doi:10.3390/molecules28072945
Schweighuber, A., Felgel-Farnholz, A., Bögl, T., Fischer, J., and Buchberger, W. (2021). Investigations on the influence of multiple extrusion on the degradation of polyolefins. Polym. Degrad. Stab. 19, 109689. doi:10.1016/j.polymdegradstab.2021.109689
Severo, C., Anjos, I., Souza, V. G. L., Canejo, J. P., Bronze, M. R., Fernando, A. L., et al. (2021). Development of cranberry extract films for the enhancement of food packaging antimicrobial properties. Food Packag. Shelf Life 28, 100646. doi:10.1016/j.fpsl.2021.100646
Singh, P., Kaur, G., Singh, A., and Dar, B. N. (2023). Effect of montmorillonite and starch nanocrystals based biodegradable films loaded with lemongrass oil nanoemulsion on quality, enzymatic activity and shelf life of strawberry. Food Chem. Adv. 3, 100343. doi:10.1016/j.focha.2023.100343
Siracusa, V., and Blanco, I. (2020). Bio-polyethylene (bio-PE), bio-polypropylene (bio-PP) and bio-poly(ethylene terephthalate) (bio-PET): recent developments in bio-based polymers analogous to petroleum-derived ones for packaging and engineering applications. Polymers 12 (8), 1641. doi:10.3390/polym12081641
Siró, I., Fenyvesi, E., Szente, L., Meulenaer, B., Devlieghere, F., Orgoványi, J., et al. (2006). Release of alpha-tocopherol from antioxidative low-density polyethylene film into fatty food simulant: influence of complexation in beta-cyclodextrin. Food Addit. Contam. 23 (8), 845–853. doi:10.1080/02652030600699064
Šlouf, M., Michálková, D., Gajdošová, V., Dybal, J., and Pilař, J. (2019). Prooxidant activity of phenolic stabilizers in polyolefins during accelerated photooxidation. Polym. Degrad. Stab. 166, 307–324. doi:10.1016/j.polymdegradstab.2019.06.013
Srivastava, V., Singh, S., and Das, D. (2023). Rice husk fiber-reinforced starch antimicrobial biocomposite film for active food packaging. J. Clean. Prod. 421, 138525. doi:10.1016/j.jclepro.2023.138525
Stoyanova, N., Spasova, M., Manolova, N., Rashkov, I., Kamenova-Nacheva, M., Staleva, P., et al. (2023). Electrospun PLA-based biomaterials loaded with melissa officinalis extract with strong antioxidant activity. Polymers 15 (5), 1070. doi:10.3390/polym15051070
UN (2023). Take Action for the Sustainable Development Goals. U. N. Sustain. Dev. Available at: https://www.un.org/sustainabledevelopment/sustainable-development-goals/(Accessed January 1, 2024).
Teribia, N., Buvé, C., Bonerz, D., Aschoff, J., Hendrickx, M., and Van Loey, A. (2021). Impact of processing and storage conditions on color stability of strawberry puree: the role of PPO reactions revisited. J. Food Eng. 294 (July 2020), 110402. doi:10.1016/j.jfoodeng.2020.110402
Vinagre-Marques, S., and Alifdalino, S. (2022). Control of enzymatic browning in strawberry, apple, and pear by physical food preservation methods: comparing ultrasound and high-pressure inactivation of polyphenoloxidase. Foods 11 (13), 1942. doi:10.3390/foods11131942
Wessling, C., Nielsen, T., and Leufve, A. (2000). The influence of alpha-tocopherol concentration on the stability of linoleic acid and the properties of low-density polyethylene. Packag. Technol. Sci. 13 (1), 19–28. doi:10.1002/(sici)1099-1522(200001/02)13:1<19::aid-pts492>3.0.co;2-b
Xia, H., Gao, H., Zhang, Y., Wang, Z., Song, L., Liu, L., et al. (2021). Natural antioxidant from bamboo leaves for the processing stability of polypropylene. J. Therm. Analysis Calorim. 146 (4), 1657–1665. doi:10.1007/s10973-020-10115-0
Xia, H., Sui, K., Ge, T., Wu, F., Sun, Q., Wang, Z., et al. (2020). Natural compounds from Punica granatum peel as multiple stabilizers for polyethylene. Polym. Eng. Sci. 60 (11), 2761–2769. doi:10.1002/pen.25506
Zhang, L., Liu, Z., Sun, Y., Wang, X., and Li, L. (2020a). Combined antioxidant and sensory effects of active chitosan/zein film containing α-tocopherol on agaricus bisporus. Food Packag. Shelf Life 24, 100470. doi:10.1016/j.fpsl.2020.100470
Zhang, L., Liu, Z., Sun, Y., Wang, X., and Li, L. (2020b). Effect of α-tocopherol antioxidant on rheological and physicochemical properties of chitosan/zein edible films. Lwt 118, 108799. doi:10.1016/j.lwt.2019.108799
Zhu, X., Lee, D., and Yam, K. L. (2012). Release property and antioxidant effectiveness of tocopherol-incorporated LDPE/PP blend films. Food Addit. Contam. Part A, Chem. Analysis, Control, Expo. Risk Assess. 29 (3), 461–468. doi:10.1080/19440049.2011.643826
Zia, J., Paul, U. C., Heredia-Guerrero, J. A., Athanassiou, A., and Fragouli, D. (2019). Low-density polyethylene/curcumin melt extruded composites with enhanced water vapor barrier and antioxidant properties for active food packaging. Polymer 175 (January), 137–145. doi:10.1016/j.polymer.2019.05.012
Keywords: bio-polyethylene, tocopherols, release kinetics, antioxidant, antifungal, active food packaging
Citation: Mellinas AC, García-Serna E, Jiménez A and Garrigós MC (2024) Active films based on bio-polyethylene and natural extracts rich in tocopherols for food packaging applications. Front. Mater. 11:1365222. doi: 10.3389/fmats.2024.1365222
Received: 03 January 2024; Accepted: 17 June 2024;
Published: 10 July 2024.
Edited by:
Ilaria Cacciotti, University Niccolò Cusano, ItalyReviewed by:
Shanshan Shi, University of South Carolina, United StatesNaima Belhaneche-Bensemra, Polytechnic School of Algiers, Algeria
Farhad Garavand, Teagasc Food Research Centre, Ireland
Copyright © 2024 Mellinas, García-Serna, Jiménez and Garrigós. This is an open-access article distributed under the terms of the Creative Commons Attribution License (CC BY). The use, distribution or reproduction in other forums is permitted, provided the original author(s) and the copyright owner(s) are credited and that the original publication in this journal is cited, in accordance with accepted academic practice. No use, distribution or reproduction is permitted which does not comply with these terms.
*Correspondence: M. C. Garrigós, bWMuZ2Fycmlnb3NAdWEuZXM=