- 1School of Environmental and Chemical Engineering, Dalian University, Dalian, Liaoning, China
- 2School of Chemical Engineering, University of Science and Technology Liaoning, Anshan, Liaoning, China
- 3Dalian Customs, Dalian, Liaoning, China
- 4Department of Chemistry, Tsinghua University, Beijing, China
The reversible phase transition of vanadium dioxide under thermal, electrical, and optical stimuli is the enabling concept for the functioning of smart materials and is the basis for the development of various device materials such as optical, electrical, thermal, and mechanical devices based on VO2 on rigid and flexible platforms. The phase transition temperature of VO2 near room temperature is considered an excellent choice and a potential candidate to replace traditional materials in a variety of applications. There is a growing interest in VO2 applications for a wide range of devices, and the use of VO2’s structure to manipulate and explore the functions of various application devices, as well as the modification of VO2 structures to improve performance in a variety of materials, can lead to extremely exciting innovations. A lot of effort has been put into the challenges of practical production and practical application, and it is necessary to find an industrially feasible manufacturing method for the preparation of VO2 films, which is the basis for the practical application of VO2-based equipment. Based on this background, we first briefly describe the structure of VO2, the phase transition mechanisms involved, and the factors and other properties induced by the phase transition of VO2. Then, the current status and advantages and disadvantages of VO2 thin film preparation technologies are introduced in detail, including pulsed laser deposition (PLD), magnetron sputtering, the sol-gel method, and chemical vapour deposition (CVD). In addition, we propose three strategies to improve the performance of VO2 thin films, including element doping, multi-layer composites, and surface structure. We also discussed the different applications of VO2 under thermal, electrical, and light stimulation, as well as the development trends and future challenges of VO2 thin films.
1 Introduction
In 1959, Morin discovered that vanadium dioxide (VO2) in the Bell laboratory has unique and reversible metal-insulator phase transformation characteristics (WITH) (Morin, 1959), and VO2 undergoes a structural phase transition (SPT), that is, from a low-temperature monoclinic rutile structure to a high-temperature tetragonal rutile structure. With the transformation of the crystal structure, VO2’s optical and electrical properties will be mutated, so in the following decades, a large number of researchers have been interested in VO2. The film was thoroughly studied (as shown in Figure 1).
Although there are quite a few other transition metal oxides (Adler, 1968), such as Mo9O26, Fe3O4, VnO2n−1, Ti2O3. They also have this MIT phase transition property. However, in comparison, the phase transition temperature of VO2 is closest to room temperature. The phase transition temperature can be further adjusted by doping elements such as W, F or Mo. This unique property near room temperature makes VO2 a photoelectric switching material (Lysenko et al., 2006), chromic coating (Manning et al., 2004), infrared imaging, and many other fields have broad application prospects.
Vanadium has a unique 3D orbital hybridization in its chemical bonds, which makes it exhibit multivalence properties, so vanadium has a wide range of oxide types, including VO, V2O3, VO2, and V2O5 and 12 others. In these 12 vanadium oxides, VO2 and V2O5 have the chemical stability required for microelectronic devices, so the characteristic, mechanism, and application research of MIT mainly focuses on VO2 and V2O5, two vanadium oxides. Among them, the phase transition temperature of VO2 is 68°C, which is closer to room temperature and has greater application potential in thermal control, so the research on VO2 is more extensive. Since the V ion in VO2 is not the highest valence state, it is difficult to guarantee that a high-purity VO2 film can be prepared. Therefore, how to prepare high-purity and high-quality VO2 films is a key problem that limits their application. The quality of VO2 films is directly related to the excellent performance of the device, which requires researchers to select appropriate preparation technology, optimize the process according to the application requirements of the device, and finally obtain high-quality VO2 films. Therefore, the study of the preparation technology of VO2 films is a foundational step for subsequent application development, and it is of great research significance. At present, the preparation process of VO2 film mainly includes magnetron sputtering (Luo and Huang, 2010), the sol-gel method (Chae et al., 2006; Li et al., 2015), chemical vapor deposition (Vernardou et al., 2006), the pulsed laser deposition method (Cole et al., 1997; Bukhari et al., 2020) and others. The conditions required for the preparation of VO2 thin films are different, so researchers at home and abroad have conducted detailed research on the preparation process of VO2 films to find out the best preparation scheme.
Therefore, in this paper, based on the background of VO2 preparation and application, i) we introduce the structural characteristics and phase transition mechanisms of VO2 materials in the second part, ii) discuss the factors that induce the phase transition of VO2, the types of phase transitions, and the changes in other characteristics of VO2, iii) focus on the advantages and disadvantages of four vanadium dioxide film preparation technologies, iv) relate to gas-phase synthesis and liquid-phase synthesis, and propose three VO2 modification strategies to achieve the purpose of regulating the performance of VO2. v) We list five popular applications according to the different thermal, electrical, and optical stimulation signals, finally put forward the challenges faced by VO2 films, and look forward to the direction of VO2 preparation and application.
2 Introduction to VO2 phase change materials
2.1 Crystal structure and band structure
Vanadium is located in the fifth subgroup of the fourth period. As a typical transition metal element, it can combine with oxygen to form 13 oxides. VO2 is a strong electron-related material, and the crystal structure of VO2 will vary depending on the preparation method, including VO2 (R), VO2 (M1), VO2 (M2), VO2 (A), VO2 (B), VO2 (C), VO2 (D), VO2 (T), etc. These different crystal structures differ greatly in some physical properties, such as electron band structure, phase transition temperature, infrared transmission and regulation characteristics, density, specific heat capacity, and thermal conductivity (Figure 2).
2.1.1 Crystal structure
As shown in Figures 3A, B, at 68°C, VO2 undergoes a metal-semiconductor structure transition (MIT) from a low-temperature monoclinic (M1) to a high-temperature rutile (R) phase, during which the lattice structure changes and the conversions are reversible. When the temperature is higher than 68°C, VO2 behaves as a metallic R phase, which is in the P42/mnm space point group. V atoms occupy the positions of the apex and body center of the unit cell; each V atom and the adjacent six oxygen atoms form a regular octahedral structure. The lattice constants are a r = br = 4.55 Å and cr = 2.85 Å. When the temperature is lower than 68°C, VO2 behaves as an insulating M1 phase, which is in the P21/c space point group. Compared with the VO2 structure of the metal R phase, the V atom moves from the center of the octahedron to the surface, deviating from the apex and body center position of the unit cell; the β angle changes from 90° to 122.6° and the V-O octahedron also changes from a regular octahedron to a hemioctahedron. Thus, the highly symmetrical quadrilateral structure changes to a low-symmetrical monoclinic structure, and the lattice constant becomes am1 = 5.75 Å and bm1 = 4.53 Å, cm1 = 5.38 Å.
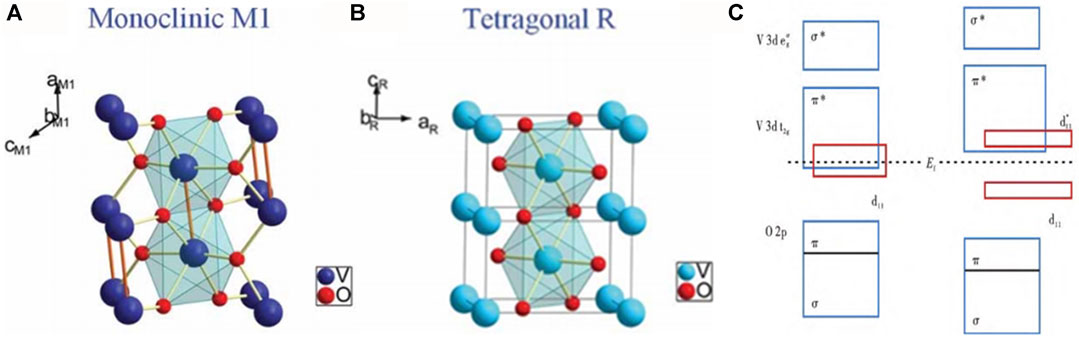
FIGURE 3. Lattice structure of VO2 at low temperature M phase (A) and (B) high temperature R phase (Wu et al., 2013); (C) Band structure changes between VO2 R phase and M phase (Eyert, 2002).
2.1.2 Band structure
In the process of phase transition, in addition to the changes of crystal structure, the changes of band structure also occur (Figure 3C). When VO2 is in the high-temperature metallic phase, the dII orbital and the π* orbital overlap, the π* orbital is partially filled with electrons, and the Fermi energy level is located in the two orbitals. When the temperature is lower than 68°C, the V atoms are displaced along the cr axis to form a jagged V-V structure, which aggravates the hybridization of the 3d orbital of V and the 2p orbital of O, resulting in the upward shift of the π* orbital, and the electrons in the π* orbital are transferred to the dII orbital due to the greater mobility, and the dII orbital splits into a full-band dII orbital and an empty dII* orbital, at which point VO2 transforms into a semiconductor monoclinic structure, which behaves as an insulating phase.
During the MIT conversion of VO2, the specific changes in the band structure from the rutile phase to the monoclinic phase are as follows: 1) The increase of the π* band above the Fermi level EF (relatively decreasing EF and distributed below the π* band) leads to the full state of dII. 2) The band splits into two bands. This phenomenon of separating states increases the activation energy of the semiconductor insulation state to a certain extent.
2.2 Phase transition mechanism
At present, there are three main explanations for the phase transition mechanism of VO2, namely, the Mott-Hubbard phase transition driven by strong electron correlation, the Peierls phase transition driven by the change of the lattice structure, and the synergistic coupling of Mott-Hubbard and Peierls mechanism.
It is believed that structural changes are what drive Peierls’ phase-transformation mechanism. The occurrence of the band gap in the low-temperature semiconductor state is due to the lattice distortion of the monoclinic phase; that is, the phase transition is caused by the instability of the crystal lattice. Budai et al. (Budai et al., 2014) used X-ray and neutron scattering techniques to discover the driving effect of non-harmonic phonons during the phase transition of VO2 (Figure 4B), confirming the decisive role of lattice distortion in the phase transition process. Cavalleri’s (Cavalleri et al., 2004) experiments using ultrafast spectroscopy have shown that the VO2 low-temperature insulator may have important band-like properties, and the band-like properties of the low-temperature insulator are caused by the distortion of Peierls, which further indicates that the MIT phase transition of VO2 is due to the structure-driven.
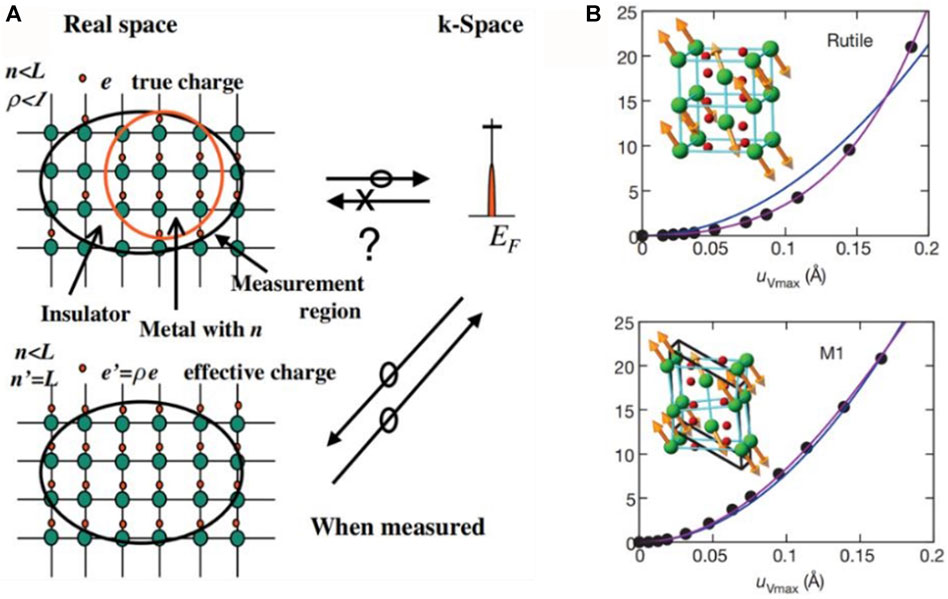
FIGURE 4. Mott phase change and Peierls phase change: (A) Mott A phase transition due to a change in the concentration of electrons in a phase transition; (B) Peierls Phase change V-V Phase transitions due to pairing and lattice distortion (Kim et al., 2004).
When the electron spacing of the crystal shrinks, the upper and lower Hubbard bands overlap and are not satisfied, which is a metallic phase. When the upper and lower Hubbard bands are empty and full, they are insulated. This change in the electron spacing affects the electron interaction, so that the overlapping state of the upper and lower Hubbard bands changes, and the process that determines the MIT transition is called the Mott-Hubbard phase transition (Mott, 1949). The phase transition theory suggests that the strong correlation between electrons is the dominant factor in the VO2 phase transition, which is independent of the change of lattice structure. According to the Mott band theory model, when the concentration of free electrons exceeds a certain concentration, additional electrons in different localized areas will be generated, allowing the material to exhibit metallic properties. When the system has been transformed into a metallic feature, its structure remains in the monoclinic phase (Figure 4A).
Since both the Peierls phase transition theory and the Mott-Hubbard phase transition theory have their own limitations, it is impossible to explain all experimental phenomena with a single theoretical model. As research continues to deepen, more and more people think that SMT is the result of the Peierls phase transition and the Mott-Hubbard phase transition synergy. Yao (Yao et al., 2010) studied MIT transitions. It is found that the curves of V-V coordination twist angle and resistance under temperature change are almost consistent, and it is believed that the electron-lattice distortion combined effect changes the band gap. Paquet (Paquet and Leroux-Hugon, 1980) proposed a phase transition model based on electron-electron interaction and lattice distortion, concluded that the phase transition at temperature is dominated by the strong interaction between electrons, and the two d-bands with overlapping Fermi levels of metallic phases depend on the influence of lattice distortion.
2.3 Factors that induce VO2 phase transition
A common method for inducing MIT is thermal triggering and changing the temperature by heating or cooling. Figure 5A shows that when the temperature rises from 341 K to 344 K, the resistance of the VO2 film decreases by almost four stops (Tokura, 2003). Other methods of triggering a phase change include electricity (Miyano et al., 1997; Qazilbash et al., 2007), optics (Qazilbash, 2007; Tokura, 2003), magnetism (Qazilbash et al., 2007) and strain (Cao et al., 2009). Examples of each method are shown in Figures 5B–E.
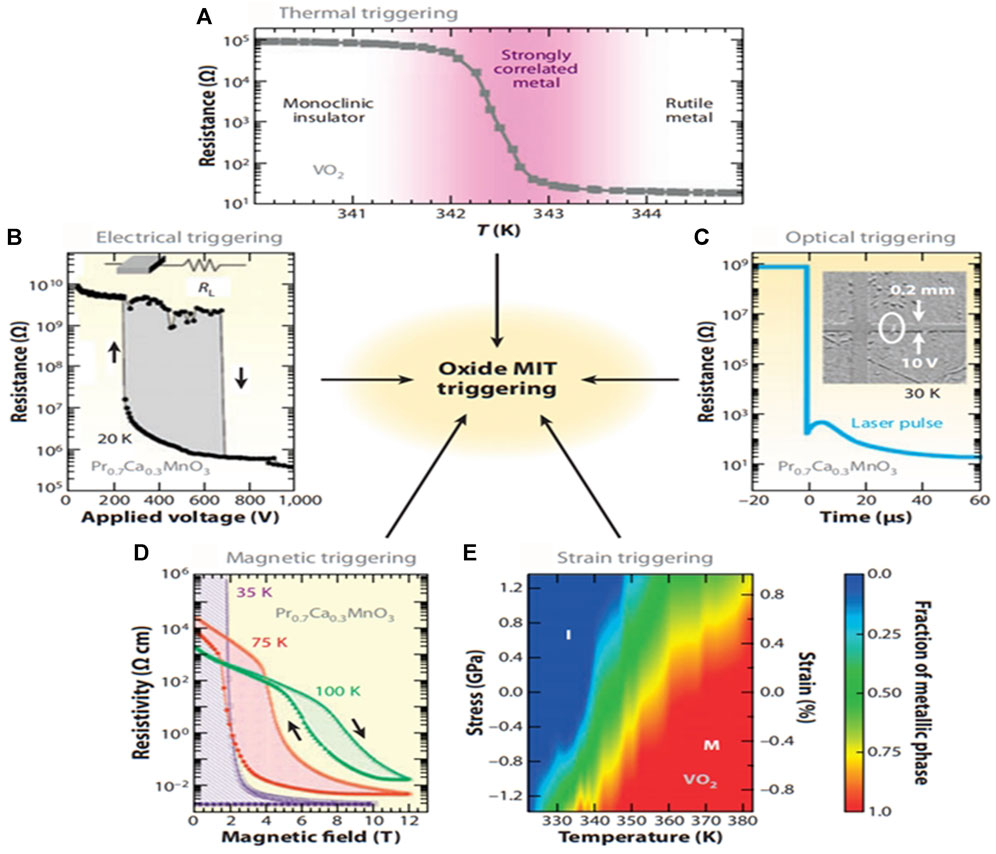
FIGURE 5. MIT triggering methods for relevant oxides: (A) temperature-triggered MIT in VO2 (Qazilbash et al., 2007); (B) electrical triggering (Tokura, 2003); (C) optical triggering (Tokura, 2003); (D) magnetic triggering (Tokura, 2003); (E) Effect of strain/stress on MIT in VO2 (J. Cao et al., 2009).
Thermally induced phase transition is the most common way to induce a phase transition of VO2, which is triggered when the outside temperature rises to the phase transition temperature. In this process, the lattice structure, optical constant (refractive index n, extinction coefficient k), and conductivity of VO2 are abruptly changed. This thermotropic property can be applied to heat-activated devices.
There are two ways to explain electro-induced phase transitions. One view is that holes and electrons introduced by the electric field cause the phase transition of VO2, while another view is that the electrolytically induced phase transition of VO2 is due to Joule heating or electric heating, which is achieved by the local melting of the insulator when an electric current flows through VO2.
In 2000, for the first time, Stefanovich (Stefanovich et al., 2000) reported that the reversible phase transition of VO2 can be triggered by applying bias and electron injection. Kim (Lee et al., 2017) reduced the critical voltage that triggers the VO2 phase transition by increasing the temperature. Wang (Bae et al., 2013) fabricated VO2 two-terminal devices using VO2 nanowires and successfully transformed VO2 from an insulating phase to a metallic phase with a bias voltage of 0.34 V. The phase transition was triggered by Joule heating generated by an electric current.
Chen (Chen et al., 2015a) prepared VO2 epitaxial crystal films on p-GaN (001) substrate by metal-organic chemical vapor deposition (MOCVD), and formed an ideal P-N junction. A bias voltage is applied to the P-N junction, and the phase change modulation of the VO2 film is realized by carrier/electron density control.
In recent years, the study of the laser response and phase transition principle of VO2 has become more and more important. Laser-induced phase transitions cause band collapse by changing the electron correlation instantaneously and finally stabilize through structural evolution.
In 1971, Roach (Roach and Balberg, 1971) and his team showed that ultrafast lasers can effectively excite the reversible phase transition of VO2, a process that is thought to be an energy conversion process between photons and phonons. Cavalleri (Cavalleri et al., 2001) used femtosecond lasers and visible light pulses to study the electrodynamic characteristics of crystal structure change during photoinduced phase transitions and found that when the laser flux increases to a certain size, VO2 can induce a structural transition from the insulating monoclinic phase to the metallic rutile phase.
2.4 Physical, optical, electrical and other properties of VO2
The physical, optical, electrical, and other properties of VO2 also show great variation. Its characteristics change as shown in Figure 6. The phase transition in VO2 can be triggered by stimuli of various types, such as heat, light, electrical, chemical, mechanical, magnetic, etc. The reconfigurable and reversible phase transition by a single-stimulus or multi-stimuli and the dynamically tunable optical properties in a broad range of wavelengths (λ) are the superior features facilitating VO2 in smart windows, optical switching, modulator, sensor, nanoactuator, sensor, smart radiator, transistor, oscillator applications, and so on.
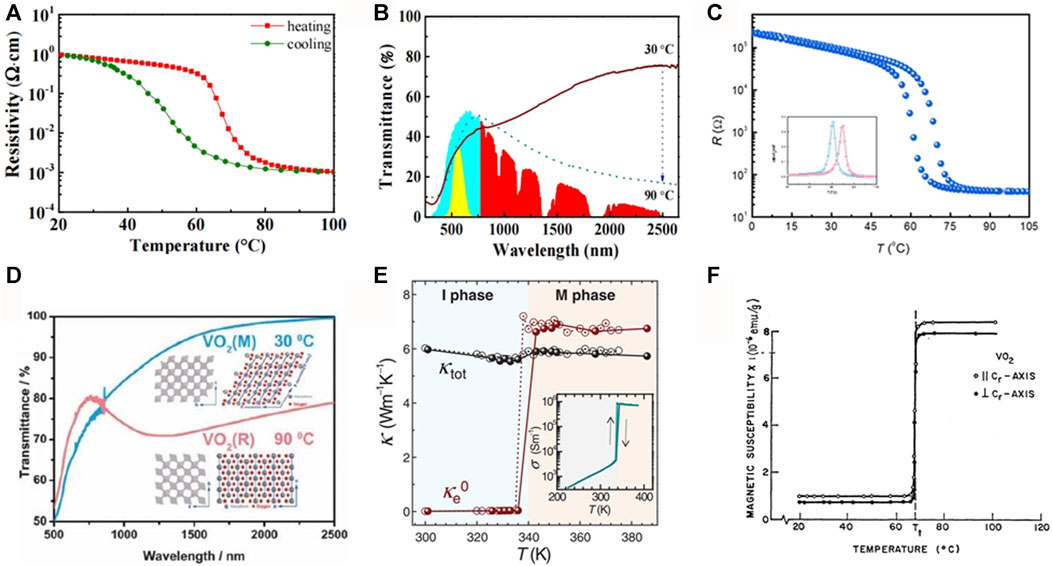
FIGURE 6. VO2 performance changes: (A) Resistivity of VO2 films as a function of temperature, Occurs before and after the VO2 phase transition (Wu et al, 2013); (B) Transmission spectra of VO2 films before and after phase transition (Berglund and Guggenheim, 1969); (C) Electricity (Li et al., 2016); (D) Optics (Li and Dho, 2011); (E) Thermodynamics (Xu et al., 2005); (F) Magnetism (Fu et al., 2013).
When the temperature rises to the phase transition point, VO2 transforms into a tetragonal rutile phase structure with a bandgap of variable conductivity, showing metallic properties, and strong absorption and reflection of low-frequency infrared light, but the transmittance of visible light remains basically unchanged. Before and after the phase transition, the transmittance of the VO2 film remained basically unchanged in the visible band and showed an obvious mutation in the infrared band (Figures 6B, D).
Relevant studies have shown that the VO2 thermal conductivity of high-temperature metal phases is significantly improved compared to VO2 at room temperature, and the VO2 thermal conductivity of metal phases can reach 6 W/MK (Figure 6E).
As shown in Figure 6F, the M1 phase VO2 at room temperature is not magnetic due to the incomplete vanadium oxide chain, while the VO2 of the R phase at high temperature behaves as a paramagnetic metal under the magnetic field, so the magnetic susceptibility increases significantly when the temperature reaches the phase transition point (Berglund and Guggenheim, 1969).
The abrupt change in resistivity is one of the most obvious features of the VO2 phase transition. When the temperature is lower than the phase change temperature, the resistivity of VO2 is very large, about 1∼10 Ω⋅cm, which is insulating or semiconductor, and when the temperature is higher than the phase change temperature, the resistivity decreases sharply, about 10–4∼10–3 Ω⋅cm, showing a metallic state. VO2 will experience a 3-5 orders of magnitude resistance mutation at the phase transition point; the transition speed is fast, stable and reversible; and the phase transition temperature of the heating process and the cooling process is different, so there is a certain hysteresis phenomenon (Figures 6A, C).
3 VO2 film preparation process
VO2 does not undergo volume change during phase change as a thin film material (Kucharczyk and Niklewski, 1979; Béteille et al., 1999), So the film morphology is often used as the main morphology for VO2 research and applications. So far, there are many preparation methods for VO2 films, and different methods are suitable for different application fields.
Currently, the common methods include the sol-gel method (Bian et al., 2016; Chang et al., 2018; Danxia et al., 2015; Fallah Vostakola et al., 2019; Manning et al., 2005; Novodvorsky et al., 2015; Théry et al., 2016; Zou et al., 2019), the magnetron sputtering method (Chang et al., 2018), the vapour phase deposition method (Manning et al., 2005), the pulsed laser precipitation method (Novodvorsky et al., 2015), and so on (Bian et al., 2016; Théry et al., 2016). These test methods have some of the same drawbacks to a certain extent, such as high requirements for test equipment, complex process parameters, difficulty stabilising the control, poor process repeatability, and film properties. To make VO2 films have a broader application prospect, future research work should focus on the following points: 1) optimise the VO2 film preparation process. Such methods as pulsed laser deposition, magnetron sputtering, and the sol-gel method can be used to obtain high-quality VO2 films, but the appropriate choice of substrate and annealing process is the main factor affecting the performance of the prepared VO2 films. Therefore, to prepare VO2 films with excellent performance, the process parameters for preparing VO2 films need to be continuously optimised. 2) Reduce the phase transition temperature of the VO2 film. 68°C phase transition temperature to a certain extent limits the application of VO2 film, due to most of the applications of VO2 requiring its phase transition temperature to be close to room temperature, and doping is currently one of the most effective means to reduce the phase transition temperature of VO2, so as far as possible, reduce the phase transition temperature of VO2 and make the doped VO2 film have optical and electrical properties to meet the requirements of the application of the future research focus. Therefore, minimizing the phase transition temperature of VO2 and making the optical and electrical properties of the doped VO2 films meet the requirements of applications are the focus of future research. 3) preparation of composite multilayer VO2 film structures to enhance the phase transition properties and improve the magnitude of changes in electrical and optical properties before and after the phase transition. Some studies have shown (Zhou et al., 2013) that optimizing the optical design of TiO2/VO2 bilayer film and preparing a TiO2 minus emission layer with a thickness of one-quarter wavelength can appropriately improve the transmittance and switching efficiency, but the study failed to address how to maintain high transmittance and switching efficiency while controlling the temperature of the phase transition. To obtain VO2 films with the desired properties, this requires more in-depth theoretical studies and technical exploration by researchers.
3.1 Pulsed laser deposition (PLD)
Pulsed laser deposition is a typical physical deposition coating process, as shown in Figure 7C, in which a pulsed laser emits a high-energy beam onto a target until it reaches a molten state, and then the target is jetted onto a sinking bottom to form the desired thin film, typically vanadium, VO2, or V2O5. The deposition atmosphere is generally oxygen or oxygen-argon mixed gas, and adjusting the partial pressure of oxygen can deposit vanadium oxide films with different oxygen contents, including vanadium dioxide.
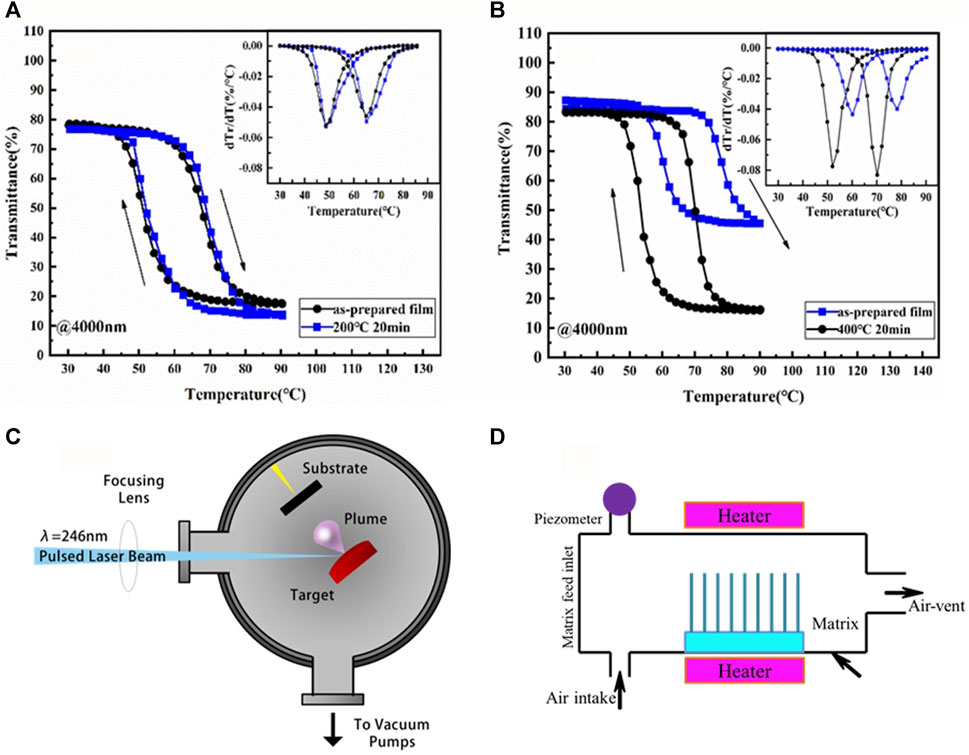
FIGURE 7. (A,B) Optical and Electrical Properties of VO2 Thin Films (D. Li et al., 2015); (C) Schematic diagram of pulse laser deposition system; (D) Schematic diagram of CVD system.
The advantage of this process is that the composition is easy to control and can prepare thin films with complex components. In 1993, M. Borek et al. (1993) attempted to deposit VO2 thin films using metal vanadium targets and introduced a mixture of argon and oxygen. A VO2 thin film with a significant phase transition effect was obtained at a substrate temperature of 500°C, and it was found that oxygen partial pressure has a significant impact on the valence state of the final product. Sayid Bukhari et al. (2020) investigated the effect of oxygen flow rate on the phase transition characteristics of VO2 thin films deposited on amorphous thermal oxide (Si/SiO2) substrates by the PLD method, suggesting that oxygen diffusion may play a crucial role due to flow rate.
The thin film prepared by this method can also be doped with other elements, and the deposition speed is fast and pollution-free, with good crystal quality and a flat surface. M. Soltani et al. (2004) used PLD to deposit W-doped VO2 films and W- and Ti-doped VO2 films on quartz surfaces, respectively. Research has found that the infrared transmittance of V1-x-yWxTiyO2 thin film is significantly higher than that of V1-xWxO2 thin film. W doping can effectively reduce the phase transition temperature point of VO2 thin film, and the introduction of Ti can reduce the width of the thermal loop and increase the resistance temperature coefficient. However, this method also has obvious drawbacks. When laser bombardment occurs, particle aggregates are formed, and their composition uniformity can only be maintained within a small area; therefore, the deposited thin film can only be obtained on a very small substrate and cannot be prepared on a large area.
3.2 Magnetron sputtering method
The sputtering method is the most common and direct method for preparing VO2 thin films, and the general sputtering device will be attached to a magnetic field, so this preparation process combining magnetron technology and bipolar sputtering technology is called the magnetron sputtering method. It belongs to the physical vapor deposition process. During sputtering, the V-containing particles are continuously emitted to the rotating substrate, and these particles collide with the substrate surface or other particles, some of which are reflected and consumed, and the other part stays on the surface. The trapped particles then undergo surface diffusion and migration, some of which evaporate again and some of which fall into the bottom of the potential energy and are adsorbed by the surface; that is, the condensation process occurs. The condensation process is generally the generation and continuous growth of island particles, that is, the formation and growth of crystal nuclei, and finally the formation of a continuous film (Z. Zhang and Lagally, 1997). Its advantages are that the deposition rate of the film is relatively fast, the composition is easy to control, the film adhesion ability is strong, and the sequential multi-layer preparation of the composite film can be realized. According to the difference in the power supply used in sputtering equipment, it can be divided into RF magnetron sputtering, ion beam sputtering, and DC magnetron sputtering.
Magnetron sputtering has the advantages of high adhesion and easy control of film thickness, but the common problem is that the film has low purity and often contains vanadium oxides in other valence states. The presence of these substances affects the phase transition temperature and its characteristics. The study of preparing VO2 films by magnetron sputtering began after 1970 (Babulanam et al., 1987), it has been widely adopted to this day and has undergone extensive research by scholars during this period. Xu Guo et al. (G. Xu et al., 2005) used radiofrequency reactive magnetron sputtering to the epitaxial growth of VO2 thin films on α-AlO (0001) substrate was studied to investigate the effect of 3–150 nm film thickness on optical properties. The results showed that as the film thickness decreased, the crystal metal phase transition temperature of VO2 thin films significantly decreased. The regulation of MIT phase transition temperature is also of great significance in the preparation of VO2 thin films. Jiang Meng et al. (M. Jiang et al., 2014) successfully synthesized VO2 thin films with various oxygen flow ratios on quartz glass through reactive magnetron sputtering. They found that by accurately adjusting the oxygen flow ratio without doping elements, the phase transition temperature can be controlled between 46°C and 72°C. Compared with traditional magnetron sputtering technology, it can deposit high-quality thin films, and the VO2 thin films deposited using this method have excellent performance.
Compared with traditional RF magnetron sputtering, the VO2 thin film prepared by HiPIMS technology is denser, smoother, and has strong anti-aging performance. As Dou et al. (2021) found, the thermochromic ability of VO2 can be optimized by adjusting the average power of HiPIMS, and VO2 deposited on polished aluminum foil can change its infrared emissivity at different temperatures, making it an ideal choice for infrared signals and detection in many industrial and military applications.
VO2 generated by sputtering is not very pure and often contains other oxides of vanadium, such as V2O5, V2O3, VO, etc. The presence of these oxides affects the phase transition properties. The presence of these oxides affects the nature of the phase transition. To improve the purity of VO2, annealing, and laser sintering can be used to control the process conditions. Annealing is at 450°C temperature in the N2 gas stream holding 2–10 h, and sintering is at 350°C with a ruby laser emitted by the 0.6943 μm laser on the membrane layer to irradiate the beam energy of a few joules per square centimeter of irradiation period of 2 ms. Annealing or laser sintering can greatly increase the temperature-phase transition of the conductivity and the amplitude of the jump. The larger the jump, the higher the purity of the VO2 component. Geng Chen (Geng et al., 2022) prepared surface nanoparticle thin films by combining HiPIMS with annealing post-treatment. The particle size of the surface nanoparticle can be adjusted by controlling the oxygen flow rate. However, there is currently a lack of detailed research on the film formation mechanism during high-energy pulse magnetron sputtering deposition.
3.3 Sol-gel method
The sol-gel method is a commonly used chemical preparation method for the preparation of VO2 films. Compared with other preparation processes, the equipment used in this process is simple, and because of the use of sol instead of powder, the product has high uniform purity, easy stoichiometry, and can be applied to the preparation of large-area films, so it is widely used. The sol-gel method can be divided into two categories according to the different formation processes: the inorganic sol-gel method and the organic sol-gel method, both of which can be used to prepare VO2 films with excellent performance.
In 1996, Lu et al. (1997) successfully prepared VO2 films using the organosol-gel method and explained that the phase transition of VO2 materials is the transition from monoclinic to tetragonal rutile by coordination field theory. Li et al. (2015) prepared VO2 films on muscovite substrates using the inorganic sol-gel method, and investigated the thermal stability of the films by changing the annealing temperature and annealing time. The crystal structure and morphology of the films before and after annealing were studied by X-ray diffraction and other equipment (Figures 7A, B), and the VO2 films were quite stable at an annealing temperature of 200°C, while when the annealing temperature reached 400°C, the phase transformation temperature of the VO2 films would rise, and the VO2 would gradually oxidize to V2O5, resulting in a decrease in the phase transformation efficiency of the VO2 films. The organosol-gel method can also be doped with other ions to improve its characteristics. Chae et al. (2008) prepared W-doped and Ti-doped films using an organosol-gel method, respectively, and combined with a simplified annealing process, the films containing 12% W atoms doped showed a decrease in the phase resistance of the insulator, while the 20% Ti atom-doped films showed a smaller change in resistance at the phase transition temperature. In the future, the organosol-gel method needs to be further studied in the selection of doped ions so that the phase change temperature is more suitable for the application and cheaper raw materials can be found to reduce the cost.
For both inorganic and organic methods, the process should be improved to reduce the preparation cycle and improve efficiency. For example, the process of melting raw materials can be combined with electromagnetic technology to improve the melting efficiency. The selection of substrate is also very important; the appropriate substrate will make the film more uniform, more adhesion, and more dense, so in addition to the commonly used mica and silicon wafers, we should also find more excellent materials.
3.4 Chemical vapor deposition (CVD)
Chemical vapor deposition is a traditional technology for preparing thin films, the principle of which is to use gaseous precursor reactants to decompose certain components in the gaseous precursor through atomic and molecular chemical reactions and form thin films or other crystals on the matrix substrate. A schematic diagram of the chemical vapor deposition method is shown (Figure 7D). The preparation of VO2 films from CVD was first reported in 1966, and this method has since been greatly popularized. At present, the vanadium sources for the preparation of VO2 films by CVD method are mainly various metal-organic compounds, such as V (acac)3, VO(acac)2, and VO(OC3H7)3 (Binions et al., 2007a; Binions et al., 2007b; Piccirillo et al., 2007). The process of preparing VO2 films from CVD generally requires the deposition of vanadium oxides (such as V2O5) and then the reduction to +4-valent VO2 films in a reducing atmosphere. This method is widely used in the development of new crystals, the purification of substances, and the deposition of various monocrystalline and polycrystalline thin film materials.
The raw materials used in this method are relatively inexpensive and do not require vacuum equipment, so the cost is low, while the deposition efficiency is very high and the product quality is very stable. At the same time, the disadvantages of the CVD method are also very obvious. The film preparation process is complex, and the structure and growth form of the film are strongly affected by the substrate temperature, reaction gas composition, gas flow rate, and other factors that are difficult to control, so it is not conducive to large-area preparation. Vernardou et al. (Vernardou et al., 2011) used atmospheric pressure vapor deposition to prepare thin films on glass substrates and found that the purity and structural properties of vanadium dioxide were affected by the solution ratio. Sahana et al. (Sahana et al., 2002) used diacetylacetone oxide vanadium oxide as a raw material to prepare thin films with glass as substrate by the low-pressure vapor deposition method and determined the temperature range (748∼793 K) with the highest purity of vanadium dioxide deposited.
The current development trend is to change the phase transition temperature defects. This is due to the fact that different materials have different evaporation rates, so it is not easy to control the proportion of components. Manning et al. (2004) used VCl4 as a raw material to fully study the CVD method for the preparation of VO2 films and successfully doped VO2 films with a variety of elements (W, Ti, Mo, and Nb). They considered that the appropriate doping elements could effectively reduce the phase transition temperature of the film, and the phase transition temperature of the film gradually decreased with the increase in doping amount.
3.5 Other preparation processes
In addition to the above commonly used preparation methods, there are the hydrothermal method, thermal decomposition, hydrogen-water balance method, evaporation method, etc. But there are limitations: it is not easy to achieve industrial production; the film obtained by the evaporation method has poor compactness and low adhesion; the hydrogen-water balance method is still in the laboratory stage; and it needs to be improved in order to achieve industrialization. It is also necessary to find new preparation processes to prepare films with different characteristics to meet different needs.
4 Regulation of VO2 film properties
VO2 flexible film is a specific film based on the thermochromic performance of VO2 nanoparticles, which can actively adjust its own optical transmittance at different external temperatures. The layered structure is the basic property of VO2 films, and light hitting different layers of the film is divided into two parts: reflection and transmission. According to the Maxwell-Garnett effective medium theory (EMT), adjusting the size of nanoparticles in layered coatings can obtain effective dielectric functions with different values, which in turn affect their transmittance and reflectivity (Laaksonen et al., 2014).
There are a number of ways to change the phase transition temperature of vanadium dioxide films, such as doping, stress, particle size, non-chemical stoichiometry, microstructure, etc. Of these, doping is considered to be the most direct, effective, and practical for application. Among them, doping is considered to be the most direct, effective, and practicable method of application. Jorgenson and Lee (1986) proposed as early as 1986 the direct preparation of ion-doped VO2 thin films (V1 -xMxO2) by sputtering, where M denotes the doped ions. Until now, there have been many reports on doped VO2 films. In addition to doping elements to enhance both visible light transmittance and solar modulation, methods such as preparing porous materials (Zhou et al., 2013; Cao et al., 2014a), compositing vanadium dioxide with other functional materials to form core-shell structures (Gao et al., 2012; Li et al., 2013), and heterostructures (Chen et al., 2004; Zhou et al., 2014) are also able to take into account both of the above-mentioned performance enhancements. While it is one of the ultimate goals of researchers to apply VO2 films on a large scale for energy-saving applications, the dimming ability of VO2 films needs to be further improved, and multilayer compositing of VO2 films has been considered the most promising process to improve the overall performance of the products. Surface structure also has an impact on the performance of VO2 films, in which the use of printing can achieve large-scale, high-volume production of VO2 films, which is conducive to expanding the application of VO2 films in the intelligence of cold chain packaging systems. In this section, we summarise the existing domestic and international research explorations on the regulation of VO2 film properties, starting from the visible light transmittance and solar energy regulation, phase transition temperature, weathering resistance, large-scale coating, and other application performance indexes of vanadium dioxide films.
4.1 Effect of elemental doping on the properties of VO2 films
4.1.1 Effect of single element doping on the properties of VO2 films
Doping is one of the most effective ways to reduce the phase transition temperature of VO2 films (Table 1), and the doping of Mg2+, Al3+, Ti4+, Tb3+, Mo6+, W6+, and F− can play a role in reducing Tc (Chen et al., 2017). So far, W6+ is the most effective dopant for reducing Tc among all elements, and every 1% of the number of W atoms doped can reduce Tc by about 20∼26°C (Chen et al., 2015).
Chen et al. (2017) suggested that this is due to the change in the electronic structure of VO2 due to W doping. For WxV1-xO2 films, W6+ enters the VO2 lattice and replaces V4+, breaks the V4+—V4+ covalent bond and reconstructs V3+—W6+ along the axis of VO2(M), and the two electrons in the d orbital of the W ion combine with the adjacent V3+—V4+ along the VO2(M) axis to compensate for the loss of the V4+—V4+ covalent bond. Therefore, the phase transition temperature of the low-temperature semiconductor VO2(M) is very unstable, the energy level of the dII. orbital and the O2p orbital decreases, and the relative orbital position of the π* and dII. orbitals shifts, which indicates that the occupancy of the dII. orbital decreases and also means that the intensity of the V—V bond interaction decreases. This will cause the Fermi level guide band to move and reduce the band gap, so Tc decreases and VO2 has distinct metallic properties. The same theoretical principle applies to the doping of other metals.
Wang et al. (2016) first reported the incorporation of terbium cation (Tb3+) into VO2 films to reduce Tc and increase Tlum. When the doped atomic fraction was 2%, the Tlum and ΔTsol of the film increased from 45.8% to 7.7%–54.0% and 8.3%, respectively. Moreover, when the atomic number fraction of Tb3+ reaches 6%, the Tlum of the film can be as high as 79.4%.
Single-element doping, in some cases, does not always produce good results, For example, single Mg doping can improve the optical properties of the film, but it is not conducive to the reduction of Tc, which hinders the application range of VO2 film.
4.1.2 Effect of multi-element co-doping on the properties of VO2 films
It has been found that the use of co-doping is used to make VO2. Modification can effectively reduce the adverse consequences of single-element doping TC and, at the same time, further adjust its optical performance.M. K. Dietrich et al. (Dietrich et al., 2017) prepared a tungsten-strontium (Sr) co-doped VO2 film, in which, with the increase in the doping amount of Sr (atomic radius of 215.1 p.m.), the atomic overlapping orbitals will decrease correspondingly, thereby increasing the band gap, and the film will gradually appear grayish-white. When the doped atomic fractions of Sr and W were 11.9% and 0.9%, respectively, the Tlum of the film reached 61.4%. In addition, the researchers also targeted W-F (Burkhardt et al., 2002), W-Mg (Wang et al., 2015a) and W-Al (Yanase et al., 2018). The plasma combination was used to explore the direction of co-doping.
In summary, it can be seen that the phase transition temperature of VO2 can be controlled by chemical element doping while maintaining the optical properties of VO2. Table 1 shows the effects of different doping elements on the properties of VO2 films. The main elements commonly used in doping VO2 materials to reduce their phase transition temperature are W6+, Mo6+, etc., which can change the energy level structure of V4+, and they are comparable to the ionic radius of V4+, which can avoid the destruction of VO2 crystal structure.
4.2 Effect of composite microstructure on the properties of VO2 thin films
Core-shell structures have received increasing attention due to their replaceable components and modifying effects. VO2-based core-shell structures can obtain more properties, such as SiO2 shells, which can significantly enhance the antioxidant and optical properties of VO2 nanoparticles, etc. Gao et al. (2012) prepared a stable and transparent SiO2/VO2 core-shell structure. It has UV-shielding properties and an obvious temperature-responsive thermochromic phenomenon in the near-infrared region. Another VO2(M)@SiO2 nanofiber structure prepared by a one-step hydrothermal method (Li et al., 2013) can have a visible light transmittance of more than 50%. This is the result of the combined effect of a smaller fibre radius and a narrower light-acting space.
In addition to the core-shell structure, the hybrid structure is also an effective way to improve the thermochromic properties of vanadium dioxide. Chen et al. (2004) prepared a nano-VO2-SiO2 monolayer hybrid membrane with high visible light transmittance using the sol-gel method. In the membrane, the presence of SiO2 increased the visible light transmittance without sacrificing other properties of the membrane. The VO2-SiO2 monolayers with a Si/V ratio of 0.05 showed up to 75% transmittance at 700 nm compared to the normal VO2 films. Zhou et al. (2014) reported VO2 hydrogel hybrid films with ultrahigh optical properties, as shown in Figure 8. Combining inorganic VO2 with organic thermochromic materials, they found that the introduction of poly N-isopropylacrylamide (PNIPAm) significantly increased the ΔTsol to 25.5% while maintaining 70.7% Tlum. The reason for this dramatic improvement in performance is that VO2 and PNIPAm hydrogels exhibit different visible transmittance and solar modulation at low and high temperatures. VO2 exhibits low Tlum and high Tsol, while PNIPAm exhibits high Tlum and low Tsol, and the coupling of the two results in a balance of Tlum and Tsol.
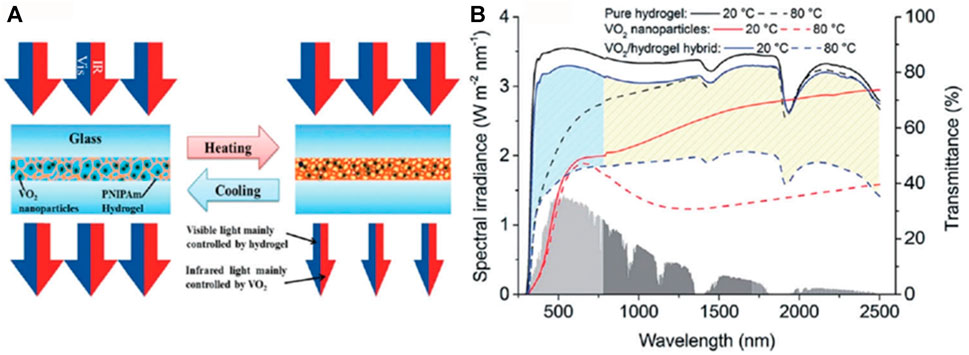
FIGURE 8. VO2/PNIPAm: (A) Solar light regulation mechanism; (B) UV-Vis NIR spectra (Zhou et al., 2014).
4.3 Effect of multi-coat composite on VO2 films
4.3.1 VO2 double-layer film composite
VO2 bilayer film is a double-layer film made by compounding unmodified VO2 film with other functional films by coating, embedding, or deposition, thereby improving the optical properties of VO2 film. Hao Q et al. (Hao et al., 2018) embedded a TiN plasmonic nanoarray with a hexagonal superlattice structure between the substrate and the VO2 film and relied on the good absorption performance of TiN for near-infrared (NIR) light to create a local heating phenomenon to promote the phase transition rate of the VO2 film. Based on this, VO2/TiN composite films can be prepared that can sensitively respond to changes in external temperature and light intensity. The smart coating blocks direct infrared light at 28°C and transmits infrared light at low light intensity or below 20°C. In addition, the film is capable of achieving a Tlum of 51% and an infrared conversion efficiency of 48% at 2000 nm. However, this method requires nitriding of titanium oxide at 850°C for 10 h and subsequent annealing of the composite film, which is complex and requires high energy consumption.
The refractive index of VO2 films is different before and after the phase transition (n (VO2(M)) = 2.7∼2.8, n (VO2(R)) = 2.0∼2.5), and all of them are greater than the refractive index of air (nair = 1) (Li et al, 2013), resulting in a higher reflectivity of the VO2 film when exposed to sunlight, which reduces the optical performance of the VO2 film. Antireflective structure (ARS) is a functional structure of thin film materials that can further reflect the light refracted in the film to reduce the loss of light and has a better anti-reflection effect on the surface of the film when it is lower or higher than the phase change temperature.
4.3.2 VO2 multilayer film (number of layers≥3) lamination
On the basis of the VO2 bilayer film, the researchers have conducted in-depth research on multilayer VO2 films with a number of n ≥ 3 layers, which can not only further reduce the loss of light to enhance the transmittance of the film but also expand the application range of VO2 multilayer films according to the characteristics of different functional layers. Studies have shown that the Tlum of a three-layer VO2 film can reach up to 86%, while the five-layer structure can achieve a ΔTsol increase of about 12% (Liu et al., 2013). Mlyuka et al. (2009) prepared a five-layer hybrid film of TiO2/VO2/TiO2/TiO2 by magnetron sputtering by using a TiO2 layer with high light transmittance and anti-reaction effect and two layers of VO2 thermochromic layer, and the hybrid film had high Tlum and ΔTsol (Tlum = 45%, ΔTsol = 12.1%).
In summary, the multi-coating structure design of VO2 films has been regarded as the most promising means to improve the comprehensive properties of thin films. Table 2 shows a comparison of the optical properties of different layered VO2 films, among which there are few reports on the structure of more than three layers, which may be due to the fact that the synthesis process of VO2 films is not easy to control and the preparation process of multilayer films is complex. Although the multi-layer coating structure of VO2 film is mainly to improve the practical performance of VO2 film itself, such as dimming ability, phase change temperature, durability, etc., there is also room for the development of multi-layer film structure design. For example, based on the performance of the composite layer, it can be multi-functional (self-cleaning, anti-fogging, scratch resistance, etc.) at the same time to meet the diverse needs of VO2 film materials in the use process.
4.4 Effect of surface structure on VO2 films
4.4.1 VO2 nanoparticle films
When light comes into contact with the surface structure of nanoparticles or thin films, different degrees of transmission and reflection occur. Core-shell nanocomposites composed of VO2 nanoparticles and highly transparent metal oxides such as TiO2 have emerged as a new material to enhance visible light transmittance (Wang et al, 2016). On the one hand, the highly transparent metal oxide housing material does not block the ingress of light, and at the same time, it can use its anti-reaction effect to reduce light loss and improve the optical properties of VO2 films. On the other hand, the housing material can prevent O2 or acid erosion and enhance the chemical stability of VO2 nanoparticles.
Ji et al. (2019) synthesized novel VO2/ZnS core-shell nanoparticles (Ji et al., 2018a) using thioglycolic acid as ligand, and used them as raw materials to prepare inks. The VO2 film prepared by inkjet printing technology based on infrared transparent nano-polyethylene has good infrared thermochromic performance, and the ΔTsol is 32.4% at a fixed wavelength of 20.0 μm. In addition, a 560 cm2 large-size VO2 nanoparticle film with good homogeneity was successfully fabricated. This is conducive to expanding the application of VO2 flexible thermal insulation and temperature control film in cold chain packaging systems and realizing the research and development of large-capacity VO2 thermochromic intelligent incubators, thereby helping to save cold chain logistics and transportation costs.
4.4.2 VO2 film surface patterning
In addition to the surface modification of nanoparticles, the design of patterned structures on the surface of thin films is also one of the research hotspots. Cao et al. (2017) prepared a single layer of dense polystyrene (PS) spheres on a fluorine-doped SnO2 conductive glass SnO2:F (FTO) substrate using a gas-liquid interface self-assembly method, followed by a mixture of SiO2 and different amounts of VOC2O4 and spin-coating directly between the PS balls, and finally toluene to remove the monolayer of PS balls. The prepared smooth film is converted into VO2/SiO2 film by a rapid thermal annealing process in a nitrogen atmosphere. The ordered VO2/SiO2 composite film has good thermochromic properties with a ΔTsol of 8.42% and an increase in Tlum to 55.6%.
Lu et al. (2016) used screen printing to prepare VO2 films with periodic and micropatterned structures. Such a network structure can enhance visible light transmittance (Tlum = 21.4%) compared to conventional continuous nanocomposite films. Due to the openness of the grid, VO2 films with a thickness of 1,000 μm can be prepared, so the ΔTsol increases with the VO2 powder content. Compared with the theoretical results of the lattice structure films, the experimentally measured ΔTsol is as high as 17.2%, which exceeds the calculated value of ΔTsol = 16.5%.
In general, the surface structure of VO2 films can be adjusted by designing the patterned structure of nanoparticles and film surfaces, the optical properties of the prepared VO2 films can be improved, and the chemical stability of the films can be enhanced.
5 Research on the application of VO2 film
The vanadium dioxide phase transition is usually achieved in thermally induced phase transformation, electrolytically induced phase transformation and photoinduced phase transformation, and the optical properties before and after the phase transformation are abruptly changed (Li et al., 2017; Taylor et al., 2013). Based on this characteristic, vanadium dioxide is widely used in smart windows, optical switches, terahertz modulators, optical storage materials, transistors and other fields. This article lists some popular applications of vanadium dioxide.
5.1 Thermochromic smart windows
Building energy consumption is an important aspect of the total energy consumption of society, accounting for about one-third of it. Windows are the least energy-efficient part of a building due to their extremely low efficiency, and their energy consumption accounts for a large part of the building’s energy consumption (Xu et al., 2018). As the demand for architectural glass surges, so does the demand for thermal insulation, so the application of smart glass is becoming more and more extensive. The thermochromic coating has high transparency for the incident indoor light at low temperatures and high reflection for the light in the incident room at high temperatures, so it can intelligently adjust the indoor temperature and reduce heating and cooling energy consumption in the building (Wu et al, 2013). As a special phase change material, vanadium dioxide film has a phase change temperature of 68°C, which can undergo SMT transformation according to the change in temperature. Before and after the phase transition, it has very different optical properties. For example, the transmittance of visible light to infrared light changes from high transmission to high reflection, but this does not happen before or after the phase transition. The monoclinic to rutile phase transition of vanadium dioxide is accompanied by a sudden change in optical properties and even reaches a modulation amplitude of 80% in the near-infrared region (Chen et al., 2019). This excellent thermochromic property makes vanadium dioxide a popular material for designing smart windows. In fact, there have been a lot of reports on the application of VO2 in the direction of smart windows. However, there are still several difficulties in the application of vanadium dioxide in the direction of smart windows: 1) The phase change temperature is adjusted to near room temperature; 2) The environmental stability of VO2 thin film coatings is altered; and 3) Improve visible light transmittance; 4) Improve the ability to regulate solar energy (Zhang et al, 2020). This necessitates the modification of the smart window to improve its performance by improving the visible light transmittance and solar energy modulation amplitude.
For example, WU et al. from Wuhan University of Technology successfully prepared a microporous vanadium dioxide film using cotton as a template (Wu et al., 2018). By changing the temperature of the hydrothermal reaction, the micropore size and the grain size of vanadium dioxide can be adjusted, and when the hydrothermal reaction reaches 180°C, the grain size of vanadium dioxide is 60.7 nm, and the modulation amplitude of sunlight is maintained at 12.9%. Other than that, Qian et al. have taken a unique approach by borrowing from a biomimetic structure (Figure 9A), where vanadium dioxide is deposited in the imitation moth eye on the nano-silica structure (Qian et al., 2014). When the size of a single moth-like eye reaches 21 nm, the visible light transmittance and sunlight energy modulation amplitudes of the film reach 44.5% and 7.1%. Most important of all, the thin film simulations show that this structure might be able to reach a 70% pass rate and a 15.5% sunlight energy modulation amplitude (Figure 9B).
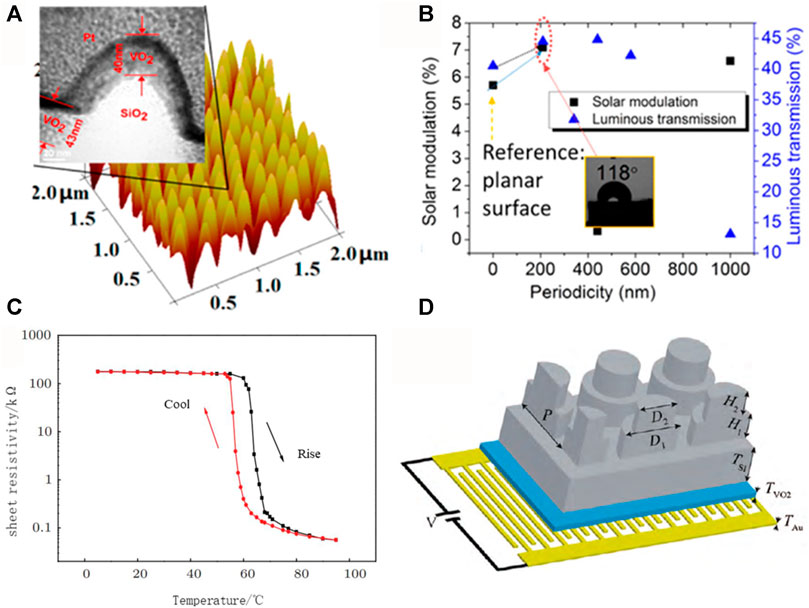
FIGURE 9. (A) Moth-like eye structure vanadium dioxide and its; (B) thermochromic properties (Qian et al., 2014); (C) the temperature of the vanadium dioxide film-Resistance change curve (Reese et al., 2004); (D) Broadband THz switch schematic (Schalch et al., 2020).
In order to solve the problem that the TC of VO2 films is too high and the Tlum is too small, the researchers studied the thermochromic properties of elemental doping. As a thermochromic coating material, the TC of VO2 film should ideally be reduced to around room temperature (25°C). Doping includes single-element doping and multi-element doping. Among the two doping methods, the study of single-element doping is more extensive and in-depth. Among the many doped elements, W has the best effect on reducing the TC of VO2 nanoparticles. Li et al. prepared mesoporous tungsten oxide nanocrystals doped with tungsten (W) by hydrothermal method (Li et al., 2019). By adjusting the doping amount of W, the phase transition temperature of VO2 changes inversely. With the increase in W content, TC decreased significantly and ΔTsol increased significantly. The paper increases the content of W from 0.2 at% to 0.6 at% and reduces the phase transition temperature of VO2 from 56.9°C to 39.5°C. Compared with the undoped VO2, the phase transition temperature (TC = 39.5°C) of the 0.6 at% doped VO2 film is very close to room temperature, which is conducive to practical application. Zhang et al. prepared pure w-doped thin films (20–50 nm) by a simple hydrothermal recrystallization method. In the range of 0–1.1 at%, the phase transition temperature of W-doped VO2 decreases with the increase in W doping amount, which is 28.4°C/W. In addition, nano-titanium dioxide films exhibit excellent thermochromic properties at 42.7°C for TC, 61.7% for Tlum, and 11.7% for ΔTsol (Zhang et al., 2020). The doping of the W element achieves the effect of reducing TC at the expense of tum and ΔTsol. Unfortunately, high-valent doping, similar to W-doping, also significantly reduces the optical properties of VO2 films. Since most single-element doping can only partially alter thermochromic properties, multi-element doping is used to improve overall performance. Doping W is the most effective way to reduce the phase transition temperature. W is mixed with cations that have lower equivalent states for magnesium (Mg), strontium (Sr), rare earths, and aluminium (Al) compared to V4+. This shows that W can improve Tlum. Guo et al. synthesised W-Zr-doped VO2 by a feasible hydrothermal method, and the appropriate W-doping level significantly reduced TC (Guo et al., 2021). The incorporation of Zr significantly increased the Tlum and ΔTsol values of VO2. The addition of W and Zr had a significant influence on the microstructure properties of the VO2 film, with samples of V0.95 W0.01 Zr0.040 O2 and V0.94 W0.02 Zr0.04 O2 showing the purple and flower-shaped shapes, respectively. The V0.95 W0.01 Zr0.040 O2 film exhibited excellent thermochromic properties (TC, Tlum and ΔTsol) at 46.9°C, 60.7%, and 10.6%, respectively. In addition to the above-mentioned co-doping with W, the researchers also found that other elements of co-doping can improve VO2 film performance. C. JI et al. used a co-doping strategy to lower the temperature at which the phases changed and keep the improved optical performance. After the VO2 film was co-doped with Fe (9.2%) and Mg (3%), all the thermochromic parameters were enhanced on the glass substrate, among which the light transmittance was increased to 42.1%, the solar modulation ability was increased to 12.8%, and the phase change temperature was reduced to 38.2°C. Ji et al. (2018b) discovered that the co-dopant Fe3+/Mg2+ works together to stop the neutralization of e-and h+ carriers. This creates a good balance between the temperature at which light passes through and the temperature at which the phase transition occurs (Ji et al., 2018c). In 2021, Y. Zhang et al. made a ZnV2O7-VO2 composite film on a quartz substrate using a magnetron co-sputtering system. They added Zn2V2O7 (Sameie et al., 2018) to make the sample more clear and bright by increasing the band gap from 1.7 eV for pure VO2 to 2.8 eV for the composite ZnV2O7-VO2, which caused a blue shift at the absorption edge and better light transmission (Zhang et al., 2021). The introduction of Zn2V2O7 makes the composite film exhibit a better thermochromic performance value, with a light transmittance of 49.0%, a solar modulation capacity of 34.1%, and a phase change temperature of 43.8°C compared with that of pure VO2 film.
The high phase transition temperature of VO2 films is a major challenge for their application in smart windows. We describe that doping, especially multi-element doping, can effectively reduce the phase transition temperature and balance the optical properties. The phase transition temperature of VO2 films can be lowered in other ways besides doping, such as by adding intrinsic defects and strains, but these methods give up the thermochromic properties. VO2 nanoparticles, on the other hand, have been improved in how they are made and how their surfaces are treated. Nucleo-shellization and hybridization, for example, have been shown to be good ways to balance the phase transition temperature and thermochromic properties. SiO2, TiO2 and ZnO are all used as VO2 coatings in the enclosure because they have high visibility or infrared transparency (Shen et al., 2021). Chen et al. described a way to directly coat vanadium dioxide nanoparticles with a layer of zinc oxide shell. The zinc oxide acts as a protective layer and an anti-reflective layer on the outer layer, and it has a higher visible light transmittance (51.0%) and sunlight energy modulation amplitude (19.1%), which means it is more stable (Chen et al., 2017).
As the preparation process of vanadium dioxide materials matures, researchers have developed an increasing number of preparation methods. When you mix vanadium dioxide film with other thermochromic materials (Y. Zhou et al., 2015) and make them more stable, you can make the film work better and use the materials in smart windows in a better way. In general, vanadium dioxide films have a wide range of applications in the field of smart windows, but the higher phase transition temperature, lower sunlight energy modulation and visible light transmittance of vanadium dioxide need to be further improved to meet the use standards. Considering the commercial use of smart windows, the large-area preparation process, production cost and service life are all factors that cannot be ignored in the research of vanadium dioxide film in the field of smart windows (Cao et al., 2020; Cui et al., 2018; Zhou et al., 2019).
5.2 Switches of photoelectric and terahertz
Switches are becoming more and more widely used in our lives because vanadium dioxide undergoes a metal-semiconductor phase transition before and after a phase change, resulting in a change in its resistance. Vanadium dioxide film’s phase transformation characteristics make it suitable as a special material in photoelectric switches. Figure 9C is a temperature-resistance curve of vanadium dioxide films (Reese et al., 2004). The photoelectric switch uses the reversible phase change of the vanadium dioxide film, which is higher than the VO2 phase change temperature, and the VO2 film shows a metal state with low resistivity, at which time the current can pass through and the switch is turned on; when the phase change temperature is lower than the VO2 phase change temperature and shows a semiconductor state, the resistivity suddenly increases and makes it difficult for the current to pass through, resulting in the switch being disconnected. Therefore, controlling the temperature change of the vanadium dioxide film realizes the automatic control of the switch. Compared to traditional switches, the ceramic photovoltaic switch prepared from vanadium dioxide film not only works under high current but also has a nanosecond counterresponse time, making it suitable for high-speed photovoltaic switches with a larger application area and longer service life.
VO2 film can also function as terahertz switches, in addition to photoelectric switches. Schalch J. et al. designed a wideband electrically tuned VO2 metamaterial terahertz switch with suppressed reflection. Figure 9D shows the switch structure, which consists of four layers. They are double-layer metamaterials with an anti-reflection structure, a silicon substrate, VO2 and staggered gold electrodes. The double-layer metamaterial structure on top of the device forms high-resistance silicon, and the specific parameters of the whole device are: D1 = 92 μm, D2 = 74 μm, H1 = 30 μm, H2 = 45 μm. The thickness of the silicon substrate is TSi = 410 μm, the thickness of VO2 is TVO2 = 0.2 μm, and the thickness of the gold interleaved electrode is TAu = 0.15 μm. Before the phase change, the film transmits the majority of the terahertz, but after the transition, the terahertz wave experiences low reflection and transmission, resulting in the dissipation of most of the energy as heat (Schalch et al., 2020).
5.3 Temperature sensor
VO2 is a metal oxide; its optical, electrical, magnetic, and thermal properties are variable, so it can be applied to different types of sensors, such as biosensors, pressure sensors, temperature sensors, etc. The function of the sensor is to convert the perceived information into electrical signals or other readable signals, which can be used to detect, process and display the information (Chang et al., 2015).
Kim et al. (2007) used Al2O3 substrate epitaxy to grow VO2 films to prepare critical temperature sensors that can be used for programming. (Kim et al., 2007). The sensor structure can measure the change of conductivity by applying voltage to the vanadium dioxide film through the Ni electrode with ohmic contact at both ends. The detection temperature range of the sensor can be adjusted by applying external voltage regulation, and the bidirectional detection of tensile stress and compressive stress can be realized. In addition, Azharudeen A. M. et al. used an enhanced mesoporous VO2/PVA nanocomposite electrochemical sensor to measure glucose (Mohamed Azharudeen et al., 2019). The measurement results show that the biosensor has high selectivity, high stability and high sensitivity. They compared the results of measuring glucose in blood samples with those of a clinical blood glucose analyzer. The relative error of the two samples was less than 4%.
5.4 Terahertz modulators
The terahertz wave in the excitation spectrum spans the microwave and infrared bands, and it covers a frequency of 0.1∼10 Hz (Rahm et al., 2013). Terahertz waves possess significant technical value and can transmit crucial physical information, which finds applications in wireless communication, non-destructive testing, imaging, spectroscopy, etc. This information requires modulators, absorbers, and filters to manipulate terahertz waves. VO2 film can modulate the terahertz wavefront, allowing for adjustable terahertz transmittance, absorption, and reflectance, under the external stimulus of phase change temperate. In its insulated state, VO2 is almost completely clear to terahertz waves. However, when it changes to its metallic state, the transmittance to terahertz waves changes because the concentration of free carriers changes during the phase transition. This feature provides a large modulation depth and a low 1 L significance requirement for VO2-based terahertz manipulation devices (Shi et al., 2011; Zhao et al., 2012; 2013).
Recently, the combination of VO2 film and metamaterials has become a popular area of research. This is because the VO2 film’s performance in ultrafast phase transition works well with the THz wave resonance properties of metamaterials. Controlling the interaction between terahertz radiation and metamaterials allows for the modulation of the output response using metamaterials. Y. Zhao et al. proposed a THz phase shifter based on VO2 combined capacitance-inductance resonance and dipole resonance, which generates a larger phase shift by dynamically modulating THz waves. The phase shifter structure consists of a ring-dumbbell composite resonator (RDCR) cell that is a mixture of a circular SRR and a vertical dumbbell dipole resonator (DR). The 11 × 10 × 0.284 micron VO2 nanofilm was fabricated on a quartz substrate by photolithography and embedded in the gaps on both sides of the RDRCR (Zhao et al., 2018).
Electrical tuning is one of the most commonly used methods for terahertz modulation. Cai et al. (2018) developed a VO2-based hybrid multifunctional metasurface (Figure 10A) to demonstrate electrically triggered switching and photonic memory effects in the teraherz region using an asymmetric split-ring resonator fabricated by substrate integration (Cai et al., 2018). Simulating the intracellular electric field distribution curves per unit of VO2 insulating state and metallic state at 0.864 THz and 0.63 THz (Figure 10B) demonstrated the electric dipole resonance at two different resonance frequencies during the VO2 phase transition. The goal of the experiments was to look into the terahertz transmission properties of VO2 hybrid metasurfaces with different current characteristics. The results showed that the electrically induced MIT threshold current is 0.4A, and the VO2 asymmetric split-ring resonator uses 225 μW of power per unit cell. VO2-based hybrid components in the terahertz band have excellent switching performance (Figure 10C). The modulation depth is 54% at 0.63 THz and 36% at 0.864 THz, and the electrically triggered modulation speed is about 2 s. In conclusion, combined with the VO2 phase change in thin film properties, it is helpful to achieve high-performance terahertz modulators.
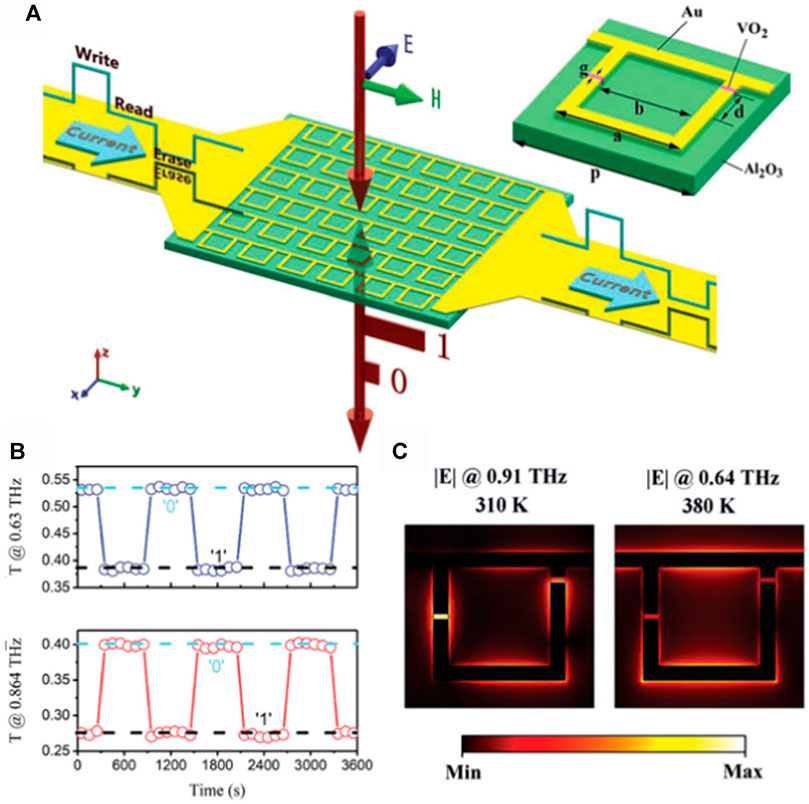
FIGURE 10. Terahertz modulator (Cai et al., 2018): (A) 3D schematic diagram of a VO2-based hybrid metasurface with a terahertz wave incident in the z-direction and its electric field polarized in the x-direction, and an inset of a VO2-based cell of a hybrid metasurface with geometric parameters; (B) The measured amplitude of transmission at 0.864 THz and 0.63 THz versus the time of bias current control; (C) Simulated electric field distribution curves in cell cells with insulated VO2 at 0.91 THz and metal VO2 at 0.64 THz.
5.5 Reconfigurable metasurfaces
Metasurfaces are two-dimensional metamaterials that can flexibly control the intensity, phase, polarization, frequency, angular momentum, and other characteristics of electromagnetic waves through artificially designed subwavelength electromagnetic structures arranged periodically or aperiodically on a plane. Compared to traditional bulk optical devices, optical metasurfaces have the characteristics of being lightweight, compact, and easy to integrate, and have good application potential in fields such as optoelectronic detection, imaging, and sensing. In recent years, research on optical reconfigurable metasurface devices has been carried out to meet the application needs of multi-dimensional detection, all optical modulation, adaptive beam deflection, and zoom lenses, and preliminary prototype verification has been achieved.
In reconfigurable metasurfaces, reconfigurable metasurfaces based on VO2 phase change materials have advantages such as a large tuning range, fast tuning speed, low power consumption, and all-solid state. However, related devices still have problems such as low efficiency and a single control dimension, which restrict their practical applications. Therefore, the development of new VO2-based thin film materials with low loss and a large tuning range and the design and preparation of high-transmittance, multi-dimensionally controllable metasurface structures are important directions for the development of this technology.
Based on VO2 phase change materials, a series of reconfigurable surface plasmon metasurfaces and all dielectric metasurfaces have been used to achieve high efficiency and multi-dimensional control of devices. Future applications can be explored in the following areas: 1) High-value phase change materials have a significant impact on the performance of reconfigurable metasurfaces, but currently there is still a lack of development and optimisation for new optical materials. Therefore, in future work, the phase change optical properties of phase change material VO2 should be further optimised through band modulation, and VO2-based phase change materials with low optical losses before and after phase change should be developed. 2) Complete the point-by-point control design of a flexible reconfigurable metasurface based on VO2 and achieve adaptive beam focusing in experiments; Design infrared camouflage devices with adjustable infrared radiation direction based on flexible device manufacturing methods.
5.6 Infrared imaging
The high temperature coefficient of resistance of VO2 gives it excellent thermal sensitivity performance, which makes vanadium dioxide a high-quality, uncooled infrared focal plane detector array-sensitive material that is used to capture the real-time dynamics of infrared radiation from the detected object (Chen et al., 2007; De Almeida et al., 2004; Gurvitch et al., 2009). Vanadium dioxide has a strong phase transition stress from the monoclinic phase to the rutile phase. This means that there is a hysteresis effect when the phase changes from monoclinic to rutile. Therefore, in practical applications, some means are usually used to inhibit the phase transformation of vanadium dioxide and only retain the high temperature coefficient of resistance (Venkatasubramanian et al., 2009).
Doping is the main means to inhibit phase transition, and heavy doping can destroy the symmetry of vanadium dioxide grains, inhibit its growth, and eliminate the effects of phase transition. Gu et al. (2016) found that heavy doping of silver ions can inhibit the vanadium dioxide phase transition, while the conductivity of vanadium dioxide films is improved and the thermal noise figure is reduced (Gu et al., 2016). A vanadium dioxide film with a room temperature resistivity of 0.24 Ω cm and a temperature coefficient of resistance greater than 5%k was obtained by heavy doping of silver.
Gu Deen et al. prepared VO2 thin films without phase change at a deposition temperature of 200°C by doping with yttrium, a rare earth element. At the same time, the introduction of yttrium reduces the resistivity of the film by two orders of magnitude and retains the temperature coefficient of resistance of 1.5%K (Zhou et al., 2020). The elemental yttrium-doped VO2 film has a unique structure of small crystalline particles spread out in the amorphous region. This makes the material less noisy and more stable.
VO2’s high temperature coefficient of resistance makes it a potential heat-sensitive material, but its application hinders the inherent phase change hysteresis and phase change stress of VO2. Doping inhibition of the VO2 phase transition can obtain VO2 films with a high temperature coefficient of resistance, high stability and low noise. The excellent thermal properties of this film will further improve the detection capability of uncooled focal plane detectors.
The high temperature coefficient of resistance of VO2 films allows them to be used as detectors in the field of infrared imaging, especially for uncooled detectors based on VO2 films. However, the disadvantages of uncooled detectors are lower sensitivity than cooled photon detectors, slow response speed, and high noise, which limit their performance applications. 1) Optimise the film preparation process: Adopt appropriate preparation methods, such as physical vapour deposition (PVD) or chemical vapour deposition (CVD), to obtain high-quality, dense VO2 films and ensure the stability of their lattice structure and phase transition temperature. 2) Film surface treatment: The surface properties of VO2 films are changed by chemical treatment, oxidation, ion implantation and other methods to enhance their absorption capacity and sensitivity to infrared radiation. 3) Optimise electrode design: design a suitable electrode structure to enhance the contact performance between the VO2 film and the electrode and improve the signal response speed and stability. 4) Introduction of nanostructures: through nanostructure manipulation, such as nanoparticle doping, nanowire growth, etc., the interface area of VO2 films can be increased, and the absorption efficiency and photoelectric conversion efficiency of infrared radiation can be improved. 5) Noise control: Reduce the impact of internal and external noise by improving the device structure and electronic design, such as using low-noise preamplifiers and optimising circuit layout. 6) Dynamic response range enhancement: The dynamic response range and sensitivity of VO2 films can be improved by optimising the device structure and detection circuit design, such as by using multi-stage charge amplifiers and adding feedback circuits.
6 Summary and prospect
The development of a suitable VO2 (M/R) films synthesis method is urgently needed for the commercial application exploration of research materials, and a large-scale, low-cost universal method is needed to obtain the VO2 phase. The study of the synthesis of ideal VO2 (M/R) films is an indispensable first step, and the synthesis of single-phase VO2 (M/R) films with more uniform particle size, small particle size, better dispersion, perfect stoichiometry, and better crystallinity is a task to be completed. With the emergence of new light sources, VO2 film research has gradually shifted from thermochromic to electrochromic and photochromic. For example, when VO2 film is used in smart window coating, the research focus is on how to reasonably reduce the phase transition temperature and increase the visible light transmittance of the film. When VO2 film is used as an uncooled infrared detector, the research focus is on the development of large-scale, wide-band, low-cost infrared focal plane arrays, reasonable control of its noise equivalent temperature difference, and improvement of the dynamic response range. In addition, the study of the electrical and thermal properties of VO2 film and its combination with two-dimensional metasurface structures will be important research hotspots in the future.
As a thermochromic material, VO2 film is not only used in the field of smart packaging but also in many fields such as photoelectric switches, smart windows, and the military. Using the principle that VO2 film exhibits a semiconductor structure at low temperatures and a metal structure at high temperatures, the switches in the circuit can be directly controlled by adjusting the temperature to reach the phase change point, and the circuit can be automatically turned on and off (Chen et al., 2018; Markov et al., 2015). In addition, the abrupt change in transmittance of VO2 film in the infrared band before and after phase change can be applied to control the relative on-off of the optical path (Wan et al., 2019). The concept of “smart window” has made VO2 material a new type of energy-saving and environmentally friendly material (Cui et al., 2018). The heat of sunlight is mainly distributed in the infrared band, and VO2 film has a high low-temperature transmittance and a low high-temperature transmittance in the infrared band, but its visible light transmittance hardly changes in the process (Ji et al., 2018d; Xu et al., 2018), Using this optical property, VO2 powders can be prepared into flexible films, coatings, etc. for practical applications. The smart window can automatically adjust the solar intake according to the ambient temperature to achieve the effect of “warm in winter and cool in summer” while saving energy consumption. In addition, VO2 film is considered to be an adaptive thermal camouflage material with a wide range of applications because it transmits and reflects infrared light waves before and after phase transition, which leads to changes in emissivity (Ji et al., 2018e; D; Liu et al., 2016). Adaptive thermal camouflage is a technique capable of creating a chameleon effect to adapt to the surrounding environment (Ji et al., 2018a), This technology can achieve a stealth effect in thermal imaging, and the lower the emissivity, the better the stealth effect. It is currently receiving more and more attention in many military applications.
Based on the description of VO2 film synthesis methods and applications in the article, VO2 film has become a breakthrough material with strong electron-electron correlation, but there are still some problems that need to be solved for the practical application of VO2 film as a smart material. First of all, after the breakthrough of MIT in VO2, the exact mechanism of MIT still needs a thorough explanation, and Mott or Perce assumptions alone are not enough to solve the origin of MIT, and linking the two theories may bring more understanding of MIT’s origins. The stimulus response of VO2 film to light fields, electrostatic fields, terahertz pulses, or stresses may complement the understanding of MIT mechanisms. Second, the exploration of the MIT mechanism is now limited to certain applications, such as smart windows and sensors. The popularity of thermochromic VO2 film smart windows is increasing, but the practical difficulties of VO2 coating need to be addressed, and the balance between Tc, transmittance, and solar modulation capacity can be extended through structural engineering, controlled porosity, and atomic-level doping. The lack of VO2 material equipment limits the application of VO2 and accelerates the research on VO2 film application to meet the needs of the real world. Finally, the way to explore the practical application of VO2 film is still a combination of simulation and experimental research. The coupling of VO2 with other functional materials can effectively change the physicochemical properties, and the composites prepared from this material have potential advantageous properties.
Utilising the phase transformation characteristics of VO2 films from semiconductors to metals requires a variety of ways to achieve this. In terms of temperature control, the phase change temperature of VO2 is usually around 68°C. By controlling the ambient temperature, it is possible to control the phase transition from a semiconductor to a metal, thereby taking advantage of its characteristics. For example, temperature sensors and feedback control systems can be used to precisely control the temperature of VO2 materials so that they achieve phase transitions within a specific temperature range. Achieved using light control, VO2 materials are highly sensitive to light, especially in the near-infrared spectral range. By using lighting, the phase transition behaviour of VO2 can be manipulated. For example, a phase transition from a semiconductor to a metal can be achieved by using an optical control switch or an optical modulator to control the phase transition state of a VO2 material. In terms of electric field control, the electric field can also affect the phase transformation behaviour of VO2 materials. By applying an appropriate electric field, the electronic structure of the VO2 material can be changed, resulting in a phase change. For example, an applied electrode and a voltage source can be used to apply an electric field to control the phase transformation properties of VO2 materials. In terms of strain control, strain can also have an impact on the phase transformation behaviour of VO2 materials. By applying the appropriate strain, the lattice structure of the VO2 material can be changed, resulting in a phase transition. For example, a mechanical device or piezoelectric material can be used to apply strain to control the phase transformation behaviour of a VO2 material.
As a result, temperature control, optical control, electric field control, and strain control can all effectively use the semiconductor-to-metal phase transformation characteristics of VO2. These methods can be selected and combined according to the needs of the specific application to achieve precise control of the phase transformation behaviour of VO2 materials.
In short, compared with traditional functional crystals (KDP, RTAP, LN, and liquid crystal), VO2 film has the characteristics of reversible changes in optical, thermal, and electrical properties, and its multi-parameter composite control and novel functional applications need to be further developed, and the goal of multi-functional integration of embedded systems needs to be realized. In view of its practical value in the field of military industry, the application of VO2 film in infrared camouflage, multicolor electrochromic, and other fields will also be a research hotspot in the future.
Author contributions
CW: Conceptualization, Investigation, Methodology, Writing–original draft. LF: Conceptualization, Investigation, Methodology, Writing–original draft. ZL: Conceptualization, Investigation, Methodology, Writing–original draft. JB: Methodology, Writing–original draft. SW: Supervision, Validation, Writing–original draft. XG: Supervision, Validation, Writing–original draft. JW: Supervision, Validation, Writing–review and editing. WY: Supervision, Validation, Writing–review and editing.
Funding
The author(s) declare that no financial support was received for the research, authorship, and/or publication of this article.
Acknowledgments
The authors gratefully acknowledge the financial support of National Natural Science Foundation of China (Grant Nos 21806065, 21777080, and 22078142), Natural Science Foundation of Liaoning (Grant No. 2019-MS-172), Young Talents Foundation of University of Science and Technology Liaoning (Grant No. 601011507-24), the Scientific Research Foundation of the Educational Department of Liaoning Province (Grant No. 6006022021).
Conflict of interest
The authors declare that the research was conducted in the absence of any commercial or financial relationships that could be construed as a potential conflict of interest.
Publisher’s note
All claims expressed in this article are solely those of the authors and do not necessarily represent those of their affiliated organizations, or those of the publisher, the editors and the reviewers. Any product that may be evaluated in this article, or claim that may be made by its manufacturer, is not guaranteed or endorsed by the publisher.
References
Adler, D. (1968). Mechanisms for metal-nonmental transitions in transition-metal oxides and sulfides. Rev. Mod. Phys. 40 (4), 714–736. doi:10.1103/revmodphys.40.714
Babulanam, S. M., Eriksson, T. S., Niklasson, G. A., and Granqvist, C. G. (1987). Thermochromic VO2 films for energy-efficient windows. Sol. energy Mater. 16 (5), 347–363. doi:10.1016/0165-1633(87)90029-3
Batista, C., Ribeiro, R. M., and Teixeira, V. (2011). Synthesis and characterization of VO2-based thermochromic thin films for energy-efficient windows. Nanoscale Res. Lett. 6 (1), 301. doi:10.1186/1556-276X-6-301
Bae, S.-H., Lee, S., Koo, H., Lin, L., Jo, B. H., Park, C., et al. (2013). The Memristive Properties of a Single VO2 Nanowire with Switching Controlled by Self-Heating. Advanced Materials 25 (36), 5098–5103. doi:10.1002/adma.201302511
Berglund, C. N., and Guggenheim, H. J. (1969). Electronic properties of VO2 near the semiconductor-metal transition. Phys. Rev. 185 (3), 1022–1033. doi:10.1103/physrev.185.1022
Béteille, F., Mazerolles, L., and Livage, J. (1999). Microstructure and metal-insulating transition of VO2 thin films. Mater. Res. Bull. 34 (14-15), 2177–2184. doi:10.1016/s0025-5408(99)00232-9
Bian, J., Wang, M., Sun, H., Liu, H., Li, X., Luo, Y., et al. (2016). Thickness-modulated metal–insulator transition of VO2 film grown on sapphire substrate by MBE. J. Mater. Sci. 51 (13), 6149–6155. doi:10.1007/s10853-016-9863-1
Binions, R., Hyett, G., Piccirillo, C., and Parkin, I. P. (2007a). Doped and un-doped vanadium dioxide thin films prepared by atmospheric pressure chemical vapour deposition from vanadyl acetylacetonate and tungsten hexachloride: the effects of thickness and crystallographic orientation on thermochromic properties. J. Mater. Chem. 17 (44), 4652. doi:10.1039/b708856f
Binions, R., Piccirillo, C., and Parkin, I. P. (2007b). Tungsten doped vanadium dioxide thin films prepared by atmospheric pressure chemical vapour deposition from vanadyl acetylacetonate and tungsten hexachloride. Surf. Coatings Technol. 201 (22–23), 9369–9372. doi:10.1016/j.surfcoat.2007.03.026
Borek, M., Qian, F., Nagabushnam, V., and Singh, R. K. (1993). Pulsed laser deposition of oriented VO2 thin films on R-cut sapphire substrates. Appl. Phys. Lett. 63 (24), 3288–3290. doi:10.1063/1.110177
Budai, J. D., Hong, J., Manley, M. E., Specht, E. D., Li, C. W., Tischler, J. Z., et al. (2014). Metallization of vanadium dioxide driven by large phonon entropy. Nature 515 (7528), 535–539. doi:10.1038/nature13865
Bukhari, S. A., Kumar, S., Kumar, P., Gumfekar, S. P., Chung, H.-J., Thundat, T., et al. (2020). The effect of oxygen flow rate on metal–insulator transition (MIT) characteristics of vanadium dioxide (VO2) thin films by pulsed laser deposition (PLD). Appl. Surf. Sci. 529, 146995. doi:10.1016/j.apsusc.2020.146995
Burkhardt, W., Christmann, T., Franke, S., Kriegseis, W., Meister, D., Meyer, B. K., et al. (2002). Tungsten and fluorine co-doping of VO2 films. Thin Solid Films 402 (1–2), 226–231. doi:10.1016/s0040-6090(01)01603-0
Cai, H., Chen, S., Zou, C., Huang, Q., Liu, Y., Hu, X., et al. (2018). Multifunctional hybrid metasurfaces for dynamic tuning of terahertz waves. Adv. Opt. Mater. 6 (14), 1800257. doi:10.1002/adom.201800257
Cao, J., Ertekin, E., Srinivasan, V., Fan, W., Huang, S., Zheng, H., et al. (2009). Strain engineering and one-dimensional organization of metal–insulator domains in single-crystal vanadium dioxide beams. Nat. Nanotechnol. 4 (11), 732–737. doi:10.1038/nnano.2009.266
Cao, X., Chang, T., Shao, Z., Xu, F., Luo, H., and Jin, P. (2020). Challenges and opportunities toward real application of VO2-based smart glazing. Matter 2 (4), 862–881. doi:10.1016/j.matt.2020.02.009
Cao, X., Wang, N., Law, J. Y., Loo, S. C. J., Magdassi, S., and Long, Y. (2014b). Nanoporous thermochromic VO2 (M) thin films: controlled porosity, largely enhanced luminous transmittance and solar modulating ability. Langmuir 30 (6), 1710–1715. doi:10.1021/la404666n
Cao, X., Wang, N., Magdassi, S., Mandler, D., and Long, Y. (2014a). Europium doped vanadium dioxide material: reduced phase transition temperature, enhanced luminous transmittance and solar modulation. Sci. Adv. Mater. 6 (3), 558–561. doi:10.1166/sam.2014.1777
Cao, Z., Lu, Y., Xiao, X., Zhan, Y., Cheng, H., and Xu, G. (2017). Tunable simultaneously visible-light and near-infrared transmittance for VO2/SiO2 composite films to enhance thermochromic properties. Mater. Lett. 209, 609–612. doi:10.1016/j.matlet.2017.08.113
Cavalleri, A., Dekorsy, Th., Chong, H. H. W., Kieffer, J. C., and Schoenlein, R. W. (2004). Evidence for a structurally-driven insulator-to-metal transition in VO2: a view from the ultrafast timescale. Phys. Rev. B 70 (16), 161102. doi:10.1103/physrevb.70.161102
Cavalleri, A., Tóth, Cs., Siders, C. W., Squier, J. A., Ráksi, F., Forget, P., et al. (2001). Femtosecond structural dynamics in VO2 during an ultrafast solid-solid phase transition. Phys. Rev. Lett. 87 (23), 237401. doi:10.1103/physrevlett.87.237401
Chae, B. G., Kim, H. T., and Yun, S. J. (2008). Characteristics of W- and Ti-doped VO2 thin films prepared by sol-gel method. Electrochem. Solid-State Lett. 11 (6), D53. doi:10.1149/1.2903208
Chae, B.-G., Kim, H.-T., Yun, S.-J., Kim, B.-J., Lee, Y.-W., Youn, D.-H., et al. (2006). Highly oriented VO2 thin films prepared by sol-gel deposition. Electrochem. Solid-State Lett. 9 (1), C12. doi:10.1149/1.2135430
Chang, C. J., Gunnlaugsson, T., and James, T. D. (2015). Sensor targets. Chem. Soc. Rev. 44 (13), 4176–4178. doi:10.1039/C5CS90058A
Chang, T., Cao, X., Dedon, L. R., Long, S., Huang, A., Shao, Z., et al. (2018). Optical design and stability study for ultrahigh-performance and long-lived vanadium dioxide-based thermochromic coatings. Nano Energy 44, 256–264. doi:10.1016/j.nanoen.2017.11.061
Chen, F. H., Fan, L. L., Chen, S., Liao, G. M., Chen, Y. L., Wu, P., et al. (2015a). Control of the metal–insulator transition in VO2 epitaxial film by modifying carrier density. ACS Appl. Mater. Interfaces 7 (12), 6875–6881. doi:10.1021/acsami.5b00540
Chen, H.-K., Hung, H.-C., Yang, T. C.-K., and Wang, S.-F. (2004). The preparation and characterization of transparent nano-sized thermochromic VO2–SiO2 films from the sol–gel process. J. non-crystalline solids 347 (1-3), 138–143. doi:10.1016/j.jnoncrysol.2004.07.065
Chen, L., Cui, Y., Shi, S., Luo, H., and Gao, Y. (2018). Exploring the work function variability and structural stability of VO2(1 1 0) surface upon noble metal (Ag, Au, Pt) adsorption and incorporation. Appl. Surf. Sci. 450, 318–327. doi:10.1016/j.apsusc.2018.04.197
Chen, R., Miao, L., Cheng, H., Nishibori, E., Liu, C., Asaka, T., et al. (2015b). One-step hydrothermal synthesis of V1−xWxO2 (M/R) nanorods with superior doping efficiency and thermochromic properties. J. Mater. Chem. A 3 (7), 3726–3738. doi:10.1039/C4TA05559D
Chen, S., Dai, L., Liu, J., Gao, Y., Liu, X., Chen, Z., et al. (2013). The visible transmittance and solar modulation ability of VO2 flexible foils simultaneously improved by Ti doping: an optimization and first principle study. Phys. Chem. Chem. Phys. 15 (40), 17537. doi:10.1039/c3cp52009a
Chen, S., Ma, H., Dai, J., and Yi, X. (2007). Nanostructured vanadium dioxide thin films with low phase transition temperature. Appl. Phys. Lett. 90 (10), 101117. doi:10.1063/1.2712427
Chen, S., Wang, Z., Ren, H., Chen, Y., Yan, W., Wang, C., et al. (2019). Gate-controlled VO2 phase transition for high-performance smart windows. Sci. Adv. 5 (3), eaav6815. doi:10.1126/sciadv.aav6815
Chen, S.-E., Lu, H.-H., Brahma, S., and Huang, J.-L. (2017a). Effects of annealing on thermochromic properties of W-doped vanadium dioxide thin films deposited by electron beam evaporation. Thin Solid Films 644, 52–56. doi:10.1016/j.tsf.2017.05.052
Chen, Y., Zeng, X., Zhu, J., Li, R., Yao, H., Cao, X., et al. (2017b). High performance and enhanced durability of thermochromic films using VO2@ZnO core–shell nanoparticles. ACS Appl. Mater. Interfaces 9 (33), 27784–27791. doi:10.1021/acsami.7b08889
Cole, D., Jordan, R., Lunney, J. G., and Coey, J. M. D. (1997). Pulsed laser deposition of Fe thin films in UHV. J. Magnetism Magnetic Mater. 165 (1–3), 246–249. doi:10.1016/s0304-8853(96)00640-3
Cui, Y., Ke, Y., Liu, C., Chen, Z., Wang, N., Zhang, L., et al. (2018). Thermochromic VO2 for energy-efficient smart windows. Joule 2 (9), 1707–1746. doi:10.1016/j.joule.2018.06.018
Dai, L., Chen, S., Liu, J., Gao, Y., Zhou, J., Chen, Z., et al. (2013). F-doped VO2 nanoparticles for thermochromic energy-saving foils with modified color and enhanced solar-heat shielding ability. Phys. Chem. Chem. Phys. 15 (28), 11723. doi:10.1039/c3cp51359a
Danxia, L., Li, W., Huang, L., Song, Q., and Shi, L. (2015). Thermal stability of VO2 thin films deposited by sol–gel method. J. Sol-Gel Sci. Technol. 75 (1), 189–197. doi:10.1007/s10971-015-3688-2
De Almeida, L. A. L., Deep, G. S., Lima, A. M. N., Khrebtov, I. A., Malyarov, V. G., and Neff, H. (2004). Modeling and performance of vanadium–oxide transition edge microbolometers. Appl. Phys. Lett. 85 (16), 3605–3607. doi:10.1063/1.1808890
Dietrich, M. K., Kuhl, F., Polity, A., and Klar, P. J. (2017). Optimizing thermochromic VO2 by co-doping with W and Sr for smart window applications. Appl. Phys. Lett. 110 (14), 141907. doi:10.1063/1.4979700
Dou, S., Zhang, W., Ren, F., Gu, J., Wei, H., Chen, X., et al. (2021). The infrared optical performance of VO2 film prepared by HiPIMS. Mater. Chem. Phys. 259, 124042. doi:10.1016/j.matchemphys.2020.124042
Eyert, V. (2002). The metal-insulator transitions of VO2: a band theoretical approach. Ann. Phys. 514 (9), 650–704. doi:10.1002/1521-3889(200210)11:9<650::aid-andp650>3.0.co;2-k
Fallah Vostakola, M., Mirkazemi, S. M., and Eftekhari Yekta, B. (2019). Structural, morphological, and optical properties of W-doped VO2 thin films prepared by sol-gel spin coating method. Int. J. Appl. Ceram. Technol. 16 (3), 943–950. doi:10.1111/ijac.13170
Fu, D., Liu, K., Tao, T., Lo, K., Cheng, C., Liu, B., et al. (2013). Comprehensive study of the metal-insulator transition in pulsed laser deposited epitaxial VO2 thin films. J. Appl. Phys. 113 (4), 043707. doi:10.1063/1.4788804
Gao, Y., Wang, S., Luo, H., Dai, L., Cao, C., Liu, Y., et al. (2012). Enhanced chemical stability of VO2 nanoparticles by the formation of SiO 2/VO 2 core/shell structures and the application to transparent and flexible VO 2-based composite foils with excellent thermochromic properties for solar heat control. Energy & Environ. Sci. 5 (3), 6104–6110. doi:10.1039/c2ee02803d
Geng, C., Dou, S., Zhao, J., Ren, F., Gu, J., Wei, H., et al. (2022). Self-templated method to fabricate VO2 nanoparticles with ultrahigh luminous transmittance for energy-efficient thermochromic windows. Appl. Surf. Sci. 592, 153267. doi:10.1016/j.apsusc.2022.153267
Gu, D., Xin, Z., Wang, Z., Tan, P., and Jiang, Y. (2016). “Effect of the introduction of Ag on the microstructures and electrical properties of polycrystalline VO2 thin films,” in 8th International Symposium on Advanced Optical Manufacturing and Testing Technologies: Optoelectronic Materials and Devices (SPIE), 121–127.9686.
Guo, H., Wang, Y. G., Jain, A., Fu, H. R., and Chen, F. G. (2021). Preparation of W/Zr co-doped VO2 with improved microstructural and thermochromic properties. J. Alloys Compd. 878, 160352. doi:10.1016/j.jallcom.2021.160352
Gurvitch, M., Luryi, S., Polyakov, A., and Shabalov, A. (2009). Nonhysteretic behavior inside the hysteresis loop of VO2 and its possible application in infrared imaging. J. Appl. Phys. 106 (10), 104504. doi:10.1063/1.3243286
Hao, Q., Li, W., Xu, H., Wang, J., Yin, Y., Wang, H., et al. (2018). VO2/TiN plasmonic thermochromic smart coatings for room-temperature applications. Adv. Mater. 30 (10), 1705421. doi:10.1002/adma.201705421
Hu, L., Tao, H., Chen, G., Pan, R., Wan, M., Xiong, D., et al. (2016). Porous W-doped VO2 films with simultaneously enhanced visible transparency and thermochromic properties. J. Sol-Gel Sci. Technol. 77 (1), 85–93. doi:10.1007/s10971-015-3832-z
Ji, C., Wu, Z., Lu, L., Wu, X., Wang, J., Liu, X., et al. (2018a). High thermochromic performance of Fe/Mg co-doped VO2 thin films for smart window applications. J. Mater. Chem. C 6 (24), 6502–6509. doi:10.1039/c8tc01111g
Ji, C., Wu, Z., Wu, X., Wang, J., Gou, J., Huang, Z., et al. (2018b). Al-doped VO2 films as smart window coatings: reduced phase transition temperature and improved thermochromic performance. Sol. Energy Mater. Sol. Cells 176, 174–180. doi:10.1016/j.solmat.2017.11.026
Ji, H., Liu, D., Cheng, H., and Tao, Y. (2019). Large area infrared thermochromic VO2 nanoparticle films prepared by inkjet printing technology. Sol. Energy Mater. Sol. Cells 194, 235–243. doi:10.1016/j.solmat.2019.02.028
Ji, H., Liu, D., Cheng, H., and Zhang, C. (2018c). Inkjet printing of vanadium dioxide nanoparticles for smart windows. J. Mater. Chem. C 6 (10), 2424–2429. doi:10.1039/c8tc00286j
Ji, H., Liu, D., Cheng, H., Zhang, C., and Yang, L. (2018d). Vanadium dioxide nanopowders with tunable emissivity for adaptive infrared camouflage in both thermal atmospheric windows. Sol. Energy Mater. Sol. Cells 175, 96–101. doi:10.1016/j.solmat.2017.10.013
Ji, H., Liu, D., Zhang, C., and Cheng, H. (2018e). VO2/ZnS core-shell nanoparticle for the adaptive infrared camouflage application with modified color and enhanced oxidation resistance. Sol. Energy Mater. Sol. Cells 176, 1–8. doi:10.1016/j.solmat.2017.11.037
Jiang, M., Cao, X., Bao, S., Zhou, H., and Jin, P. (2014). Regulation of the phase transition temperature of VO2 thin films deposited by reactive magnetron sputtering without doping. Thin Solid Films 562, 314–318. doi:10.1016/j.tsf.2014.04.090
Jorgenson, G., and Lee, J. (1986). Doped vanadium oxide for optical switching films. Sol. Energy Mater. 14 (3-5), 205–214. doi:10.1016/0165-1633(86)90047-x
Kim, B.-J., Lee, Y. W., Chae, B.-G., Yun, S. J., Oh, S.-Y., Lim, Y.-S., et al. (2007). Temperature dependence of Mott transition in VO2 and programmable critical temperature sensor. Appl. Phys. Lett. 90 (2), 023515. doi:10.1063/1.2431456
Kim, H. T., Chae, B. G., Youn, D. H., Maeng, S. L., Kim, G., Kang, K. Y., et al. (2004). Mechanism and observation of Mott transition in VO2-based two-and three-terminal devices. New J. Phys. 6 (1), 52. doi:10.1088/1367-2630/6/1/052
Kucharczyk, D., and Niklewski, T. (1979). Accurate X-ray determination of the lattice parameters and the thermal expansion coefficients of VO2 near the transition temperature. J. Appl. Crystallogr. 12 (4), 370–373. doi:10.1107/s0021889879012711
Laaksonen, K., Li, S.-Y., Puisto, S. R., Rostedt, N. K. J., Ala-Nissila, T., Granqvist, C. G., et al. (2014). Nanoparticles of TiO2 and VO2 in dielectric media: conditions for low optical scattering, and comparison between effective medium and four-flux theories. Sol. Energy Mater. Sol. Cells 130, 132–137. doi:10.1016/j.solmat.2014.06.036
Lee, S., Hippalgaonkar, K., Yang, F., Hong, J., Ko, C., Suh, J., et al. (2017). Anomalously low electronic thermal conductivity in metallic vanadium dioxide. Science 355 (6323), 371–374. doi:10.1126/science.aag0410
Li, B., Tian, S., Tao, H., and Zhao, X. (2019). Tungsten doped M-phase VO2 mesoporous nanocrystals with enhanced comprehensive thermochromic properties for smart windows. Ceram. Int. 45 (4), 4342–4350. doi:10.1016/j.ceramint.2018.11.109
Li, C.-I., Lin, J.-C., Liu, H.-J., Chu, M.-W., Chen, H.-W., Ma, C.-H., et al. (2016). Van der Waal epitaxy of flexible and transparent VO2 film on muscovite. Chem. Mater. 28 (11), 3914–3919. doi:10.1021/acs.chemmater.6b01180
Li, D., Huang, W., Song, L., and Shi, Q. (2015). Thermal stability of VO2 thin films deposited by sol–gel method. J. Sol-Gel Sci. Technol. 75 (1), 189–197. doi:10.1007/s10971-015-3688-2
Li, J., and Dho, J. (2011). Anomalous optical switching and thermal hysteresis behaviors of VO2 films on glass substrate. Appl. Phys. Lett. 99 (23), 231909. doi:10.1063/1.3668089
Li, M., Magdassi, S., Gao, Y., and Long, Y. (2017). Hydrothermal synthesis of VO2 polymorphs: advantages, challenges and prospects for the application of energy efficient smart windows. Small 13 (36), 1701147. doi:10.1002/smll.201701147
Li, S., Li, Y., Jiang, M., Ji, S., Luo, H., Gao, Y., et al. (2013a). Preparation and characterization of self-supporting thermochromic films composed of VO2 (M)@ SiO2 nanofibers. ACS Appl. Mater. interfaces 5 (14), 6453–6457. doi:10.1021/am401839d
Li, Y., Ji, S., Gao, Y., Luo, H., and Kanehira, M. (2013b). Core-shell VO2@TiO2 nanorods that combine thermochromic and photocatalytic properties for application as energy-saving smart coatings. Sci. Rep. 3 (1), 1370. doi:10.1038/srep01370
Liu, C., Wang, N., and Long, Y. (2013). Multifunctional overcoats on vanadium dioxide thermochromic thin films with enhanced luminous transmission and solar modulation, hydrophobicity and anti-oxidation. Appl. Surf. Sci. 283, 222–226. doi:10.1016/j.apsusc.2013.06.085
Liu, D., Cheng, H., Xing, X., Zhang, C., and Zheng, W. (2016). Thermochromic properties of W-doped VO2 thin films deposited by aqueous sol-gel method for adaptive infrared stealth application. Infrared Phys. Technol. 77, 339–343. doi:10.1016/j.infrared.2016.06.019
Long, S., Cao, X., Sun, G., Li, N., Chang, T., Shao, Z., et al. (2018). Effects of V2O3 buffer layers on sputtered VO2 smart windows: improved thermochromic properties, tunable width of hysteresis loops and enhanced durability. Appl. Surf. Sci. 441, 764–772. doi:10.1016/j.apsusc.2018.02.083
Long, S., Zhou, H., Bao, S., Xin, Y., Cao, X., and Jin, P. (2016). Thermochromic multilayer films of WO3/VO2/WO3 sandwich structure with enhanced luminous transmittance and durability. RSC Adv. 6 (108), 106435–106442. doi:10.1039/c6ra23504b
Lu, Q., Liu, C., Wang, N., Magdassi, S., Mandler, D., and Long, Y. (2016). Periodic micro-patterned VO2 thermochromic films by mesh printing. J. Mater. Chem. C 4 (36), 8385–8391. doi:10.1039/C6TC02694J
Lu, S., Hou, L., and Gan, F. (1997). Structure and optical property changes of sol-gel derived VO2 thin films. Adv. Mater. 9 (3), 244–246. doi:10.1002/adma.19970090313
Luo, F. Y., and Huang, W. G. (2010). “Effects of sputtering power on structure and properties of VO2 thin films prepared by magnetron sputtering,” in Proceedings of the 7th National Conference on Functional Materials and Applications (Changsha, China: China Institute of Instrumentation, China Society of Metals), 1208–1211.
Lysenko, S., Rua, A., Vikhnin, V., Jimenez, J., Fernandez, F., and Liu, H. (2006). Light-induced ultrafast phase transitions in VO2 thin film. Appl. Surf. Sci. 252 (15), 5512–5515. doi:10.1016/j.apsusc.2005.12.137
Manning, T. D., Parkin, I. P., Blackman, C., and Qureshi, U. (2005). APCVD of thermochromic vanadium dioxide thin films—solid solutions V2−xMxO2 (M= Mo, Nb) or composites VO2: SnO2. J. Mater. Chem. 15 (42), 4560–4566. doi:10.1039/b510552h
Manning, T. D., Parkin, I. P., Pemble, M. E., Sheel, D., and Vernardou, D. (2004). Intelligent window coatings: atmospheric pressure chemical vapor deposition of tungsten-doped vanadium dioxide. Chem. Mater. 16 (4), 744–749. doi:10.1002/chin.200418012
Markov, P., Marvel, R. E., Conley, H. J., Miller, K. J., Haglund, R. F., and Weiss, S. M. (2015). Optically monitored electrical switching in VO2. ACS Photonics 2 (8), 1175–1182. doi:10.1021/acsphotonics.5b00244
Miller, M. J., and Wang, J. (2016). Multilayer ITO/VO2/TiO2 thin films for control of solar and thermal spectra. Sol. Energy Mater. Sol. Cells 154, 88–93. doi:10.1016/j.solmat.2016.04.044
Miyano, K., Tanaka, T., Tomioka, Y., and Tokura, Y. (1997). Photoinduced insulator-to-metal transition in a perovskite manganite. Phys. Rev. Lett. 78 (22), 4257–4260. doi:10.1103/PhysRevLett.78.4257
Mlyuka, N. R., Niklasson, G. A., and Granqvist, C. G. (2009). Thermochromic multilayer films of VO2 and TiO2 with enhanced transmittance. Sol. Energy Mater. Sol. Cells 93 (9), 1685–1687. doi:10.1016/j.solmat.2009.03.021
Mohamed Azharudeen, A., Karthiga, R., Rajarajan, M., and Suganthi, A. (2019). Enhancement of electrochemical sensor for the determination of glucose based on mesoporous VO2/PVA nanocomposites. Surfaces Interfaces 16, 164–173. doi:10.1016/j.surfin.2019.05.005
Morin, F. J. (1959). Oxides which show a metal-to-insulator transition at the neel temperature. Phys. Rev. Lett. 3 (1), 34–36. doi:10.1103/physrevlett.3.34
Mott, N. F. (1949). The basis of the electron theory of metals, with special reference to the transition metals. Proc. Phys. Soc. Sect. A 62 (7), 416–422. doi:10.1088/0370-1298/62/7/303
Novodvorsky, O. A., Parshina, L. S., Khramova, O. D., Mikhalevsky, V. A., Shcherbachev, K. D., and Panchenko, V.Ya. (2015). Influence of the conditions of pulsed laser deposition on the structural, electrical, and optical properties of VO2 thin films. Semiconductors 49 (5), 563–569. doi:10.1134/S1063782615050188
Paquet, D., and Leroux-Hugon, P. (1980). Electron correlations and electron-lattice interactions in the metal-insulator, ferroelastic transition in VO2: a thermodynamical study. Phys. Rev. B 22 (11), 5284–5301. doi:10.1103/PhysRevB.22.5284
Piccirillo, C., Binions, R., and Parkin, I. P. (2007). Synthesis and functional properties of vanadium oxides: V2O3, VO2, and V2O5 deposited on glass by aerosol-assisted CVD. Chem. Vap. Depos. 13 (4), 145–151. doi:10.1002/cvde.200606540
Powell, M. J., Quesada-Cabrera, R., Taylor, A., Teixeira, D., Papakonstantinou, I., Palgrave, R. G., et al. (2016). Intelligent multifunctional VO2/SiO2/TiO2 coatings for self-cleaning, energy-saving window panels. Chem. Mater. 28 (5), 1369–1376. doi:10.1021/acs.chemmater.5b04419
Qazilbash, M. M., Brehm, M., Chae, B.-G., Ho, P.-C., Andreev, G. O., Kim, B.-J., et al. (2007). Mott transition in VO2 revealed by infrared spectroscopy and nano-imaging. Science 318 (5857), 1750–1753. doi:10.1126/science.1150124
Qian, X., Wang, N., Li, Y., Zhang, J., Xu, Z., and Long, Y. (2014). Bioinspired multifunctional vanadium dioxide: improved thermochromism and hydrophobicity. Langmuir 30 (35), 10766–10771. doi:10.1021/la502787q
Rahm, M., Li, J.-S., and Padilla, W. J. (2013). THz wave modulators: a brief review on different modulation techniques. J. Infrared, Millim. Terahertz Waves 34 (1), 1–27. doi:10.1007/s10762-012-9946-2
Reese, C. E., Mikhonin, A. V., Kamenjicki, M., Tikhonov, A., and Asher, S. A. (2004). Nanogel nanosecond photonic crystal optical switching. J. Am. Chem. Soc. 126 (5), 1493–1496. doi:10.1021/ja037118a
Roach, W. R., and Balberg, I. (1971). Optical induction and detection of fast phase transition in VO2. Solid State Commun. 9 (9), 551–555. doi:10.1016/0038-1098(71)90144-x
Sahana, M. B., Dharmaprakash, M. S., and Shivashankar, S. A. (2002). Microstructure and properties of VO2 thin films deposited by MOCVD from vanadyl acetylacetonate. J. Mater. Chem. 12 (2), 333–338. doi:10.1039/b106563g
Sameie, H., Alvani, A. S., Naseri, N., Du, S., and Rosei, F. J. C. I. (2018). First-principles study on ZnV2O6 and Zn2V2O7: two new photoanode candidates for photoelectrochemical water oxidation. Ceram. Int. 44 (6), 6607–6613. doi:10.1016/j.ceramint.2018.01.064
Schalch, J. S., Chi, Y., He, Y., Tang, Y., Zhao, X., Zhang, X., et al. (2020). Broadband electrically tunable VO2-Metamaterial terahertz switch with suppressed reflection. Microw. Opt. Technol. Lett. 62 (8), 2782–2790. doi:10.1002/mop.32354
Shen, N., Chen, S., Chen, Z., Liu, X., Cao, C., Dong, B., et al. (2014). The synthesis and performance of Zr-doped and W–Zr-codoped VO2 nanoparticles and derived flexible foils. J. Mat. Chem. A 2 (36), 15087–15093. doi:10.1039/c4ta02880e
Shen, N., Chen, S., Huang, R., Huang, J., Li, J., Shi, R., et al. (2021). Vanadium dioxide for thermochromic smart windows in ambient conditions. Mater. Today Energy 21, 100827. doi:10.1016/j.mtener.2021.100827
Shi, Q., Huang, W., Zhang, Y., Yan, J., Zhang, Y., Mao, M., et al. (2011). Giant phase transition properties at terahertz range in VO2 films deposited by sol–gel method. ACS Appl. Mater. Interfaces 3 (9), 3523–3527. doi:10.1021/am200734k
Soltani, M., Chaker, M., Haddad, E., Kruzelecky, R. V., and Margot, J. (2004). Effects of Ti–W codoping on the optical and electrical switching of vanadium dioxide thin films grown by a reactive pulsed laser deposition. Appl. Phys. Lett. 85 (11), 1958–1960. doi:10.1063/1.1788883
Stefanovich, G., Pergament, A., and Stefanovich, D. (2000). Electrical switching and Mott transition in VO2. J. Phys. Condens. Matter 12 (41), 8837–8845. doi:10.1088/0953-8984/12/41/310
Sun, G., Cao, X., Gao, X., Long, S., Liang, M., and Jin, P. (2016). Structure and enhanced thermochromic performance of low-temperature fabricated VO2/V2O3 thin film. Appl. Phys. Lett. 109 (14), 143903. doi:10.1063/1.4964432
Taylor, A., Parkin, I., Noor, N., Tummeltshammer, C., Brown, M. S., and Papakonstantinou, I. (2013). A bioinspired solution for spectrally selective thermochromic VO2 coated intelligent glazing. Opt. Express 21 (S5), A750. doi:10.1364/oe.21.00a750
Théry, V., Boulle, A., Crunteanu, A., Orlianges, J. C., Beaumont, A., Mayet, R., et al. (2016). Role of thermal strain in the metal-insulator and structural phase transition of epitaxial VO2 films. Phys. Rev. B 93 (18), 184106. doi:10.1103/PhysRevB.93.184106
Tokura, Y. (2003). Correlated-electron physics in transition-metal oxides. Phys. Today 56 (7), 50–55. doi:10.1063/1.1603080
Venkatasubramanian, C., Cabarcos, O. M., Allara, D. L., Horn, M. W., and Ashok, S. (2009). Correlation of temperature response and structure of annealed VOx thin films for IR detector applications. J. Vac. Sci. Technol. A Vac. Surfaces, Films 27 (4), 956–961. doi:10.1116/1.3143667
Vernardou, D., Paterakis, P., Drosos, H., Spanakis, E., Povey, I. M., Pemble, M. E., et al. (2011). A study of the electrochemical performance of vanadium oxide thin films grown by atmospheric pressure chemical vapour deposition. Sol. Energy Mater. Sol. Cells 95 (10), 2842–2847. doi:10.1016/j.solmat.2011.05.046
Vernardou, D., Pemble, M. E., and Sheel, D. W. (2006). The growth of thermochromic VO2 films on glass by atmospheric-pressure CVD: a comparative study of precursors, CVD methodology, and substrates. Chem. Vap. Depos. 12 (5), 263–274. doi:10.1002/cvde.200506419
Wan, C., Zhang, Z., Woolf, D., Hessel, C. M., Rensberg, J., Hensley, J. M., et al. (2019). On the optical properties of thin-film vanadium dioxide from the visible to the far infrared. Ann. Der Phys. 531 (10), 1900188. doi:10.1002/andp.201900188
Wang, M., Tian, J., Zhang, H., Shi, X., Chen, Z., Wang, Y., et al. (2016a). Novel synthesis of pure VO2@SiO2 core@shell nanoparticles to improve the optical and anti-oxidant properties of a VO2 film. RSC Adv. 6 (110), 108286–108289. doi:10.1039/c6ra20636k
Wang, N., Duchamp, M., Dunin-Borkowski, R. E., Liu, S., Zeng, X., Cao, X., et al. (2016b). Terbium-doped VO2 thin films: reduced phase transition temperature and largely enhanced luminous transmittance. Langmuir 32 (3), 759–764. doi:10.1021/acs.langmuir.5b04212
Wang, N., Liu, S., Zeng, X. T., Magdassi, S., and Long, Y. (2015a). Mg/W-codoped vanadium dioxide thin films with enhanced visible transmittance and low phase transition temperature. J. Mater. Chem. C 3 (26), 6771–6777. doi:10.1039/c5tc01062d
Wang, N., Liu, S., Zeng, X. T., Magdassi, S., and Long, Y. (2015b). Mg/W-codoped vanadium dioxide thin films with enhanced visible transmittance and low phase transition temperature. J. Mater. Chem. C 3 (26), 6771–6777. doi:10.1039/c5tc01062d
Wu, C., Feng, F., and Xie, Y. (2013a). Design of vanadium oxide structures with controllable electrical properties for energy applications. Chem. Soc. Rev. 42 (12), 5157. doi:10.1039/c3cs35508j
Wu, S., Tian, S., Liu, B., Tao, H., Zhao, X., Palgrave, R. G., et al. (2018). Facile synthesis of mesoporous VO2 nanocrystals by a cotton-template method and their enhanced thermochromic properties. Sol. Energy Mater. Sol. Cells 176, 427–434. doi:10.1016/j.solmat.2017.11.001
Wu, Y. F., Fan, L. L., Chen, S. M., Chen, S., Zou, C. W., and Wu, Z. Y. (2013b). Spectroscopic analysis of phase constitution of high quality VO2 thin film prepared by facile sol-gel method. AIP Adv. 3 (4), 042132. doi:10.1063/1.4802981
Xu, F., Cao, X., Luo, H., and Jin, P. (2018). Recent advances in VO2-based thermochromic composites for smart windows. J. Mater. Chem. C 6 (8), 1903–1919. doi:10.1039/c7tc05768g
Xu, G., Jin, P., Tazawa, M., and Yoshimura, K. (2005). Thickness dependence of optical properties of VO2 thin films epitaxially grown on sapphire (0001). Appl. Surf. Sci. 244 (1–4), 449–452. doi:10.1016/j.apsusc.2004.09.157
Yanase, I. (2018). Hydrothermal synthesis and thermal change in IR reflectance of Al/W co-doped VO2 powder. Materials Research Bulletin. doi:10.1016/j.materresbull.2017.12.017
Yao, T., Zhang, X., Sun, Z., Liu, S., Huang, Y., Xie, Y., et al. (2010). Understanding the nature of the kinetic process in a VO2 metal-insulator transition. Phys. Rev. Lett. 105 (22), 226405. doi:10.1103/PhysRevLett.105.226405
Zhang, L., Xia, F., Yao, J., Zhu, T., Xia, H., Yang, G., et al. (2020a). Facile synthesis, formation mechanism and thermochromic properties of W-doped VO2 (M) nanoparticles for smart window applications. J. Mater. Chem. C 8 (38), 13396–13404. doi:10.1039/D0TC03436C
Zhang, R., Xiang, B., Feng, M., Xu, Y., Xu, L., and Xia, L. (2020b). Design of VO2-based photoactuators for smart windows. J. Mater. Sci. 55 (24), 10689–10698. doi:10.1007/s10853-020-04811-3
Zhang, Y., Li, B., Wang, Z., Tian, S., Liu, B., Zhao, X., et al. (2021). Facile preparation of Zn2V2O7 –VO2 composite films with enhanced thermochromic properties for smart windows. ACS Appl. Electron. Mater. 3 (5), 2224–2232. doi:10.1021/acsaelm.1c00176
Zhang, Z., Gao, Y., Luo, H., Kang, L., Chen, Z., Du, J., et al. (2011). Solution-based fabrication of vanadium dioxide on F:SnO2 substrates with largely enhanced thermochromism and low-emissivity for energy-saving applications. Energy & Environ. Sci. 4 (10), 4290. doi:10.1039/c1ee02092g
Zhang, Z., and Lagally, M. G. (1997). Atomistic processes in the early stages of thin-film growth. Science 276 (5311), 377–383. doi:10.1126/science.276.5311.377
Zhao, Y., Chen, C., Pan, X., Zhu, Y., Holtz, M., Bernussi, A., et al. (2013). Tuning the properties of VO2 thin films through growth temperature for infrared and terahertz modulation applications. J. Appl. Phys. 114 (11), 113509. doi:10.1063/1.4821846
Zhao, Y., Hwan Lee, J., Zhu, Y., Nazari, M., Chen, C., Wang, H., et al. (2012). Structural, electrical, and terahertz transmission properties of VO2 thin films grown on c-r-and m-plane sapphire substrates. J. Appl. Phys. 111 (5), 053533. doi:10.1063/1.3692391
Zhao, Y., Zhang, Y., Shi, Q., Liang, S., Huang, W., Kou, W., et al. (2018). Dynamic photoinduced controlling of the large phase shift of terahertz waves via vanadium dioxide coupling nanostructures. ACS Photonics 5 (8), 3040–3050. doi:10.1021/acsphotonics.8b00276
Zheng, J., Bao, S., and Jin, P. (2015). TiO2(R)/VO2(M)/TiO2(A) multilayer film as smart window: combination of energy-saving, antifogging and self-cleaning functions. Nano Energy 11, 136–145. doi:10.1016/j.nanoen.2014.09.023
Zhou, J., Gao, Y., Zhang, Z., Luo, H., Cao, C., Chen, Z., et al. (2013b). VO2 thermochromic smart window for energy savings and generation. Sci. Rep. 3 (1), 3029. doi:10.1038/srep03029
Zhou, M., Bao, J., Tao, M., Zhu, R., Lin, Y., Zhang, X., et al. (2013a). Periodic porous thermochromic VO 2 (M) films with enhanced visible transmittance. Chem. Commun. 49 (54), 6021–6023. doi:10.1039/c3cc42112k
Zhou, X., Gu, D., Li, Y., Sun, Z., Jiang, Y., and Long, Y. (2020). Abnormal dependence of microstructures and electrical properties of Y-doped VO2 thin films on deposition temperature. Ceram. Int. 46 (11), 18315–18321. doi:10.1016/j.ceramint.2020.05.053
Zhou, Y., Cai, Y., Hu, X., and Long, Y. (2014). Temperature-responsive hydrogel with ultra-large solar modulation and high luminous transmission for “smart window” applications. J. Mater. Chem. A 2 (33), 13550–13555. doi:10.1039/c4ta02287d
Zhou, Y., Cai, Y., Hu, X., and Long, Y. (2015). VO2/hydrogel hybrid nanothermochromic material with ultra-high solar modulation and luminous transmission. J. Mater. Chem. A 3 (3), 1121–1126. doi:10.1039/C4TA05035E
Zhou, Y., Wan, C., Yang, Y., Yang, H., Wang, S., Dai, Z., et al. (2019). Highly stretchable, elastic, and ionic conductive hydrogel for artificial soft electronics. Adv. Funct. Mater. 29 (1), 1806220. doi:10.1002/adfm.201806220
Zhu, B., Tao, H., and Zhao, X. (2016). Effect of buffer layer on thermochromic performances of VO2 films fabricated by magnetron sputtering. Infrared Phys. Technol. 75, 22–25. doi:10.1016/j.infrared.2016.01.004
Keywords: VO2 films, phase transition, preparation technology, modification, application
Citation: Wen C, Feng L, Li Z, Bai J, Wang S, Gao X, Wang J and Yao W (2024) A review of the preparation, properties and applications of VO2 thin films with the reversible phase transition. Front. Mater. 11:1341518. doi: 10.3389/fmats.2024.1341518
Received: 20 November 2023; Accepted: 05 January 2024;
Published: 19 January 2024.
Edited by:
Malik Maaza, National Research Foundation, South AfricaReviewed by:
Preeti Gupta, Leibniz Institute for Solid State and Materials Research Dresden (IFW Dresden), GermanyLakshmi Narayanan Mosur Saravana Murthy, Intel, United States
Copyright © 2024 Wen, Feng, Li, Bai, Wang, Gao, Wang and Yao. This is an open-access article distributed under the terms of the Creative Commons Attribution License (CC BY). The use, distribution or reproduction in other forums is permitted, provided the original author(s) and the copyright owner(s) are credited and that the original publication in this journal is cited, in accordance with accepted academic practice. No use, distribution or reproduction is permitted which does not comply with these terms.
*Correspondence: Jian Wang, d2FuZ2ppYW43MjgwMEAxNjMuY29t; Wenqing Yao, eWFvd3FAbWFpbC50c2luZ2h1YS5lZHUuY24=