- Department of Ultrasound, Beijing Tiantan Hospital, Capital Medical University, Beijing, China
Introduction: Poly(vinylidene fluoride-trifluoroethylene) [P(VDF-TrFE)] stands out as an organic polymer with remarkable piezoelectric properties, coupled with high chemically stability and biocompatibility, rendering it the most promising organic piezoelectric nanomaterial. Therefore, we aim to explore its developmental history, find current research hotspots, and identify emerging trends.
Methods: To comprehensively review the pertinent literature on poly(vinylidene fluoride-trifluoroethylene) or P(VDF-TrFE) in English from 1992 to 2022, we utilized the Web of Science Core Collection. Additionally, bibliometric tools such as CiteSpace, VOSviewer, Bibliometrics online analysis platforms (https://bibliometric.com), along with SCImage graphics, were employed to delineate historical features, track the evolution of active topics, and identify emerging trends on the P(VDF-TrFE) field.
Results: The annual publication and citation counts for articles on P(VDF-TrFE) have exhibited consistent growth since 1992, with Asia, notably China, contributing the highest number of publications on P(VDF-TrFE) materials. Interestingly, researchers in the United States demonstrated the most robust international collaborations across various countries. Keywords were categorized into nine clusters: 0# vinylidene fluoride, 1# thin-film transient, 2# energy harvesting, 3# dielectric property, 4# thin film, 5# film, 6# mobile modeling, 7# growth, and 8# pyroelectric sensor. The performance of constituent materials in electronic energy-storage applications and current and future research in nanofields, including nanofilms, nanofibers, and nanogenerator, are considered hotspots and developmental trends, respectively. Among the most cited articles, these articles are commonly related to PVDF and P(VDF-TrFE) ferroelectricity.
Conclusion: Therefore, we believe that the electromechanical coupling performance of P(VDF-TrFE) and the research in nano fields is research hotspot that will trend into the future.
1 Introduction
Polyvinylidene fluoride (PVDF) stands out as an outstanding polymer, which was synthesized for the first time in 1948. Despite its early inception, PVDF did not garner immediate attention. However, subsequent investigations revealed that PVDF films exhibit robust piezoelectric effects under uniaxial stretching conditions, elevated temperatures, and high electric fields. As a result, it has earned recognition as a pioneering piezoelectric organic polymer. Over time, extensive research has led to widespread applications of PVDF in various fields, including sensors, transducers, optical devices, and biomedical scaffold materials (Pierre, 2001; Salimi and Yousefi, 2003; Liu et al., 2011; Karan et al., 2015; Lu et al., 2020). In a bid to delve deeper into the ferroelectric properties of PVDF, scholars have introduced trifluoroethylene in proportion to PVDF (Teyssedre et al., 1995). This addition has given rise to a ferroelectric polymer termed poly(vinylidene fluoride–trifluoroethylene) [P(VDF–TrFE)]. Notably, this material undergoes ferroelectric–paraelectric phase transitions. Moreover, exposure to high-energy electron radiation induces a pronounced electrostrictive effect on P(VDF–TrFE) films (Zhang et al., 1998). Consequently, P(VDF–TrFE) has emerged as a focal point in the exploration of ferroelectric polymers. The underlying source of the piezoelectric and ferroelectric attributes of P(VDF–TrFE) lies in its molecular backbone, which incorporates highly electronegative fluorine atoms. These atoms confer significant polarity to the C-F bonds within the material. The rotational potential energy between TrFE and VDF units in the polymer prevents the formation of a helical P(VDF–TrFE) molecular chain structure; rather an all-trans configuration (TTTT) is formed (María et al., 2022), as shown in Supplementary Figure S1. In other words, fluorine atoms in P(VDF–TrFE) are gathered on one side of the main carbon chain to the maximum extent, leading to dipole moments between fluorine and hydrogen atoms. The dipoles in each cell are oriented in the same direction and form a strongly polar β-phase structure. The semi-crystalline structure of P(VDF–TrFE) is characterized by the non-alignment of internal positive and negative charges, enabling spontaneous polarization. However, the polarization directions within distinct regions, referred to as ferroelectric domains, are not uniformly distributed, leading to a macroscopic cancellation of polarization when observed externally. Nevertheless, it is essential to note that within each ferroelectric domain, polarization directions maintain consistency. External electric fields or forces can significantly influence these ferroelectric domains. In response, the volume of each domain increases, thereby enhancing its polarization intensity. Notably, when compared to PVDF, P(VDF–TrFE) demonstrates superior thermal stability, effective electromechanical coupling factors, and heightened piezoelectric performance (Lovinger et al., 1983; Gregorio and Botta, 1998).
In 1978, Hicks et al. (1978) reported that the dielectric constant of P(VDF–TrFE) exhibits temperature dependency, and the transition from the ferroelectric state to the paraelectric state is governed by the temperature. The systematic arrangement of dipoles in the β-phase P(VDF–TrFE) crystal was confirmed through slow annealing, establishing its ferroelectric nature (Hafner et al., 2021). However, the practical application of P(VDF–TrFE) is constrained by its piezoelectric coefficient (d33), which is less than −30 pC·N⁻1, which limits its practical applications (Hu et al., 2020). Consequently, researchers have explored the development of piezoelectric nanocomposites by incorporating inorganic piezoelectric materials (Hu et al., 2019; Khan et al., 2021), piezoelectric ceramics (Wang et al., 2018), and metal ions (Jia et al., 2019; Wang et al., 2019) into P(VDF–TrFE) as a matrix, with excellent piezoelectric properties reported. (Yin et al., 2019). Such materials have been widely used to measure electroacoustic, ultrasonic, underwater acoustic, biomedical engineering, and mechanical properties (Zhou et al., 2020).
Over the past three decades, the annual research output on P(VDF–TrFE) has witnessed a consistent increase, resulting in the publication of numerous articles across a diverse spectrum of journals. The majority of these contributions are categorized under multidisciplinary materials science, applied physics, condensed matter physics, and nanotechnology/nanoscience. Searching the literature to determine the current state of P(VDF–TrFE) material research and the associated hotspots is a time-consuming and laborious task. In contrast to traditional reviews, bibliometrics are capable of more objectively and comprehensively providing a historical overview and determining the research hotspots and developmental trends in a specific field.
In this study, we used CiteSpace, VOSviewer, and bibliometric online analysis platforms (https://bibliometric.com) and SCImage graphics software to visually analyze English articles related to P(VDF–TrFE) materials published since 1992, with the aim of exploring its developmental history and finding the current research hotspots.
2 Methods
2.1 Data collection
All relevant results on poly(vinylidene fluoride–trifluoroethylene) or P(VDF–TrFE) in English between 1992 and 2022 were retrieved from the Web of Science Core Collection (WoSCC) as the object database, through the Science Citation Index Expanded (SCIE). A total of 1,968 eligible records were saved as plain text files and tab-delimited files in the “Full Record and Cited References” format for analysis.
2.2 Bibliometric analysis tools
CiteSpace, VOSviewer, and bibliometric online analysis platforms (https://bibliometric.com) and SCImage graphics software were used in this study. Different analysis software tools were used for each topic.
2.2.1 The historical features of P(VDF–TrFE)-related articles
We clicked on Citation Report on the data collection page of the WoSCC and adjusted the publication time to 1992–2022. We then analyzed the citation index corresponding to the number of papers published annually from 1992 to 2022 and obtained information on the top 10 cited papers.
2.2.2 Scientific cooperation between different regions
Studied research topics can depend on the geographical region, and the national research status can be reflected through publications. However, scientific research requires extensive cooperation, and investigating scientific cooperation can reveal the research status of specific scientific fields more deeply (Chen, 2004). VOSviewer preprocesses and then processes the data using SCImage to reveal the global geographic distributions of publications and the intensity of national cooperation. CiteSpace processes the data to produce timelines and clustering relationships between different countries.
2.2.3 Scientific cooperation between institutions and researchers
The relationships between researchers, institutions, and countries can better reflect the research status of a certain field. CiteSpace defines scientific cooperation by analyzing the convergence relationship between authors and institutions and the research timeline and analyzes the most influential research institutions and researchers using the bibliometric online analysis platform.
2.2.4 Keywords
Keywords related to the P(VDF–TrFE) research theme are time-dependent, and this dependency can objectively reflect the research trend in a specific scientific field. CiteSpace was used to analyze keyword clustering between 1992 and 2022, with the date objectively presented in the form of a keyword timeline chart. VOSviewer was used to analyze the thermographic keyword. We used a specific text data-mining algorithm to identify “activity bursts” and used them to show how interests in certain research topics change over time, using CiteSpace to detect keyword bursts (Kleinberg, 2003).
2.2.5 References
Co-citation can highlight the reading value and research trend of cited literature, which enables scholars to quickly obtain valuable research articles from thousands of published articles and mine them in an in-depth manner (Chen et al., 2010; Chen et al., 2014). Information related to the most cited articles and associated research papers was obtained through CiteSpace clustering analysis, with information pertaining to highly cited references at various stages between 1992 and 2022 researched through burst detection.
2.2.6 Journal analysis
More reliable reference options can be provided to scholars when writing papers and selecting target journals by analyzing journals that publish papers related to P(VDF–TrFE). The top 10 journals with the most citations, total citations, and average citations were selected through the bibliometric online analysis platform, after which the coexisting relationships between journals were analyzed through CiteSpace clustering. The relationship between periodic publishing and time was analyzed by detecting bursts.
3 Results and discussion
3.1 Historical features of P(VDF–TrFE)-related articles
The number of research reports on P(VDF–TrFE) grew with each passing day between January 1992 and December 2022, with a cumulative total of 1,968 papers spanning across 64 disciplines. Multidisciplinary materials science emerged as the predominant category, which accounted for 42.23% (831/1,968) of the articles, followed by applied physics and condensed matter physics, which accounted for 39.02% (768/1,968) and 18.50% (364/1,968) of the articles, respectively (Supplementary Figure S2). In 1992, 11 articles related to P(VDF–TrFE) were published, accompanied by a single citation. Since then, the annual publication rate has consistently risen, reaching its highest level in 2019 with 154 papers. Simultaneously, the number of citations also increased significantly, peaking in 2021 at 6,462 times, resulting in a total of 45,712 citations and an average of 23.23 citations per article (Figure 1). Chinese researchers have been particularly prolific in contributing to P(VDF–TrFE) literature, publishing the highest number of articles with 677 articles (34.40%). This represents a significant share, with an average of 60 relevant articles per year in the most recent 4 years. Researchers from the United States (USA) published the second largest number of articles with 312 papers (15.85%), which is less than half the number of articles published by Chinese researchers (Supplementary Figure S3). Therefore, we have a reason to believe that research on P(VDF–TrFE) is increasing, with Chinese researchers taking the lead.
We conducted an analysis of the top 10 most cited articles, as outlined in Table 1. The article authored by Zhang et al. (1998) stands out with an impressive citation count of 1,246, having been published in Science, a premier international journal. This seminal paper was the first to propose that irradiating P(VDF–TrFE) with electrons induces significant electrostriction and ferroelectric relaxation. The key insight presented suggests that electron irradiation disrupts the coherent polarization domain (all-trans chains) in the normal ferroelectric P(VDF–TrFE) copolymer, transforming it into a relaxor ferroelectric with nanopolar regions (nanometer-size, all-trans chains interrupted by trans and gauche bonds). The top 10 most frequently cited articles all found their place in Journal Citation Reports (JCR) Q1 magazines, most of which are the top journals in the field, including Science, Advanced Materials, and Nature Materials, among others. Notably, the study by Kim et al. (2014), published in the Journal of the American Chemical Society, consistently garnered the highest annual citation count on average. This particular paper marks a significant advancement toward plastic electronic products with excellent charge mobilities (14.4 cm2/V·s), achieved through coating with P(VDF–TrFE) and poly(thienoisoindigo-alt-naphthalene).
Therefore, the research on P(VDF–TrFE) mainly focuses on its electrostriction and ferroelectric relaxation and coating modification to obtain more excellent ferroelectric and piezoelectric properties.
3.2 Scientific cooperation between regions
The global distribution of publications on P(VDF–TrFE) reveals a concentration in Asia, North America, and Europe (Figure 2). Notably, researchers in France (1992), Brazil (1993), Germany (1993), Japan (1993), and the United States (1993) were among the pioneers, starting investigations on P(VDF–TrFE) materials in 1992 and 1993. Subsequently, China (1995) and Russia (1996) joined the ranks in 1995 and 1996, respectively, contributing significantly over extended periods (Figure 2B).
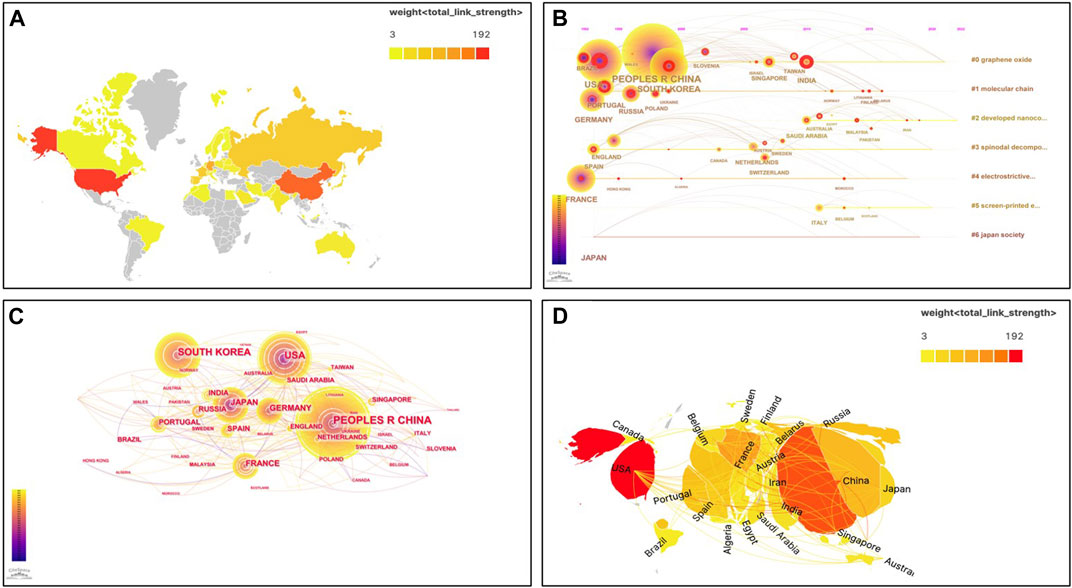
FIGURE 2. Strength chart of the published articles in different countries. (A) General geographical distribution map of global publications; (B) timeline chart of the articles published by different countries; (C) cluster diagram of the articles published in different countries; and (D) overall contact map of different countries.
Cumulatively, Chinese researchers take the lead in P(VDF–TrFE) publications with 677 papers, followed by the United States (312 papers) and South Korea (287 papers). The cluster chart in Figure 2C visually represents the contributions from various countries, with the size of each circle indicating the publication volume. Despite China having the highest number of papers, the United States emerges as the leader in international collaboration, followed by China, France, and Japan (Supplementary Figure S4). The color intensity, as shown in Figure 2D, signifies the level of international cooperation from other countries.
The identification of strong reference bursts has allowed for an in-depth analysis of P(VDF–TrFE) research across different countries and time periods, revealing hotspots and trends (Supplementary Figure S5). The red line in the figure highlights the most active time span for published articles. Notably, although French researchers initiated P(VDF–TrFE) investigations, their activity was concentrated between 1992 and 1998. In contrast, researchers from India (2018–2022), Australia (2019–2022), Chinese Taiwan (2020–2022), and Austria (2020–2022) began their activities later (in 2010, 2011, 2009, and 2007, respectively) and are currently engaged in ongoing research.
3.3 Scientific cooperation between institutions and researchers
The Pennsylvania State University takes the lead among single institutions in publishing P(VDF–TrFE)-related articles, boasting a total citation frequency of 2,575 and the highest average and average first-author citation frequencies. Although China contributed the largest number of publications, the institutional influence of The Pennsylvania State University surpassed that of any single Chinese institution. Notably, seven Chinese institutions, namely, the Hong Kong Polytechnic University, the Chinese Academy of Sciences, Xi’an Jiaotong University, Nanjing University, Tsinghua University, Zhejiang University, and Fudan University, prominently feature under the top 10 institutional influences (Table 2).
Examining the clustering graph of the total number of published articles according to institutions, The Pennsylvania State University is enclosed within the largest circle, signifying that researchers from this institution published the highest total number of articles (Figure 3A). Further clustering, where 15 is set as the lower boundary value for the total number of articles published by an institution, underscores China’s dominance in the number of articles published by a single Chinese institution, which is still less than that of The Pennsylvania State University in the United States (Figure 3B).
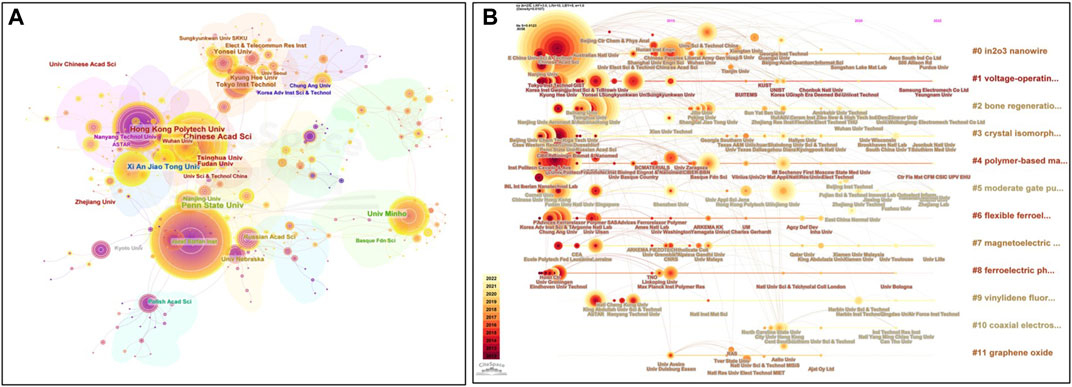
FIGURE 3. Articles published by different institutions. (A) Cluster diagram of the articles published by different institutions; (B) timeline chart of the articles published by different institutions.
Timeline charts for P(VDF–TrFE)-related research papers from various institutions highlight the popularity and research hotspots in different countries. The Pennsylvania State University and the Chinese Academy of Sciences emerge as leaders in conducting research on P(VDF–TrFE) materials. Notably, numerous institutions worldwide engage in P(VDF–TrFE) material research as the timeline progresses, including the Hong Kong Polytechnic University and South China University.
For evaluating author influence, their contribution and impact were assessed based on the citation frequency and average citation frequency of articles related to P(VDF–TrFE) materials written by the author as the first or corresponding author (Table 3). Noteworthy authors include Martins and Lanceros (Fujisaki et al., 2007), with their papers considered representative studies. Among the top 10 authors, teams in the United States that include Zhang et al. (1998) received the highest number of citations (Supplementary Table S1). This analysis underscores the importance of considering both the number of papers published and their impact, as demonstrated by the example of Chan and his team, who published a significant number of articles but received fewer citations on average (Supplementary Table S2). This emphasizes the need to evaluate an author’s influence by examining both the quantity and impact of their work.
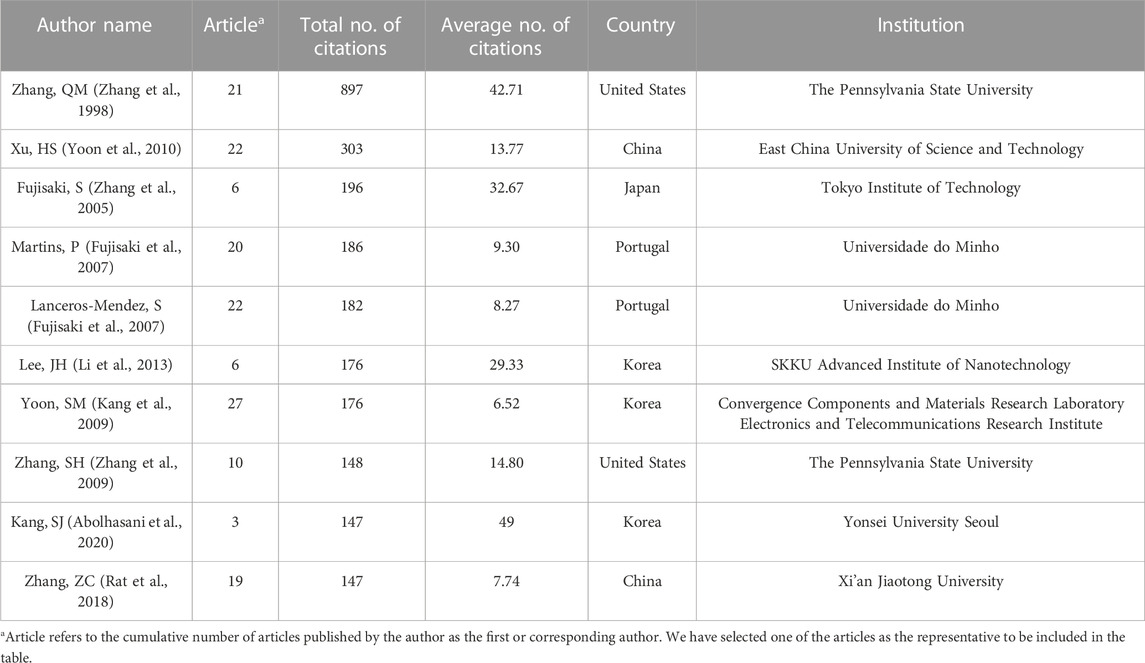
TABLE 3. Top 10 most cited authors who published P(VDF–TrFE) articles as the first or corresponding author.
3.4 Keywords
The keyword clustering analysis, conducted using CiteSpace software, revealed nine distinct clusters, each encompassing specific themes within P(VDF–TrFE) research. Figure 4A illustrates the following nine clusters: cluster 0# vinylidene fluoride, cluster 1# thin-film transient, cluster 2# energy harvesting, cluster 3# dielectric property, cluster 4# thin film, cluster 5# film, cluster 6# mobile modeling, cluster 7# growth, and cluster 8# pyroelectric sensor. A thermographic keyword display was created to delve deeper into each cluster, and vinylidene fluoride (VDF) is the raw material used to synthesize P(VDF–TrFE), and it ranked first in the keyword cluster (Figure 4B). As excellent piezoelectric materials, PVDF and P(VDF–TrFE) can create electrical energy for storage by widely collecting mechanical energy (Yoon et al., 2010). Therefore, significant research attention has been directed toward nanogenerators in recent years (Supplementary Figure S6). A P(VDF–TrFE) piezoelectric film was prepared using electrospinning technology for use in sewage treatment (Tseng et al., 2013), ultrasonic transducers (Jeong and Shung, 2013), and magnetoelectric strain-coupling (Mardana et al., 2011) applications, as well as cell experiments (Li et al., 2022). The keyword “temperature” is presented in the heatmap, which is not as hot as film or VDF, but the piezoelectricity and ferroelectricity of P(VDF–TrFE) films are affected by the temperature and the morphology of the films, as well as the conformation and orientation of the crystals change significantly after thermal annealing (Qian et al., 2018; Ng et al., 2020; Hafner et al., 2021).
P(VDF–TrFE) forms nanoparticles with both ferroelectric and piezoelectric properties. The keyword “nano” exhibited a burst, mainly between 2018 and 2022 and included nanoparticle (2018–2022, strength: 8.47), polymer nanocomposite (2019–2022, strength: 8.15), nanogenerator (2020–2022, strength: 8.95), and nanofiber (2020–2022, strength: 6.82) (Supplementary Figure S7). Therefore, we believe that the preparation of nanogenerators using P(VDF–TrFE) will be a research hotspot in the next few years owing to its excellent piezoelectric properties.
3.5 References
Citation analysis using CiteSpace is limited to a maximum of 1,000 papers. To overcome this limitation, the citations were analyzed in two parts: the first 15 years (1992–2007) and the last 15 years (2007–2022). Supplementary Figures S8, S9 include a cluster diagram and the top 25 references with the strongest article citation bursts between 1992 and 2007, respectively. In 2014, Martins et al. (2014) published their research on the electrical activities of PVDF and P(VDF–TrFE), cited at a frequency of up to 85 times per year. Subsequently, Hu et al. (2008) in 2008 explored high-density arrays of ferroelectric polymer nanostructures in Nature Materials, receiving a citation frequency of 49 times per year. The third notable reference is a review article on ferroelectric-based organic nonvolatile memory devices by Naber et al. (2010) in 2010, cited up to 35 times per year. A cluster diagram of citations since 2007 is depicted in Figure 5.
Supplementary Figure S10 showcases the top 25 articles with the strongest citation bursts between 2007 and 2022. Naber et al. (2005) published their research on organic nonvolatile memory-based transistors, with a strength of up to 24.38 observed. Since 2017, cited references have dramatically changed, featuring works by Liu et al. (2018), Chen et al. (2017), and Katsouras et al. (2016). These recent articles are commonly related to PVDF and P(VDF–TrFE) ferroelectricity, suggesting that the electromechanical coupling performance of P(VDF–TrFE) remains a prominent and evolving research hotspot.
3.6 Journal analysis
Applied Physics Letters stands out as the journal with the highest number of citations related to P(VDF–TrFE) materials, publishing a total of 84 related articles with a cumulative citation count of 660. Among the top 10 journals, 60% of journals are classified as JCR Q1, 30% as JCR Q2, and 10% as JCR Q4. These journals are primarily based in the United States (six), England (two), and the Netherlands (two) (Table 4). Ferroelectrics, among the various journals, has published the largest number of articles on P(VDF–TrFE) materials, totaling up to 114 (Supplementary Table S3).
The cited journal frequency cluster diagram (Figure 6) illustrates strong relationships between the cited journals. The Journal of Applied Physics exhibited the highest strength (67.72) when analyzing the top 25 cited journals with the strongest bursts. The burst analysis reveals that many journals were cited extensively from the beginning of 2000, with some continuing until 2013 (Supplementary Figure S11). As an example, Science, despite having only three papers related to P(VDF–TrFE) materials published between 1992 and 2022, has had a significant impact. Zhang et al. (1998) published in 1998 and Neese et al. (2008) and Guo et al. (2021) published in 2008 and 2021, respectively, with these three articles being cited on an average of 117.67 times per year (Supplementary Table S4). Since 2019, articles published in the Journal of Materials Chemistry A, Polymers, ACS Applied Materials & Interfaces, Advanced Electronic Materials, Nano Energy, Advanced Science, Composite Materials Part B: Engineering, and Advanced Materials Technologies have continued to receive citations until 2022. Notably, these journals all fall under the JCR division Q1, suggesting that choosing such journals to publish articles related to P(VDF–TrFE) materials may represent a trend in the field.
4 Conclusion
Research on P(VDF–TrFE) materials has seen a continuous increase since 1992, accompanied by a significant rise in the citation rate of articles. Notably, a substantial proportion of articles are contributed by researchers in Asia, with China leading the way and accounting for 34.40% of the total number of articles. The top 10 influential institutions engaged in P(VDF–TrFE) research predominantly hail from China (six institutions), followed by the United States (three institutions). Keyword analysis has been employed to offer insights into the historical progression and research trends of P(VDF–TrFE) materials. These trends extend to nanomaterials, including nanofibers and nanogenerators, showcasing the evolving performance of constituent materials and their applications in energy reserves for use in electronics. A notable observation is the commonality between cited articles and published journals, suggesting that future trends may revolve around ferroelectric research involving P(VDF–TrFE) and the meticulous selection of high-quality journals for publication. This reflects the anticipation of sustained interest and advancements in these areas within the field of nanomaterials.
Data availability statement
The original contributions presented in the study are included in the article/Supplementary Material; further inquiries can be directed to the corresponding authors.
Author contributions
ZC: conceptualization, formal analysis, and writing–original draft. WH: conceptualization and writing–review and editing. WZ: funding acquisition and writing–review and editing.
Funding
The authors declare that financial support was received for the research, authorship, and/or publication of this article. This work was supported by the National Natural Science Foundation of China under grants 82001840 and 82171935.
Acknowledgments
The authors thank Wiley Editing Services for doing a lot of research on the grammar and vocabulary of the article.
Conflict of interest
The authors declare that the research was conducted in the absence of any commercial or financial relationships that could be construed as a potential conflict of interest.
Publisher’s note
All claims expressed in this article are solely those of the authors and do not necessarily represent those of their affiliated organizations, or those of the publisher, the editors, and the reviewers. Any product that may be evaluated in this article, or claim that may be made by its manufacturer, is not guaranteed or endorsed by the publisher.
Supplementary material
The Supplementary Material for this article can be found online at: https://www.frontiersin.org/articles/10.3389/fmats.2023.1292256/full#supplementary-material
References
Abolhasani, M. M., Naebe, M., Hassanpour Amiri, M., Shirvanimoghaddam, K., Anwar, S., Michels, J. J., et al. (2020). Hierarchically structured porous piezoelectric polymer nanofibers for energy harvesting. Adv. Sci. (Weinh). 7 (13), 2000517. doi:10.1002/advs.202000517
Chen, C. (2004). Searching for intellectual turning points: progressive knowledge domain visualization. Proc. Natl. Acad. Sci. U. S. A. 101, 5303–5310. suppl. doi:10.1073/pnas.0307513100
Chen, C., Dubin, R., and Kim, M. C. (2014). Emerging trends and new developments in regenerative medicine: a scientometric update (2000 - 2014. Expet Opin. Biol. Ther. 14 (9), 1295–1317. doi:10.1517/14712598.2014.920813
Chen, C., Ibekwe-SanJuan, F., and Hou, J. (2010). The structure and dynamics of cocitation clusters: a multiple-perspective cocitation analysis. J. Am. Soc. Inf. Sci. Technol. 61 (7), 1386–1409. doi:10.1002/asi.21309
Chen, X., Han, X., and Shen, Q. (2017). PVDF-Based ferroelectric polymers in modern flexible electronics. Adv. Electron. Mater. 3 (5), 1600460. doi:10.1002/aelm.201600460
Fujisaki, S., Ishiwara, H., and Yoshihisa, F. (2007). Low-voltage operation of ferroelectric poly(vinylidene fluoride-trifluoroethylene) copolymer capacitors and metal-ferroelectric- insulator-semiconductor diodes. Appl. Phys. Lett. 90. doi:10.1063/1.2723678
Gregorio, R., and Botta, M. M. (1998). Effect of crystallization temperature on the phase transitions of P(VDF/TrFE) copolymers. J. Polym. Sci. Part B Polym. Phys. 36, 403–414. doi:10.1002/(sici)1099-0488(199802)36:3<403::aid-polb2>3.0.co;2-s
Guo, M., Guo, C., Han, J., Chen, S., He, S., Tang, T., et al. (2021). Toroidal polar topology in strained ferroelectric polymer. Science 371 (6533), 1050–1056. doi:10.1126/science.abc4727
Hafner, J., Benaglia, S., Richheimer, F., Teuschel, M., Maier, F. J., Werner, A., et al. (2021). Multi-scale characterisation of a ferroelectric polymer reveals the emergence of a morphological phase transition driven by temperature. Nat. Commun. 12 (1), 152. doi:10.1038/s41467-020-20407-6
Hicks, J. C., Jones, T. E., and Logan, J. C. (1978). Ferroelectric properties of poly(vinylidene fluoride-tetrafluoroethylene). J. Appl. Phys. 49 (12), 6092–6096. doi:10.1063/1.324528
Hu, X., Jiang, Y., Ma, Z., Xu, Y., and Zhang, D. (2019). Bio-inspired flexible lateral line sensor based on P(VDF-TrFE)/BTO nanofiber mat for hydrodynamic perception. Sensors (Basel) 19 (24), 5384. doi:10.3390/s19245384
Hu, X., Yu, S., and Chu, B. (2020). Increased effective piezoelectric response of structurally modulated P(VDF-TrFE) film devices for effective energy harvesters. Mater. Des. 192, 108700. doi:10.1016/j.matdes.2020.108700
Hu, Z., Tian, M., Nysten, B., and Jonas, A. M. (2008). Regular arrays of highly ordered ferroelectric polymer nanostructures for non-volatile low-voltage memories. Nat. Mater. 8 (1), 62–67. doi:10.1038/nmat2339
Jeong, J. S., and Shung, K. K. (2013). Improved fabrication of focused single element P(VDF-TrFE) transducer for high frequency ultrasound applications. Ultrasonics 53 (2), 455–458. doi:10.1016/j.ultras.2012.08.013
Jia, E., Wei, D., Cui, P., Ji, J., Huang, H., Jiang, H., et al. (2019). Efficiency enhancement with the ferroelectric coupling effect using P(VDF-TrFE) in CH3NH3PbI3 solar cells. Adv. Sci. (Weinh) 6 (16), 1900252. doi:10.1002/advs.201900252
Kang, S. J., Bae, I., Park, Y. J., Ho, T., Sung, J., Yoon, S. C., et al. (2009). Non-volatile ferroelectric poly(vinylidene fluoride-co-trifluoroethylene) memory based on a single-crystalline tri-isopropylsilylethynyl pentacene field-effect transistor. Adv. Funct. Mater. 19, 1609–1616. doi:10.1002/adfm.200801097
Karan, S. K., Mandal, D., and Khatua, B. B. (2015). Self-powered flexible Fe-doped RGO/PVDF nanocomposite: an excellent material for a piezoelectric energy harvester. Nanoscale 7 (24), 10655–10666. doi:10.1039/c5nr02067k
Katsouras, I., Asadi, K., Li, M., van Driel, T. B., Kjær, K. S., Zhao, D., et al. (2016). The negative piezoelectric effect of the ferroelectric polymer poly(vinylidene fluoride). Nat. Mater 15 (1), 78–84. doi:10.1038/nmat4423
Khan, S. A., Saqib, M., Rehman, M. M., Mutee Ur Rehman, H. M., Rahman, S. A., Yang, Y., et al. (2021). A full-range flexible and printed humidity sensor based on a solution-processed P(VDF-TrFE)/Graphene-Flower composite. Nanomater. (Basel) 11 (8), 1915. doi:10.3390/nano11081915
Kim, G., Kang, S. J., Dutta, G. K., Han, Y. K., Shin, T. J., Noh, Y. Y., et al. (2014). A thienoisoindigo-naphthalene polymer with ultrahigh mobility of 14.4 cm2/V·s that substantially exceeds benchmark values for amorphous silicon semiconductors. J. Am. Chem. Soc. 136 (26), 9477–9483. doi:10.1021/ja504537v
Kleinberg, J. (2003). Bursty and hierarchical structure in streams. Data Min. know;edge Discov. 4 (7), 373–397. doi:10.1023/A:1024940629314
Lee, J. H., Lee, K. Y., Gupta, M. K., Kim, T. Y., Lee, D. Y., Oh, J., et al. (2014). Highly stretchable piezoelectric-pyroelectric hybrid nanogenerator. Adv. Mater 26 (5), 765–769. doi:10.1002/adma.201303570
Lee, J. H., Yoon, H. J., Kim, T. Y., Gupta, M. K., Lee, J. H., Seung, W., et al. (2015). Micropatterned P(VDF-TrFE) film-based piezoelectric nanogenerators for highly sensitive self-powered pressure sensors. Adv. Funct. Mater. 25 (21), 3203–3209. doi:10.1002/adfm.201500856
Li, M., Wondergem, H. J., Spijkman, M. J., Asadi, K., Katsouras, I., Blom, P. W., et al. (2013). Revisiting the δ-phase of poly (vinylidene fluoride) for solution-processed ferroelectric thin films. Nat. Mater 12 (5), 433–438. doi:10.1038/nmat3577
Li, X., Heng, B. C., Bai, Y., Wang, Q., Gao, M., He, Y., et al. (2022). Electrical charge on ferroelectric nanocomposite membranes enhances SHED neural differentiation. Bioact. Mater 20, 81–92. doi:10.1016/j.bioactmat.2022.05.007
Liu, F., Awanis Hashim, N., Liu, Y., Moghareh Abed, M. R., and Li, K. (2011). Progress in the production and modification of PVDF membranes. J. Membr. Sci. 375 (1-2), 1–27. doi:10.1016/j.memsci.2011.03.014
Liu, Y., Aziguli, H., Zhang, B., Xu, W., Lu, W., Bernholc, J., et al. (2018). Ferroelectric polymers exhibiting behaviour reminiscent of a morphotropic phase boundary. Nature 562 (7725), 96–100. doi:10.1038/s41586-018-0550-z
Lou, Z., Chen, S., Wang, L., Jiang, K., and Shen, G. (2016). An ultra-sensitive and rapid response speed graphene pressure sensors for electronic skin and health monitoring. Nano Energy 23, 7–14. doi:10.1016/j.nanoen.2016.02.053
Lovinger, A. J., Furukawa, T., Davis, G. T., and Broadhurst, M. G. (1983). Crystallographic changes characterizing the Curie transition in three ferroelectric copolymers of vinylidene fluoride and trifluoroethylene: 2. Oriented or poled samples. Polymer 24, 1233–1239. doi:10.1016/0032-3861(83)90051-4
Lu, L., Ding, W., Liu, J., and Yang, B. (2020). Flexible PVDF based piezoelectric nanogenerators. Nano Energy 78, 105251. doi:10.1016/j.nanoen.2020.105251
Mandal, D., Sun, Y., and Kim, K. J. (2011). Origin of piezoelectricity in an electrospun poly (vinylidene fluoride-trifluoroethylene) nanofiber web-based nanogenerator and nano-pressure sensor. Macromol. Rapid Commun. 32 (11), 831–837. doi:10.1002/marc.201100040
Mardana, A., Ducharme, S., and Adenwalla, S. (2011). Ferroelectric control of magnetic anisotropy. Nano Lett. 11 (9), 3862–3867. doi:10.1021/nl201965r
María, N., Le Goupil, F., Cavallo, D., Maiz, J., and Müller, A. J. (2022). Effect of the TrFE content on the crystallization and SSA thermal fractionation of P(VDF-co-TrFE) copolymers. Int. J. Mol. Sci. 23 (18), 10365. doi:10.3390/ijms231810365
Martins, P., Lopes, A. C., and Lanceros-Mendez, S. (2014). Electroactive phases of poly (vinylidene fluoride): determination, processing and applications. Prog. Polym. Sci. 39 (4), 683–706. doi:10.1016/j.progpolymsci.2013.07.006
Martins, P., Moya, X., Phillips, L. C., KarNarayan, S., Mathur, N. D., and LancerosMendez, S. (2012). Linear anhysteretic direct magnetoelectric effect in Ni0.5Zn0.5Fe2O4/poly(vinylidene fluoride-trifluoroethylene) 0-3 nanocomposites. J. Phys. D Appl. Phys. 44 (48), 482001–482004. (4). doi:10.1088/0022-3727/44/48/482001
Naber, R., Asadi, K., Blom, P., Leeuw, D. M. D., and Boer, B. D. (2010). Organic nonvolatile memory devices based on ferroelectricity. Adv. Mater. 22 (9), 933–945. doi:10.1002/adma.200900759
Naber, R., Boer, B. D., Blom, P., and de Leeuw, D. M. (2005). Low-voltage polymer field-effect transistors for nonvolatile memories. Appl. Phys. Lett. 87 (20), 203509–203509-3. doi:10.1063/1.2132062
Neese, B., Chu, B., Lu, S. G., Wang, Y., Furman, E., and Zhang, Q. M. (2008). Large electrocaloric effect in ferroelectric polymers near room temperature. Science 321 (5890), 821–823. doi:10.1126/science.1159655
Ng, C. Y. B., Gan, W. C., Velayutham, T. S., Goh, B. T., and Hashim, R. (2020). Structural control of the dielectric, pyroelectric and ferroelectric properties of poly(vinylidene fluoride-co-trifluoroethylene) thin films. Phys. Chem. Chem. Phys. 22 (4), 2414–2423. doi:10.1039/c9cp01556f
Peng, B. L., Fan, H. Q., and Zhang, Q. (2013). A giant electrocaloric effect in nanoscale antiferroelectric and ferroelectric phases coexisting in a relaxor Pb0.8Ba0.2ZrO3 thin film at room temperature. Adv. Funct. Mater. 23 (23), 2987–2992. doi:10.1002/adfm.201202525
Pierre, U. (2001). PVDF piezoelectric polymer. Sens. Rev. 21 (2), 118–126. doi:10.1108/02602280110388315
Qian, J., Jiang, S., Wang, Q., Yang, C., Duan, Y., Wang, H., et al. (2018). Temperature dependence of piezo- and ferroelectricity in ultrathin P(VDF-TrFE) films. RSC Adv. 8 (51), 29164–29171. doi:10.1039/c8ra05648j
Rat, S., Piedrahita-Bello, M., Salmon, L., Molnár, G., Demont, P., and Bousseksou, A. (2018). Coupling mechanical and electrical properties in spin crossover polymer composites. Adv. Mater 30 (8). doi:10.1002/adma.201705275
Salimi, A., and Yousefi, A. A. (2003). Analysis method. Polym. Test. 22 (6), 699–704. doi:10.1016/S0142-9418(03)00003-5
Teyssedre, G., Bernes, A., and Lacabanne, C. (1995). Cooperative movements associated with the Curie transition in P(VDF-TrFE) copolymers. J. Polym. Sci. Part B Polym. Phys. 33, 879–890. doi:10.1002/polb.1995.090330603
Tseng, H. J., Tian, W. C., and Wu, W. J. (2013). P(VDF-TrFE) polymer-based thin films deposited on stainless steel substrates treated using water dissociation for flexible tactile sensor development. Sensors (Basel) 13 (11), 14777–14796. doi:10.3390/s131114777
Wang, A., Hu, M., Zhou, L., and Qiang, X. (2018). Self-Powered wearable pressure sensors with enhanced piezoelectric properties of aligned P(VDF-TrFE)/MWCNT composites for monitoring human physiological and muscle motion signs. Nanomater. (Basel) 8 (12), 1021. doi:10.3390/nano8121021
Wang, X., Shen, H., Chen, Y., Wu, G., Wang, P., Xia, H., et al. (2019). Multimechanism synergistic photodetectors with ultrabroad spectrum response from 375 nm to 10 µm. Adv. Sci. (Weinh) 6 (15), 1901050. doi:10.1002/advs.201901050
Xia, F., Cheng, Z. Y., Xu, H. S., Li, H. F., Belfield, K. D., Kavarnos, G., et al. (2002). High electromechanical responses in a poly (vinylidene fluoride–trifluoroethylene–chlorofluoroethylene) terpolymer. Adv. Mater. 14 (21), 1574–1577. doi:10.1002/1521-4095(20021104)14:21<1574::aid-adma1574>3.0.co;2-#
Xu, H., Liu, X., Fang, X., Xie, H., Li, G., Meng, X., et al. (2009). Domain stabilization effect of interlayer on ferroelectric poly(vinylidene fluoride-trifluoroethylene) copolymer ultrathin film. J. Appl. Phys. 105 (3), 22. doi:10.1063/1.3075897
Yin, Z., Tian, B., Zhu, Q., and Duan, C. (2019). Characterization and application of PVDF and its copolymer films prepared by spin-coating and Langmuir-blodgett method. Polym. (Basel) 11 (12), 2033. doi:10.3390/polym11122033
Yoon, S. M., Yang, S., Byun, C., Park, S. H. K., Cho, D. H., Jung, S. W., et al. (2010). Fully transparent non-volatile memory thin-film transistors using an organic ferroelectric and oxide semiconductor below 200 °C. Adv. Funct. Mater. 20 (6), 921–926. doi:10.1002/adfm.200902095
Zhang, Q. M., Bharti, V. V., and Zhao, X. (1998). Giant electrostriction and relaxor ferroelectric behavior in electron-irradiated poly (vinylidene fluoride-trifluoroethylene) copolymer. Science 280 (5372), 2101–2104. doi:10.1126/science.280.5372.2101
Zhang, S., Zhang, N., Huang, C., Ren, K., and Zhang, Q. (2005). Microstructure and electromechanical properties of carbon nanotube/poly(vinylidene fluoride — trifluoroethylene — chlorofluoro-ethylene) composites. Adv. Mater. 17 (15), 1897–1901. doi:10.1002/adma.200500313
Zhang, Z., Meng, Q., and Chung, T. (2009). Energy storage study of ferroelectric poly(vinylidene fluoride-trifluoroethylene-chlorotrifluoroethylene) terpolymers. Polymer 50 (2), 707–715. doi:10.1016/j.polymer.2008.11.005
Keywords: bibliometric, poly(vinylidene fluoride–trifluoroethylene), nanofilms, piezoelectric nanomaterial, polyvinylidene fluoride
Citation: Chen Z, He W and Zhang W (2023) Bibliometric visual analysis of the poly(vinylidene fluoride–trifluoroethylene) piezoelectric nanomaterial: research history, hotspots, and developmental trend. Front. Mater. 10:1292256. doi: 10.3389/fmats.2023.1292256
Received: 11 September 2023; Accepted: 13 November 2023;
Published: 28 November 2023.
Edited by:
Fahmi Zairi, Université de Lille, FranceReviewed by:
Svitlana Kopyl, University of Aveiro, PortugalSumanta Kumar Karan, The Pennsylvania State University (PSU), United States
Copyright © 2023 Chen, He and Zhang. This is an open-access article distributed under the terms of the Creative Commons Attribution License (CC BY). The use, distribution or reproduction in other forums is permitted, provided the original author(s) and the copyright owner(s) are credited and that the original publication in this journal is cited, in accordance with accepted academic practice. No use, distribution or reproduction is permitted which does not comply with these terms.
*Correspondence: Wen He, aGV3ZW5AYmp0dGgub3Jn; Wei Zhang, dWx0cmF6d0BzaW5hLmNvbQ==