- College of Civil Engineering, Guizhou University, Guiyang, China
Fire accidents happen randomly which may occur in the construction of concrete buildings. In this study, the effects of high temperature and curing ages on shear properties of early-age concrete were experimentally studied. The pore structure of early-age concrete before heating, after heating, and re-curing after heating were examined through nuclear magnetic resonance and scanning electron microscope tests. Experimental results reveal that high temperature treatment of early-age concrete multiplies the number of pores but has little influence on the change of pore size distribution, while the re-curing treatment reduces pore size but has little influence on the change of pore numbers. Under the same exposed temperature, the shear strength of concretes before heating, after heating, and re-curing after heating increases gradually with curing ages. For concretes with certain curing ages, the shear strength increases first and then decreases with the exposed high temperature. While the cohesion and internal friction angle show a negative relationship with elevated temperature. The research can provide base data for buildings encountering fire accidents.
1 Introduction
Fire has been one of the major threats to the safety of buildings. The mechanical properties of concrete after fire have become one of the research hotspots (Nadeem et al., 2014; Khan and Abbas, 2015; Laim et al., 2022). Fire accidents happen randomly and many of them happen during the construction period of concrete buildings. For example, a large fire accident happen in a reinforced concrete building in Wuhan, China only after 3 days of concreting (Wang et al., 2022). The physical-mechanical properties and durability of concrete show significant degradation after exposure to high temperature. Hence, it is crucial to understand the thermal damage behavior of early-age concrete after fire.
The thermal damage behavior of concrete has been widely investigated since the 1940s. The compression strength (Pan et al., 2018; Monte et al., 2019; Zhao et al., 2021a), thermal conductivity (Nguyen et al., 2019), composite behavior (Xotta et al., 2015), dynamic behavior (Bi et al., 2020), and microstructure change (Liu et al., 2018; Jia et al., 2019) of concrete exposed to elevated temperature have been investigated through experimental works. Previous studies have illustrated that the mechanical properties of concrete suffered significant degradation when exposed to high temperature. The degradation mechanism of concrete strength can be attributed to three reasons: 1) incompatibilities between the aggregates and the cement paste (Zhao et al., 2021b); 2) chemical changes (such as siliceous aggregates transforming from α phase to β phase) (Masse et al., 2002); 3) and moisture transport (Lim and Mondal, 2014; Ahn et al., 2016). It should be noted that these experimental works mainly focused on the thermal behavior of in-service concrete. Research on the thermal behavior and mechanical properties of early-age concrete, however, is less studied. It is well known that the microstructure and chemical composition of early-age concrete is quite different from that of concrete in service due to inadequate hydration (Wang et al., 2022). Some scholars have explored the effect of temperature on the mechanical properties of early age concretes. For example, Li et al. (Li et al., 2017) presented a comprehensive experimental study on the degradation of mechanical properties of cement-based materials at their early age after fire. Chen et al. (2009) investigated the splitting tensile strengths of early-age concretes after heating up to 800°C. Yuan et al. (2021) studied the cracking resistance of early-age concrete under elevated temperatures. Shu et al. (2021) analyzed the compressive strength and tensile strength of early-age concrete after high temperature treatment. Previous studies mainly focus on the compression or tension properties of early-age concrete. Research on the shear properties of early-age concrete, however, is very limited, especially under the effect of thermal damage. Shear failure has been detected in many concrete structure engineerings in practice. For example, tunnel lining concrete may suffer shear damage due to the rupture of surrounding rock or the excavation of an adjacent tunnel (Zhang et al., 2018; 2019; Zhao et al., 2020; Zhao et al., 2021a).
In this study, the effect of high temperature on the shear properties of early-age concrete is experimentally studied. Pore structure evolution of early-age concrete before heating, after heating, and re-curing after heating is investigated through NMR and SEM tests.
2 Experimental study
2.1 Specimens preparation
Cement-based materials were used in this study. Cubic specimens were prepared with size of 70 × 70 × 70 mm. They were made of white Portland cement, water, and standard sand. First, these materials were stirred in a cement mixer and then placed into 70 × 70 × 70 mm molds. After casting for 1 day, the mixtures were demoulded and were placed in a curing room with a constant temperature of 25 °C and humidity of 90%.
After the specimens were cured for certain days, they were exposed to high temperature to simulate fire conditions. To investigate the effects of curing ages on the shear behavior of thermal-damaged concretes, four curing ages (i.e. 3, 7, 14, and 28 days) were designed.
2.2 Test procedure
Figure 1 shows the sketch of the test procedure. After specimens were cured for certain days, NMR tests were first carried out on the early age concrete to obtain the initial pore structure. Then, these early-age concrete specimens were heated through an electrical heating furnace with a heating rate of 5 °C/min. Previous studies suggest that concretes exposed to temperature over 600 °C exhibited serious damage and the mechanical properties were degraded significantly (Li et al., 2017). Therefore, in this study, four groups of temperature (room temperature, 150°C, 350°C, and 550°C) were set. To evaluate the repairability of thermal-damaged concretes, the early-age concretes were re-cured for another 28 days. NMR tests were carried out again to investigate the pore changes induced by thermal damage and the re-curing process. Finally, direct shear tests were conducted on these thermal-damaged concretes.
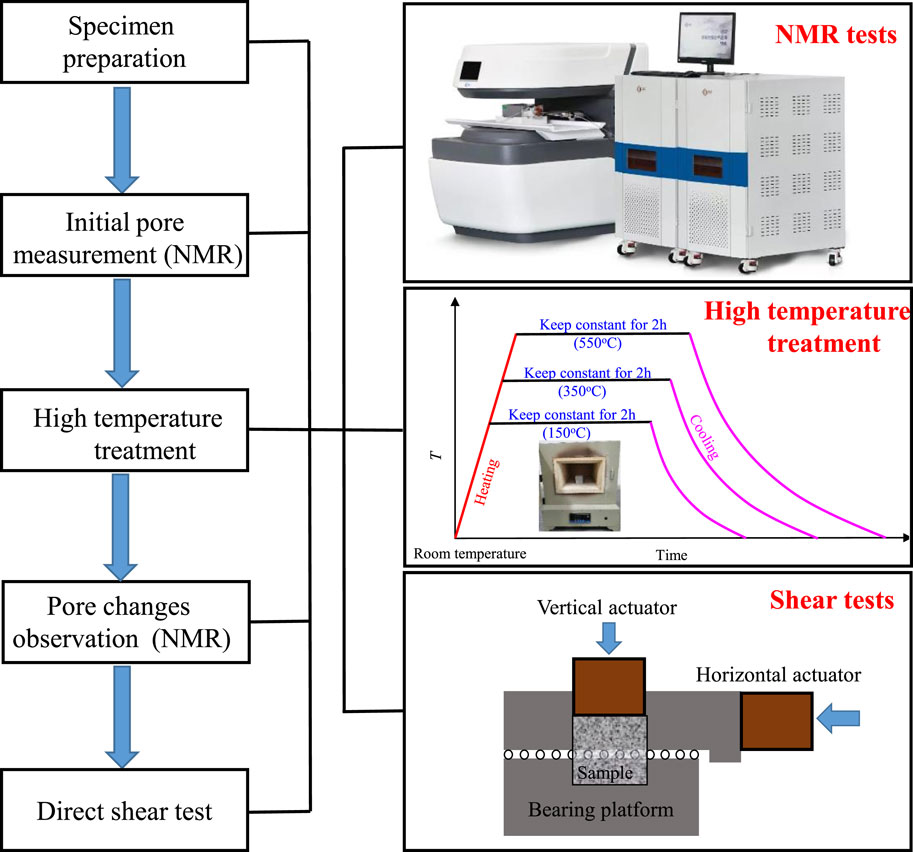
FIGURE 1. Schematic diagram of test procedure (Wang et al., 2022).
3 Experimental results and analysis
Figure 2 shows the T2 distribution of early-age concretes before heating, after heating, and re-curing after heating, respectively. All NMR tests were successively carried out on specimens with different curing ages, with the main results being exemplified by specimens with a curing age of 14 days. As shown in the figure, all T2 curves exhibited two peaks, which correspond to the micro-pores and macro-pores, respectively. High temperature causes thermal damage to early-age concrete. Compared to the initial curve, the T2 area is significantly enlarged after exposure to high temperature. Both the two T2 peaks become higher after the high temperature treatment, while the position of the peaks remains unchanged. Previous studies indicated that the T2 peak represents the number of pores and the T2 distribution reflects the form of the pore size distribution (PSD) (Frosch et al., 2000; Weng et al., 2018; Zhao et al., 2022). Therefore, high temperature treatment of early-age concrete multiplies the number of pores but has little influence on the change of PSD. Moreover, the multiple of pores increases with the exposed temperature. For example, for samples heating up to 150°C and 550°C, respectively, the amplitude of the first T2 curve increased by 29.654 and 78.822 a. u., respectively.
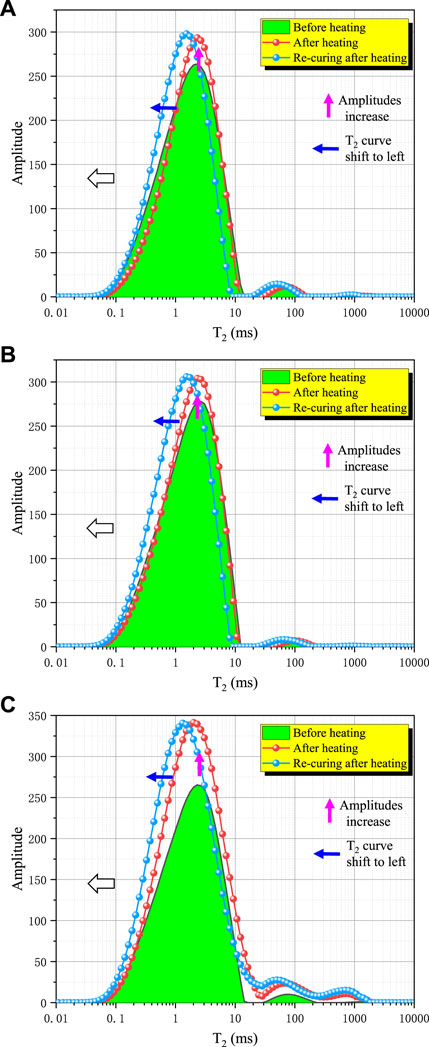
FIGURE 2. T2 distribution of early-age concretes (14 days curing ages) before heating, after heating, and re-curing after heating (A) exposed to high temperature of 150°C; (B) exposed to high temperature of 350°C; and (C) exposed to high temperature of 550°C.
After heating, the early-age concretes were re-cured for another 28 days. The T2 curve of re-cured concrete after heating is also presented in Figure 2. As shown in the figure, the T2 curve shits to left on the T2 relaxation time axis but the amplitude of the T2 peaks remains nearly unchanged. This indicates that pore size decreased after re-curing but the number of pores kept unchanged.
3.2 Effect of curing ages on shear properties
Figure 3 shows the peak shear strength of tested specimens with varied curing ages. Note that average values are presented from three identical specimens. As expected, the peak shear strength of early-age concrete before heating increases with the curing ages due to the cement hydration reaction. After exposure to different high temperatures, one group of specimens was used to carry out shear tests directly, while the other group was re-cured for another 28 days.
The peak shear strength of concretes after heating shows a similar changing trend to that of specimens before heating, i.e., the shear strength increases with curing ages. After re-curing, the peak shear strength increases to a certain level depending on the curing ages. In general, the strength recoverability decreases with the curing ages. For example, in the case of re-cured concretes after exposing to 350°C, the peak shear strength increased by 0.93, 0.6, 0.24, and 0.15 MPa for concretes with 3, 7, 14, and 28 days curing ages, respectively.
To further interpret the test results, SEM tests were carried out on concretes with different curing ages before heating, after heating, and re-curing after heating. Figure 4 shows the SEM images of the concretes before heating. With the increase of curing ages, the hydration products (such as C-S-H, CH) increase gradually, which results in the enhancement of concrete density and integration. For specimens with curing ages of 3 and 7 days, the degree of hydration is very low. There exist a lot of free water and pore voids within the material. Therefore, the flocculent structure of hydration products and the internal structure are loose (Li et al., 2017). While for specimens with curing ages of 14 and 28 days, the degree of hydration is much higher than the one with 3 and 7 days curing ages. A larger number of hydration products are generated which results in a decrease in free water and porosity. Hence, the flocculent structure of hydration products and the internal structure become denser. This provides an explanation that the peak shear strength of early-age concrete before heating increases with the curing ages.
Figure 5 shows the SEM images of the concretes after exposure to temperature of 350°C with different curing ages. Thermal-induced cracks are observed at this temperature regardless of curing ages. In the case of concretes with curing ages of 3 and 7 days, thermal-induced cracks were induced because of the loose internal structure. Although the integrity of concretes with 14 and 28 days curing ages are denser, the free water and crystal water were difficult to release when subjected to high temperature, leading to high water vapor pressure inside the specimen. As a result, thermal-induced cracks were also observed in concretes with 14 and 28 days curing ages.
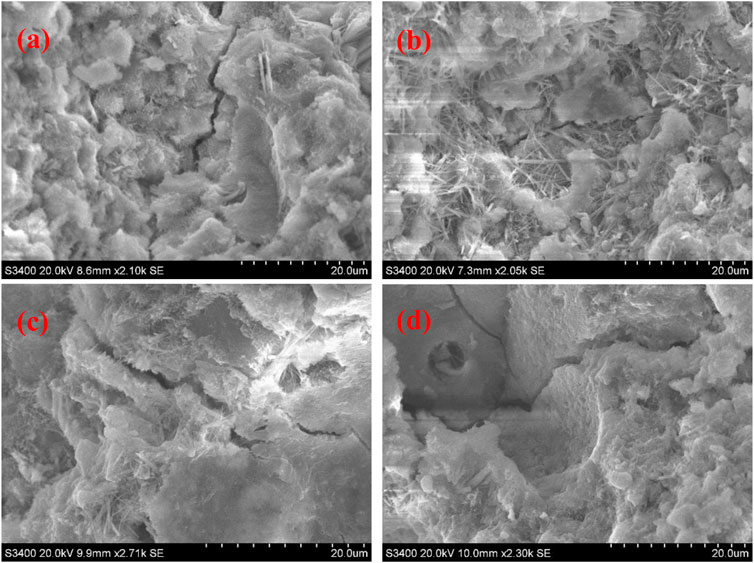
FIGURE 5. SEM images of the concretes after exposure to temperature of 350°C with different curing ages; (A) 3 days; (B) 7 days; (C) 14 days; and (D) 28 days.
Figure 6 shows the SEM image of the concretes (curing ages 7 days) re-curing after heating to 350°C. By detail examining the SEM image of the specimen, there are many remnants of hydration products within concretes after re-curing. For the specimens with curing ages less than 28 days, the degree of hydration is low. The less the curing ages before heating, the less the hydration products. After re-curing, many remnants of hydration products were generated, especially for specimens with low hydration degrees. As shown in Figure 6, these remnants of hydration products can fill the voids or cracks induced by thermal damage, hence improving the specimen strength. Since the specimen with 3 days curing ages before heating had the largest cement continuing to hydrate, the shear strength increment after re-curing was the largest compared to the concretes with other curing ages.
3.3 Effect of high temperature on shear properties
3.3.1 Peak shear strength
Figure 7 shows the peak shear strength of early-age concretes under different temperature treatments. Under the same curing ages, the peak shear strength of early-age concretes increases first and then decreases with the exposed high temperature. For example, the peak shear strength of concretes at curing ages of 14 days is 4.55 MPa before heating, which increased to 4.79 MPa when heating to 150°C. While exposed to temperature of 350°C and 550°C, the peak shear strength of early-age concretes decreased to 3.67 and 2.12 MPa, respectively.
The main characteristic of early-age concrete is inadequate hydration reaction. The effect of high temperature on the early-age concrete is twofold. On the one hand, high temperature treatment can accelerate the hydration process. Meanwhile, the evaporation of free water may dehydrated the C-S-H gel, which results in the hardening of the cement slurry, and an increase in the chemical bonding forces (Kocak and Nas, 2014; Li et al., 2021). These factors cause an increase in the peak shear strength. On the other hand, thermal damage occurs in concrete when exposed to high temperatures. High temperature can cause microstructural changes in cement pastes, and produce thermal stress which induces micro-cracking in concrete. As a result, the strength of thermal damaged concretes is less than that of unheated concretes.
In the case of early-age concretes exposed to 150°C, the thermal damage to the concrete can be ignored. As shown in Figure 8, no obvious cracks are observed on the concrete surface. While the hydration reaction is promoted and the chemical bonding forces is strengthened, leading to an increase in shear strength. In the case of early-age concretes exposed to 350°C, part of the chemical-bound water is removed. Several micro-cracks are observed on the crack surface due to high temperature. Although the accelerated hydration of cement improves the shear strength, the damage to concretes is more significant due to the thermal cracking. Therefore, the peak shear strength of early-age concrete exposed to 350°C is lower than that of unheated specimens. After exposure to 550°C, both physical and chemical bond water are released completely. Thermal-induced micro-cracks increase considerably causing macroscopic damages in early-age concretes. As shown in Figure 8, a large number of cracks are observed on the concrete surface. These cracks coalesced causing a rapid decrease in shear strength.
3.3.2 Shear strength parameters
To obtain the shear strength parameters, additional shear tests were conducted under different normal stresses. Previous studies have indicated that normal stress can considerably enhance the shear resistance of concretes (Zhang et al., 2019; Zhang et al., 2020). Experimental results show that with the increase of normal stress, the peak strength of specimens for the same temperature treatments increases linearly. The Mohr-Coulomb criterion can be used to describe the relationship.
where τf and σN represent the peak shear stress and normal stress, respectively, c and φ are the cohesion and internal friction angle, respectively.
Figure 9 shows the evolution of cohesion c with exposed temperature. With the increase of exposed temperature, cohesion c decreases linearly. Meanwhile, concretes with less curing ages exhibited more reduction of cohesion under the same thermal treatment conditions. However, this tendency was not found in the evolution of internal friction angle with exposed temperature. As shown in Figure 10, the internal friction angle decreased nonlinearly with temperature. For example, the internal friction angle decreased by 4.38%, 17.67%, and 65.9% for specimens with 14 days curing ages when the specimens were heated to 150 °C, 350 °C, and 350 °C, respectively. In general, both the nominal cohesion and internal friction angle were reduced after high temperature treatments. Therefore, thermal damage can result in deterioration of shear resistance of concrete, especially for concretes with less curing ages.
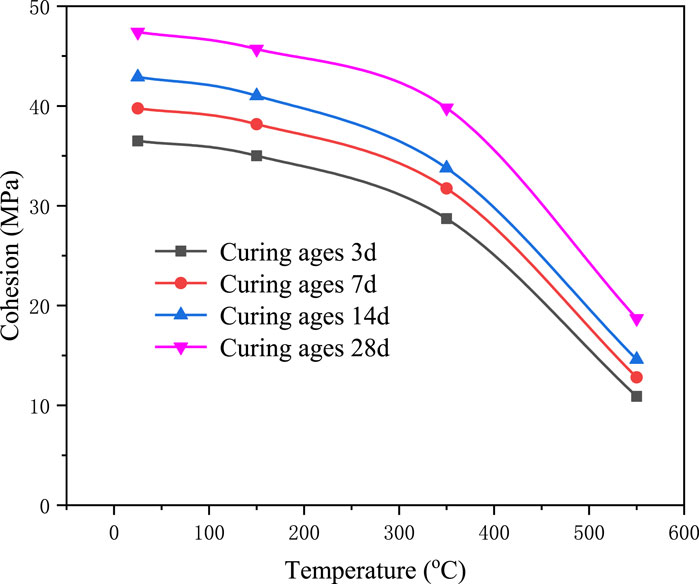
FIGURE 10. Internal friction angle of early-age concrete (14d) under different temperature treatments.
The cohesion of concretes consists of two parts: 1) interlock between cement and aggregate; 2) adhesion between the cementitious pastes and the aggregates (Zhang et al., 2019). Previous studies show that temperature below 550°C removes the physical and chemical water which mainly causes the damage of cement pastes but has little influence on the aggregates (Zhao et al., 2021a). The thermal damage destroys the cementations matrix leading to the weakening of the cement-aggregate interlock and adhesion. Hence, the concrete cohesion decreases linearly with the exposed temperature.
Internal friction angle includes two portions: contact friction and shear dilation (Wong et al., 2007). After high temperature treatment, the pore structures become loose due to the removal of physical and chemical bound water. This change of microstructure may result in a decrease in shear dilation angle and friction resistance of concrete. Such reduction increases with the exposed temperature. Hence, the concrete internal friction angle decreases non-linearly with the exposed temperature.
4 Conclusion
In this paper, a comprehensive experimental study on shear properties of early-age concretes under elevated temperature is presented. Microstructure characteristic of early-age concretes before heating, after heating, and re-curing after heating is analyzed through NMR and SEM tests. The following conclusions can be drawn.
(1) NMR test results show that the peaks of the T2 curve become higher after the high temperature treatment, but the position of the peaks remains unchanged. This indicates that high temperature treatment of early-age concrete multiplies the number of pores but has little influence on the change of pore size distribution. After re-curing, the T2 curve shits to left but the amplitude of the T2 peaks remains nearly unchanged, indicating that re-curing treatment reduces pore size of concretes but has little influence on pore numbers.
(2) Under the same exposed temperature, the shear strength of concretes before heating, after heating, and re-curing after heating increases gradually with curing ages. The peak shear strength of early-age concrete before and after heating increases with the curing ages due to the cement hydration reaction. After re-curing, many remnants of hydration products were generated, which fill the voids or cracks induced by thermal damage, hence improving the specimen strength.
(3) High temperature treatment can accelerate the hydration process but also cause thermal damage occurs in concrete. Therefore, the peak shear strength of early-age concretes increases first and then decreases with the exposed high temperature.
(4) Cohesion and international friction angle were altered due to thermal damage. Both of them decreased over exposed temperature. This can be attributed to that thermal damage destroying the cementations matrix leading to the weakening of the cement-aggregate interlock and adhesion.
Data availability statement
The raw data supporting the conclusion of this article will be made available by the authors, without undue reservation.
Author contributions
ML: Writing–original draft. FG: Conceptualization, Supervision, Writing–review and editing.
Funding
The authors declare financial support was received for the research, authorship, and/or publication of this article. This research was supported by the Guizhou Provincial Science and Technology Foundation (Nos[2021]292 and [2021]N404).
Conflict of interest
The authors declare that the research was conducted in the absence of any commercial or financial relationships that could be construed as a potential conflict of interest.
Publisher’s note
All claims expressed in this article are solely those of the authors and do not necessarily represent those of their affiliated organizations, or those of the publisher, the editors and the reviewers. Any product that may be evaluated in this article, or claim that may be made by its manufacturer, is not guaranteed or endorsed by the publisher.
References
Ahn, Y. B., Jang, J. G., and Lee, H. K. (2016). Mechanical properties of lightweight concrete made with coal ashes after exposure to elevated temperatures. Cem. Concr. Compos. 72, 27–38. doi:10.1016/j.cemconcomp.2016.05.028
Bi, J., Liu, P., and Gan, F. (2020). Effects of the cooling treatment on the dynamic behavior of ordinary concrete exposed to high temperatures. Constr. Build. Mater. 248, 118688. doi:10.1016/j.conbuildmat.2020.118688
Chen, B., Li, C. L., and Chen, L. Z. (2009). Experimental study of mechanical properties of normal-strength concrete exposed to high temperatures at an early age. Fire Saf. J. 44, 997–1002. doi:10.1016/j.firesaf.2009.06.007
Frosch, G. P., Tillich, J. E., Haselmeier, R., Holz, M., and Althaus, E. (2000). Probing the pore space of geothermal reservoir sandstones by Nuclear Magnetic Resonance. Geothermics 29, 671–687. doi:10.1016/s0375-6505(00)00031-6
Jia, Z., Chen, C., Shi, J., Zhang, Y., Sun, Z., and Zhang, P. (2019). The microstructural change of C-S-H at elevated temperature in Portland cement/GGBFS blended system. Cem. Concr. Res. 123, 105773. doi:10.1016/j.cemconres.2019.05.018
Khan, M. S., and Abbas, H. (2015). Effect of elevated temperature on the behavior of high volume fly ash concrete. Struct. Eng. 19 (6), 1825–1831. doi:10.1007/s12205-014-1092-z
Kocak, Y., and Nas, S. (2014). The effect of using fly ash on the strength and hydration characteristics of blended cements. Constr. Build. Mater 73, 25–32. doi:10.1016/j.conbuildmat.2014.09.048
Laim, L., Santiago, A., Caetano, H., Craveiro, H. D., and Shahbazian, A. (2022). Numerical analysis and structural fire design of protected SHS steel columns with thermally enhanced gypsum-based mortars. J. Build. Eng. 54, 104629. doi:10.1016/j.jobe.2022.104629
Li, Q., Liu, L., Huang, Z., and Yuan, G. (2017). Residual compressive strength of cement-based grouting material with early ages after fire. Constr. Build. Mater. 138, 316–325. doi:10.1016/j.conbuildmat.2017.02.025
Li, Q., Lu, L., Yuan, G., and Doh, J. H. (2021). Influence of curing age on the mechanical properties of fly ash concrete exposed to elevated temperature. Struct. Concr. 22, E868–E883. doi:10.1002/suco.202000155
Lim, S., and Mondal, P. (2014). Micro- and nano-scale characterization to study the thermal degradation of cement-based materials. Mater. Char. 92, 15–25. doi:10.1016/j.matchar.2014.02.010
Liu, Y., Jin, B., Huo, J., and Li, Z. (2018). Effect of microstructure evolution on mechanical behaviour of concrete after high temperatures. Mag. Concr. Res. 70 (15), 770–784. doi:10.1680/jmacr.17.00197
Masse, S., Vetter, G., Boch, P., and Haehnel, C. (2002). Elastic modulus changes in cementitious materials submitted to thermal treatments up to 1000°C. Adv. Cem. Res. 14, 169–177. doi:10.1680/adcr.2002.14.4.169
Monte, F. L., Felicetti, R., and Miah, M. J. (2019). The influence of pore pressure on fracture behaviour of Normal-Strength and High-Performance Concretes at high temperature. Cem. Concr. Comp. 104, 103388. doi:10.1016/j.cemconcomp.2019.103388
Nadeem, A., Memon, S. A., and Lo, T. Y. (2014). The performance of fly ash and metakaolin concrete at elevated temperatures. Constr. Build. Mater. 62, 67–76. doi:10.1016/j.conbuildmat.2014.02.073
Nguyen, T. D., Pham, D. T., and Vu, M. N. (2019). Thermo-mechanically-induced thermal conductivity change and its effect on the behaviour of concrete. Constr. Build. Mater. 198, 98–105. doi:10.1016/j.conbuildmat.2018.11.146
Pan, Z., Tao, Z., Cao, Y. F., Wuhrer, R., and Murphy, T. (2018). Compressive strength and microstructure of alkali-activated fly ash/slag binders at high temperature. Cem. Concr. Comp. 86, 9–18. doi:10.1016/j.cemconcomp.2017.09.011
Shu, Q. J., Lu, L. M., Yuan, G. L., Zhong, C. S., and Xu, B. (2021). Experimental investigation on the mechanical properties of early-age concrete after heating up to 550 oC. Eur. J. Environ. Civ. En. 25 (8), 1364–1378. doi:10.1080/19648189.2019.1578269
Wang, C., Zhao, H., Zhao, Y., and Bi, J. (2022). Thermal characteristics and recoverability of early-age mortar consisting of a flame retardant Mg(OH)2. J. Build. Eng. 57, 104897. doi:10.1016/j.jobe.2022.104897
Weng, L., Wu, Z., and Li, X. (2018). Mesodamage characteristics of rock with a pre-cut opening under combined static–dynamic loads: A nuclear magnetic resonance (NMR) investigation (NMR). Investig. Rock Mech. Rock Eng. 51, 2339–2354. doi:10.1007/s00603-018-1483-4
Wong, R. C. K., Ma, S. K. Y., Wong, R. H. C., and Chau, K. T. (2007). Shear strength components of concrete under direct shearing. Cem. Concr. Res. 37 (8), 1248–1256. doi:10.1016/j.cemconres.2007.02.021
Xotta, G., Mazzucco, G., Salomoni, V. A., Majorana, C. E., and Willam, K. J. (2015). Composite behavior of concrete materials under high temperatures. Int. J. Solids Struct. 64–65, 86–99. doi:10.1016/j.ijsolstr.2015.03.016
Yuan, M., Qiang, S., Xu, Y., Li, Y., and Xu, W. (2021). Research on cracking mechanism of early-age restrained concrete under high-temperature and low-humidity environment. Materials 14, 4084. doi:10.3390/ma14154084
Zhang, Z. Y., Jin, X. G., Lin, S. Z., and Bi, J. (2019). Direct shear behavior of sulfate-exposed shotcrete: experimental and modelling research. Constr. Build. Mater. 210, 607–619. doi:10.1016/j.conbuildmat.2019.03.229
Zhang, Z. Y., Jin, X. G., and Luo, W. (2018). Mechanical responses of shotcrete specimens in direct shear tests. Constr. Build. Mater. 188, 305–313. doi:10.1016/j.conbuildmat.2018.08.097
Zhang, Z. Y., Zhou, J. T., Zou, Y., Yang, J., and Bi, J. (2020). Change on shear strength of concrete fully immersed in sulfate solutions. Constr. Build. Mater. 235, 117463. doi:10.1016/j.conbuildmat.2019.117463
Zhao, Y., Ding, D., Bi, J., Wang, C., and Liu, P. (2021a). Experimental study on mechanical properties of precast cracked concrete under different cooling methods. Constr. Build. Mater. 301, 124141. doi:10.1016/j.conbuildmat.2021.124141
Zhao, Y., Wang, C., and Bi, J. (2020). Analysis of fractured rock permeability evolution under unloading conditions by the model of elastoplastic contact between rough surfaces. Rock Mech. Rock Eng. 53, 5795–5808. doi:10.1007/s00603-020-02224-x
Zhao, Y., Wang, C., Ning, L., Zhao, H. F., and Bi, J. (2022). Pore and fracture development in coal under stress conditions based on nuclear magnetic resonance and fractal theory. Fuel 309, 122112. doi:10.1016/j.fuel.2021.122112
Keywords: early age concrete, thermal effect, shear behavior, NMR, fire
Citation: Li M and Gan F (2023) Experimental study of high temperature on the shear properties of early-age concrete. Front. Mater. 10:1280057. doi: 10.3389/fmats.2023.1280057
Received: 19 August 2023; Accepted: 12 September 2023;
Published: 20 September 2023.
Edited by:
Anbang Li, Xi’an University of Architecture and Technology, ChinaReviewed by:
Peng Xie, Hainan University, ChinaYi-Xiang Song, Hebei University of Technology, China
Duofeng Cen, Ningbo University, China
Copyright © 2023 Li and Gan. This is an open-access article distributed under the terms of the Creative Commons Attribution License (CC BY). The use, distribution or reproduction in other forums is permitted, provided the original author(s) and the copyright owner(s) are credited and that the original publication in this journal is cited, in accordance with accepted academic practice. No use, distribution or reproduction is permitted which does not comply with these terms.
*Correspondence: Fei Gan, 1071384683@qq.com