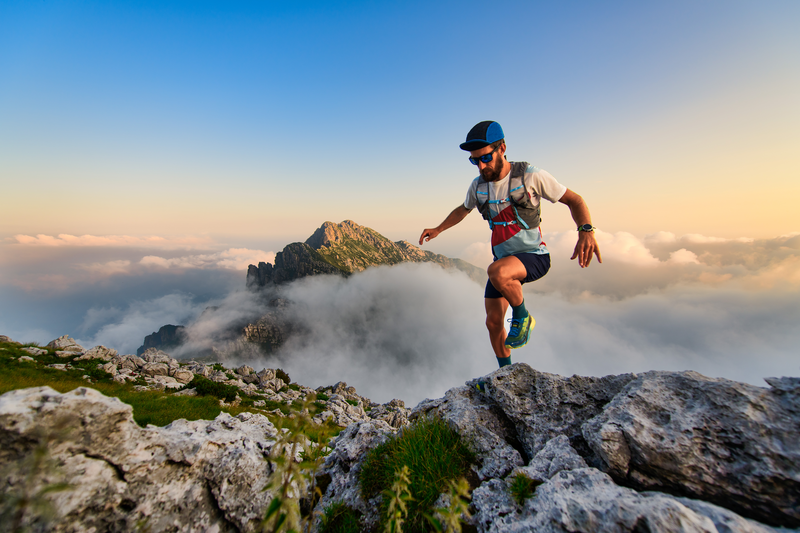
94% of researchers rate our articles as excellent or good
Learn more about the work of our research integrity team to safeguard the quality of each article we publish.
Find out more
ORIGINAL RESEARCH article
Front. Mater. , 25 August 2023
Sec. Energy Materials
Volume 10 - 2023 | https://doi.org/10.3389/fmats.2023.1247086
Downhole anticorrosion in sour gas (H2S or CO2) wells is one of the technical problems in petroleum engineering, and the corrosion law of cement paste, which is the “first barrier of the wellbore,” needs to be focused on. Aiming at the problems existing in the current research on the corrosion of underground cement paste, a curing method for interfacial corrosion is proposed. X-ray diffraction and scanning electron microscopy are used to investigate the corrosion mechanism of cement paste cured under hydrogen sulfide (H2S) conditions in natural gas wells. Experimental results showed that the corrosion depth of cement paste is proportional to the partial pressure of H2S and the corrosion time, and the compressive strength of cement paste after corrosion is inversely proportional to the H2S partial pressure value and the corrosion time. Due to the gradual enrichment, accumulation, and migration of products after the cement paste is corroded by H2S, the cement paste forms a relatively stable dense layer or corrosion transition zone. The porosity and permeability of cement paste after corrosion increased with corrosion time, showing the characteristics of first increasing and then decreasing and finally making it more difficult for the corrosive medium to enter the interior of the cement paste. It is an important method stable corrosion transition zone forms as soon as possible, which is important to maintain the long-term sealing and chemical integrity of the cement sheaths in natural gas wells containing H2S.
At present, some gas wells in domestic high-temperature deep wells, such as those at the Tarim Kela and Sichuan Luojiazhai gas fields, have annular pressure to varying degrees, which ultimately directly affects the safe development of gas wells. The content of H2S in the Feixianguan Formation gas reservoirs in Luojiazhai, Dukouhe, Tieshanpo, Wolonghe, and other gas fields in China is 10%–16%, and the H2S content in the Longgang gas field is 30–180 g/m3. The high content of H2S not only causes serious corrosion to high-strength steel underground and on the ground, but its strong toxicity also directly threatens personal safety and makes drilling and completion risks high (Xu et al., 2018; Mei et al., 2019; Mei et al., 2020). In the downhole system of formation (gas layer)-cement sheath-casing, the cement sheath is the first barrier to prevent corrosion due to H2S, CO2, and other acidic media Therefore, to solve the phenomenon that the underground pipes are corroded by acid gas, it is necessary to pay attention to the research on anti-corrosion cement slurry systems (Mei et al., 2021a; Zhang et al., 2022).
At present, domestic and foreign scholars have conducted in-depth research and discussion on the corrosion of oil well cement, and have achieved certain results in both cement multiphase (Bihua et al., 2018; Zhang et al., 2021a) and cement single mineral research (Mei et al., 2018). On this basis, a variety of CO2 and H2S resistant materials have been developed, such as geopolymer materials (Mei et al., 2021b), polymer materials (Zhang et al., 2021b), resin materials (Peng et al., 2022), supplementary cementitious materials (Zhang et al., 2013; Mei et al., 2022), and nano materials. However, for the study of anti-corrosion cement systems, it is necessary to understand the nature of the corrosion of cement paste in an acidic environment, and then we can take corresponding anti-corrosion measures to fundamentally improve the cementing quality of sour gas wells. Through the investigation of the current domestic and foreign literature on the corrosion resistance of cement paste in acidic media, it was found that the previous corrosion experiments were all carried out by immersing the cement paste sample in an acidic environment (Huang et al., 1999; Yang et al., 2001; Xu et al., 2010). In fact, the corrosion of the cement sheath downhole starts from the contact interface between the gas layer and the cement sheath, and thus not the entire cement paste is corroded at the same time, so that the previous experimental results of cement paste corroded by acid gas are not completely consistent with the actual situation.
In this study, in view of the many problems existing in the corrosion resistance of cement paste in an acid medium at present, an experimental method of interfacial corrosion is proposed. The corrosion resistance mechanism of the cement sheath interface of the Sichuan–Chongqing sour gas well was explored by testing various properties before and after corrosion with cement paste in the Sichuan–Chongqing gas field, including corrosion depth, compressive strength, permeability, and porosity. Truly reflecting the corrosion status of downhole cement in an acidic environment is of great significance for evaluating and ensuring the safety of high content of H2S/CO2 gas reservoirs in the production process and prolonging the life of gas wells.
Table 1 shows the test data of the corrosion depth of the 5-inch tailpipe cement paste (dispersant (code: FS-31L) and fluid-loss additives (coed: SD10) cement slurry system, density 1.88 g/cm3) of Well M in the Sichuan–Chongqing gas well after being corroded by H2S and CO2 gas by completely soaking the cement paste sample. Figure 1 is a comparison of the appearance of uncorroded and corroded cement paste in the H2S gas phase environment of 3 MPa. In any corrosion test containing H2S gas, the corrosion depth of the cement paste is to break down the entire cement stone, and the corrosion is quite serious. If we think that the cement sheath of the Sichuan-Chongqing high sulfur gas well will be corroded by H2S and other acidic gases over time, the consequences will be disastrous, and the actual situation of the sulfur gas well in Sichuan-Chongqing gas field is also different. The cement sheath in the gas well is not corroded in all directions at the same time, but only the interface between the gas layer and the cement sheath is subject to continuous acid corrosion.
TABLE 1. Corrosion depth data of 5-inch liner cement paste by acid gas in Sichuan–Chongqing Well M (The diameter of cement paste was 25 mm; the total pressure of the corrosion test was 10 MPa).
FIGURE 1. Corrosion comparison diagram of cement paste under H2S phase environment with a pressure of 3 MPa.
In summary, the concept of interfacial corrosion is proposed in this paper, with the following experimental scheme. The main corrosive media in sour gas wells in the Sichuan–Chongqing gas field are H2S and CO2 (Ding et al., 2009; Huang et al., 2010), so these two acid gases were mainly considered in the experimental plan.
(1) The cement slurry was prepared and maintained on site according to the API specification, and cured for 7 days under the conditions of 90°C and 20.7 MPa After the end, the core (Φ25 mm × 50 mm) was taken out to prepare the cement paste sample;
(2) The cement paste sample was put into a corrosion-resistant mold with an inner diameter of 26 mm and a length of 52 mm, and epoxy resin was used to seal the uncontacted parts of the cement paste and the mold (to ensure the tightness between the cement paste and the mold, and to simulate the cementation of the interface between the formation and the cement sheath), and the end face of the cement paste was polished with sandpaper, as shown in Figure 2.
(3) The cement sample with corrosion-resistant mold was put into a high-temperature and high-pressure corrosion apparatus for corrosion experimentation. The experiment used 7-inch liner cement slurry (FS-31L + SD10 cement slurry system, density 1.90 g/cm3) of the Sichuan-Chongqing gas field X gas well onsite sampling (hereinafter referred to as the designated cement paste). The experimental conditions are shown in Table 2.
A scanning electron microscope (SEM) was used to observe the changes in surface morphology of the corroded cement and analyze the evolution of the internal structure of the cement. The test method was as follows: the end face and internal area of the dried corroded sample were maked into small flake samples, and then the block sample was glued to the sample base with conductive adhesive. The fresh section of the sample was sprayed with gold by ion sputtering and placed into the scanning electron microscope for observation. The working voltage was 5 kV and the emission current was 10 μA.
The phase change of the corroded cement paste was tested by X-ray diffraction analysis (XRD). The test process was as follows: the end faces of cement paste samples corroded for 7 and 14 days were selected and ground into powder of about 325 meshes with mortar. They were then put into the diffractometer with the scanning step length set at 0.04°, each step time set at 1 s, and the diffraction angle range set at 5°–80°.
Figure 3 shows the appearances of the specified cement pastes from different corrosion environments. Under the condition of the interfacial corrosion of cement pastes, the surface corrosion of cement paste can be clearly observed with the naked eye, which is completely different from the breakdown result obtained by the complete invasion of cement paste (as shown in Figure 1). The interfacial corrosion only partially corroded the end face of the cement paste. The experimental data observed and tested the depth of the corrosive components’ intrusion into the cement paste by using a stereo microscope according to the change of the color of the cement paste after corrosion.
Figure 4 is the corrosion depth of cement paste cured under different conditions. With the increase of H2S partial pressure, the penetration depth of corrosive components increases. Corrosion depth increases with corrosion time and, after the cement paste was corroded in the formation water environment for 7 days and cured for 14 days, the corrosion depth hardly changed, which was related to the less corrosive medium of the acidic formation water.
Figure 5 is the change of the compressive strength of cement paste after 7 and 14 days of interfacial corrosion in water-wet H2S and carbon dioxide composite acid gas environment and acid formation water. With the increase in corrosion time, the compressive strength of cement pastes showed a downward trend and, with the increase in H2S partial pressure, the compressive strength of cement pastes decreased (the decrease of compressive strength means the increase of corrosion degree). For group H3C1-10, the compressive strength of the cement sample decreased by 46.81% after 7 days of corrosion and 55.74% after 14 days of corrosion. For group H1.7C1-10, the compressive strength of the cement sample decreased by 20.43% after 7 days of corrosion and 31.06% after 14 days of corrosion. It is worth noting that the corrosion rate of cement paste was weakened after 7 days of corrosion. Under the condition of acidic formation water, the strength value increased after 7 days of corrosion. This may be because the formation water contained less acidic corrosion medium. After 7 and 14 days, acidic formation water mainly played a role in the maintenance of cement paste, with the extension of time, the strength of the cement paste still showed a trend of decreasing strength under the condition of acid formation water immersion.
To explore the mechanism of the effect of corrosion on the mechanical properties of cement paste, this study tested the changes in porosity and permeability of cement paste after corrosion under different conditions. The experimental results are shown in Figures 6, 7. As shown in Figure 6, for the group H3C1-10, the porosity of cement paste decreased by 56.40% after 7 days of corrosion, and by 63.37% after 14 days of corrosion. For the group H1.7C1-10, the porosity of cement paste decreased by 29.65% after 7 days of corrosion, and 44.19% after 14 days of corrosion. For A-0.1, the porosity of cement paste decreased by 3.49% after 7 days of corrosion, and by 18.02% after 14 days of corrosion. As observed in Figure 7, the permeability exhibits the same variation pattern as the porosity. The above analysis shows that the partial pressure of H2S had a significant impact on the changes in porosity and permeability of cement. In addition, the porosity and permeability of cement pastes after acid gas corrosion decreased, which is opposite to the increase of porosity and permeability of cement pastes after corrosion described in the relevant literature (Guo et al., 2004; Ma et al., 2008; Yan et al., 2010; Zhang et al., 2010; Gu et al., 2017; Wang et al., 2020). This is because during the end face corrosion process, the corrosion rate slows down compared to the complete immersion corrosion in previous studies, and the formed corrosion products fill the microcracks and micropores in the cement paste during transportation. At the same time, some of the corrosion products formed have expansibility, compressing the pores and cracks in the cement paste, thereby exhibiting a decrease in porosity and permeability. However, due to the production of corrosion products that do not possess cementitious and expansive properties during the corrosion process, the original dense structure of cement paste is destroyed, resulting in a decrease in the compressive strength of cement pastes.
To further verify the above inference, this study used the H3C1-10 group of cement paste samples with the most severe corrosion as an example to analyze the phase composition and microstructure of the corroded cement pastes using X-ray diffraction and scanning electron microscopy. Figures 8, 9 are the X-ray diffraction results after 7 and 14 days of cement-paste interface corrosion under CO2 with a partial pressure of 1 MPa and H2S with a partial pressure of 3 MPa. Analysis of Figures 8, 9 showed that, after the specified cement-paste interface corrosion, the main phases were SiO2, AFt, C-S-H gel, CaCO3, CaSO4∙2H2O, CaS, etc. (Feng et al., 2018; Sun, 2019; Zhang et al., 2019; Zhao et al., 2019; Yue et al., 2020; Wu et al., 2021). Its reaction equation is as follows:
Figure 10 is the micromorphology of the cement paste interface after 7 and 14 days of corrosion at the cement paste interface in the environment of 1 MPa CO2 and 3 MPa H2S, respectively. When compound acid gas corrodes cement paste, the volume of intumescent corrosion products CaCO3, CaS, and CaSO4∙2H2O increases. In the corrosion layer on the cement paste surface, the Ca(OH)2 component is completely corroded, and its product CaSO4∙2H2O is transported to the cement paste surface with the progress of the reaction. It is precisely because of the appearance and migration of these expansive corrosion products that the surface of the corroded cement paste is denser than the interior of the cement paste. The surface of the corroded cement paste is covered with a layer of corrosion products, and the coating layer is dense. Below the dense coating layer is the internal structure of the cement paste. By comparing the microstructure of the surface and interior of cement pastes corroded for 7 and 14 days, it could be concluded that that the internal structure of the corroded cement paste was relatively loose compared to the dense layer, and some larger pores could be observed. These pores may have been left by the reaction between the corrosion medium and the hydration products in the cement paste. At the same time, the reacted corrosion products are transported to the surface of the cement paste through the pores and gel pores, and finally, a dense coating layer is formed on the surface of the cement paste. This also explains the decrease in porosity and permeability of cement pastes after corrosion.
By analyzing and summarizing the results of the abovementioned experiments, the mechanism of the corrosion of the cement paste under the sulfur-containing gas wells in the Sichuan–Chongqing area by H2S, CO2 and other acid gases is described as follows:
Under the sour gas well, in the horizontal direction, acid gases such as H2S and CO2 move from the interface between the production layer and the cement sheath to the direction of the cement sheath and casing. In the vertical direction, from the interface between the production layer and the cement sheath to the upper part of the gas well, the cement sheath is corroded. In the complete corrosion zone on the contact surface between the cement sheath and the gas layer, due to the chemical reaction between the acid gas and the cement sheath, the compactness of the cement sheath is destroyed, resulting in an increase in the porosity and permeability of the corroded surface of the cement sheath. With the passage of time, the acidic corrosion medium continues to act inside the cement sheath, and its corrosion products SiO2, AFt, CaCO3, CaSO4∙2H2O, and CaS migrate to the surface by using the pores in the completely corroded zone on the surface of the cement sheath, gradually forming a dense transition zone. This dense transition zone blocks pores due to the accumulation of many corrosion products, which reduces the porosity and permeability here instead of increasing, and finally makes it difficult for acidic corrosion media such as H2S and CO2 to continue to corrode the cement sheath laterally and longitudinally.
Through the experimental method of interfacial corrosion, the mechanism of downhole cement paste being corroded by acid gases such as H2S and CO2 was analyzed, and the following conclusions and recommendations were drawn:
(1) The partial pressure value and corrosion time of H2S are in direct proportion to the corrosion depth of cement paste; the partial pressure value and corrosion time of H2S are inversely proportional to the compressive strength value of cement paste after corrosion;
(2) The main phases of the cement paste after being corroded by acid gas are SiO2, AFt, CaCO3, CaSO4∙2H2O, and CaS, etc. The corrosion products gradually enrich, accumulate, and migrate, so that the cement paste forms a relatively stable dense layer or corrosion transition zone, which leads to a decrease of the porosity and permeability of the cement paste after corrosion, and finally makes it difficult for the external corrosive medium to continue to migrate into the cement paste;
(3) For the first time, the method of interfacial corrosion was proposed to study the corrosion resistance mechanism of cement sheaths in sour gas wells. This method can be used as the basis for evaluating the corrosion resistance of cement sheaths;
(4) Making the cement sheath form a relatively stable corrosion transition zone as soon as possible, or reducing the corrosion rate of the cement sheath, while maintaining a certain corrosion depth, is an important method to maintain the long-term sealing and chemical integrity of the mud sheaths in sour gas wells.
The original contributions presented in the study are included in the article/Supplementary Material, further inquiries can be directed to the corresponding author.
CS: conceptualization, investigation, writing-original draft, and data curation. YZ: validation, resources, writing—review and editing, supervision. LC: validation, formal analysis, investigation, and data curation. YF: formal analysis and project administration. JY: formal analysis and project administration. JC: methodology and conceptualization. All authors contributed to the article and approved the submitted version.
This work was supported by the key R&D project of Sichuan Province, “New Technology for Harmless Utilization of Oil Base Cuttings in Shale Gas Wells,” project number 2021YFS0278, and the project of PetroChina Southwest Oil and Gas Field Branch, “Study on the Evaluation Method of Self-healing Cement Slurry,” project number: 20220302-02.
Authors CS, YZ, LC, YF, and JY were employed by PetroChina Southwest Oil and Gas Field Company.
The remaining author declares that the research was conducted in the absence of any commercial or financial relationships that could be construed as a potential conflict of interest.
All claims expressed in this article are solely those of the authors and do not necessarily represent those of their affiliated organizations, or those of the publisher, the editors and the reviewers. Any product that may be evaluated in this article, or claim that may be made by its manufacturer, is not guaranteed or endorsed by the publisher.
Bihua, X., Bin, Y., and Yongqing, W. (2018). Anti-corrosion cement for sour gas (H2S-CO2) storage and production of HTHP deep wells. Appl. Geochem. 96, 155–163. doi:10.1016/j.apgeochem.2018.07.004
Ding, S., Zhou, S., and Lei, C. (2009). Supporting cementing technology for high temperature, high pressure and high sulfur gas wells in Northeast Sichuan [J]. Nat. Gas. Ind. 29 (2), 58–60.
Feng, F., Liu, Z., Lu, D., Zhang, D., pan, R., Yan, M., et al. (2018). Research progress on the influence of CO2 on the corrosion mechanism and sealing of cement paste [J]. J. Silic. 46 (02), 247–255.
Gu, T., Guo, X., Li, Z., Cheng, X., Fan, X., Korayem, A., et al. (2017). Coupled effect of CO2 attack and tensile stress on well cement under CO2 storage conditions. Constr. Build. Mater. 130, 92–102. doi:10.1016/j.conbuildmat.2016.10.117
Guo, Z., Zhao, Q., and Yan, P. (2004). Study on the corrosion resistance of cementing stone[J]. Drill. Complet. Fluids 21 (6), 37–40.
Huang, B., Lin, E., and Lv, G. (1999). Research on the corrosion of cemented ring column[J]. Oilfield Chem. 16 (4), 377–383.
Huang, H., Liu, A., and Chen, G. (2010). Research on cementing technology of "three high" gas wells in Sichuan-Chongqing gas region [J]. Nat. Gas. Ind. 30 (4), 70–73.
Ma, K., Zhou, S., and Chu, Y. (2008). Study on the mechanism of H2S gas corrosion on cement stone at high temperature [J]. Pet. Drill. Technol. 36 (6), 4–8.
Mei, K., Cheng, X., Gu, T., Zheng, Y., Gong, P., Li, B., et al. (2021a). Effects of Fe and Al ions during hydrogen sulphide (H2S)-induced corrosion of tetracalcium aluminoferrite (C4AF) and tricalcium aluminate (C3A). J. Hazard. Mater. 403, 123928. doi:10.1016/j.jhazmat.2020.123928
Mei, K., Cheng, X., Pu, Y., Ma, Y., Gao, X., Yu, Y., et al. (2020). Evolution of silicate structure during corrosion of tricalcium silicate (C3S) and dicalcium silicate (C2S) with hydrogen sulphide (H2S). Corros. Sci. 163, 108301. doi:10.1016/j.corsci.2019.108301
Mei, K., Cheng, X., Zhang, H., Yu, Y., Gao, X., Zhao, F., et al. (2018). The coupled reaction and crystal growth mechanism of tricalcium silicate (C3S): an experimental study for carbon dioxide geo-sequestration wells. Constr. Build. Mater. 187, 1286–1294. doi:10.1016/j.conbuildmat.2018.08.080
Mei, K., Cheng, X., Zhang, L., Guo, X., Zhuang, J., and Zhang, C. (2019). Self-healing mechanism of Zn-enhanced cement stone: an application for sour natural gas field. Constr. Build. Mater. 227, 116651. doi:10.1016/j.conbuildmat.2019.08.032
Mei, K., Gu, T., Zheng, Y., Zhang, L., Zhao, F., Gong, P., et al. (2021b). Effectiveness and microstructure change of alkali-activated materials during accelerated carbonation curing. Constr. Build. Mater. 274, 122063. doi:10.1016/j.conbuildmat.2020.122063
Mei, K., Zhang, L., Wang, Y., Cheng, X., Xue, Q., Gan, M., et al. (2022). Structural evolution in micro-calcite bearing Ca-montmorillonite reinforced oil well cement during CO2 invasion. Constr. Build. Mater. 315, 125744. doi:10.1016/j.conbuildmat.2021.125744
Peng, Z., Lv, F., Feng, Q., and Zheng, Y. (2022). Enhancing the CO2-H2S corrosion resistance of oil well cement with a modified epoxy resin. Constr. Build. Mater. 326, 126854. doi:10.1016/j.conbuildmat.2022.126854
Sun, G. (2019). Experimental study on the performance of cement paste in acid gas corrosive environment [J]. Petrochem. Technol. 26 (10), 101–102.
Wang, S., Yao, M., He, X., Wu, B., Liu, L., Wang, S., et al. (2020). Corrosion evolution of a concrete/casing steel in simulated formation water under different CO2 partial pressures. Int. J. Electrochem. Sci. 15, 9948–9970. doi:10.20964/2020.10.40
Wu, Z., Yue, J., Xie, R., Xing, X., and fan, B. (2021). Study on ultra high temperature corrosion resistance and gas channeling prevention cementing slurry system in the South China Sea [J]. Petrochem. Appl. 40 (09), 47–51.
Xu, B., Song, M., and Li, S. (2010). etc. Research on salt resistance and CO2/H2S corrosion resistance of cement stone [J]. Drill. Complet. Fluids 27 (5), 58–60.
Xu, B., Yuan, B., Wang, Y., and Zhu, L. (2018). H2S-CO2 mixture corrosion-resistant Fe2O3-amended wellbore cement for sour gas storage and production wells. Constr. Build. Mater. 188, 161–169. doi:10.1016/j.conbuildmat.2018.08.120
Yan, S., Dai, Z., and Pei, G. (2010). Analysis on the corrosion of gas well cementing cement by gaseous carbon dioxide [J]. Nat. Gas. Ind. 30 (9), 55–59.
Yang, Y., Guo, X., Zhang, Y., Chen, Y. J., Lin, C. H., Huang, Z. D., et al. (2001). Frequent gain of copy number on the long arm of chromosome 3 in human cervical adenocarcinoma. Nat. Gas. Ind. 21 (2), 48–53. doi:10.1016/s0165-4608(01)00510-6
Yue, J., Wu, Z., Wang, X., Huang, W., Yang, X., Zhao, Q., et al. (2020). Cement stone anti-H2S/CO2 corrosion mechanism and prevention measures [J]. Contemp. Chem. Ind. 49 (09), 2033–2036.
Zhang, C., Zhang, J., and Peng, B. (2010). The depth and prediction model of CO2 corrosion of oil well cement [J]. J. Silic. 38 (9), 1782–1787.
Zhang, J., Wang, C., and Peng, Z. (2021b). Corrosion integrity of oil cement modified by environment responsive microspheres for CO2 geologic sequestration wells. Cem. Concr. Res. 143, 106397. doi:10.1016/j.cemconres.2021.106397
Zhang, L., Dzombak, D. A., Nakles, D. V., Hawthorne, S. B., Miller, D. J., Kutchko, B. G., et al. (2013). Characterization of pozzolan-amended wellbore cement exposed to CO2 and H2S gas mixtures under geologic carbon storage conditions. Int. J. Greenh. Gas. Control. 19, 358–368. doi:10.1016/j.ijggc.2013.09.004
Zhang, X., Zheng, Y., Guo, Z., Ma, Y., Wang, Y., Gu, T., et al. (2021a). Effect of CO2 solution on Portland cement paste under flowing, migration, and static conditions. J. Nat. Gas. Sci. Eng. 95, 104179. doi:10.1016/j.jngse.2021.104179
Zhang, Y., song, X., Xu, M., Wang, X., and Liu, R. (2019). Assessment for sustainable use of quarry fines as pavement construction materials: part II-stabilization and characterization of quarry fine materials. Appl. Chem. Ind. 48 (10), 2450–2455. doi:10.3390/ma12152450
Zhang, Y., Xu, M., Song, J., Wang, C., Wang, X., and Hamad, B. A. (2022). Study on the corrosion change law and prediction model of cement stone in oil wells with CO2 corrosion in ultra-high-temperature acid gas wells. Constr. Build. Mater. 323, 125879. doi:10.1016/j.conbuildmat.2021.125879
Keywords: natural gas well containing H2S, corrosion depth, corrosion mechanism, porosity, permeability
Citation: She C, Zheng Y, Chen L, Fan Y, Yang J and Cai J (2023) Corrosion law of cement paste under hydrogen sulfide conditions in natural gas wells. Front. Mater. 10:1247086. doi: 10.3389/fmats.2023.1247086
Received: 25 June 2023; Accepted: 07 August 2023;
Published: 25 August 2023.
Edited by:
Kunal Mondal, Idaho National Laboratory (DOE), United StatesReviewed by:
Arnab Bose, Boehringer Ingelheim, United StatesCopyright © 2023 She, Zheng, Chen, Fan, Yang and Cai. This is an open-access article distributed under the terms of the Creative Commons Attribution License (CC BY). The use, distribution or reproduction in other forums is permitted, provided the original author(s) and the copyright owner(s) are credited and that the original publication in this journal is cited, in accordance with accepted academic practice. No use, distribution or reproduction is permitted which does not comply with these terms.
*Correspondence: Jingxuan Cai, NzI0NTQ0MTgzQHFxLmNvbQ==
Disclaimer: All claims expressed in this article are solely those of the authors and do not necessarily represent those of their affiliated organizations, or those of the publisher, the editors and the reviewers. Any product that may be evaluated in this article or claim that may be made by its manufacturer is not guaranteed or endorsed by the publisher.
Research integrity at Frontiers
Learn more about the work of our research integrity team to safeguard the quality of each article we publish.