- 1School of Traffic and Transportation Engineering, Changsha University of Science and Technology, Changsha, China
- 2Airport Engineering Research Center of CAAC, Beijing, China
- 3School of Civil Engineering, Hubei Engineering University, Xiaogan, China
- 4Hunan Expressway Maintenance Engineering Co, Ltd, Changsha, China
- 5Henan College of Transportation, Zhengzhou, China
- 6Guangxi Key Laboratory of Road Structure and Materials, Nanning, China
- 7Department of Civil Engineering, Chongqing Jiaotong University, Chongqing, China
Deicing agents are widely used to remove ice and snow on airport pavement, ensuring air traffic operation and safety. However, excessive spraying may affect the performance of airport pavement. The effects of different chloride deicing agents on the performance of airport asphalt and mixture have been assessed by previous studies. The three deicing agents (NaCl, KHF-1A, and NW056) were selected. Conducting various tests to examine their influence on the performance of asphalt and asphalt mixture. The tests include the DSR test, BBR test, indirect tensile test, bending test of small beam, and freeze-thaw splitting test. The results indicate that the asphalt anti-rutting factor G*/sinδ varies by 25%–45%, the asphalt creep stiffness increases by 15%–50%, and the anti-fatigue factor (G* sinδ) increases by 10%–40% under the effect of the deicing agents. The splitting strength of the mixture decreases by 10%–40%, the bending stiffness modulus increases by 6%–20%, and the freeze-thaw splitting strength ratio does not change significantly under the effect of the deicing agents. NW056 deicing agent has minimal effect on the performance of airport pavement and has optimal performance. The replacement of traditional chloride deicing agents helps to prolong the service life of airport asphalt pavement.
1 Introduction
According to the Civil Aviation Administration of China, China had 248 domestic transport airports by the end of 2021 (Civil Aviation Administration of China, 2022). The ‘National Comprehensive Three-dimensional Transportation Network Planning Outline’ suggests that the number of national civil transport airports will increase to about 400 from 2021 to 2035 (Ministry of Transport of China, 2021). This means China must construct 10 new airports yearly for the next 15 years. Therefore, airport planning and construction remain a fundamental challenge at this stage. Airport runways can be made of cement or asphalt pavement materials. Asphalt pavement has become a common choice for building new airports and reconstructing old runways because of its advantages in viscoelasticity, construction, maintenance, and later paving (Qiu and Nixon, 2008; Koščák et al., 2020). However, asphalt pavement also poses a security risk for airport runway operation during ice and snow events, affecting flight safety and efficiency. One of the effective methods to remove snow and prevent icing on airports and highways is to spray deicing agents (Hanke et al., 2019; Vignisdottir et al., 2019).
The effects of deicers on airport pavement have been widely investigated in foreign literature. For example, Nazari et al. reported that deicers could cause deterioration of asphalt performance (Nazari and Shi, 2019), such as reduced low-temperature performance and viscosity, as well as damage to aggregate and surface structure, leading to decreased pavement elasticity and skid resistance. At lower temperatures, calcium chloride and magnesium chloride were more effective than sodium chloride, but they were more difficult to handle, more expensive, and more damaging to infrastructure (Du et al., 2019). Ylva Edwards found that the duration, temperature, and concentration of deicer exposure mainly influenced the change in pavement performance (Edwards and Aurstad, 2000). Fay Laura et al. explained that the performance degradation of pavement materials caused by deicing agents was due to the physical and chemical reactions between the ions of deicing agents and the materials, resulting in alterations in the internal structure of the materials (Shi et al., 2009; Fay and Shi, 2011). The most traditional and common airport pavement deicers were chloride salts, such as sodium chloride, calcium chloride, and magnesium chloride (Hanke et al., 2019; Terry et al., 2020; Gruber et al., 2023a). Although they could ensure the safety of the pavement in winter conditions, they also caused severe corrosion to the pavement structure and airport facilities (Fischel, 2001; Fay and Shi, 2012; Peng et al., 2023). Previous studies have shown that chloride ions could penetrate the pavement, causing pavement cracking, increased permeability, and reduced compressive strength, ultimately leading to a significant decrease in pavement performance (Shi et al., 2014; Das and Pradhan, 2019; Gruber et al., 2023b). Sodium ions could reduce soil permeability and aeration, and affect plant growth, and 10%–60% of sodium chloride would accumulate in groundwater, affecting the environment (Findlay and Kelly, 2011).
Compared with highway asphalt pavement, the smoothness of airport pavement was higher, and the deicer residue lasted longer, which led to the damage and shedding of pavement structure and seriously affected the safety of aircraft taking off and landing. Because of the severe corrosion and environmental damage caused by chloride deicers to the pavement, chlorine-free deicers have been increasingly studied (Fortin et al., 2014). Among them, acetate has received considerable attention from researchers because its aqueous solution has a much lower freezing point than chloride salt solution and has minor corrosion to metal structures and pavement infrastructure (Frolova et al., 2015; Meng et al., 2023). Acetate products have been widely used as alternative deicers to control ice and snow on airport pavements and bridges (Terry et al., 2020). However, although acetate deicing agent is generally considered less damaging to asphalt than traditional chloride salt, it still has some adverse effects. So far, few studies have investigated the influence of acetate deicing agents on the overall performance of airport pavement, and the mechanism of action is far from clear (Zhang et al., 2021a). Hence, it is vital to examine the effect of acetate deicing agents on the performance of asphalt and asphalt mixture.
In this study, the commonly used chlorine salt deicer NaCl and two main acetate deicers NW056h and KHF-1A3 (the main components of the two are the same, but the types of additives and active ingredients are different, and their deicing performance is also quite different) are selected. Based on the environmental parameters of the airport asphalt pavement, the paper conducts low-temperature and fatigue performance tests of asphalt, and high-temperature, low-temperature, and water stability performance tests of the mixture. The paper aims to comprehensively and systematically study the effect of deicing agents on airport pavement, verify the feasibility of using NW056 and KHF-1A as alternatives to traditional chloride salt deicers, and provide references for selecting deicing agents for airport snow removal, thereby extending the service life of asphalt pavements.
2 Materials and methods
2.1 Materials
2.1.1 Deicing agents
The conventional chloride salt deicer NaCl was chosen as the control group for comparison with KHF-1A and NW056. The technical indexes of the deicing agents are presented in Table 1.
2.1.2 Asphalt mixture
This experiment followed the “Technical Specification for Construction of Asphalt Mixture Pavement of Civil Airport” (MH/T 5011–2019) (Civil Aviation Administration of China, 2019) and used Karamay 110 matrix asphalt and 6% Yuehua SBS modifier to prepare modified asphalt. The performance test results of the modified asphalt are shown in Table 2. The aggregate was basalt gravel, with coarse aggregate sizes of 15–10 mm and 5–10 mm and fine aggregate sizes of 0–5 mm. The mineral powder was alkaline limestone powder, and the fiber was polyacrylonitrile. The aggregate, mineral powder, and fiber properties met the specification requirements. The SMA-13 gradation range satisfies the specification requirements (Accompanying diagram 1), and the optimal oil-stone ratio is 5.9%. Table 3 presents the test results of the asphalt mixture performance.
2.2 Laboratory tests
Laboratory tests were conducted to study the deicing agents of influence on airport asphalt pavement performance, as shown in Figure 1. The mass fractions of the deicing agent were chosen based on the following considerations: Feng et al. suggested that when the mass fraction of the salt solution was less than 3%, the freeze-thaw process was the dominant factor for the deterioration of the mixture performance (Feng et al., 2010). When the mass fraction exceeded 3%, the salt solution was the main factor for the decline in the mixture performance. Zhang et al. indicated that the optimal mass fraction of NaCl deicing agent solution was about 10%. The maximum solubility of NaCl solution at room temperature was 35.9 g, and the corresponding mass fraction was about 26% (Zhang et al., 2014; Zhang and Zhang, 2015; Zhang et al., 2019). Therefore, 2%, 10%, 20%, and 26% were selected as the critical mass fractions for preparing the deicing agent.
The deicing agent solutions were prepared: three different deicing agents were dissolved in distilled water to obtain solutions with mass fractions of 0%, 2%, 10%, 20%, and 26%. They were stored at room temperature and covered with plastic wrap at the cup mouth to prevent leakage and evaporation of the deicing agent. Then, a laboratory test was conducted to study the effects of the deicing agent on the performance of the asphalt and asphalt mixture.
2.2.1 Asphalt performance tests
The asphalt performance test was conducted as follows: a thin film oven test was used to prepare a thin layer sample with a thickness of about 0.1 cm. Then, the deicing agent was poured over the asphalt sample until it was 1 cm above the surface, and the mixture was stirred well and left for 30 min. Next, the model was placed in the experimental environment (heat preservation/soaking) for a specific time, and then the performance of the treated specimen was tested. The laboratory test of the effect of the deicing agent on asphalt performance is shown in Table 4.
2.2.2 Asphalt mixture performance tests
The asphalt mixture was prepared according to the design gradation. The molded specimen was immersed in the deicing agent solution until the immersion was completed, and then the mixture performance test was performed. The laboratory test of the effect of the deicing agent on the mixture performance is shown in Table 5.
Several foreign research institutions, including the National Academy of Sciences, the Academy of Engineering, the Transportation Research Council, and the New Jersey Department of Transportation, conducted studies on the relationship between rutting tests and Indirect Tensile (IDT) strength. The results of these studies revealed a strong correlation between IDT strength and rutting resistance. The IDT test was recommended as a simple method to evaluate the rutting resistance of asphalt mixtures in the 2004 Traffic Research Notice titled “Simple Test Method for Asphalt Mixture Performance.” This method was later included in the US "Asphalt Mixture Performance Specification” in 2016 (Button et al., 2004; Christensen et al., 2004; McCarthy et al., 2016). For Marshall specimens, the best correlation between IDT strength and rutting results was achieved at a temperature of 40°C and a loading rate of 50 mm/min. This mechanical index could be used to evaluate the high-temperature performance of asphalt mixtures (Christensen and Bonaquist, 2002; Bennert et al., 2018).
3 Results and discussion
3.1 Effect of deicer on rheological properties of asphalt
3.1.1 High-temperature performance
DSR tested the shear rheological properties of asphalt film samples after high-temperature insulation for 96 h. The DSR test used a strain control of 10%, an angular velocity of 10 rad/s, a test plate diameter of 25 mm, and a gap of 1,000 μm. The changes of asphalt anti-rutting factor G*/sinδ and phase angle δ with temperature after treatment with different deicing agent solutions are shown in Figure 2.
The treatment of the deicing agent solution reduced the phase angle δ of asphalt by 1.5°–5°. It increased the anti-rutting factor G*/sinδ by 25%–45%, indicating that the deicing agent significantly improved the high-temperature performance of asphalt. This was because the soluble salt in the deicing agent solution penetrated the asphalt. The ions further immersed in the asphalt molecule, which reduced the content of light components in the asphalt, significantly reduced the bonding between the asphalt molecules, reduced the shear rheological properties of the asphalt, and increased the anti-rutting factor (Xiong et al., 2019; Zhang et al., 2021a; Meng et al., 2021). However, this change also enhanced the high-temperature deformation ability of the asphalt to some extent, but it significantly weakened its fatigue performance.
The influence of the acetate deicing agent on the asphalt phase angle and anti-rutting factor was greater than that of the chloride deicing agent. When the mass fraction was 10%, the asphalt phase angle was NaCl > NW056 > KHF-1A, and the anti-rutting factor was NaCl < NW056 < KHF-1A. When the mass fraction was 26%, the phase angle was NaCl > KHF-1A > NW056, and the anti-rutting factor was NaCl < NW056 < KHF-1A. This showed that NW056 and KHF-1A significantly improved the high-temperature performance of asphalt more than traditional NaCl deicing agents. In addition, the influence of different dosages of deicing agents on the high-temperature performance of asphalt was distinct. When the NW056 solution was 10% and 26%, the anti-rutting factor of asphalt was the same. In comparison, the NaCl solution decreased by 10%–20%, indicating that the influence of NW056 solution on the high-temperature performance of asphalt was less affected by a mass fraction, KHF-1A was moderate, and NaCl was most affected by a mass fraction.
The high-temperature performance of asphalt was not significantly affected by the temperature of the deicing agent. The variation of different deicing agents and original samples with temperature showed a similar trend.
3.1.2 Low-temperature performance
The BBR test measured the low-temperature performance of asphalt after holding at −25 °C for 96h, and the loading time was 60s. The creep stiffness (S) and creep rate (m) of asphalt treated with different deicing agent solutions are shown in Figure 3. Both met the specification requirements that the creep stiffness modulus of asphalt should not exceed 300 MPa, and the creep rate should not be less than 0.3. In fact, the higher the S value, the more brittle the material and the more prone to road surface cracking. The higher the m value, the better the stress relaxation ability of the material and the better the low-temperature crack resistance (Li et al., 2017).
Using the deicing agent increased the creep stiffness of asphalt by 15%–50%. It decreased the creep rate by 3%–11%, indicating that the deicing agent deteriorated the low-temperature crack resistance of asphalt, resulting in the brittleness of asphalt and the deterioration of stress relaxation ability. This is due to the significant difference in the effect of different deicers on the low-temperature performance of asphalt. Overall, acetate deicer had a more substantial impact on the creep stiffness (S) and creep rate (m) of asphalt than chloride deicer. After soaking in NaCl solution, KHF-1A solution, and NW056 solution, the creep stiffness of asphalt increased by 16%, 49%, and 26% on average, and the creep rate decreased by 3%, 6%, and 4%, respectively. The low-temperature performance of the acetate deicing agent changed significantly because the acetate solution contained a large number of lipophilic organic chains (-CH3) and hydrophilic groups (-COO) that could act as emulsifiers in the aqueous solution and emulsify the asphalt (Pan et al., 2008). The proportion of small molecular components in the asphalt decreased, resulting in a decrease in the stress relaxation ability of the asphalt, which in turn deteriorated the low-temperature performance (Pan, 2007).
The deicing agent’s mass fraction also affected asphalt’s low-temperature performance. NaCl and NW056 deicing agents showed that the higher the mass fraction of the deicing agent, the more severe the deterioration of the low-temperature performance. Although both KHF-1A and NW056 were acetate deicing agents and had the same primary components, KHF-1A showed the opposite trend. This might have been because the types of additives were different, and some additives (N, P, S, Pb, and Cd) in KHF-1A caused this phenomenon.
3.1.3 Fatigue performance
DSR tested the shear rheological properties of asphalt film samples after soaking them at room temperature for 30 days. The DSR test used a strain control of 10% and an angular velocity of 10 rad/s. The changes in asphalt anti-fatigue factor (G*·sinδ) with temperature after treatment with different deicing agent solutions are shown in Figure 4. Previous studies have shown that G*·sinδ could represent the fatigue resistance of materials, and the lower the value, the stronger the fatigue resistance (Yang et al., 2022).
The results showed that the deicer solution treatment increased the anti-fatigue factor (G*·sinδ) of asphalt by 50-200KPa, which implied that the deicer impaired the anti-fatigue performance of asphalt. This might have been attributed to the penetration of salt ions from the deicer solution into the asphalt, which disrupted the molecular bond and increased the content of solid asphaltene. Consequently, the asphalt became more brittle, reducing its fatigue performance (Zhang et al., 2021a).
The type of deicing agent significantly influenced the fatigue performance degradation of asphalt. NW056 had the most severe effect on asphalt degradation among the three deicing agents. NaCl and KHF-1A had a minor effect on the anti-fatigue factor of asphalt. The ranking of the anti-fatigue factor of asphalt at 10% mass fraction was NW056 > NaCl > KHF-1A, while at 26% mass fraction, it was KHF-1A > NW056 > NaCl. At the same temperature, the acetate deicing agent’s mass fraction positively correlated with the anti-fatigue factor of asphalt. This was because the higher concentration of acetate ions penetrated the asphalt more effectively, which damaged the asphalt molecular bond faster and more extensively. This also made the asphalt more susceptible to fatigue.
The deicing agent’s temperature had a negligible effect on the fatigue performance of asphalt. The results indicated that the three deicing agents had the same influence on the anti-fatigue factor of asphalt as the original sample. The only exception was the 10% NaCl asphalt, which showed an abnormal increase in the anti-fatigue factor. This might have been due to the rapid reaction between salt ions in NaCl solution and asphalt under NaCl’s optimal deicing mass fraction.
3.1.4 Comprehensive effection for asphalt performance
The effects of different experimental parameters on asphalt performance were investigated using four key indicators: the anti-rutting factor G*/sinδ, the creep stiffness S, the creep rate m, and the anti-fatigue factor G*·sinδ. The relative changes of these indicators for asphalt treated with different deicing agents compared to the untreated sample are shown in Figure 5.
A more pronounced effect was observed on the high-temperature performance of asphalt due to the use of deicing agents than on the low-temperature performance. The high-temperature performance was improved by more than 40%, while the low-temperature performance was reduced by less than 40%. The fatigue performance of asphalt was deteriorated by different deicing agents, and the difference was noticeable.
At 10% mass fraction, the three types of deicing agents had similar effects on the high-temperature performance of asphalt. Only KHF-1A deicing agent caused a higher creep stiffness S of asphalt at low temperatures, but the difference was insignificant. KHF-1A deicing agent had the most negligible effect and the best performance regarding asphalt fatigue degradation. At 26% mass fraction, the NW056 deicing agent had a much more substantial impact on the high-temperature performance of asphalt than the other two deicing agents. In contrast, the effects on the low-temperature performance were comparable. NW056 deicing agent also had a much stronger effect on asphalt fatigue degradation than NaCl, while KHF-1A had a moderate impact.
Based on the comprehensive evaluation of the effects of the conventional deicing agent NaCl and two acetate deicing agents, KHF-1A and NW056, on asphalt performance, KHF-1A was found to perform better than NW056. It also had similar or better performance than NaCl in some aspects.
3.2 Effect of deicing agent on mixture performance
3.2.1 High-temperature performance
The IDT test measured the splitting strength (MPa) of asphalt mixture samples after soaking them in the deicing agent for 30 min. The models had a diameter of 150 mm and a thickness of 50 mm. Figure 6 shows the results of the splitting strength test for the samples treated with different mass fractions of deicing agent solution.
Figure 6 shows that using the deicing agent reduced the splitting strength of the asphalt mixture by 10%–40% compared to the original, indicating a significant deterioration of the high-temperature performance. NaCl solution had the most pronounced effect on the splitting strength among the three deicing agents, while NW056 and KHF-1A solutions had relatively minor effects. This might have been due to the cracking of the interface between the asphalt layer and the aggregate caused by the crystallization expansion of the deicer in the freeze-thaw environment. The cracks allowed more water to infiltrate the asphalt binder and the total, reducing the splitting strength (Meng et al., 2022).
The effect of the chloride deicing agent on the splitting strength of the mixture was seriously affected by the mass fraction and the number of cycles. The acetate deicing agent was more stable. The ranking of the deicing agents by their impact on the splitting strength was NaCl > KHF-1A > NW056. At 10% mass fraction, the mixture treated with NW056 and KHF-1A solution showed a significant decrease in splitting strength by 8.2% and 12.6%, respectively, from 10 to 20 cycles. At 26% mass fraction, the mixture treated with NaCl solution showed a drastic reduction in splitting strength by 33.3%, while the changes of NW056 and KHF-1A were relatively small. In general, the splitting force of the mixture decreased as the number of freeze-thaw cycles increased. The mass fraction significantly affected the splitting strength when the number of cycles was 10. After 20 cycles, the splitting force changed slightly with the mass fraction, all within 2%.
3.2.2 Low-temperature performance
The bending test of the beams was conducted to study the effect of the deicing agent on the mixture’s low-temperature performance. The length of the test trabecular was 250 ± 2.0 mm, a width of 30 ± 2.0 mm, and a height of 35 ± 2.0 mm. After soaking in the deicing agent solution for 24 h, they were subjected to freeze-thaw cycles according to the “high-temperature performance freeze-thaw cycle test conditions.” The mixture’s bending stiffness modulus was measured; the results are shown in Figure 7.
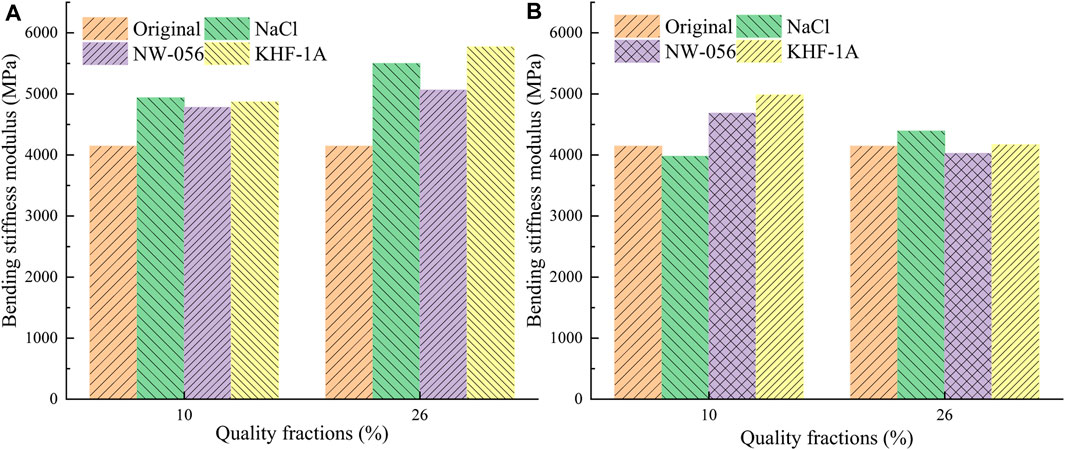
FIGURE 7. Bending stiffness modulus under different conditions. (A) Cycle 10 times (B) Cycle 20 times.
The deicing agent significantly affected the low-temperature crack resistance of the asphalt mixture. Using a deicing agent increased the bending stiffness modulus of the asphalt mixture by 6%–20%, indicating a deterioration of the low-temperature crack resistance. This might have been due to the penetration of the chloride deicing agent solution into the pores of the specimen, which reduced the adhesion between the asphalt mixture components during the wet-dry cycle. As a result, the flexural tensile strength of the asphalt mixture decreased, and the bending stiffness modulus increased. Among the three deicing agents, KHF-1A had the most substantial effect on the bending stiffness modulus of the cross, followed by NaCl and NW056. The impacts of KHF-1A and NW056 deicing agent solutions on the asphalt mixture were mainly caused by emulsification. The emulsification reduced the asphalt content, lowered the flexural strength of the asphalt mixture, and raised the bending stiffness model (Zhang et al., 2021b).
The concentration of the deicing agent strongly influenced the bending stiffness modulus of the mixture, and the trend varied with different cycles of the mass fraction. At 10 cycles, the ranking of the three deicing agents by their effect on the bending stiffness modulus was KHF-1A > NaCl > NW056; the bending stiffness modulus of the mixture increased by 21%, 13.6%, and 6.8%, respectively, from 10% to 26% concentration. At 20 cycles, the increases were 19.7%, 23.2%, and 15.9%, respectively. The bending stiffness modulus of the mixture decreased as the number of freeze-thaw cycles increased. After 20 cycles, the bending stiffness modulus of the three deicing agents became more stable and approached the original value at 26% concentration.
3.2.3 Water stability performance
The freeze-thaw splitting test evaluated the water stability of the mixture. The test measured the mixture’s freeze-thaw splitting strength ratio (TSR), shown in Figure 8.
The deicing agent had a negligible effect on the water stability of the asphalt mixture. The acetate and chloride deicing agents did not significantly affect the freeze-thaw splitting strength ratio of the mix, and some ratios were even higher than those of the water solution. This might be explained by the fact that the water volume in the pores of the asphalt mixture changed constantly during the freezing and melting processes. The addition of a deicing agent reduced the volume expansion rate of the solution when it froze (Ma et al., 2021), which in turn reduced the volume expansion of the answer in the pores of the mixture during the freeze-thaw cycle and the structural damage of the asphalt mixture.
The mixture treated with a high mass fraction deicing agent had a higher freeze-thaw splitting strength ratio because of the lower volume expansion rate of the high concentration deicing agent solution. The low mass fraction deicing agent had little effect on the freeze-thaw splitting strength ratio of the mixture. The freeze-thaw splitting strength ratio of the mixture increased with the number of compaction times. This might be because higher compaction times decreased the porosity of the mix, which reduced the reaction rate between the deicing agent and the asphalt mixture and ultimately lowered the splitting strength ratio of the mix.
3.2.4 Comprehensive evaluation of the effect on the performance of asphalt and mixture
The analysis of the effect of the deicing agent on the high and low-temperature performance of asphalt and mixture was based on the following indicators: the anti-rutting factor G*/sinδ of asphalt and the splitting strength of medicine at high temperatures and the creep stiffness S and the bending stiffness modulus of the mixture at low temperatures. The relative changes of these indicators for asphalt and variety treated with deicing agents compared to the untreated samples are shown in Figure 9 and Figure 10.
The deicing agent had opposite effects on the high-temperature performance of asphalt and mixture. It improved the high-temperature performance of asphalt but reduced the mixture’s high-temperature performance. Figure 9 shows that the higher the improvement of the high-temperature performance of asphalt, the lower the reduction of the mixture of high-temperature performance, indicating a negative correlation between the deicing agent and the high-temperature performance of asphalt and variety. At 10% mass fraction, KHF-1A and NW056 deicing agents improved the high-temperature performance of asphalt more than the conventional NaCl deicing agent and reduced the high-temperature performance of the mixture less. At 26% mass fraction, KHF-1A and NW056 deicing agents still improved the high-temperature performance of asphalt more than NaCl deicing agents. Still, the ranking of the deicing agents by their impact on the mixture’s high-temperature performance was KHF-1A > NW056 > NaCl, with a slight difference.
The deicing agent deteriorated the low-temperature performance of asphalt and mixture. Figure 10 shows that the type of deicing agent greatly influenced its effect on the low-temperature performance of asphalt and mix. At 10% mass fraction, the KHF-1A deicing agent had a much stronger deterioration effect on the low-temperature performance of asphalt and mixture than NW056 and NaCl deicing agents. In contrast, the NaCl deicing agent had the most minor impact on the low-temperature performance of the mix. At 26% mass fraction, the NW056 deicing agent had the most substantial reduction effect on the low-temperature performance of asphalt among the three but the weakest effect on the low-temperature performance of the mixture. NaCl and KHF-1A deicing agents had similar effects on the low-temperature performance degradation of asphalt and mix.
Based on the comprehensive evaluation of the effects of chloride deicing agent NaCl and two acetate deicing agents, KHF-1A and NW056, on the high and low-temperature performance of asphalt and mixture, NW056 deicing agent was found to have the best performance among the three.
4 Conclusion
This study used laboratory tests to investigate the effects of three deicing agents (NaCl, KHF-1A, and NW056) on airport asphalt and mixture performance. The main findings are as follows.
(1) The change in asphalt performance is greater than 10%. Although the fact that the high-temperature performance of asphalt increases by more than 25%, the low-temperature crack resistance and fatigue resistance are significantly weakened, by nearly 50%.
(2) Compared with NaCl, deicers NW056 and KHF-1A have minor effects on the performance of asphalt. Due to the emulsifying effect of acetic acid on asphalt, the low-temperature performance of NW056 and KHF-1A is slightly higher than that of NaCl.
(3) The mixture’s high-temperature performance decreases by 10%–40%, and the low-temperature performance decreases by 6%–20%. Furthermore, the more significant the improvement of the high-temperature performance of the asphalt by the deicing agent, the smaller the reduction of the mixture’s high-temperature performance.
(4) The two acetate deicers have better pavement performance than the NaCl deicer. Even after prolonged use, the asphalt pavement surface may be damaged, but due to the weak corrosion of the acetate deicing agent, it will not cause severe damage to the pavement. In particular, the NW056 deicing agent has a minor effect on the high and low temperatures of the mixture and is an excellent alternative material for pavement deicing.
This study investigated the effects of the combined deicing agent NaCl and the acetate deicing agents NW056 and KHF-1A on the performance of airport pavements in detail. It verified the feasibility of replacing traditional chloride deicing agents with NW056 and KHF-1A. This has a good application prospect and helps to provide a reference for selecting deicing agents for airport deicing. However, this study also has some limitations. On the one hand, due to the large variety of acetate deicing agents, this paper only selects NW056 and KHF-1A as representatives. On the other hand, the impact of deicing agents on pavement skid resistance, wear resistance, low-temperature fracture, and aggregate performance has not been considered. This is also the direction of further research in the future.
Data availability statement
The original contributions presented in the study are included in the article/Supplementary material, further inquiries can be directed to the corresponding authors.
Author contributions
PL is the experimental designer of this study and contributes to the writing and revision of the thesis. WP participated in the writing of the first draft of the article and conducted data analysis. YH participated in the test and compiled the experimental data and some manuscripts. ZL and BW participated in the experimental design and provided help in data analysis. WL participated in the test of experimental samples. WW participated in the English polishing of the final manuscript. All authors contributed to the article and approved the submitted version.
Funding
This work was supported by the National Key Research and Development Program of China (2021YFB2601000), Guangxi Key Research and Development Program (AB21220070), Airport Engineering Research Center of CAAC Program (ERCAOTP20220301), the National Natural Science Foundation of China (51878075), Hunan Provincial Natural Science Foundation of China (2022JJ30594), Postgraduate Scientific Research Innovation Project of Hunan Province (QL20220189), and Key Scientific Research Projects of Universities in Henan Province (23A580005/23B580005).
Conflict of interest
BW was employed by Hunan Expressway Maintenance Engineering Co., Ltd.
The remaining authors declare that the research was conducted in the absence of any commercial or financial relationships that could be construed as a potential conflict of interest.
The reviewer (HY) declared a shared parent affiliation with the authors (PL, WP, YH, ZL) to the handling editor at the time of the review.
Publisher’s note
All claims expressed in this article are solely those of the authors and do not necessarily represent those of their affiliated organizations, or those of the publisher, the editors and the reviewers. Any product that may be evaluated in this article, or claim that may be made by its manufacturer, is not guaranteed or endorsed by the publisher.
Supplementary material
The Supplementary Material for this article can be found online at: https://www.frontiersin.org/articles/10.3389/fmats.2023.1231483/full#supplementary-material
References
Bennert, T., Haas, E., and Wass, E. (2018). Indirect tensile test (IDT) to determine asphalt mixture performance indicators during quality control testing in New Jersey. Transp. Res. Rec. 2672, 394–403. doi:10.1177/0361198118793276
Button, J. W., Timothy, B. A., Ronald, C., Frank, F., Gary, L. F., Thomas, P. H., et al. (2004). New simple performance test for asphalt mixes. Washington, DC: Transp. Res. Board, 1–44.
Christensen, D. W., Bonaquist, R., Anderson, D. A., and Gokhale, S. (2004). “Indirect tension strength as a simple performance test,” in Transportation research circular, number E-C068, 44–57.
Christensen, D. W., and Bonaquist, R. (2002). Use of strength tests for evaluating the rut resistance of asphalt concrete. J. Assoc. Asphalt Paving Technol., 71.
Civil Aviation Administration of China (2022). 2021 statistical bulletin of civil aviation industry development. 7.
Civil Aviation Administration of China (2019). Specifications for asphalt pavement construction of civil airports. MH/T 5011-2019. China Civil Aviation Publishing House.
Das, J. K., and Pradhan, B. (2019). Effect of cation type of chloride salts on corrosion behaviour of steel in concrete powder electrolyte solution in the presence of corrosion inhibitors. Constr. Build. Mater 208, 175–191. doi:10.1016/j.conbuildmat.2019.02.153
Du, S., Akin, M., Bergner, D., Xu, G., and Shi, X. (2019). Synthesis of material application methodologies for winter operations.
Edwards, Y., and Aurstad, J. (2000). The influence of runway de-icing agents on the durability of asphalt concrete pavements for airfields. Road. Mater Pavement 1, 387–405. doi:10.1080/14680629.2000.12067152
Fay, L., and Shi, X. (2012). Environmental impacts of chemicals for snow and ice control: state of the knowledge. Water, Air, and Soil Pollut. 223, 2751–2770. doi:10.1007/s11270-011-1064-6
Fay, L., and Shi, X. (2011). Laboratory investigation of performance and impacts of snow and ice control chemicals for winter road service. J. COLD Reg. Eng. 25, 89–114. doi:10.1061/(asce)cr.1943-5495.0000025
Feng, D., Yi, J., Wang, D., and Chen, L. (2010). Impact of salt and freeze–thaw cycles on performance of asphalt mixtures in coastal frozen region of China. COLD Reg. Sci. Technol. 62, 34–41. doi:10.1016/j.coldregions.2010.02.002
Findlay, S. E., and Kelly, V. R. (2011). Emerging indirect and long-term road salt effects on ecosystems. Ann. N. Y. Acad. Sci. 1223, 58–68. doi:10.1111/j.1749-6632.2010.05942.x
Fortin, C., Tjaden, L., and Mulhem, N. (2014). Chloride free snow and ice control material. Transp. Res. synthesis.
Frolova, E. A., Kondakov, D. F., Nikolaev, V. V., Tin Gaev, O. P., and Danilov, V. P. (2015). Phase equilibria in the sodium formate–potassium formate–water system below 0° c and the anti-icing properties of salt compositions. Theor. Found. Chem. EN+ 49, 719–720. doi:10.1134/s004057951505005x
Gruber, M. R., Hofko, B., Hoffmann, M., Stinglmayr, D., and Grothe, H. (2023a). Analysis of metal corrosion methods and identification of cost-efficient and low corrosion deicing agents. Corros. Eng. Sci. Technol. 58, 452–463. doi:10.1080/1478422x.2023.2200008
Gruber, M. R., Hofko, B., Hoffmann, M., Stinglmayr, D., Seifried, T. M., and Grothe, H. (2023b). Deicing performance of common deicing agents for winter maintenance with and without corrosion-inhibiting substances. COLD Reg. Sci. Technol. 208, 103795, 103795. doi:10.1016/j.coldregions.2023.103795
Hanke, H., Nutz, P., Maier-Farkas, H., Neuhold, J., Rizzardo, F., Jezek, P., et al. (2019). International development of application methods of de-icing chemicals-state of the art and best practice.
Koščák, P., Berežný, Š., Vajdová, I., Koblen, I., Ojciec, M., Matisková, D., et al. (2020). Reducing the negative environmental impact of winter airport maintenance through its model design and simulation. Int. J. Env. Res. PUB HE 17, 1296. doi:10.3390/ijerph17041296
Li, B., Huang, W., Tang, N., Hu, J., Lin, P., Guan, W., et al. (2017). Evolution of components distribution and its effect on low temperature properties of terminal blend rubberized asphalt binder. Constr. Build. MATER 136, 598–608. doi:10.1016/j.conbuildmat.2017.01.118
Ma, H., Yu, H., Tan, Y., and Da, B. (2021). Ice pressure and icing volume expansion rate of acetate-based deicers under freezing conditions. Constr. Build. MATER 305, 124751. doi:10.1016/j.conbuildmat.2021.124751
McCarthy, L. M., Callans, J., Quigley, R., and Scott, S. V. (2016). Performance specifications for asphalt mixtures.
Meng, Y., Lai, J., Ling, L., Zhang, C., Chen, J., and Zhu, J. (2023). Preparation of an eco-friendly de-icing filler and its effects on the performance of different asphalt mastic. Constr. Build. Mater 364, 129967. doi:10.1016/j.conbuildmat.2022.129967
Meng, Y., Xu, R., Guo, H., Ling, L., Xi, C., Qin, Y., et al. (2021). Influences of sodium salt erosion on the self-healing ability and fatigue life of bitumen. Constr. Build. Mater 286, 122884. doi:10.1016/j.conbuildmat.2021.122884
Meng, Y., Zhang, C., Chen, J., Ling, L., Lai, J., Yan, T., et al. (2022). Deicing characteristics and pavement performance of eco-friendly de-icing asphalt mixture. Constr. Build. Mater 360, 129565. doi:10.1016/j.conbuildmat.2022.129565
Ministry of Transport Highway Research Institute (2011). Standard test methods of bitumen and bituminous mixtures for highway engineering. Industry Standard - Transportation.
Ministry of Transport of China (2021). Outline of national comprehensive three-dimensional transportation network planning.
Nazari, M. H., and Shi, X. (2019). Developing renewable agro-based anti-icers for sustainable winter road maintenance operations. J. Mater Civ. Eng. 31, 4019299. doi:10.1061/(asce)mt.1943-5533.0002963
Pan, T., He, X., and Shi, X. (2008). Laboratory investigation of acetate-based deicing/anti-icing agents deteriorating airfield asphalt (with discussion). J. Assoc. Asphalt Paving Technol., 77773–793.
Pan, T. (2007). “Mitigation of moisture and deicer effects on asphalt thermal cracking through polymer modification,” in Prepared for the US department of transportation research and innovative Technology administration.
Peng, W., Li, P., Gong, W., Tian, S., Wang, Z., Liu, S., et al. (2023). Preparation and mechanism of rubber-plastic alloy crumb rubber modified asphalt with low viscosity and stabilized performance. Constr. Build. MATER 388, 131687. doi:10.1016/j.conbuildmat.2023.131687
Qiu, L., and Nixon, W. A. (2008). Effects of adverse weather on traffic crashes: systematic review and meta-analysis. Transp. Res. Rec. 2055, 139–146. doi:10.3141/2055-16
Shi, X., Akin, M., Pan, T., Fay, L., Liu, Y., and Yang, Z. (2009). Deicer impacts on pavement materials: introduction and recent developments. Open Civ. Eng. J. 3, 16–27. doi:10.2174/1874149500903010016
Shi, X., Jungwirth, S., Akin, M., Wright, R., Fay, L., Veneziano, D. A., et al. (2014). Evaluating snow and ice control chemicals for environmentally sustainable highway maintenance operations. J. Transp. Eng. 140, 5014005. doi:10.1061/(asce)te.1943-5436.0000709
Terry, L. G., Conaway, K., Rebar, J., and Graettinger, A. J. (2020). Alternative deicers for winter road maintenance—a review. Water, Air, Soil Pollut. 231, 394–429. doi:10.1007/s11270-020-04773-x
Vignisdottir, H. R., Ebrahimi, B., Booto, G. K., O'Born, R., Brattebø, H., Wallbaum, H., et al. (2019). A review of environmental impacts of winter road maintenance. COLD Reg. Sci. Technol. 158, 143–153. doi:10.1016/j.coldregions.2018.10.013
Xiong, R., Jiang, W., Yang, F., Li, K., Guan, B., and Zhao, H. (2019). Investigation of voids characteristics in an asphalt mixture exposed to salt erosion based on CT images. MATERIALS 12, 3774. doi:10.3390/ma12223774
Yang, K., Li, R., Underwood, B. S., and Castorena, C. (2022). Effect of laboratory oxidative aging on dynamic shear rheometer measures of asphalt binder fatigue cracking resistance. Constr. Build. MATER 337, 127566. doi:10.1016/j.conbuildmat.2022.127566
Zhang, K., Li, W., and Han, F. (2019). Performance deterioration mechanism and improvement techniques of asphalt mixture in salty and humid environment. Constr. Build. MATER 208, 749–757. doi:10.1016/j.conbuildmat.2019.03.061
Zhang, K., and Zhang, Z. (2015). Deterioration of mechanical properties of asphalt mixture in salty and humid environment. J. South China Univ. Technol. Sci. Ed. 43, 106–112. doi:10.3969/j.issn.1000-565X.2015.08.016
Zhang, K., Zhang, Z., Yi, F., Montserrat, N., Hishida, T., Esteban, C. R., et al. (2014). Direct conversion of human fibroblasts into retinal pigment epithelium-like cells by defined factors. J. WUHAN Univ. Technol. 36, 48–58. doi:10.1007/s13238-013-0011-2
Zhang, Q., Wu, D., Zhang, X., Chang, K., and Wang, Y. (2021a). Effect of organic deicing agents on asphalt rheology and analysis of the mechanism. Constr. Build. MATER 284, 122649. doi:10.1016/j.conbuildmat.2021.122649
Keywords: deicing agent, airport pavement, asphalt performance, mixture performance, acetate deicing agent
Citation: Li P, Peng W, Han Y, Wang B, Liu Z, Li W and Wei W (2023) Effect of deicing agents on the performance of airport asphalt pavement. Front. Mater. 10:1231483. doi: 10.3389/fmats.2023.1231483
Received: 30 May 2023; Accepted: 14 August 2023;
Published: 28 August 2023.
Edited by:
Dawei Wang, RWTH Aachen University, GermanyReviewed by:
Huanan Yu, Changsha University of Science and Technology, ChinaDi Wang, Aalto University, Finland
Yue Xiao, Chang’an University, China
Copyright © 2023 Li, Peng, Han, Wang, Liu, Li and Wei. This is an open-access article distributed under the terms of the Creative Commons Attribution License (CC BY). The use, distribution or reproduction in other forums is permitted, provided the original author(s) and the copyright owner(s) are credited and that the original publication in this journal is cited, in accordance with accepted academic practice. No use, distribution or reproduction is permitted which does not comply with these terms.
*Correspondence: Wenju Peng, cHdqMTExMkBmb3htYWlsLmNvbQ==; Yanlong Han, aGFuMTgzNDgzNDg3NDBAMTYzLmNvbQ==