- 1School of Transportation, Civil Engineering and Architecture, Foshan University, Foshan, China
- 2Shenzhen Oceanpower Industrial Co., Ltd., Shenzhen, China
- 3School of Architecture, Harbin Institute of Technology, Shenzhen, China
- 4Analysis and Testing Center, South China Normal University, Guangzhou, China
The current understanding of the influence of smoke suppressants on the smoke inhibition effect and working performance of different types of asphalt is limited. In this study, portable gas detector (PGD) tests were used to investigate the influence of the smoke suppressant on different types of asphalt and the smoke purification effect. Subsequently, gas chromatography-mass spectrometry (GC-MS) was used to analyze the influence of the smoke suppressant on the volatile organic compounds (VOCs) in different types of asphalt fume, so as to compare and verify the PGD tests results. Finally, scanning electron microscopy and energy dispersive spectrometry were used to observe and analyze the influence of the smoke suppressant on the microstructure and element composition of different types of asphalt. The results show that the smoke suppressant can purify various harmful gases volatilized from different types of asphalt, but the effect and the optimal dosage of smoke suppressant are slightly different. The purification rate of the smoke suppressant for SO2 or H2S in the fumes of various asphalts can reach >70% and the NO and VOC purification rates can reach >40%. The influence of the smoke suppressant on the VOC purification rate of different asphalts detected through the GC-MS and PGD tests is similar. The smoke suppressant has little effect on the asphalt surface microstructure and on the changes in the element content in asphalt, indicating that it has no effect on the main properties of the asphalt itself. This study is helpful to further understand the relationship between smoke suppressants and the smoke inhibition effect and the working performance of different types of asphalt. Additionally, it provides basis and guidance for the development of low-smoke asphalt with a better emission reduction effect, which has a certain guiding and application significance.
1 Introduction
Mankind has attached more and more importance to environmental protection during the construction of infrastructure (Zhang et al., 2022; Sun et al., 2023). However, the construction of asphalt pavement has always been one of the main sources of volatile organic pollutants. At present, the construction of asphalt pavements mainly adopts the hot mixing and hot paving method (Li et al., 2021; Lin et al., 2022; Shi et al., 2022). Asphalt generally needs to be heated to >160°C, and even >175 °C if it is modified asphalt. At high temperatures, asphalt emits a certain amount of “green smoke,” which contains more than 100 types of volatile organic substances, as well as carbon oxides, nitrogen oxides, and sulfides. Asphalt fume not only causes serious air pollution, but also damages the respiratory system of construction personnel (Rhomberg et al., 2015; Lin et al., 2016; Autelitano and Giuliani, 2018; Wu et al., 2018; Mo et al., 2019; Qu et al., 2021), especially in relatively closed construction environments (such as tunnels). Although the construction of asphalt pavement is to meet the traffic needs, it also introduces a series of problems that run counter to the direction of social development, such as environmental protection.
Therefore, many scholars have conducted a large amount of researches on ensuring the construction quality and performance of asphalt pavement while effectively reducing harmful gas emissions during construction with asphalt mixtures. The research focus is to reduce atmospheric pollution and protect the health of construction personnel. Some scholars have proposed that asphalt construction technology can be improved to reduce or eliminate the emission of asphalt fume at the source, wherein the warm mix asphalt (WMA) and the cold mix asphalt (CMA) construction technology are undoubtedly the two most effective methods (Hasan et al., 2017; Shanbara et al., 2021). The construction temperatures of WMA and CMA are generally more than 50°C and 100°C lower than that of hot mix asphalt (HMA), respectively. Therefore, the emissions of asphalt fume during the construction of WMA and CMA are significantly less than those of HMA (Rubio et al., 2013; Toraldo et al., 2014; Hansen et al., 2017; Dash et al., 2022). However, compared to HMA, WMA and CMA still fall slightly short in terms of road performance. Furthermore, despite WMA and CMA’s energy saving features, the use of additives and improved equipment in production would still increase costs. As a result, for reasons mentioned above and many others, WMA and CMA are not as widely used as HMA.
Due to the high temperature and large amount of fume emission of HMA, many scholars have researched on the addition of asphalt smoke suppressants to HMA to reduce emissions. As early as 2014, Cui et al. (2014) (Cui, 2015) found that adding styrene-butadiene-styrene (SBS) into the matrix asphalt can help the asphalt form a network structure to limit the movement of light components, thus increase the volatilization conditional threshold of the volatile organic compounds (VOCs). However, they also reported that the addition of SBS can improve the high and low temperature performance of asphalt materials, but the smoke inhibition effect is limited. Additionally, SBS needs to be used together with activated carbon filler to achieve an ideal smoke inhibition effect. Other types of materials have also been studied as asphalt smoke suppressants. Huang et al. (2014) (Huang et al., 2015) found that the expanded graphite (EG) layer will swell during the hot mixing process owing to the light components in the asphalt. At high temperature, the light components and polycyclic aromatic hydrocarbons in the asphalt can partially plug between the layers of EG and nucleate crystallization growth, or be absorbed on the EG surface. The lattice bonding and Van der Waals force can effectively suppress the release of asphalt fume. The HMA mixture prepared with EG has a good paving performance. Wu et al. (2016) studied the flame retarding mechanism of hydrated lime on asphalt mastic through cone calorimeter tests, thermogravimetry and differential scanning calorimetry analyses. They showed that hydrated lime has a strong flame retarding effect and can effectively reduce the total heat release rate, CO yield, and total smoke release rate of asphalt mastic. Xiao et al. (2017) analyzed and evaluated the effect of activated carbon on VOCs in asphalt materials using gas chromatography-mass spectrometry (GC-MS) and ultraviolet-visible spectrophotometry. The results show that activated carbon has a good inhibition effect on the asphalt VOC emission process, and the addition of activated carbon does not change the chemical composition of the asphalt. Zhang (2017) studied the inhibition effect of tourmaline powder on asphalt smoke emission, and found that tourmaline powder has little impact on asphalt smoke emission, but its inhibition effect on light components is better than that on heavy components. Tourmaline powder can replace mineral powder and has no effect on the stability and deformation resistance of asphalt mixtures at high temperature. However, tourmaline powder slightly reduces the ductility of asphalt. Wang et al. (2020) prepared an environmentally-friendly asphalt by adding SBS, Mg(OH)2, and deodorant into the matrix asphalt, effectively reducing the smoke emission, odor grade, and content of polycyclic aromatic hydrocarbons and mercaptan in the asphalt. Tang et al. (2020) found that adding geopolymer to asphalt effectively reduces VOC and particulate matter (PM) emissions in the asphalt production process, according to the results of thermogravimetry together with mass spectrometry and PM tests.
There are many types of asphalt smoke suppressants, they can be roughly divided into the three categories (Peng et al., 2011; Yang et al., 2022), namely, adsorbents with physical or chemical reaction adsorption; flame retardants that can reduce surface temperature to achieve smoke inhibition; and polymer modifiers that can form a network structure in asphalt to prevent volatilization of asphalt fumes. Previous research results provide rich references for the development, smoke inhibition effect, smoke inhibition mechanism, and working performance of asphalt smoke suppressants. However, the test methods, research objects, evaluation indicators, and smoke suppressants of different sources used by different researchers are varied, which could affect the reliability of the test results. Additionally, it is difficult to compare the smoke inhibition performance of smoke suppressants using unified evaluation indicators. More importantly, most of the existing literature only focuses on a single type of asphalt, while the smoke inhibition effect and working performance of the proposed smoke suppressant in other types of asphalt remains unknown. Therefore, the promotion and application of the smoke suppressant is limited.
Based on the research background and the perspective of the effect of smoke suppressants on different types of asphalt, this study first used a portable gas detector (PGD) and GC-MS to study the composition, quantification, and smoke inhibition effect of asphalt fume before and after adding smoke suppressant to four common types of asphalt. Subsequently, the microscopic morphology and element changes of the asphalts before and after adding smoke suppressant were analyzed by scanning electron microscopy (SEM) and energy dispersive spectrometry (EDS). The results of this study can help determine the impact of smoke suppressants on the smoke purification effect and work performance of different types of asphalt. Thereby a low smoke asphalt with a good emission reduction effect could be developed, so as to control and reduce the emission of asphalt fumes from the source, protect the atmospheric environment and the health of construction personnel, and therefore provide significant social and environmental benefits.
2 Materials and instruments
2.1 Asphalt
The main test objects of this study are four different types of asphalt, namely 70# matrix asphalt, SBS-modified asphalt, SBS high viscosity modified asphalt, and Trinidad Lake asphalt (TLA). Their properties are shown in Table 1. The test methods and properties of the four types of asphalt are in accordance with specification requirements (Research Institute of Highway Ministry of Transport, 2004; Research Institute of Highway Ministry of Transport, 2011).
2.2 Smoke suppressant
The asphalt smoke suppressant used in this study was provided by Shenzhen Oceanpower Industrial Co., Ltd., and is mainly composed of aldehydes and carboxylic ester compounds. Table 2 lists the technical indicators of the smoke suppressant. The smoke suppressant is a white powder at room temperature, which is convenient to store and easy to handle when mixed with asphalt, as shown in Figure 1A. The technical principle of this technology is that the additives can directly react with specific elements that have an impact on the air quality in the asphalt, including gases, particles, and molecules. The reaction could generate non-volatile macromolecules, minimize the possibility of escape of volatile harmful gas molecules, thus reduce the potential for air pollution and harm to construction personnel. Additionally, the smoke suppressant has a strong climatic resistance, therefore, the smoke inhibition effect is not easily affected by temperature or environment.
2.3 Instruments
2.3.1 PGD
PGDs have many advantages, such as easy to carry, simple to operate, and they can effectively detect harmful gases. PGDs have been widely used in recent years for indoor air quality detection, industrial safety monitoring, environmental protection monitoring, etc. The instrument used in this study was a MultiRAE six-in-one PGD. The PGD can test the SO2, NO, H2S, CO, NO2 and VOCs in the measured gas, with a minimum counting unit of 0.1 ppm. PGDs also need to be equipped with a filter when used. In addition, a 500 ml three-port flask and asphalt mixer were also used in combination with the PGD. Figures 1B–D show the related instruments.
2.3.2 GC-MS
GC-MS is an instrument that integrates the characteristics of gas chromatography and mass spectrometry to identify different substances in a sample. Gas chromatography is a physical separation method that mainly uses the differences in the boiling point, polarity, and adsorption properties of substances to achieve the separation of mixtures. As the detector of gas chromatography, mass spectrometers use an ionization source to ionize various constituent molecules into mass spectrometry fragments, and retrieves the fragment information through the corresponding spectrum library, providing the matching degree of this information with a chemical substance to qualitatively analyze a substance. GC-MS can accurately analyze the organic pollutants in a measured gas and is often used in many fields such as the petrochemical, food safety, and drug safety industries. The GC-MS apparatus used in this study was a SHIMADZU TD-30 thermal desorption instrument, as shown in Figures 1E, F.
2.3.3 SEM and EDS
SEM and EDS were also used in this study. The working principle of SEM is to form an electron beam from the electron gun, which then converge through the condenser and objective lens. The high-energy electron beam formed by focusing is used to bombard the surface of the sample so as to obtain the detailed microstructural characteristics of materials. SEM is widely used in materials and other fields because it can provide a direct observation of the interface morphology, microstructure and appearance characteristics of various components of materials. EDS can use the X-ray photon energies of different elements to qualitatively and quantitatively analyze the element composition of the test object. Currently, it is often used in conjunction with SEM.
3 Test design
Asphalt emits a certain amount of smoke at high temperature, and the smoke often contains many harmful gases. In indoor test, due to the small amount of asphalt and the low concentration of its volatile flue gas, it is very important to select a gas test method with reliable accuracy and easy operation according to the characteristics of the asphalt fume release.
The test scheme of this study is mainly divided into three parts, as described below.
3.1 Part 1
A PGD was used to analyze the changing relationship between the four types of asphalt and the SO2, H2S, CO, NO, NO2, and VOCs in the asphalt fumes with different dosages of the smoke suppressant. The aim was to analyze the impact of the smoke suppressant on the different types of asphalt and its effect on smoke purification, as well as to determine the optimal dosage of smoke suppressant for the four types of asphalt.
The specific operation steps for one single test were: 1) 240 g heated asphalt was poured into a 500 ml three-port flask sealed by pistons on both sides, and then the smoke suppressant was added. 2) The three-port flask was then placed in the thermostatic oil bath of the asphalt mixer with the temperature set, and the stirring shaft of the foldable stirring blade was then placed in the middle of the three-port flask and the height of the stirring shaft was fixed. 3) The uniform rotational speed was set and the mixture was mixed for 10 min so that the smoke suppressant can fully react with the asphalt. 4) The PGD was calibrated to zero with fresh air. The piston on one side of the three-port flask was then pulled out, the gas test part of the PGD was aligned with the exhaust port, and the test was performed for 20 min. The asphalt mixing was not stopped during the test to simulate the smoke environment generated by mixing asphalt during construction. 5) After the test was completed, the PGD was connected to a computer to export data.
3.2 Part 2
The influence of the smoke suppressant on the VOCs of the fumes from different types of heated asphalt was analyzed using the separation ability of chromatography and the qualitative function of mass spectrometry in GC-MS. And the results were compared with that of the PGD test so as to verify the latter’s accuracy and feasibility.
The sample was prepared in the same manner as described in part 1. The smoke suppressant was then added to the asphalt and mixed for 10 min. A small amount of asphalt was weighed and placed in a petri dish for sealing. After the cooling completed, 10 mg asphalt was placed in a test tube using liquid nitrogen freezing and crushing. When the sample was prepared, it was placed in the GC-MS, and the volatile gas of the sample was collected at specified temperature for 30 min. Then, the collected gas was separated and tested. The detection condition was a programmed increase of temperature. The initial temperature was 50°C and maintained for 1 min, then it was increased to 300°C at the speed of 10°C/min and maintained for more than 5 min at 300°C. The process duration was 30 min.
3.3 Part 3
SEM and EDS were used to observe and analyze the influence of the smoke suppressant on the micro-morphology, microstructure, distribution, and element composition of the different types of asphalt.
The production of the sample was similar to that described in Section 3.2. Part 2. Liquid nitrogen freezing and crushing was also used to prepare a small asphalt sample to place on the SEM stage. The sample was placed in a gold spray coating machine to prepare an asphalt sample with a charged surface so that the asphalt sample and the sample stage can form a good conductive body. During the scanning process, the electron beam formed by the SEM will be transferred due to the conductivity of the asphalt, which inhibits the aggregation of electrons to create SEM images, affecting the follow-up observation. Finally, the gold-coated asphalt sample was placed in the SEM chamber, and the microstructure was observed using the SEM and the element composition of the asphalt sample was determined using the EDS.
4 Analysis of the PGD test results
In this study, the average value of the gas concentration emitted by the tested sample in the interval period (20 min) was taken as the fume emission performance of the sample in this period. Three samples with the dosage of each group of smoke suppressants were prepared and the average value was reported.
The ratio of the gas emission reduction of asphalt with smoke suppressant to asphalt without smoke suppressant was determined using:
where C is the specific gas concentration reduction rate or purification rate (%), A is the average concentration of the specific gas during the test time of the asphalt sample without smoke suppressant (ppm), and B is the average concentration of the specific gas during the test time of the asphalt sample after adding smoke suppressant (ppm).
It should be noted that the gas concentration measured in this test was based on the direct connection of the test port of the test instrument to the exhaust port of the test object. Therefore, the measured gas concentration is relatively high, which does not represent the indoor gas concentration during the test.
4.1 Effect of the smoke suppressant on matrix asphalt
70# matrix asphalt is often used as the base asphalt to produce modified asphalt and can also be directly used for the preparation of asphalt mixtures for the middle and lower surface course, or low-grade pavement. The mixing temperature of matrix asphalt during construction often reaches 165°C. Under high temperature, asphalt will emit a certain amount of smoke, which contains many volatile harmful substances that are harmful to the air environment and the health of construction personnel. After considering the initial range recommended by the manufacturer and the economics, the dosage of the smoke suppressant in this study was proposed to be 0, 0.02%, 0.03%, 0.04%, and 0.05%. During the test, the temperature of the thermostatic oil bath of the asphalt mixer, namely, the mixing temperature was set to 165°C. Figures 2, 3 show the statistical analysis results of the data obtained from the PGD test of the impact of the different smoke suppressant dosages on the 70# matrix asphalt. It was found that the gas emission concentration of NO2 was 0, therefore, this gas data is not listed in Figure 2.
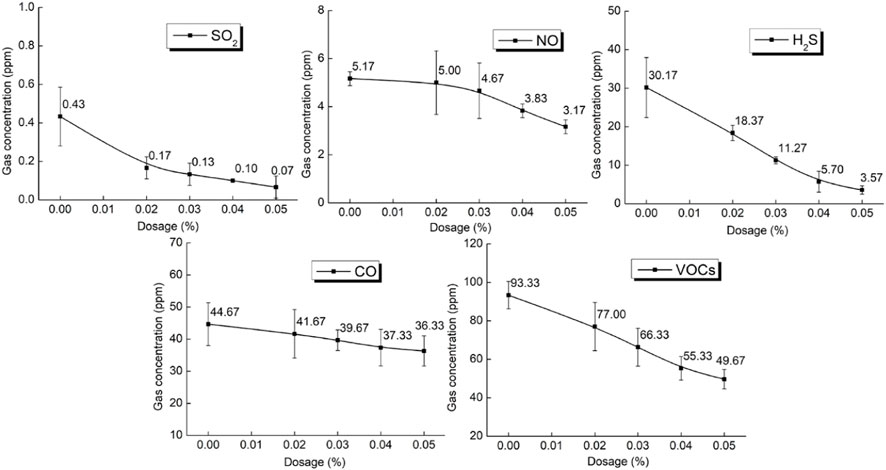
FIGURE 2. In the matrix asphalt, the relationship between the concentration of each gas and the smoke suppressant dosage.
The data show that 1) the emission concentration of SO2, NO, H2S, CO, and VOCs from the matrix asphalt decreased with an increasing dosage of smoke suppressant, indicating that the smoke suppressant can effectively purify the harmful gases in the matrix asphalt fumes. Additionally, the emission concentration of each gas and the effect of the smoke suppressant differed. 2) Figure 3A shows the results of the statistical analysis of the purification rate of various gases as a function of the smoke suppressant dosage, which shows that the smoke suppressant had the best effect on H2S and SO2 in the asphalt fume, that is, the smoke suppressant can achieve a higher purification rate of the two gases at lower dosages. At the maximum smoke suppressant dosage (0.05%), the purification rate of the two gases exceeded 80%. However, since the SO2 emission from this asphalt is very low, the inhibition effect of the smoke suppressant on SO2 must be judged in combination with the test results of other asphalt types. Furthermore, the smoke suppressant also had a good inhibition effect on the VOCs and NO in the asphalt fumes. The VOC and NO purification rates were 46.8% and 38.7%, respectively, at a smoke suppressant dosage of 0.05%. Finally, the effect of the smoke suppressant on CO was limited, and the maximum purification rate was only 18.7%. 3) The total gas emission concentration of each sample can be obtained by aggregating the emission concentration of the five gases of each group of samples, and then calculating the purification rate (hereinafter referred to as the “total purification rate”) of the total gas emission concentration with different smoke suppressant dosages, as shown in Figure 3B. It is clear that the addition of smoke suppressant can effectively reduce the total emission concentration of the matrix asphalt gas, and that a higher dosage of smoke suppressant can result in a higher total purification rate. When the dosage of the smoke suppressant was 0.02%–0.04%, the total purification rate increased rapidly. However, the growth rate weakened at dosages >0.04%. The total purification rate of matrix asphalt was 41.1% and 46.6% when the smoke suppressant dosage was 0.04% and 0.05%, respectively. There was little difference in the total purification rate of the two dosages, indicating that the smoke suppressant dosage was close to saturation. The effect of the two dosages on the purification rate of various gases was comprehensively compared. The purification rates of NO, SO2, H2S, VOCs, and CO at a smoke suppressant dosage of 0.04% were 12.9%, 7.7%, 7.1%, 6.1%, and 2.3% lower than those of the sample with a smoke suppressant dosage of 0.05%. Except for NO, there was little difference between the two dosages. Considering the low NO emission of this asphalt, which is not the factor of main concerned, and the economic cost, the optimal dosage of smoke suppressant for this matrix asphalt is 0.04%.
4.2 Effect of the smoke suppressant on SBS-modified asphalt
Although SBS contributes to the formation of an asphalt network structure and can inhibit the volatilization of smoke to a certain extent, its smoke inhibition effect on asphalt is limited. Additionally, SBS-modified asphalt also contains a small amount of other modified materials. The mixing temperature during construction is usually >175°C, which is higher than the construction temperature of matrix asphalt. Therefore, SBS-modified asphalt will still volatilize more harmful gases at higher temperatures, and it is recommended that the smoke suppressant dosage be appropriately increased. In this study, the smoke suppressant dosages in SBS-modified asphalt were 0, 0.02%, 0.04%, 0.06%, 0.08%, and 0.1%, and the mixing temperature was 175°C. Figures 4, 5 show the statistical results of the data obtained from the PGD test of the influence of different smoke suppressant dosages on SBS-modified asphalt. This asphalt also had no NO2 emissions.
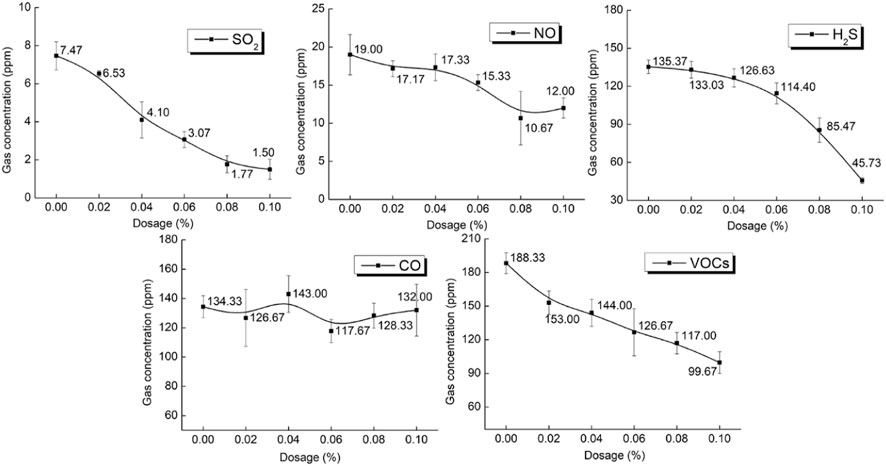
FIGURE 4. In the SBS-modified asphalt, the relationship between the concentration of each gas and the smoke suppressant dosage.
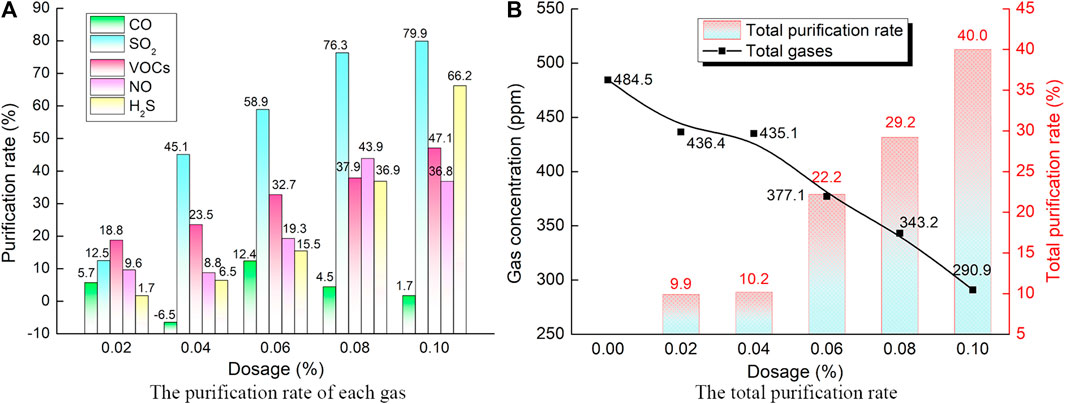
FIGURE 5. Influence of the smoke suppressant dosage on the purification rate of SBS-modified asphalt.
The above data show that 1) except for CO, the emission concentrations of various gases from SBS-modified asphalt exhibited a decreasing trend with an increasing smoke suppressant dosage, indicating that the smoke inhibition effect of the smoke suppressant on the SBS-modified asphalt was effective. 2) The smoke suppressant significantly inhibited the sulfides in the SBS-modified asphalt fumes, and the purification rates of SO2 and H2S in the asphalt fume were 80% and 66.2%, respectively, when the smoke suppressant dosage was 0.1%. 3) The smoke suppressant also exhibited a clear inhibition effect on NO and VOCs in the SBS-modified asphalt fumes, with the highest purification rates being 43.9% and 47.1%, respectively. 4) The inhibition effect of the smoke suppressant on CO was poor, with a purification rate of only 12.4%. Furthermore, the smoke suppressant dosage and gas concentration curves did not decrease with an increasing smoke suppressant dosage. 5) When the smoke suppressant dosage was 0.1%, the total purification rate of SBS-modified asphalt was 40%, and the comprehensive effect was at its optimal, which has obvious advantages over the other dosages. Furthermore, except for the purification rates of NO and CO, the inhibition effect of the dosage on the remaining gases was optimal; therefore, the optimal smoke suppressant dosage for SBS-modified asphalt is 0.1%.
4.3 Effect of the smoke suppressant on high viscosity modified asphalt
The construction mixing temperature of high viscosity modified asphalt is often >180°C, and it is more likely to emit harmful gases at high temperatures. In this study, the smoke suppressant dosage range was also 0%–0.1%, and the mixing temperature was 180°C. Figures 6, 7 show the statistical results of the data obtained from the PGD test of the influence of different smoke suppressant dosages on high viscosity modified asphalt. This asphalt also had no NO2 emissions.
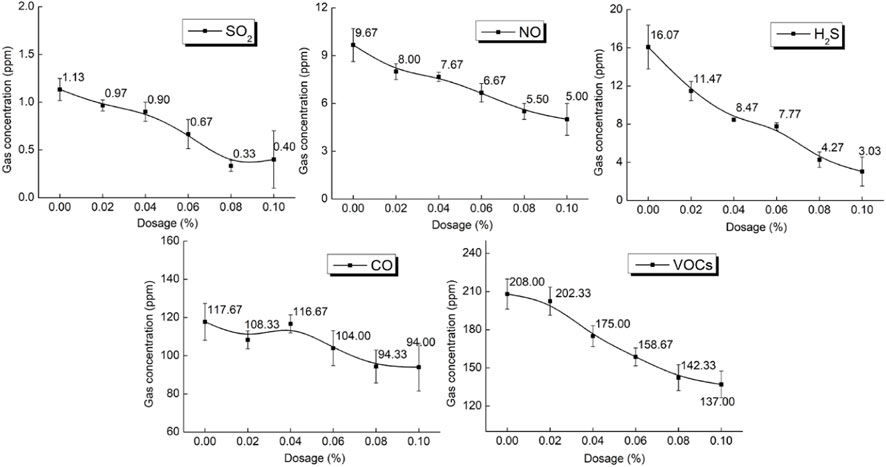
FIGURE 6. In the high viscosity modified asphalt, the relationship between the concentration of each gas and the smoke suppressant dosage.
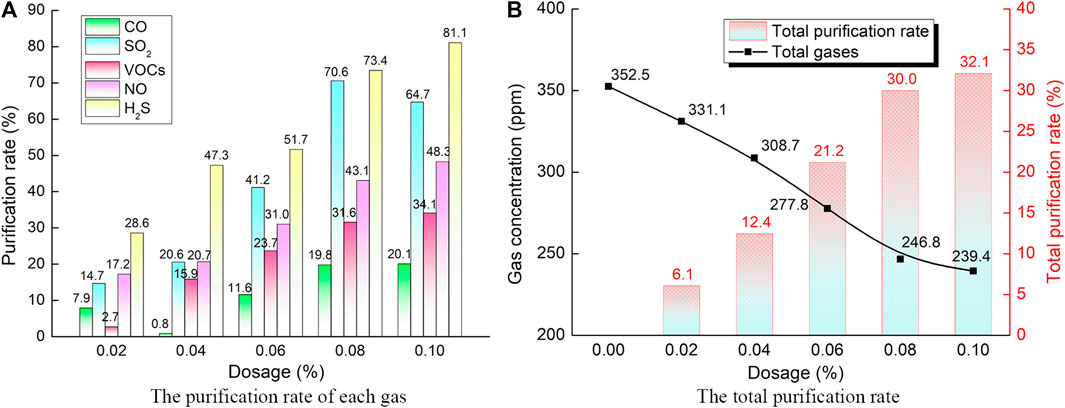
FIGURE 7. Influence of the smoke suppressant dosage on the purification rate of high viscosity modified asphalt.
The above data show that 1) the gas emission concentration of high viscosity modified asphalt decreased with an increasing smoke suppressant dosage, indicating that the smoke inhibition effect of the smoke suppressant on high viscosity modified asphalt fume was effective. 2) The highest SO2 and H2S purification rates in the high viscosity modified asphalt fumes were 70.6% and 81.1%, respectively, indicating that the inhibition effect of the smoke suppressant on the sulfides in the asphalt fumes was excellent. 3) The NO and VOC purification rates in the high viscosity modified asphalt fumes were 48.3% and 34.1% respectively, which are relatively significant inhibition effects. 4) The smoke suppressant had a moderate effect on the CO in the high viscosity modified asphalt fumes, and the maximum purification rate was only 20.1%. 5) The total purification rates of the high viscosity modified asphalt were 30% and 32.1% when the smoke suppressant dosage was 0.08% and 0.1%, respectively, indicating that when the effect of the smoke suppressant on high viscosity modified asphalt was close to saturation, to increase the dosage would only have limited smoke inhibition effect. The effects of the two dosages on the inhibition of various gases were comprehensively compared. The H2S and NO purification rates for the sample with the 0.1% dosage were only 7.7% and 5.2% higher than those of the sample with the 0.08% dosage. However, the SO2 purification rate was reduced by 5.9% and the CO and VOC purification rates were similar. Therefore, considering the economic cost, 0.08% is the optimal smoke suppressant dosage for high viscosity modified asphalt.
4.4 Effect of the smoke suppressant on TLA
TLA is a type of natural asphalt, that is, often added to matrix asphalt as a modifier to produce TLA-modified asphalt. Due to the excellent compatibility between TLA and ordinary asphalt, its allowable mixing amount is significantly high. The usual maximum mixing amount is 50%, and the maximum mixing amount of special pavement, such as on steel bridges, can even reach 70% (Feng et al., 2013; He et al., 2019). The mixing temperature of TLA-modified asphalt for construction is slightly higher than that of matrix asphalt and a certain amount of ash remains after refining the TLA. Therefore, it is more likely to emit more harmful gases. In this study, the dosage range of the smoke suppressant was also 0%–0.1%, the mixing temperature was 170°C, and the research object was a single TLA. Figures 8, 9 show the statistical results of the data obtained from the PGD test of the impact of different smoke suppressant dosages on TLA. No NO2 was released during the test.
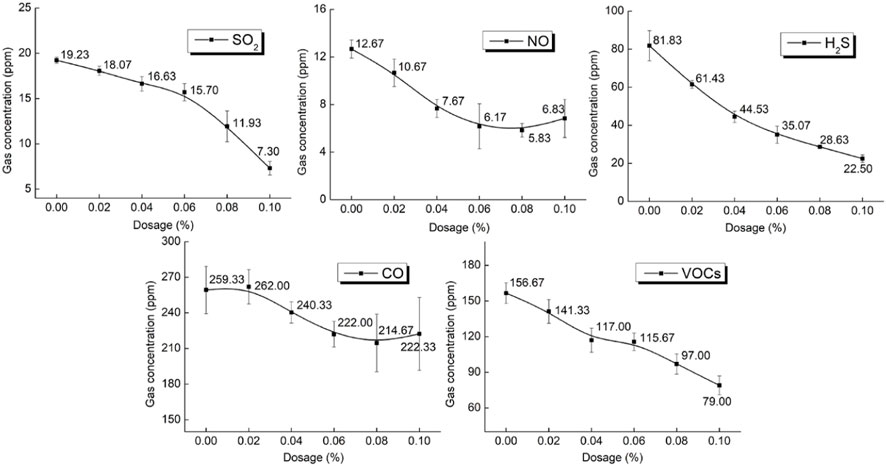
FIGURE 8. In the TLA, the relationship between the concentration of each gas and the smoke suppressant dosage.
The above data show that 1) the emission concentration of various gases from TLA decreased with an increasing smoke suppressant dosage, indicating that the smoke suppressant can also suppress the smoke from TLA. 2) The smoke suppressant significantly inhibited the sulfides in the TLA fumes, and the SO2 and H2S purification rates in the TLA fumes could reach up to 62.0% and 72.5% respectively. 3) The smoke suppressant also exhibited a good inhibition effect on NO and VOCs in the TLA fumes, with the highest NO and VOC purification rates being 53.9% and 49.6% respectively. However, the smoke suppressant exhibited a weak effect on CO, and the highest purification rate was only 18.5%. 4) When the smoke suppressant dosages were 0.08% and 0.1%, the total TLA purification rates were 33.0% and 36.2%, respectively, which were similar and indicated that the smoke suppressant dosages were close to saturation. The influence of the 0.08% and 0.1% dosages on the inhibition of various gases was analyzed. The H2S and VOC purification rates with a smoke suppressant dosage of 0.1% were 7.5% and 11.5% higher than that of the sample with a smoke suppressant dosage of 0.08%. However, the NO and CO purification rates were 7.8% and 4.2% lower than that of the sample with a smoke suppressant dosage of 0.08%, showing little difference. In contrast, the SO2 purification rate at a dosage of 0.1% was 24% higher than that of the 0.08% dosage, indicating a significant difference. Considering that SO2 is a gas with the potential to significantly harm the human body and that VOCs are an important indicator for environmental protection evaluation, it is necessary to effectively inhibit the emission of both these gases. Accordingly, the 0.1% smoke suppressant dosage was selected as the optimal dosage of smoke suppressant for TLA.
4.5 Comprehensive analysis
The analysis of the influence of the smoke suppressant on the four types of asphalt shows that although the mixing temperatures differ and the emission concentrations of various gases are different, the smoke suppressant can inhibit the smoke from the four types of asphalt.
First, the total emissions of all the gases from matrix asphalt were significantly lower than that from the other three types of asphalt, which is mainly due to the low mixing temperature of matrix asphalt and the low content of other modifiers or mineral components in matrix asphalt. Therefore, it is reasonable that the smoke suppressant dosage range for the designed matrix asphalt is less than that for the other three types of asphalt. The emission concentration of various gases is mainly related to the temperature and asphalt material itself. The H2S concentration in the fumes of the SBS-modified asphalt used in this test was the highest, and the concentrations of SO2 and CO released by TLA were significantly higher than that released by matrix asphalt and the other two modified asphalts. The NO emissions from matrix asphalt were the lowest, whereas that from the other three types of asphalt were similar. Matrix asphalt discharged the lowest amount of VOCs, the VOC quantity discharged from SBS-modified asphalt was similar to that discharged from TLA, and the VOC quantity discharged from high viscosity modified asphalt was the highest. NO2 was not detected in the four types of asphalt, indicating that the asphalt fume contains almost no NO2. Additionally, the proportion of VOCs and CO in the fume emitted by the four types of asphalt were significantly higher.
Second, by comparing the purification rate of the smoke suppressant on various gases, it was found that the smoke suppressant inhibited sulfides the most, and the highest SO2 or H2S purification rates were generally >70%. This is because the smoke suppressant can decompose H2S in asphalt fumes into water molecules and non-volatile organic sulfides. It can also decompose SO2 into water molecules and non-volatile alkyl sulfonic compounds. The smoke suppressant also plays a role in inhibiting NO and VOCs, and the average NO and VOC purification rates in the various asphalt fumes was >40% with the optimal dosage of smoke suppressant, indicating that the smoke suppressant can also help to reduce and decompose some NO and VOCs. The effect of the smoke suppressant on CO was poor. The relationship between the smoke suppressant dosage and the change in the purification rate was not stable and the purification rate was generally not high, indicating that this type of smoke suppressant had a limited effect on inhibiting CO.
Finally, the smoke inhibition effect of the smoke suppressant on different asphalts differed. The total purification rate showed that the smoke suppressant exhibited the best compatibility with matrix asphalt, that is, the optimal dosage of the smoke suppressant was the lowest but its effect was the greatest, and the total purification rate was >40%. The analysis shows that this was mainly because matrix asphalt contains very few modifiers or impurities. Therefore, the reaction between the smoke suppressant and asphalt was not affected by the presence of other components. The effect of the smoke suppressant on SBS-modified asphalt was also ideal, and the total purification rate with the optimal dosage was 40.0%. The smoke suppressant also played a significant role in TLA and high viscosity modified asphalt, and the total purification rates of both at the optimal dosage were >30%.
5 Analysis of the GC-MS test results
The inhibition effect and optimal dosage of the smoke suppressant on different types of asphalt fumes can be determined using the PGD test. To verify the validity of the PGD test results, GC-MS was also used to test the four types of asphalt fumes with different dosages of smoke suppressant. The previous PGD test results showed that the proportion of VOCs in the fume released from asphalt was relatively high. Considering that VOCs are one of the more serious air polluters and are highly representative, the amount of VOCs released by the sample within 30 min was used as the evaluation index in this test. To conduct the GC-MS test, the sample needs to be gasified, separated, and detected. The measured data is often expressed as the peak area. If the peaks appear at different times, the boiling points and polarity of the organic components of the peaks differ, that is, each retention time corresponds to different organic components. Therefore, the greater the total peak area, the greater the amount of VOCs in the tested sample.
5.1 Effect of the smoke suppressant on the VOCs in matrix asphalt
According to the PGD test results, the smoke suppressant dosages of 0.04% and 0.05% exhibited ideal inhibition effects on the 70# matrix asphalt fumes. Therefore, the samples with these two dosages and the sample without the smoke suppressant were selected for the VOC test. The specified temperature of the collected gas was consistent with the mixing temperature of the PGD test, which was 165°C. Figure 10A shows the VOC peak area variation of the asphalt samples with the three smoke suppressant dosages. To determine the effect of the smoke suppressant on the release of organic components with different boiling points, the VOC peak area was counted every 5 min, as shown in Figure 10B. Finally, the total VOC peak area was counted for each sample, and the total amount of VOCs as well as the purification rate were calculated (the purification rate was also calculated from the total VOC peak area, and the calculation method used was the same as shown in Eq. 1, as shown in Figure 10C.
The above data show that 1) the matrix asphalt samples with different smoke suppressant dosages produced significantly different VOC peak area curves, which indicates that the smoke suppressant can change the amount of VOCs in the asphalt fumes. The peak area curve of the samples with smoke suppressant was significantly lower than that of the samples without smoke suppressant, which also indicated that the quantity of VOCs in the samples with smoke suppressant was generally lower. 2) In the GC-MS tests, the initial temperature was 50°C, which was maintained for 1 min and then increased to 300°C at 10°C/min. Therefore, the peak at 0–5 min indicates that the detected boiling point of the organic components was approximately 50°C–100°C, and the peak at 5–10 min indicates that the boiling point of the organic components was 100°C–150°C, and similarly for the remaining ranges. 3) There was little difference in the quantity of VOCs at 0–5 min, indicating that the influence of the smoke suppressant on organic components with a boiling point below 100°C was low. However, at 5–10 min, the quantity of VOCs in the samples with smoke suppressant was significantly lower than that of the samples without smoke suppressant, indicating that the smoke suppressant began to inhibit the release of organic components with a boiling point >100°C. 4) The VOCs in the matrix asphalt sample were mainly released at 5–25 min, and the quantity of VOCs reached its highest value at 15–20 min, indicating that the main organic components in the matrix asphalt fumes had a large boiling point range, from 100°C to 300°C, and that the organic components with a boiling point of 200°C–250 °C accounted for the biggest proportion. The quantity of VOCs started to decline gradually after reaching 20 min, and the quantity of VOCs at 20–25 min was lower than that at 5–10 min and at 10–15 min, indicating that not many organic components with a boiling point >250°C were released from the matrix asphalt fume, and even fewer components with a boiling point up to 300°C were released from the matrix asphalt fume. 5) After 5 min, the quantity of VOCs in the sample without smoke suppressant was always higher than that of the samples with the smoke suppressant. The peak area of the sample with a smoke suppressant dosage of 0.05% was the smallest over the entire time range, indicating that the smoke suppressant effectively inhibited the release of the main VOCs in the matrix asphalt fumes, and the comprehensive effect was more ideal when the dosage of the smoke suppressant was 0.05%, as compared to that of 0.04%. (6) The VOC purification rate of the asphalt fume was 35.5% and 46.5% at the smoke suppressant dosages of 0.04% and 0.05%, respectively. The PGD test results showed that the VOC purification rate was 40.7% and 46.8% when the smoke suppressant dosage was 0.04% and 0.05%, respectively. This comparison showed that there was a difference between the results of the two test samples with the 0.04% dosage and that the results of the samples with the 0.05% dosage were similar.
There are many reasons for the above-mentioned differences. First, it is caused by the different quality of the asphalt samples. The GC-MS test sample weighed only 10 mg, whereas that of the PGD test sample was 240 g. Second, the instruments used for the tests were different. The VOCs range of GC-MS encompasses more organic substances, and the test accuracy is higher. Third, the test status was inconsistent. The PGD test was conducted under the condition that asphalt is continuously mixed, and the GC-MS test used the gas collected at the set temperature. Although there were significant differences between the two tests, these differences did not affect the similarity of the two results. The two test results showed that the smoke suppressant has a good inhibition effect on the VOCs in the matrix asphalt fumes, which also further verifies the validity of the PGD test results. At a smoke suppressant dosage of 0.05%, the VOC purification rate increased by 11%, as compared to that at a smoke suppressant dosage of 0.04%, which is significantly advantageous. However, according to the PGD test results, the difference between the two was only 6%. Therefore, it is necessary to reevaluate the optimal dosage of smoke suppressant for matrix asphalt. Considering that the GC-MS is more accurate for the detection of VOCs and the importance of VOCs in the environmental protection evaluation index, the optimal dosage was revised to be 0.05%.
5.2 Effect of the smoke suppressant on the VOCs in SBS-modified asphalt
According to the PGD test results, the smoke suppressant dosages of 0.08% and 0.1% exhibited a relatively ideal inhibition effect on the SBS-modified asphalt fumes. Therefore, the samples with these two dosages and the samples without the addition of smoke suppressant were selected for the VOC test, and the specified temperature of the collected gas was 175°C. Figure 11 shows the test results of the influence of different smoke suppressant dosages on the VOCs in SBS-modified asphalt.
The above data show that 1) the VOC peak area curves of the SBS-modified asphalt samples with different smoke suppressant dosages were inconsistent, indicating that the amount of VOCs was also inconsistent. The peak area values of the samples with smoke suppressant were significantly lower than those of the samples without smoke suppressant, indicating that the amount of VOCs was also lower. 2) At 0–10 min, the quantity of detected VOCs in each sample was small, indicating that the quantity of organic components with a boiling point <150°C released from the SBS-modified asphalt fumes was lower. Additionally, there was little difference in the quantity of VOCs in each sample at 0–5 min. However, the quantity of VOCs in the sample with smoke suppressant was lower than that in the sample without smoke suppressant at 5–10 min, indicating that the smoke suppressant had no obvious effect on the release of organic components with a boiling point <100°C, but it began to inhibit the release of organic components with a boiling point >100°C. 3) At 10–20 min, the quantity of VOCs in each sample was large and achieved the highest value at 15–20 min, indicating that the SBS-modified asphalt fumes released more organic components with a high boiling point and that the boiling point was concentrated at 150°C–250 °C. The quantity of VOCs started decreasing at 20–25 min. At > 25 min, the quantity of VOCs decreased to a lower value, indicating that the SBS-modified asphalt fumes contained very few organic components with a boiling point >300°C. 4) After 5 min, the quantity of VOCs in the sample with smoke suppressant was lower than that of the sample without smoke suppressant. The peak area of the sample with a smoke suppressant dosage of 0.1% was still the smallest, indicating that the smoke suppressant had an inhibition effect on the release of various main organic components with different boiling points in the SBS-modified asphalt fumes and that the inhibition effect was more ideal when the smoke suppressant dosage was 0.1% than when it was 0.08%. 5) When the smoke suppressant dosages were 0.08% and 0.1%, the VOC purification rates were 27.6% and 40.9%, respectively. The PGD test results showed that the VOC purification rates were 38.6% and 47.5% at the smoke suppressant dosages of 0.08% and 0.1%, respectively. This comparison shows that the results of the two test samples with a smoke suppressant dosage of 0.08% differed, whereas the results of the samples with a dosage of 0.1% were similar. However, both tests showed that the smoke suppressant had a good inhibition effect on the VOCs in the SBS-modified asphalt fumes, which verifies the validity of the PGD test results. The causes of the differences were mentioned above. Finally, the two test results showed that the smoke inhibition effect on the SBS-modified asphalt was the best at a smoke suppressant dosage of 0.1%.
5.3 Effect of the smoke suppressant on the VOCs in high viscosity modified asphalt
Similarly, according to the PGD test results, high viscosity modified asphalt samples with smoke suppressant dosages of 0, 0.08%, and 0.1% were selected for the VOC test, and the specified temperature of the collected gas was 180°C. The test results are shown in Figure 12.
The data show that 1) the VOC peak area curves of the three high viscosity modified asphalt samples with different smoke suppressant dosages were clearly different, indicating that the VOC amounts in the three samples differed. The addition of smoke suppressant increased the VOC peak at some times. However, the overall peak area value of the sample with smoke suppressant was still lower than that of the sample without smoke suppressant, indicating that the amount of VOCs in the sample with smoke suppressant was also lower. 2) At 0–5 min, the amount of VOCs in each sample exhibited little difference. At 5–10 min, the amount of VOCs in the sample with smoke suppressant was higher than that of the sample without smoke suppressant, indicating that the inhibition effect of the smoke suppressant on the release of organic components with a relatively low boiling point in the high viscosity modified asphalt fumes was limited, and that the release of organic components within this boiling point range was unstable or even increased due to the addition of the smoke suppressant. 3) At 10–25 min, the quantity of VOCs in the high viscosity modified asphalt was large, achieving the maximum at 15–20 min, indicating that the boiling point of the main organic components released from the high viscosity modified asphalt fume was high and was concentrated at 150°C–300°C. From 10 min after entering till the end of test, the quantity of VOCs in the samples with the smoke suppressant was continuously lower than that of the samples without the smoke suppressant, indicating that the smoke suppressant had a good inhibition effect on the organic components with a high boiling point in the high viscosity modified asphalt, and that the curves of the VOC quantities at different time periods of the two samples with the smoke suppressant dosages of 0.08% and 0.1% were relatively similar. This indicates that there was no significant difference in the VOC inhibition effect between the two smoke suppressant dosages. (4) The VOC purification rates at the smoke suppressant dosages of 0.08% and 0.1% were 30.6% and 36.5%, respectively, corresponding to the PGD test results of 31.9% and 34.2%, respectively. This comparison shows that two test results were similar, which verifies the PGD test results. Because the effect of the two smoke suppressant dosages did not differ much, the best and most cost-effective smoke suppressant dosage for high viscosity modified asphalt is 0.08%. This conclusion is consistent with the conclusion of the PGD tests.
5.4 Effect of the smoke suppressant on the VOCs in TLA
Similarly, TLA samples with smoke suppressant dosages of 0, 0.08%, and 0.1% were selected for the VOC test, and the specified temperature was 170 °C. The test results are shown in Figure 13.
The test results show that 1) the VOC peak area curves of the TLA samples with different smoke suppressant dosages differed, and the quantity of VOCs was also different. The peak area values of the samples with smoke suppressant were generally lower than that of the samples without smoke suppressant; therefore, the VOC release was also lower. 2) At 0–15 min, the quantity of VOCs in each sample exhibited little difference, indicating that the smoke suppressant had no obvious inhibition effect on the release of organic components with a boiling point lower than 200°C in the TLA fumes. 3) The quantity of VOCs at 0–15 min was large and achieved the maximum value at 15–20 min, indicating that the main organic components released from the TLA fumes also had a high boiling point, which was concentrated at 150°C–250°C. From 15 min after entering till the end of test, the amount of VOCs in the sample with the smoke suppressant was always lower than that of the sample without the smoke suppressant, indicating that the release inhibition effect of the organic components with a higher boiling point in the TLA by the smoke suppressant was clearly better. Additionally, the curves of the VOC quantities of the two smoke suppressants dosages at different time periods were similar. Therefore, the inhibition effect on the VOCs was also similar. 4) The VOC purification rates at the smoke suppressant dosages of 0.08% and 0.1% were 38.9% and 44.3%, respectively, and the corresponding PGD test results were 38.1% and 49.6%, respectively. The two test results were similar, confirming the validity of the PGD test results. Both tests showed that there was little difference in the effect of the two smoke suppressant dosages. Therefore, a dosage of 0.08% should also be used as the optimal smoke suppressant dosage for TLA. However, the PGD test results showed that a smoke suppressant dosage of 0.1% had a better SO2 purification rate. Therefore, to combine with the previous analysis, the optimal dosage of smoke suppressant for TLA was determined to be 0.1%.
5.5 Comprehensive analysis
The GC-MS tests confirmed that the smoke suppressant can effectively purify the VOCs in the different types of asphalt and verified the rationality of the PGD test results. Additionally, the VOC release characteristics of the different types of asphalt were different and the effect of the smoke suppressant was also different.
First, the VOC test results showed that the matrix asphalt exhibited peaks in the front, middle, and rear sections, indicating that the main organic components released from it have both low and high boiling points, whereas the peaks of the other three types of asphalt were concentrated in the middle and second half sections, indicating that the main organic components released have high boiling points. The peak area diagram was in bulge form, that is, the isomer peaks were concentrated and released in large amounts. Therefore, it is speculated that matrix asphalt releases not only VOCs with relatively low boiling points, such as short-chain alkanes, organic solvents, benzene hydrocarbons, and others, but also releases VOCs with relatively high boiling points, such as petroleum hydrocarbon volatiles, and others. The other three types of asphalt release VOCs with relatively high boiling points, such as petroleum hydrocarbon volatiles, and contain are few organic substances with relatively low boiling points. However, because there are thousands of volatiles in asphalt and the amount of volatiles released is large, it is necessary to comprehensively determine which organic components in asphalt are the main volatiles, and further tests and studies are needed.
Second, the inhibition effect of the smoke suppressant on organic components with different boiling points released from different asphalts was not consistent. The smoke suppressant exhibited a good inhibition effect on the release of organic components in the matrix and SBS-modified asphalts with lower and higher boiling points. The inhibition effect of the smoke suppressant on the release of organic components in the high viscosity modified asphalt and TLA with lower boiling points was not obvious, but the inhibition effect on the release of organic compounds with higher boiling points was good. Third, at the optimal smoke suppressant dosage, the VOC purification rate for matrix asphalt and TLA was 46.5% and 44.3% respectively, which is ideal. The VOC purification rate for SBS-modified asphalt was 40.9%, which is considered to be good, and that for high viscosity modified asphalt was 30%, which is considered to be acceptable. The results showed that the inhibition effect of the smoke suppressant on the release of different asphalt VOCs was different.
Finally, the total amount of VOCs in TLA was the highest, that in matrix asphalt was similar to that in high viscosity modified asphalt, and that in the SBS-modified asphalt was the lowest. Considering that the specified temperatures of the SBS-modified and high viscosity modified asphalts was significantly higher, SBS should be conducive to reducing the release of VOCs from asphalt. However, the PGD test results showed that the concentration of VOCs in high viscosity modified asphalt was the highest, that in SBS-modified asphalt was similar to TLA, and that in matrix asphalt was the lowest. It was clear that more VOCs were released at the higher set asphalt mixing temperatures. The analysis shows that the main reason for the difference lies in the test state and test method. The asphalt was continuously mixed during the PGD tests, whereas the asphalts in the GC-MS detection method were static. Therefore, the inhibition effect of the network structure formed by SBS on VOCs is slightly weakened when in a state of mixing, and the effect of temperature seems to be the more important factor. Additionally, the principles and detection ranges of the two test methods are also significantly different. Therefore, there are certain differences between the two test results. In fact, the two test methods have their own advantages. The GC-MS test method is more accurate, has a wide range of detection, and covers a variety of organic substances, though the sample preparation is difficult and the test cost is high. The PGD test method is convenient to use, and the asphalt is in the mixing state, which is close to the actual construction state. However, the detection accuracy and range require improvement. Although there are differences between the two test results, they still have commonalities, that is, they demonstrated that the smoke suppressant can inhibit the VOCs in different asphalts, and the data of the influence of the smoke suppressant on the VOC purification rate for the different asphalts measured by the two tests were similar.
6 Analysis of the SEM and EDS test results
This study used SEM to observe and analyze the microstructures of the four types of asphalt before and after the addition of the optimal smoke suppressant dosage. The SEM magnification was 1000 times, and the test results are shown in Figure 14.
Figure 14 shows that 1) the surfaces of the matrix, SBS-modified, and high viscosity modified asphalts were smooth and homogeneous, in which the network structure of SBS-modified asphalt was clearly visible, whereas the network structure of high viscosity modified asphalt, which also contains SBS, was not obvious. The TLA surface contained a small quantity of granular objects, indicating that natural asphalt contains more impurities than petroleum asphalt. 2) The SEM images of the four types of asphalt before and after the addition of smoke suppressant exhibited little difference. After the addition of smoke suppressant, a small quantity of wrinkles were observed on the asphalt surface, and the comparison was only obvious in the matrix asphalt. However, it is also possible that after being frozen by liquid nitrogen, the asphalt sample slowly softened with time and under the influence of the electron beam. When the electron beam hit the sample, the gas inside escaped out of the sample surface, thus causing the wrinkles. Therefore, it was demonstrated that the smoke suppressant had little effect on the microstructure of the asphalt surface after it was fully dissolved in the asphalt. No new network structure was formed, indicating that the chemical reaction between the smoke suppressant and the asphalt does not change the microstructure of the original asphalt, nor does it have much effect on the main performance of the asphalt. In fact, the ideal smoke suppressant should only change the fumes emitted by the asphalt without interfering with the performance of the asphalt itself, which not only ensures the research and development cost of the smoke suppressant but also ensures that it can be applied to other types of asphalt.
This study also used EDS to analyze the elemental composition of the four types of asphalt before and after adding the optimal smoke suppressant dosage. It should be noted that this test is limited to the detection instrument; therefore, only the element after the fourth element is detected, and it is difficult to detect certain elements when their content is very low. Each sample was tested twice and the average value was reported. Figure 15 shows the test results.
The data in Figure 15 show that 1) the weight and atomic percentage of carbon in the four types of asphalt were the highest, indicating that the main constituent element of asphalt is carbon. The weights and atomic percentages of carbon in the matrix, SBS-modified, and high viscosity modified asphalts were >90%, but the weight and atomic percentage of carbon in TLA were a little over 70% and 80%, respectively, indicating that there were more impurities in TLA, which was similar to the conclusion obtained by the SEM analysis. Apart from carbon, sulfur, and oxygen, TLA also contained silicon, aluminum, iron, sodium, calcium, and other trace elements. 2) The weight and atomic percentage of oxygen in TLA were high, both values were approximately 10%, whereas the weight and atomic percentage of oxygen in the other three asphalts were similar, indicating that the oxygen content in TLA was higher. The weight and atomic percentage of sulfur in the four types of asphalt were similar, indicating that the sulfur content in the four types of asphalt was similar. 3) Nitrogen was not detected in the four types of asphalt; however, the PGD tests showed that the asphalt fumes contained trace NO, indicating that the nitrogen content in the asphalts was very low, which may not be detectable by EDS. 4) After the addition of smoke suppressant, the overall changes in the various elements in the four types of asphalt were small. The change in carbon was close to zero, the weight and atomic percentage of oxygen slightly increased, and that of sulfur slightly decreased, indicating that the addition of smoke suppressant did not affect the carbon in a large total amount of asphalt, but did cause a small change in the elements during the transformation process of harmful substances discharged from asphalt. Theoretically, the addition of smoke suppressant only produces chemical reactions with volatile and harmful substances in the asphalt, and the weight and atoms of the elements should remain constant. Therefore, whether the change in the elements is caused by the conversion of volatile organic substances, oxides, and sulfides in the asphalt into non-volatile substances by the smoke suppressant or by the measurement errors of the instrument needs to be analyzed and verified by further comprehensive tests.
Based on the SEM and EDS analysis, it is believed that the smoke suppressant had little effect on the microstructure of the asphalt surface and little effect on the change in the element content in asphalt. Therefore, the smoke suppressant only produced a chemical reaction with volatile and harmful substances in the asphalt and converted them into non-volatile substances. Furthermore, the conversion process did not affect the other main properties of the asphalt; therefore, it has specificity. This characteristic is also conducive to the wide application of the smoke suppressant in other types of asphalt.
7 Conclusion
This study first analyzed the influence of a smoke suppressant on different types of asphalt and its smoke inhibition effect using the PGD test. Then, the influence of the smoke suppressant on the VOCs in different types of asphalt was comprehensively analyzed using GC-MS and compared with the PGD test results. Finally, SEM and EDS were used to observe and analyze the influence of the smoke suppressant on the micro-morphology and elemental composition changes of the different types of asphalt. The main research conclusions are as follows:
(1) The smoke suppressant exhibited a smoke inhibition effect on the four types of asphalt, but the inhibition effect and the optimal smoke suppressant dosage differed. The smoke suppressant exhibited the best inhibition effect on sulfides, and the highest SO2 or H2S purification rates were generally >70%. The smoke suppressant also had a good inhibition effect on NO and VOCs, with the highest NO and VOC purification rate reaching >40% on average. However, the inhibition effect of the smoke suppressant on CO was limited, and the relationship between the smoke suppressant dosage and the change in the purification rate was not stable. Additionally, the purification rate was generally not high.
(2) The smoke inhibition effect of the smoke suppressant on the organic components released from different asphalts was not consistent. The smoke suppressant had a good inhibition effect on the organic components in the matrix and SBS-modified asphalts with lower and higher boiling points. The inhibition effect of the smoke suppressant on the organic components in the high viscosity modified asphalt and TLA with a low boiling point was not obvious, but the inhibition effect on the organic components with a high boiling point was good. The GC-MS test results further confirmed that the smoke suppressant effectively inhibited the VOCs in the different types of asphalt and that the inhibition effect was different, which verified the accuracy and rationality of the PGD test results. Additionally, the influence data of the two test results on the VOC purification rate of the different asphalts were roughly close, and there was a certain similarity.
(3) The PGD tests showed that the smoke suppressant had the best effect on matrix asphalt, followed by SBS-modified asphalt, and the total purification rate for both was >40.0%. The effect of the smoke suppressant on high viscosity modified asphalt and TLA was slightly weak, and the total purification rate for both was >30%. The GC-MS tests showed that the VOC inhibition effect of the smoke suppressant on matrix asphalt was the best, followed by the TLA and SBS-modified asphalt. The VOC purification rate for the three types of asphalt was >40%. The VOC purification rate of the smoke suppressant for the high viscosity modified asphalt was the weakest. However, it still reached >30%. Therefore, the smoke suppressant exhibited the best compatibility with the matrix asphalt and the weakest compatibility with the high viscosity modified asphalt. Finally, based on the results of two tests, the optimal smoke suppressant dosage for matrix asphalt, SBS-modified asphalt, high viscosity modified asphalt, and TLA were determined to be 0.05%, 0.1%, 0.08%, and 0.1%, respectively.
(4) The smoke suppressant had little effect on the microstructure of the asphalt surfaces and the change in the elemental content in the asphalts. Therefore, the smoke suppressant only produced chemical reactions with volatile and harmful substances in the asphalt and converted them into non-volatile substances. The reaction process had no impact on the main performance of the asphalt itself. This characteristic is also conducive to the wide application of the smoke suppressant in various types of asphalt.
The above conclusions not only explore the influence and action of the smoke suppressant on harmful gas emissions, microstructure, and elemental changes in different asphalts, but also concluded that there were similarities between the two tests in evaluating the influence of the smoke suppressant on asphalt by comparing the PGD and GC-MS test results in terms of VOCs. Concurrently, it was also confirmed that this type of smoke suppressant, which is mainly composed of aldehydes and carboxylic ester compounds, can react with the gas molecules in asphalt fumes, effectively decompose SO2, H2S, NO, VOCs, and other gases in the smoke, and inhibit the volatilization of harmful substances.
The recommended dosages of smoke suppressant drawn from this study can provide a suitable reference value for the corresponding type of asphalt. However, in practice, the optimal smoke suppressant dosage may vary slightly due to differences in the oil source and refining process of asphalt, but the appropriate dosage for the same type of asphalt will not differ significantly. The difference in the optimal smoke suppressant dosage for different types of asphalt further indicates that the reaction mechanism of the smoke suppressant with different asphalts is different and cannot be generalized. It is more appropriate to conduct tests before using each asphalt to determine the optimal smoke suppressant dosage. Additionally, the smoke suppressant is of low cost. For example, the cost of one ton of matrix asphalt with a smoke suppressant dosage of 0.05% only increases the cost by approximately US $30, which makes it widely applicable.
The results of this study are helpful to determine the influence of smoke suppressants on the smoke inhibition effect and working properties of different types of asphalt and can provide guidance and a basis for the development of low-smoke asphalt with good emission reduction effects. The research process and experience also provide relevant guidance and suggestions for peers. This study has good practical value and promotional significance. However, this study has some limitations. Only six types of gases were analyzed with the PGD test, and the coverage was limited. The GC-MS detection test only detected VOCs. This study did not perform a comprehensive microscopic analysis of how the smoke suppressant reacts with asphalt to inhibit the emission of harmful gases. Therefore, follow-up research should focus on exploring these aspects.
Data availability statement
The original contributions presented in the study are included in the article/Supplementary Material, further inquiries can be directed to the corresponding author.
Author contributions
HL writing/original draft preparation, investigation, and formal analysis. PZ conceptualization, supervision, and resources. RX and JL conducted the experiments. XL methodology. XX and LS writing-review and editing. All authors listed have made a substantial, direct, and intellectual contribution to the work and approved it for publication.
Funding
This study was supported by Guangdong Basic and Applied Basic Research Foundation (Grant No 2021A1515110602 and 2022A1515010845), Special Fund for Science and Technology Innovation Cultivation of Guangdong University Students (Grant No pdjh2023b0550). The authors would like to express their sincere appreciation to the organization.
Conflict of interest
Author PZ is employed by the company Shenzhen Oceanpower Industrial Co., Ltd.
The remaining authors declare that the research was conducted in the absence of any commercial or financial relationships that could be construed as a potential conflict of interest.
Publisher’s note
All claims expressed in this article are solely those of the authors and do not necessarily represent those of their affiliated organizations, or those of the publisher, the editors and the reviewers. Any product that may be evaluated in this article, or claim that may be made by its manufacturer, is not guaranteed or endorsed by the publisher.
References
Autelitano, F., and Giuliani, F. (2018). Analytical assessment of asphalt odor patterns in hot mix asphalt production. J. Clean. Prod. 172, 1212–1223. doi:10.1016/j.jclepro.2017.10.248
Cui, P. (2015). Research methodologies on the VOC emissions from bituminous materials and its inhibitor. Wuhan, China: Wuhan University of Technology. [dissertation/doctor's thesis]. [Wuhan (CHN)].
Cui, P., Wu, S., Li, F., Xiao, Y., and Zhang, H. (2014). Investigation on using SBS and active carbon filler to reduce the VOC emission from bituminous materials. Materials 7 (9), 6130–6143. doi:10.3390/ma7096130
Dash, S. S., Chandrappa, A. K., and Sahoo, U. C. (2022). Design and performance of cold mix asphalt - a review. Constr. Build. Mater. 315, 125687. doi:10.1016/j.conbuildmat.2021.125687
Feng, X., Zha, X., and Feng, Z. (2013). Study on application technology of Trinidad Lake asphalt. Guangdong Highw. Commun. 2, 12–19. doi:10.3969/j.issn.1671-7619.2013.02.004
Hansen, K. R., Copeland, A., and Ross, T. (2017). Asphalt pavement industry survey on recycled materials and warm-mix asphalt usage 2016 (Information Series 138) 7th Annual Survey. Greenbelt, Maryland: National Asphalt Pavement Association. doi:10.13140/RG.2.2.24846.05449
Hasan, M. R. M., You, Z., and Yang, X. (2017). A comprehensive review of theory, development, and implementation of warm mix asphalt using foaming techniques. Constr. Build. Mater. 152, 115–133. doi:10.1016/j.conbuildmat.2017.06.135
He, R., Zheng, S., Chen, H., and Kuang, D. (2019). Investigation of the physical and rheological properties of Trinidad lake asphalt modified bitumen. Constr. Build. Mater. 203, 734–739. doi:10.1016/j.conbuildmat.2019.01.120
Huang, G., He, Z., Huang, Y., Zhou, C., and Yuan, X. (2014). Mechanism of fume suppression and performance on asphalt of expanded graphite for pavement under high temperature condition. J. Wuhan Univ. Technology-Mater. Sci. Ed. 29, 1229–1236. doi:10.1007/s11595-014-1073-6
Huang, G., He, Z., Zhou, C., Lin, Z., Song, S., and Wu, C. (2015). Discriminative clustering via extreme learning machine. China J. Highw. Transp. 28 (10), 1–8. doi:10.1016/j.neunet.2015.06.002
Li, S., Fan, M., Xu, L., Tian, W., Yu, H., and Xu, K. (2021). Rutting performance of semi-rigid base pavement in RIOHTrack and laboratory evaluation. Front. Mater. 7, 590604. doi:10.3389/fmats.2020.590604
Lin, B., Shi, L., Zhang, X., Liang, H., and Wang, D. (2022). Study on meso-structural characteristics and homogeneity of asphalt mixture skeleton contacts. Case Stud. Constr. Mater. 17, e01277. doi:10.1016/j.cscm.2022.e01277
Lin, S., Hung, W., and Leng, Z. (2016). Air pollutant emissions and acoustic performance of hot mix asphalts. Constr. Build. Mater. 129, 1–10. doi:10.1016/j.conbuildmat.2016.11.013
Mo, S., Wang, Y., Xiong, F., and Ai, C. (2019). Effects of asphalt source and mixing temperature on the generated asphalt fumes. J. Hazard. Mater. 371, 342–351. doi:10.1016/j.jhazmat.2019.03.025
Peng, X., Qian, S., Xiao, F., Zhang, P., and Li, Z. Y. (2011). The experimental study on the road asphalt fumes suppressive effect of SBS and nano calcium carbonate. Adv. Mater. Res. 233-235, 507–511. doi:10.4028/www.scientific.net/amr.233-235.507.233-235.507
Qu, S., Fan, S., Wang, G., He, W., Xu, K., Nie, L., et al. (2021). Air pollutant emissions from the asphalt industry in Beijing, China. J. Environ. Sci. 109, 57–65. doi:10.1016/j.jes.2021.02.027
Research Institute of Highway Ministry of Transport (2011). Standard test methods of bitumen and bituminous mixtures for highway engineering (JTG E20-2011). Beijing, China: China Communications Press. [Beijing(CHN)].
Research Institute of Highway Ministry of Transport (2004). Technical specifications for construction of highway asphalt pavements (JTG F40-2004). Beijing, China: China Communications Press. [Beijing(CHN)].
Rhomberg, L. R., Mayfield, D. B., Goodman, E. J., Butler, E. L., Nascarella, M. A., and Williams, D. R. (2015). Quantitative cancer risk assessment for occupational exposures to asphalt fumes during built-up roofing asphalt (BURA) operations. Crit. Rev. Toxicol. 45 (10), 873–918. doi:10.3109/10408444.2015.1094450
Rubio, M. D., Moreno, F., Martinez-Echevarria, M. J., Martínez, G., and Vázquez, J. M. (2013). Comparative analysis of emissions from the manufacture and use of hot and half warm mix asphalt. J. Clean. Prod. 41, 1–6. doi:10.1016/j.jclepro.2012.09.036
Shanbara, H. K., Dulaimi, A., Al-Mansoori, T., Al-Busaltan, S., Herez, M., Sadique, M., et al. (2021). The future of eco-friendly cold mix asphalt. Renew. Sustain. Energy Rev. 149, 111318. doi:10.1016/j.rser.2021.111318
Shi, L., Xiao, X., Wang, X., Liang, H., and Wang, D. (2022). Mesostructural characteristics and evaluation of asphalt mixture contact chain complex networks. Constr. Build. Mater. 340, 127753. doi:10.1016/j.conbuildmat.2022.127753
Sun, X., Ou, Z., Xu, Q., Qin, X., Guo, Y., Lin, J., et al. (2023). Feasibility analysis of resource application of waste incineration fly ash in asphalt pavement materials. Environ. Sci. Pollut. Res. 30, 5242–5257. doi:10.1007/s11356-022-22485-z
Tang, N., Yang, K., Alrefaei, Y., Dai, J. G., Wu, L. M., and Wang, Q. (2020). Reduce VOCs and PM emissions of warm-mix asphalt using geopolymer additives. Constr. Build. Mater. 244, 118338. doi:10.1016/j.conbuildmat.2020.118338
Toraldo, E., Martinez-Arguelles, G., and Mariani, E. (2014). “Effect of reinforced fibers on stiffness of recycled asphalt half-warm emulsion mixes. Sustainability, Eco-efficiency, and Conservation in Transportation Infrastructure Asset Management,” in Proceedings paper. 3rd international conference on transportation infrastructure (Amsterdam, Netherlands: Elsevier), 53–59. doi:10.1201/b16730-10
Wang, H., Huang, W., Cong, Y., Fu, H. R., Wang, X. D., Wang, L., et al. (2020). The phosphodiesterase-4 inhibitor roflumilast, a potential treatment for the comorbidity of memory loss and depression in alzheimer's disease: A preclinical study in app/PS1 transgenic mice. J. Build. Mater. 23 (3), 700–711. doi:10.1093/ijnp/pyaa048
Wu, K., Zhu, K., Kang, C., Wu, B., and Huang, Z. (2016). An experimental investigation of flame retardant mechanism of hydrated lime in asphalt mastics. Mater. Des. 103, 223–229. doi:10.1016/j.matdes.2016.04.057
Wu, X., Vu, T. V., Shi, Z., Harrison, R. M., Liu, D., and Cen, K. (2018). Characterization and source apportionment of carbonaceous PM2. 5 particles in China-A review. Atmos. Environ. 189, 187–212. doi:10.1016/j.atmosenv.2018.06.025
Xiao, Y., Wan, M., Jenkins, K. J., Wu, S. P., and Cui, P. Q. (2017). Using activated carbon to reduce the volatile organic compounds from bituminous materials. J. Mater. Civ. Eng. 29 (10), 04017166. doi:10.1061/(ASCE)MT.1943-5533.0002024
Yang, X., Wang, G., Rong, H., Meng, Y., Liu, X., Liu, Y., et al. (2022). Review of fume-generation mechanism, test methods, and fume suppressants of asphalt materials. J. Clean. Prod. 347, 131240. doi:10.1016/j.jclepro.2022.131240
Zhang, M., Lian, C., Wang, J., Wang, H., and Cheng, B. (2022). Modification of asphalt modified by packaging waste EVA and graphene oxide. Front. Mater. 9, 833593. doi:10.3389/fmats.2022.833593
Keywords: environment protection, asphalt, asphalt fume, volatile organic compounds, smoke suppressant
Citation: Liang H, Zhao P, Xu R, Liang X, Xiao X, Shi L and Lv J (2023) Influence of smoke suppressant on the smoke inhibition effect and properties of different types of asphalt. Front. Mater. 10:1205050. doi: 10.3389/fmats.2023.1205050
Received: 13 April 2023; Accepted: 08 May 2023;
Published: 22 May 2023.
Edited by:
Xiaolong Sun, Guangdong University of Technology, ChinaReviewed by:
Qian Chen, Chang’an University, ChinaChaoliang Fu, RWTH Aachen University, Germany
Ran Zhang, Beijing University of Civil Engineering and Architecture, China
Copyright © 2023 Liang, Zhao, Xu, Liang, Xiao, Shi and Lv. This is an open-access article distributed under the terms of the Creative Commons Attribution License (CC BY). The use, distribution or reproduction in other forums is permitted, provided the original author(s) and the copyright owner(s) are credited and that the original publication in this journal is cited, in accordance with accepted academic practice. No use, distribution or reproduction is permitted which does not comply with these terms.
*Correspondence: Pu Zhao, zhaopuszhc@163.com