- 1School of Pharmacy, Hebei Medical University, Shijiazhuang, China
- 2College of Animal Science and Veterinary Medicine, Shandong Agricultural University, Tai’an, China
A type II band aligned BaTiO3/CeO2 photocatalysts was constructed by a simple one-step solution synthesis method. The BaTiO3/CeO2 photocatalysts contain only cubic phase BaTiO3 and CeO2, and the particles are approximately spherical with a small amount of adhesion and agglomeration, and the average particle size is about 50 nm. Transmission electron microscopy (TEM) observation and element Mapping characterization confirmed the formation of special heterojunction between BaTiO3 and CeO2 and the presence of a large number of oxygen vacancies, which resulted in high visible optical absorption coefficient. The BaTiO3/CeO2 photocatalysts demonstrated high photocatalytic activity for the degradation of oxytetracycin hydrochloride, and the optimal drug concentration, catalyst content and pH value are 50 mg/L, 1 g/L and 7, respectively. Simultaneously, the BaTiO3/CeO2 photocatalysts were selective for degradation of aureomycin hydrochloride, doxycycline hydrochloride and tetracycline hydrochloride. Other photocatalytic experiments show that the BaTiO3/CeO2 photocatalysts have high chemical and cyclic stability, and the synergistic effect of holes, hydroxyl radicals and superoxide radicals plays an important role in the whole photocatalytic process. This simple synthesis route provides a technical reference for constructing other wide-band gap semiconductors to respond to simulated sunlight.
1 Introduction
In the process of production and life, human beings depend very much on drugs, especially antibiotics (Verma et al., 2022). When many diseases occur, people use large amounts of antibiotics, but only a small part of these antibiotics are absorbed by the body, most of which will be excreted in the stool (Nazarov, 2022; Li et al., 2023; Wang et al., 2023). If these excluded antibiotics are discharged directly into the environment, it will cause great pollution to the living environment. In the process of aquaculture, in order to make aquaculture products high yield and reduce morbidity, a large number of antibiotics will be used (Okeke et al., 2022; Tong et al., 2022). To reduce the effects of antibiotics on ecological environment and water environment, antibiotics should be degraded from the source.
Photocatalysis is a green technology that can use sunlight to degrade antibiotics, thus reducing environmental pollution (Han et al., 2022; Sahoo et al., 2022; Wang et al., 2022; Zhang et al., 2022). The key to the photocatalytic degradation of antibiotics is the selection of photocatalyst, and the semiconductor photocatalyst that can respond to visible light should be selected in order to effectively utilize sunlight. Barium titanate (BaTiO3) and cerium dioxide (CeO2) are two common photocatalysts, but they can only respond to ultraviolet light because of their large optical band gap (Gu et al., 2022; Xiao et al., 2022; Masekela et al., 2023; Passi and Pal, 2023). Therefore, researchers are focusing on enhancing their light response, especially to simulated sunlight, through ion doping, surface modification, and heterostructural construction (Cheng et al., 2022; Gao et al., 2022; Prajapati et al., 2022). Generally, when heterojunctions are constructed, the coupling of semiconductor materials with a small band gap that can respond to simulated sunlight and semiconductor materials with a wide band gap is selected to enhance the photocatalytic activity of the system (Jing et al., 2022; Masula et al., 2022; Yu et al., 2023). Although BaTiO3 and CeO2 have been coupled together, there are no reports of coupling the two to construct special oxygen vacancies to study their photocatalytic activity (Tarasevich and Lebedev, 2010; Khatkhatay et al., 2013; Rehman et al., 2021; Yan et al., 2022a; Yan et al., 2022b).
In this paper, we propose a novel one-step solution synthesis method to construct a type II band aligned BaTiO3/CeO2 photocatalysts and investigate its photocatalytic activity. The phase structure, microstructure, optical properties and photocatalytic activity of BaTiO3/CeO2 photocatalysts were studied by various characterization methods. By studying the effects of different drug concentrations, catalyst contents, pH values and antibiotics on the photocatalytic activity of BaTiO3/CeO2 photocatalyst, the optimal experimental conditions and the mechanism of drug selectivity of BaTiO3/CeO2 photocatalysts were revealed. Based on a series of photocatalytic experiments and band arrangement theory, a reasonable photocatalytic mechanism of BaTiO3/CeO2 photocatalysts for the degradation of drugs has been proposed.
2 Experimental
2.1 Starting materials
Tetrabutyl titanate (CHO₄Ti, 99.5%), barium nitrate (Ba(NO3)2, 99.5%), Cerium sulfate (Ce2(SO4)3·8H2O, 99.5%), oxalic acid (H2C2O4, AR), urea (CO(NH2)2, AR), acrylamide (C3H5NO, AR), and ammonium hydroxide (NH3·H2O, AR) were purchased from Aladdin Reagent (Shanghai) Co., Ltd. These reagents are not further purified when used to prepare BaTiO3/CeO2 photocatalysts.
2.2 One step synthesize of BaTiO3/CeO2 photocatalysts
According to the molar ratio of nBaTiO3: nCeO2 = 1:1, stoichiometric amounts of CHO₄Ti, Ba(NO3)2 or Ce2(SO4)3·8H2O were sequential dissolved in a beaker containing 50 mL of deionized water to form a solution of 0.03 mol/L (Relative to metal ions). Subsequently, the dissolution process of the above reagent was observed. After being completely dissolved, 5 g oxalic acid is added to make it fully complex with metal ions to form a complex. After the reagent continued to react uniformly without precipitation, 10 g urea was added as an accelerant. Acrylamide is added within 2 min after the accelerant is added as a network precursor that restricts the free movement of metal ions. After the reagent was completely dissolved, the pH value of the reaction solution was adjusted to 4 with ammonia water, and the deionized water was continuously added to reach 200 mL. After pH is adjusted, heat the oil bath to 90°C until black gel appears. The black gel was then placed in a drying oven to dry at 120°C for 48 h to obtain xerogel. The xerogel was then placed in a tubular sintering furnace at 700°C for 10 h to obtain the final products. Figure 1 shows the illustrated formation process of BaTiO3/CeO2 photocatalysts. For comparison, single-component BaTiO3 and CeO2 photocatalysts were synthesized using the same method. The BaTiO3, CeO2 and BaTiO3/CeO2 photocatalysts are marked as Samples S-1, S-2 and S-3, respectively.
2.3 Sample measurement
The BaTiO3, CeO2 and BaTiO3/CeO2 photocatalysts were characterized by X-ray powder diffraction (XRD), performed on a DX-2007BH diffractometer at room temperature to inquire the phase structure and purity. The morphology microstructures of BaTiO3, CeO2 and BaTiO3/CeO2 photocatalysts were recorded by HITACHI S-4800 type field emission scanning electron microscopy (FE-SEM) and JEOL JEM-2100 type high-resolution transmission electron microscopy (TEM). Ultraviolet–visible absorption spectra measurements of BaTiO3, CeO2 and BaTiO3/CeO2 photocatalysts were performed on a UV1901 UV-Visible spectrophotometer.
2.4 Photocatalytic activity measurements
The photocatalytic degradation of oxytetracycin hydrochloride (OH), aureomycin hydrochloride (AH), doxycycline hydrochloride (DH), or tetracycline hydrochloride (TH) solution by simulated sunlight was studied using BaTiO3, CeO2 and BaTiO3/CeO2 photocatalysts. The degradation of OH, AH, DH or TH was investigated in a Pyrex beaker under simulated sunlight irradiation by placing the beaker in a Xenon lamp light source. In each experiment, 0.05, 0.10, 0.15 or 0.20 g of BaTiO3, CeO2 or BaTiO3/CeO2 photocatalysts was added to 100 mL of aqueous solution containing 10, 30, 50, 70 or 90 mg/L drug. Prior to illumination, the above solution was magnetically stirred in the dark for 30 min to disperse the BaTiO3, CeO2 or BaTiO3/CeO2 photocatalysts and establish an adsorption/desorption equilibrium to exclude the effect of adsorption on photocatalysis. After that, the solution was irradiated with simulated sunlight irradiation. During simulated sunlight irradiation process, magnetically stirring was continuously executed to keep the homogeneous mixture in suspension. Every 30 min, 3 mL of the drug solution was sampled and centrifugated immediately to remove the BaTiO3, CeO2 or BaTiO3/CeO2 photocatalysts. Then the supernatant solution was analyzed by recording the absorbance curve of the remained drug using a UV-visible spectrophotometer. The degradation percentage of BaTiO3, CeO2 or BaTiO3/CeO2 photocatalysts is named as ((A0 − At)/A0) × 100%, where A0 and At are the drug absorbance (OH, AH, DH or TH) before and after simulated sunlight irradiation, respectively. To perform the capture experiment, the ethylenediaminetetraacetic acid disodium salt (EDTA-2Na), isopropyl alcohol (IPA), and p-benzoquinone (BQ) have been used to detect the holes, hydroxyl radicals, and superoxide radicals, respectively. Different from the photocatalytic experiment, the capture experiment requires adding 1 mmol of the trapping agent in the reaction solution.
3 Results and discussions
3.1 Phase structure and purity
The XRD diffraction patterns of BaTiO3, CeO2 and BaTiO3/CeO2 photocatalysts are displayed in Figure 2. For the Sample S-1, all peaks indexed as (100), (101), (111), (002), (102), (211), (202), (310), (311), (222), and (320) in XRD patterns are ascribed to cubic BaTiO3 phase with the space group: Pm-3 m (221) according to JCPDS standard card number: 74-1996. The diffraction peaks of Sample S-2 can be indexed as the (111), (200), (220), (311), (222), (400), (331), and (420) planes of CeO2 with the JCPDS standard card number: 01-071-6329 and the space group: Fm-3 m (225), showing their cubic structure. When BaTiO3/CeO2 photocatalysts (Sample S-3) are synthesized in one-step synthesis method, the sample contains only the diffraction peaks of BaTiO3 and CeO2, but no other impurity peaks. The average crystal size (D) of BaTiO3, CeO2 or BaTiO3/CeO2 photocatalysts is determined from by broadening the diffraction peaks (111), (101), (211), and (311) plane using Debye-Scherer’s formula (Chavan et al., 2017).
Where, k is the constant, k = 0.9 is used in the work due to the spherical nanoparticles, λ is the wavelength of the X-ray (λ = 1.5406 Å), B is the broadening of the diffraction line measured at half maximum intensity, and θ is the diffraction angle. The D values of Samples S-1, S-2 and S-3 were calculated to be 75 nm, 38 nm, and 47 nm, respectively. To further validate the synthesis of nanoscale particles by one-step synthesis method, microstructure characterization is needed.
3.2 Microstructure characterization
Figures 3A–C shows the SEM images of Samples S-1, S-2 and S-3. The BaTiO3, CeO2 or BaTiO3/CeO2 photocatalysts were nanocrystalline with similar morphology of particles. These particles are approximately spherical, with a few particles showing slight adhesion agglomeration. To calculate the particle size distribution of these nanoparticles, 100 particles from each sample were used for statistics. Figures 3D–F shows the particle size distributions of Samples S-1, S-2 and S-3. The average particle size of Samples S-1, S-2 and S-3 were calculated to be 80 nm, 40 nm, and 50 nm, respectively. The results observed by SEM are similar to those calculated by XRD. Compared with BaTiO3, the particle size of BaTiO3/CeO2 photocatalysts decreased significantly. The main reason for this phenomenon may be that cerium ions affect the coordination environment of barium and titanium ions.
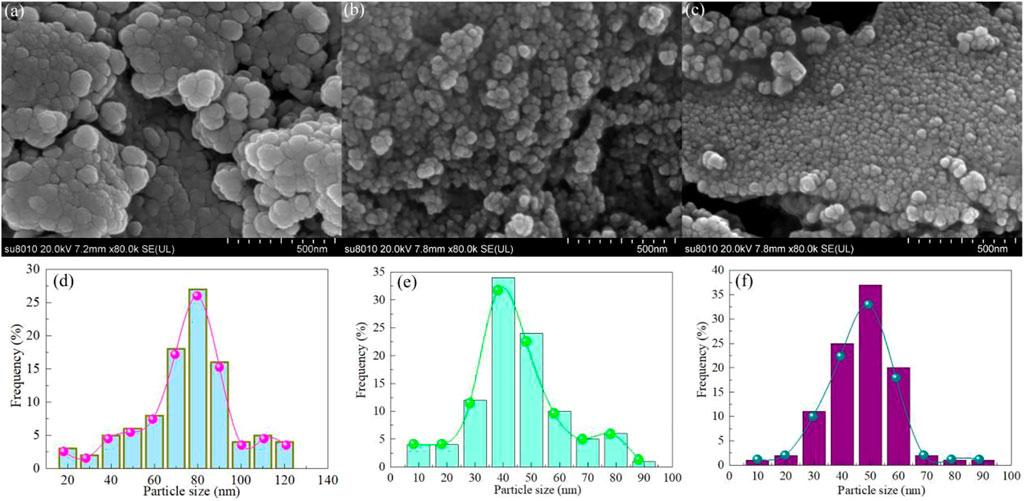
FIGURE 3. The SEM images of Samples (A) S-1, (B) S-2 and (C) S-3. Particle size distributions of Samples (D) S-1, (E) S-2 and (F) S-3.
TEM and element Mapping characterization experiments were performed to further confirm the formation of heterojunction between BaTiO3 and CeO2. Figure 4A shows the TEM image of Sample S-3. As can be seen from the Figure 4A, the outlines of large particles and fine particles are very clear, consistent with SEM observation results. Figure 4B shows the high-resolution TEM (HRTEM) image of Sample S-3. The interplanar spacing of large and fine particles is 0.28 nm and 0.31 nm, respectively, corresponding to the (101) crystal plane of BaTiO3 and the (111) crystal plane of CeO2. The BaTiO3 particles and CeO2 particles are closely connected, forming a special heterojunction structure. To further explore the heterojunction formed between BaTiO3 and CeO2, the relevant BF-TEM, EDS layered images and element Mapping images are given in Figures 4C–H. It can be seen from the figure that CeO2 is evenly dispersed on the BaTiO3 parent, indicating that a heterojunction is formed between BaTiO3 and CeO2.
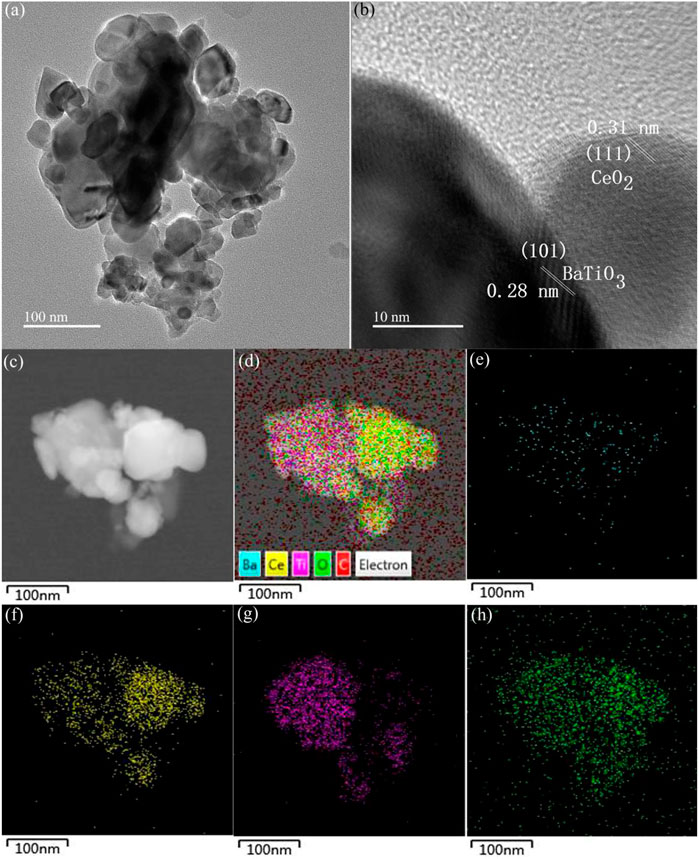
FIGURE 4. (A) TEM, (B) HRTEM, (C) BF-TEM, (D) EDS layered images of Sample S-3. The elemental maps of the region shown in panel (C). (E) Ba, (F) Ti, (G) Ce and (H) O.
3.3 Optical properties and Eg values
Figure 5A shows the UV-vis absorption spectra of Samples S-1, S-2 and S-3. In the range of 230 nm–800 nm, the optical absorption coefficients of the three samples decreased with the increase of wavelength. In the UV range, Sample S-2 has the highest optical absorption coefficient, Sample S-1 has the lowest optical absorption coefficient. In the visible range, Sample S-3 has a high optical absorption coefficient, which may be caused by the appearance of a large amount of surface defect oxygen at the interface of BaTiO3 and CeO2 after the formation of heterojunction (Yu et al., 2023). This phenomenon will be conducive to BaTiO3/CeO2 photocatalyst producing visible light photocatalytic activity for degradation of drugs. Figures 5B–D shows the estimated band gap (Eg) of Samples S-1, S-2 and S-3. The Eg values of Samples S-1, S-2 and S-3 were calculated to be 3.06, 3.15, and 2.92 eV, respectively. In general, two semiconductors coupled together do not change the Eg value of the main lattice. However, when BaTiO3 and CeO2 are coupled, the obvious decrease of Eg value may be due to the presence of a large amount of surface defect oxygen at the interface of the two.
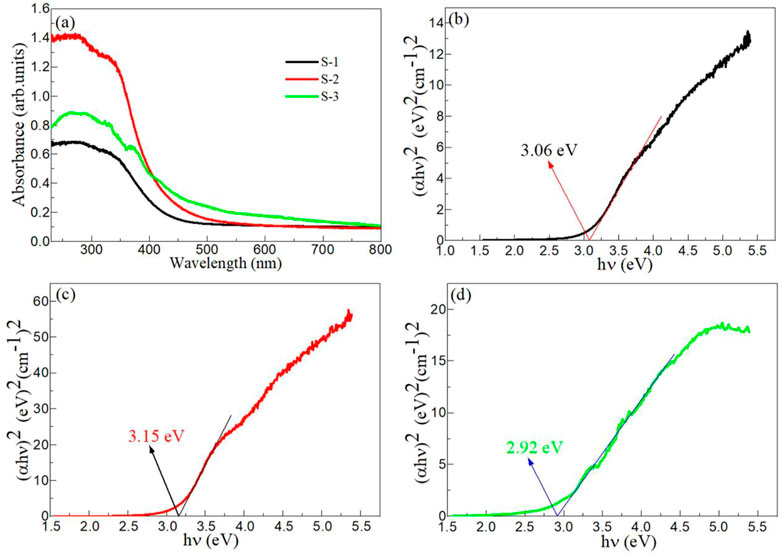
FIGURE 5. (A) UV-vis absorption spectra of Samples S-1, S-2 and S-3. The estimated band gap of Samples (B) S-1, (C) S-2 and (D) S-3.
3.4 Photocatalytic activity
Figure 6A shows the photocatalytic oxytetracycin hydrochloride (OH) degradation over BaTiO3, CeO2 and BaTiO3/CeO2 photocatalysts under visible light irradiation. In order to verify whether OH can be degraded without photocatalyst under visible light irradiation conditions, blank experiments were performed. After 3 h of light exposure, OH without catalyst is difficult to be degraded by visible light, indicating that OH is difficult to be degraded naturally under visible light conditions. With the increase of the time of visible light, the degradation rate of OH in all samples increased continuously. The photocatalytic degradation rate of BaTiO3 and CeO2 with single component is very low, while the photocatalytic degradation rate of BaTiO3/CeO2 composite photocatalyst is the highest. Figure 6B shows the -ln (At/A0) ∼ time curve for the photodegradation of OH over BaTiO3, CeO2 and BaTiO3/CeO2 photocatalysts. It shows a good linear relationship between -ln (At/A0) and time. First-order rate constant (k) for the photodegradation of OH over BaTiO3, CeO2 and BaTiO3/CeO2 photocatalysts as displayed in Figure 6C. The k values of BaTiO3, CeO2 and BaTiO3/CeO2 photocatalysts are 0.00379, 0.00486 and 0.01248 min−1, respectively. The degradation rate of BaTiO3/CeO2 photocatalysts is 15.80 times that of the blank experiment, and 3.29 times that of BaTiO3. Figure 6D shows the degradation percentage for the photodegradation of OH over BaTiO3, CeO2 and BaTiO3/CeO2 photocatalysts. The degradation percentage of BaTiO3, CeO2 and BaTiO3/CeO2 photocatalysts are 51%, 61%, and 91%, respectively. The results showed that the BaTiO3/CeO2 photocatalysts showed high photocatalytic activity for the degradation of OH.
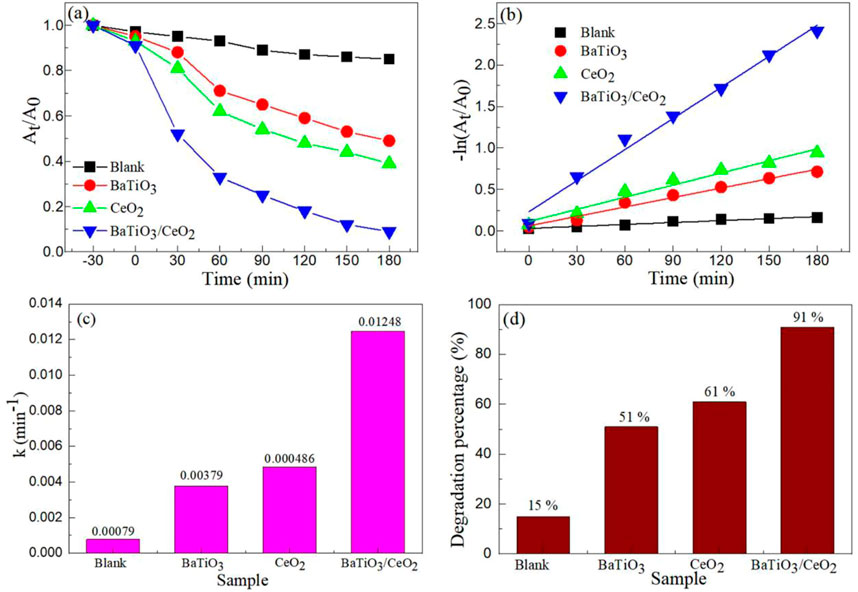
FIGURE 6. (A) Photocatalytic oxytetracycin hydrochloride (OH) degradation over BaTiO3, CeO2 and BaTiO3/CeO2 photocatalysts under visible light irradiation. (B) -ln (At/A0) ∼ time curve, (C) First-order rate constants and (D) Degradation percentage for the photodegradation of OH over BaTiO3, CeO2 and BaTiO3/CeO2 photocatalysts.
The photocatalytic activity of photocatalyst is affected by drug concentration, catalyst content and pH value. Figure 7A shows the OH degradation yields at different drug concentrations. With the increase of drug concentration, the degradation percentage of BaTiO3/CeO2 photocatalysts initially increased with the increase of drug concentration, but decreased with the increase of drug concentration when the drug concentration reached 50 mg/L. The reason why the photocatalytic activity decreases under high drug concentration is mainly due to the shielding effect of light, which reduces the chance of photogenerated carrier generation (Kaur et al., 2016). As can be seen from Figure 7A, the optimal drug concentration is 50 mg/L. Figure 7B shows the OH degradation yields at different catalyst contents. Similar to the concentration of different drugs, the photocatalytic activity of BaTiO3/CeO2 photocatalysts firstly increased and then decreased with the increase of catalyst content. When the concentration of photocatalyst is too high, the path length of photon entering the reaction solution becomes longer due to the light scattering effect, and the separation and transfer rate of photogenerated carrier decreases, which leads to the decrease of photocatalytic activity (Hiragond et al., 2022). The optimal catalyst content was 1 g/L of BaTiO3/CeO2 photocatalysts for the degradation of OH. Figure 7C shows the OH degradation yields at different pH values. It can be seen from the figure that the BaTiO3/CeO2 photocatalysts showed the highest photocatalytic activity in the degradation of OH under neutral and alkaline conditions. The main reason for this phenomenon is that the charge states on the surface of BaTiO3/CeO2 photocatalysts and drug molecules show different electrical properties under different acid-base conditions. To verify this hypothesis, Figure 7D shows the pH value of the reaction solution before and after photocatalysis. The PZC value of BaTiO3/CeO2 photocatalysts is 6.18. Under acidic conditions, the degradation rate of BaTiO3/CeO2 photocatalysts is significantly lower than that of basic conditions. In the neutral and basic condition, the degradation rate of BaTiO3/CeO2 photocatalysts reached the maximum, which was basically consistent with the value of PZC. When pH < PZC, the surface of BaTiO3/CeO2 photocatalysts presents positive charge, while when pH > PZC presents negative charge (Zeng, 2011; Mahamud et al., 2023). The BaTiO3/CeO2 photocatalysts can degrade OH in a neutral and basic environment. The relevant chemical expression can be described as follows:
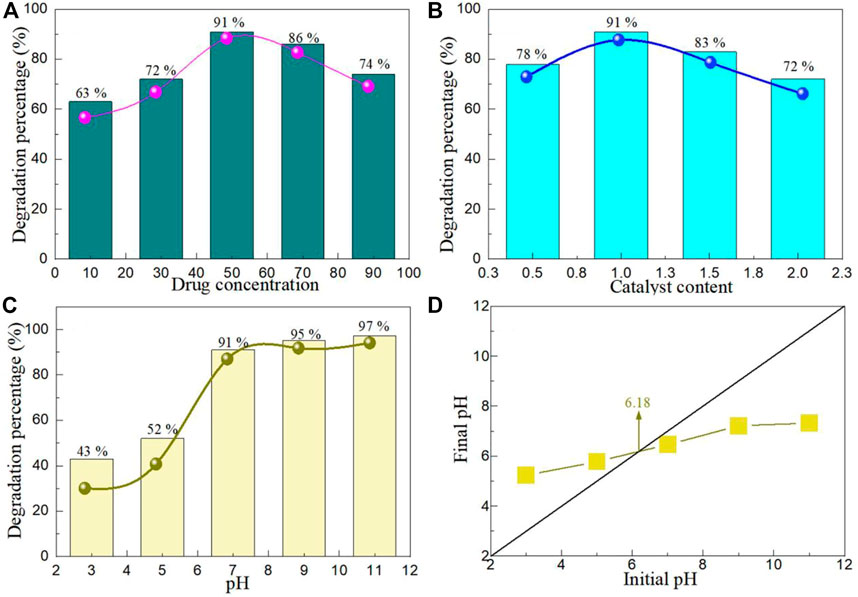
FIGURE 7. OH degradation yields at (A) different drug concentrations, (B) different catalyst contents, (C) different pH values. (D) The pH value of the reaction solution before and after photocatalysis.
The photocatalyst needs high stability and reuse to be used in industrial applications. In order to prove that the BaTiO3/CeO2 photocatalysts can be applied commercially, Figure 8A shows the recyclability tests of BaTiO3/CeO2 photocatalysts under simulated sunlight irradiation. Before each reuse experiment, the catalyst used last time will be centrifuged, separated, filtered, dried and sintered at low temperature before the next photocatalytic experiment is performed. After five cycles, the degradation percentage of BaTiO3/CeO2 photocatalyst decreased by only 8%, indicating that the catalyst could be recycled. The slight decrease in degradation percentage can be attributed to the loss of photocatalyst during use. Figure 8B shows the XRD patterns of BaTiO3/CeO2 photocatalysts before and after 5 recycles. Except for the change in the intensity of the diffraction peak, the position of the diffraction peak of BaTiO3/CeO2 photocatalysts did not change, which confirmed that the catalyst was stable and would not decompose into other oxides after repeated use.
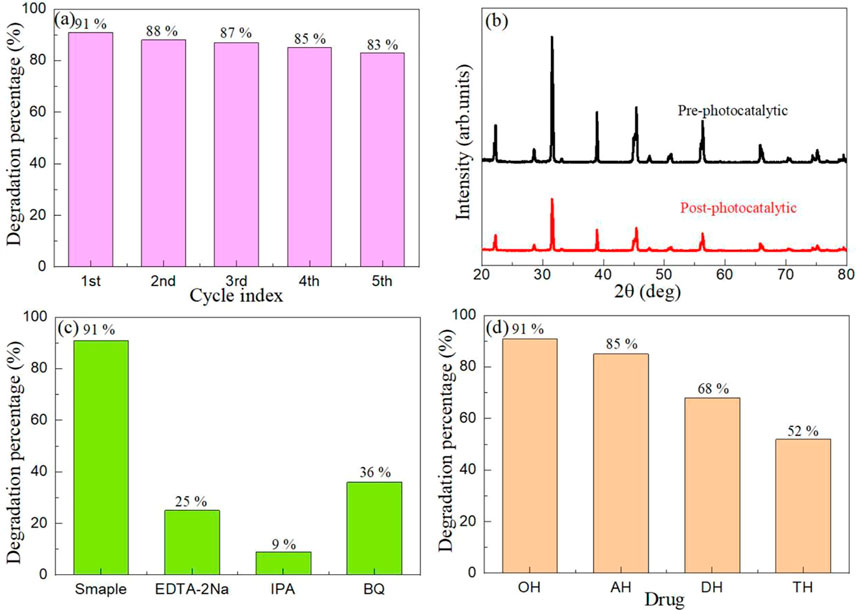
FIGURE 8. (A) The recyclability tests of BaTiO3/CeO2 photocatalysts under simulated sunlight irradiation, and (B) XRD patterns of BaTiO3/CeO2 photocatalysts before and after 5 recycles. (C) Trapping experiment of active species during the photocatalytic degradation of OH over BaTiO3/CeO2 photocatalysts under simulated sunlight irradiation. (D) Degradation percentage for the photodegradation of OH, AH, DH and TH over BaTiO3/CeO2 photocatalysts.
The high photocatalytic activity of BaTiO3/CeO2 photocatalysts may be due to the synergistic action of various free radicals, which makes its photocatalytic activity higher than that of single component BaTiO3 and CeO2. To confirm this conjecture, Figure 8C shows the trapping experiment of active species during the photocatalytic degradation of OH over BaTiO3/CeO2 photocatalysts under simulated sunlight irradiation. The ethylenediaminetetraacetic acid disodium salt (EDTA-2Na), isopropyl alcohol (IPA), and p-benzoquinone (BQ) have been used to detect the holes, hydroxyl radicals, and superoxide radicals, respectively. The only difference between the free radical capture experiment and the photocatalysis experiment is that 1 mmol of the trapping agent needs to be added to the reaction solution before the photocatalysis. As can be seen from the Figure 8C, after the addition of EDTA-2Na, IPA and BQ to the BaTiO3/CeO2 photocatalysts, the photocatalytic activity decreased significantly, indicating that holes, hydroxyl radicals and superoxide radicals all played an important role in the whole photocatalytic process.
In order to explore that the BaTiO3/CeO2 photocatalysts can degrade other drugs of the same type as OH, the drugs including AH, DH and TH were also selected to perform photocatalytic experiments. Figure 8D shows the degradation percentage for the photodegradation of OH, AH, DH and TH over BaTiO3/CeO2 photocatalysts. It can be seen from the figure that all BaTiO3/CeO2 photocatalysts can degrade OH, AH, DH and TH drugs, but the degradation percentage decreases successively. This may be related to the number of -OH groups contained in these drugs, the number of Cl ions, and the location of N-H functional groups at different locations in the carbon ring. From the perspective of molecular structure of different drugs, the preference of BaTiO3/CeO2 photocatalysts for different functional groups led to the obvious difference in the percentage of photocatalytic degradation. On the other hand, the pH of different drug molecules is different. When reacting with photocatalyst, the electrical properties of the surface molecular charge are obviously different, resulting in different percentage of photocatalytic degradation. The results showed that the BaTiO3/CeO2 photocatalysts was selective to the degradation of drugs.
3.5 Photocatalytic mechanism
It can be seen from the photocatalytic experiments, cyclic stability experiments and capture experiments that the BaTiO3/CeO2 photocatalysts has high photocatalytic degradation activity for the degradation of OH under the simulated sunlight irradiation. Based on the capture experiment, it can be seen that hole, hydroxyl radical and superoxide radical play an important role. These important roles need to be further confirmed by theoretical research. The BaTiO3/CeO2 photocatalysts showed high photocatalytic activity in the degradation of OH, and its mechanism could be analyzed in combination with the energy band theory. Based on formulas 4, 5 (He et al., 2021; He et al., 2023a), conduction band potential (ECB) and valence band potential (EVB) of BaTiO3 and CeO2 can be obtained.
Where, the Eg values for the BaTiO3 and CeO2 is 3.06 eV and 3.15 eV, respectively. Ee is 4.5 eV. According to the formulas 6, 7, the X values for the BaTiO3 and CeO2 are 5.13 eV and 5.55 eV, respectively.
Basd on this calculation, the ECB values of BaTiO3 and CeO2 are −0.90 eV and −0.53 eV, respectively, while the EVB values of BaTiO3 and CeO2 are 2.16 eV and 2.62 eV, respectively. According to the results of the above calculation, an energy level diagram can be proposed as shown in Figure 9. It can be seen from the figure that the combination of BaTiO3 and CeO2 forms a type II band arrangement, which is conducive to the transfer and separation of photogenic carriers. However, the band gap values of BaTiO3 and CeO2 of a single component are large, which is difficult to respond to visible light. When the two are combined together, they form defect structures such as vacancies, which make it possible to respond to simulated sunlight and promote the transfer and separation of electron and hole pairs. In the conduction band, since the redox potential of O2/•O2− is −0.13 V, (Arai et al., 2007), the following reactions can occur:
In the valence band, the following chemical reactions can occur due to the redox potentials of H2O/•OH and OH−/•OH are +2.72 V and +1.89 V versus NHE: (Tachikawa et al., 2007; He et al., 2022; He et al., 2023b):
According to the above analysis, the hole, hydroxyl and superoxide radicals promote the degradation of drug over BaTiO3/CeO2 photocatalysts. This way of vacancy construction can promote the application of semiconductor materials with large band gap values in the field of visible light photocatalysis.
4 Conclusion
A simple one-step solution synthesis method was used to construct a type II band aligned BaTiO3/CeO2 photocatalysts. XRD characterization confirms that the obtained BaTiO3/CeO2 photocatalysts contains only cubic phase BaTiO3 and CeO2 diffraction peaks. SEM observation showed that the BaTiO3/CeO2 photocatalysts were spherical particles with an average particle size of about 50 nm. TEM measurements confirmed the formation of special heterojunction between BaTiO3 and CeO2. The optical absorption coefficient of BaTiO3/CeO2 photocatalysts in the visible range is greatly improved due to the presence of oxygen vacancy compared with that of single component BaTiO3 and CeO2. The BaTiO3/CeO2 photocatalysts demonstrated high photocatalytic activity for the degradation of OH drugs due to the synergistic effect of holes, hydroxyl radicals and superoxide radicals.
Data availability statement
The raw data supporting the conclusion of this article will be made available by the authors, without undue reservation.
Author contributions
All authors listed have made a substantial, direct, and intellectual contribution to the work and approved it for publication.
Funding
This work was supported by the Science and Technology Research Project of Hebei Higher Education Institutions (No. QN2020200) and the Hebei Province Natural Science Fund (No. H2022206517).
Conflict of interest
The authors declare that the research was conducted in the absence of any commercial or financial relationships that could be construed as a potential conflict of interest.
Publisher’s note
All claims expressed in this article are solely those of the authors and do not necessarily represent those of their affiliated organizations, or those of the publisher, the editors and the reviewers. Any product that may be evaluated in this article, or claim that may be made by its manufacturer, is not guaranteed or endorsed by the publisher.
References
Arai, T., Yanagida, M., Konishi, Y., Iwasaki, Y., Sugihara, H., and Sayama, K. (2007). Efficient complete oxidation of acetaldehyde into CO2 over CuBi2O4/WO3 composite photocatalyst under visible and UV light irradiation. J. Phys. Chem. C 111 (21), 7574–7577. doi:10.1021/jp0725533
Chavan, P., Naik, L. R., Belavi, P. B., Chavan, G., Ramesha, C. K., and Kotnala, R. K. (2017). Studies on electrical and magnetic properties of Mg-substituted nickel ferrites. J. Electron. Mater. 46, 188–198. doi:10.1007/s11664-016-4886-6
Cheng, Z., Luo, S., Liu, Z., Zhang, Y., Liao, Y., Guo, M., et al. (2022). Visible-light-driven hierarchical porous CeO2 derived from wood for effective photocatalytic degradation of methylene blue. Opt. Mater. 129, 112429. doi:10.1016/j.optmat.2022.112429
Gao, X., Li, B., and Jian, S. (2022). Step-scheme CeO2/CaIn2S4 heterostructured photocatalysts for efficient reduction of Cr (Ⅵ) under visible light. Colloids Surfaces A Physicochem. Eng. Aspects 648, 129168. doi:10.1016/j.colsurfa.2022.129168
Gu, J., Liu, H., Peng, T., Li, S., Xu, L., Zhang, J., et al. (2022). Ag@ CeO2–Au nanorod plasmonic nanohybrids for enhanced photocatalytic conversion of benzyl alcohol to benzaldehyde. ACS Appl. Nano Mater. 5 (4), 4972–4982. doi:10.1021/acsanm.1c04563
Han, M., Wang, S., Chen, X., Liu, H., Gao, H., Zhao, X., et al. (2022). Spinel CuB2O4 (B= Fe, Cr, and Al) oxides for selective adsorption of Congo red and photocatalytic removal of antibiotics. ACS Appl. Nano Mater. 5 (8), 11194–11207. doi:10.1021/acsanm.2c02349
He, Z., Fareed, H., Yang, H., Xia, Y., Su, J., Wang, L., et al. (2023). Mechanistic insight into the charge carrier separation and molecular oxygen activation of manganese doping BiOBr hollow microspheres. J. Colloid Interface Sci. 629, 355–367. doi:10.1016/j.jcis.2022.08.164
He, Z., Siddique, M. S., Yang, H., Xia, Y., Su, J., Tang, B., et al. (2022). Novel Z-scheme In2S3/Bi2WO6 core-shell heterojunctions with synergistic enhanced photocatalytic degradation of tetracycline hydrochloride. J. Clean. Prod. 339, 130634. doi:10.1016/j.jclepro.2022.130634
He, Z., Yang, H., Su, J., Xia, Y., Fu, X., Wang, L., et al. (2021). Construction of multifunctional dual Z-scheme composites with enhanced photocatalytic activities for degradation of ciprofloxacin. Fuel 294, 120399. doi:10.1016/j.fuel.2021.120399
He, Z., Yang, H., Wong, N. H., Sunarso, J., Huang, Z., Xia, Y., et al. (2023). Construction of Cu7S4@ CuCo2O4 yolk-shell microspheres composite and elucidation of its enhanced photocatalytic activity, mechanism, and pathway for carbamazepine degradation. Small 49, 2207370. doi:10.1002/smll.202207370
Hiragond, C. B., Powar, N. S., and Lee, J. S. I. (2022). Single-atom catalysts (SACs) for photocatalytic CO2 reduction with H2O: Activity, product selectivity, stability, and surface chemistry. Small 18 (29), 2201428. doi:10.1002/smll.202201428
Jing, P., He, C., Huang, S., Li, H., Liu, J., Cui, Y., et al. (2022). 2D Z-scheme heterojunction and oxygen deficiency synergistically boosting the photocatalytic activity of a layered BaTiO3/BiOIO3 composite. Appl. Mater. Today 29, 101574. doi:10.1016/j.apmt.2022.101574
Kaur, A., Umar, A., and Kansal, S. K. (2016). Heterogeneous photocatalytic studies of analgesic and non-steroidal anti-inflammatory drugs. Appl. Catal. A General 510, 134–155. doi:10.1016/j.apcata.2015.11.008
Khatkhatay, F., Chen, A., Lee, J. H., Zhang, W., Abdel-Raziq, H., and Wang, H. (2013). Ferroelectric properties of vertically aligned nanostructured BaTiO3–CeO2 thin films and their integration on silicon. ACS Appl. Mater. interfaces 5 (23), 12541–12547. doi:10.1021/am403834k
Li, M., Wang, S., Gao, H., Yin, Z., Chen, C., Yang, H., et al. (2023). Selective removal of antibiotics over MgAl2O4/C3N4/YMnO3 photocatalysts: Performance prediction and mechanism insight. J. Am. Ceram. Soc. 106 (4), 2420–2442. doi:10.1111/jace.18946
Mahamud, M., Taddesse, A. M., Bogale, Y., and Bezu, Z. (2023). Zeolite supported CdS/TiO2/CeO2 composite: Synthesis, characterization and photocatalytic activity for methylene blue dye degradation. Mater. Res. Bull. 161, 112176. doi:10.1016/j.materresbull.2023.112176
Masekela, D., Hintsho-Mbita, N. C., and Mabuba, N. (2023). Application of a piezo-photocatalytic thin film (FTO/BaTiO3/SnO2) for enhanced degradation of organic pollutants and disinfection of wastewater. Ceram. Int. 49 (5), 7566–7579. doi:10.1016/j.ceramint.2022.10.251
Masula, K., Bhongiri, Y., Rao, G. R., Kumar, P. V., Pola, S., and Basude, M. (2022). Evolution of photocatalytic activity of CeO2–Bi2O3 composite material for wastewater degradation under visible-light irradiation. Opt. Mater. 126, 112201. doi:10.1016/j.optmat.2022.112201
Nazarov, P. A. (2022). MDR pumps as crossroads of resistance: Antibiotics and bacteriophages. Antibiotics 11 (6), 734. doi:10.3390/antibiotics11060734
Okeke, E. S., Chukwudozie, K. I., Nyaruaba, R., Ita, R. E., Oladipo, A., Ejeromedoghene, O., et al. (2022). Antibiotic resistance in aquaculture and aquatic organisms: A review of current nanotechnology applications for sustainable management. Environ. Sci. Pollut. Res. 29 (46), 69241–69274. doi:10.1007/s11356-022-22319-y
Passi, M., and Pal, B. (2023). Design of a novel Ag-BaTiO3/GO ternary nanocomposite with enhanced visible-light driven photocatalytic performance towards mitigation of carcinogenic organic pollutants. Sep. Purif. Technol. 308, 122839. doi:10.1016/j.seppur.2022.122839
Prajapati, P. K., Malik, A., Nandal, N., Pandita, S., Singh, R., Bhandari, S., et al. (2022). Morphology controlled Fe and Ni-doped CeO2 nanorods as an excellent heterojunction photocatalyst for CO2 reduction. Appl. Surf. Sci. 588, 152912. doi:10.1016/j.apsusc.2022.152912
Rehman, S., Kim, H., Patil, H., Kadam, K. D., Sagar, R. U. R., Aziz, J., et al. (2021). Current rectification, resistive switching, and stable NDR effect in BaTiO3/CeO2 heterostructure devices. Adv. Electron. Mater. 7 (6), 2001237. doi:10.1002/aelm.202001237
Sahoo, R. K., Manna, A. K., Das, A., Mitra, A., Mohapatra, M., Sarangi, S. N., et al. (2022). Facile synthesis of Super-paramagnetic Au@ α-Fe2O3 hybrid nanoparticle and its assembly on graphene substrate for visible light photo-catalysis. Appl. Surf. Sci. 577, 151954. doi:10.1016/j.apsusc.2021.151954
Tachikawa, T., Fujitsuka, M., and Majima, T. (2007). Mechanistic insight into the TiO2 photocatalytic reactions: Design of new photocatalysts. J. Phys. Chem. C 111 (14), 5259–5275. doi:10.1021/jp069005u
Tarasevich, T. V., and Lebedev, S. A. (2010). Microstructure and dielectric properties of BaTiO3-CeO2-SnO2 ceramics. Inorg. Mater. 46, 79–84. doi:10.1134/s0020168510010188
Tong, B., Shi, L., and Liu, X. (2022). Sol–gel synthesis and photocatalytic activity of graphene oxide/ZnFe2O4-based composite photocatalysts. Front. Mater. 9, 934759. doi:10.3389/fmats.2022.934759
Verma, T., Aggarwal, A., Singh, S., Sharma, S., and Sarma, S. J. (2022). Current challenges and advancements towards discovery and resistance of antibiotics. J. Mol. Struct. 1248, 131380. doi:10.1016/j.molstruc.2021.131380
Wang, S., Chen, X., Fang, L., Gao, H., Han, M., Chen, X., et al. (2022). Double heterojunction CQDs/CeO2/BaFe12O19 magnetic separation photocatalysts: Construction, structural characterization, dye and POPs removal, and the interrelationships between magnetism and photocatalysis. Nucl. Anal. 1 (3), 100026. doi:10.1016/j.nucana.2022.100026
Wang, S., Li, M., Gao, H., Yin, Z., Chen, C., Yang, H., et al. (2023). Construction of CeO2/YMnO3 and CeO2/MgAl2O4/YMnO3 photocatalysts and adsorption of dyes and photocatalytic oxidation of antibiotics: Performance prediction, degradation pathway and mechanism insight. Appl. Surf. Sci. 608, 154977. doi:10.1016/j.apsusc.2022.154977
Xiao, S., Zhang, N., Tao, Y., Song, X., Li, G., Li, H., et al. (2022). Carbon nanotube-threaded mesocrystalline CeO2 for enhanced photocatalytic NO removal. ACS Appl. Nano Mater. 5 (3), 3581–3590. doi:10.1021/acsanm.1c04210
Yan, X., He, H., Liu, G., Zhao, Z., Pei, Y., Liu, P., et al. (2022). A robust memristor based on epitaxial vertically aligned nanostructured BaTiO3− CeO2 films on silicon. Adv. Mater. 34 (23), 2110343. doi:10.1002/adma.202110343
Yan, X., Yan, H., Liu, G., Zhao, J., Zhao, Z., Wang, H., et al. (2022). Silicon-based epitaxial ferroelectric memristor for high temperature operation in self-assembled vertically aligned BaTiO3-CeO2 films. Nano Res. 15 (10), 9654–9662. doi:10.1007/s12274-022-4604-z
Yu, C., Wang, S., Zhang, K., Li, M., Gao, H., Zhang, J., et al. (2023). Visible-light-enhanced photocatalytic activity of BaTiO3/γ-Al2O3 composite photocatalysts for photodegradation of tetracycline hydrochloride. Opt. Mater. 135, 113364. doi:10.1016/j.optmat.2022.113364
Zeng, M. (2011). Surface reaction characteristics at low temperature synthesis BaTiO3 particles by barium hydroxide aqueous solution and titanium tetraisopropoxide. Appl. Surf. Sci. 257 (15), 6636–6643. doi:10.1016/j.apsusc.2011.02.090
Keywords: one-step solution synthesis method, BaTiO3, CeO2, oxytetracycin hydrochloride, wide-band gap
Citation: Ye C and Li C (2023) Construction of BaTiO3/CeO2 heterojunction and comparative study on photocatalytic activity of degradation of different drugs. Front. Mater. 10:1183819. doi: 10.3389/fmats.2023.1183819
Received: 10 March 2023; Accepted: 28 March 2023;
Published: 06 April 2023.
Edited by:
Hua Yang, Lanzhou University of Technology, ChinaReviewed by:
Fei Wang, Chongqing University of Technology, ChinaZuming He, Changzhou University, China
Yuxiang Yan, Nanjing University, China, in collaboration with reviewer ZH
Copyright © 2023 Ye and Li. This is an open-access article distributed under the terms of the Creative Commons Attribution License (CC BY). The use, distribution or reproduction in other forums is permitted, provided the original author(s) and the copyright owner(s) are credited and that the original publication in this journal is cited, in accordance with accepted academic practice. No use, distribution or reproduction is permitted which does not comply with these terms.
*Correspondence: Chao Ye, aGlfY2xvdmVyMTk4NUAxNjMuY29t