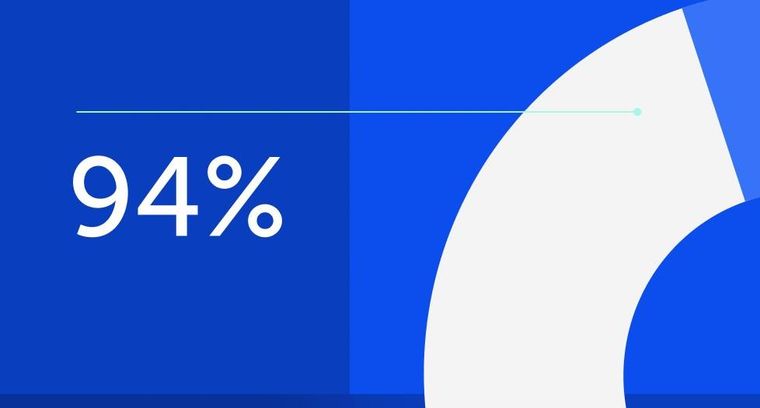
94% of researchers rate our articles as excellent or good
Learn more about the work of our research integrity team to safeguard the quality of each article we publish.
Find out more
ORIGINAL RESEARCH article
Front. Mater., 31 May 2023
Sec. Biomaterials and Bio-Inspired Materials
Volume 10 - 2023 | https://doi.org/10.3389/fmats.2023.1114981
Introduction: Due to the COVID-19 pandemic in recent years, many patients after extubation had stenosis in all tracheal areas because of long-term intubation. Therefore, tracheal stenosis in these patients is benign and can be treated using silicone stents, and many patients need silicone stents during recovery. A silicone stent is an artificial support that plays a significant role in managing airway obstruction.
Methods: This research aims to fabricate an optimal silicone stent reinforced with Nanosilica by vacuumed injection molding. Materials-based nanocomposites are made of rtv2 silicone with 1wt%, 3wt%, and 5wt% of hydrophilic and hydrophobic Nanosilica particles. Hardness, tensile, and hydrophobicity properties have been performed for the experimental characterization of the nanocomposites.
Results and Discussion: The uniform distribution of nanoparticles in the silicone matrix has been confirmed using SEM images. Adding Nano-silica increases hardness and tensile strength and improves the silicone matrix’s mechanical properties. Also, the addition of nanoparticles changes the surface hydrophobicity properties and roughness. Although the presence of nanoparticles improves the mechanical properties, it also reduces the transparency and increases the viscosity. Our results show that adding 1wt%. Hydrophobic Nano-silica improves nanocomposites’ mechanical properties and preserves transparency and viscosity (mold-ability) for stent construction. Adding 3wt%. Hydrophilic Nano-silica improves mechanical and hydrophobic properties, but moldability is not easy. Finally, the fabricated nanocomposite airway stents were successfully placed on the sheep trachea in the pilot animal study.
The Stent is an artificial support that helps to maintain a tubular path (Mehta and Dasgupta, 1999). In recent years, stents have turned from laboratory equipment into components that are vitally used in many medical fields, including cardiac, vascular, urological, and pulmonary (Breen and Dutau, 2009; Wang et al., 2018; Wu et al., 2019; Ion et al., 2021; Xu et al., 2022; Zhu et al., 2022). Obstruction in the central airways may lead to dyspnea and suffocation. Therefore, it needs immediate and appropriate treatment (Oki and Saka, 2013; Xu et al., 2022). One of the urgent treatments for airway obstruction is to use a stent. Today, several commercially available stents are in the research stage (Freitag et al., 1995). The main purpose of stents is to maintain airway patency. Stents usually treat tracheobronchial obstructions, while other interventional treatments are not possible. Stents are also used to treat broncho-esophageal fistula and malignant tracheobronchial obstruction stenosis (Marquette et al., 1995; Sökücü et al., 2020).
An ideal stent should conform to the airway anatomy. Does not move after placement, can be removed; if necessary. It needs to be made of biocompatible materials and does not cause infection. Does not lead to granulation of tissue and mucous impaction. Does not disrupt mucosal fellow and prevent tumor growth. It should be strong enough to withstand the pressure of the trachea and be flexible enough to adapt to the trachea wall. It should not be expensive, and preferably, it should be customized by cutting and sizing (Puma et al., 2000; Saito, 2004; Wang et al., 2013; Guibert et al., 2020).
Stents are made of different materials. One of the oldest types of stents is silicone stents. Montgomery developed a silicone stent in 1965. Its advantages include flexibility, low reactivity with tissue, and non-hardening due to prolonged contact with temperature and secretions (MONTGOMERY, 1965). Silicone stents are flexible and placed with rigid bronchoscopy. Another type is metal stents, which are made of nitinol or stainless steel and may be self-expanding or balloon-expanded. Metal stents are placed by flexible bronchoscopy and stick to the respiratory wall (Bolliger et al., 2002; Ratnovsky et al., 2015; Folch and Keyes, 2018). Covered metallic stent have less hydrophobic properties compared silicon stent and Mucus plugging was found in 17% of the cases, all of them being asymptomatic (Dahlqvist et al., 2016).
In the research conducted between 2013 and 2017, no significant difference was found in the conditions of patients who used silicone and metal stents (Sökücü et al., 2020). Another research shows metal airway stents are unsafe for long-term treatment due to infectious and metal mesh breakage (Puma et al., 2000). In general, using metal airway stents is not a priority for treatment, and the FDA also suggests not using metal stents until other alternative airway stents exist (Folch and Keyes, 2018; Huang et al., 2018).
Silicone stent may be migrated and engage with the mucosa system and may cause accumulation and occlusion. Its diameter is unchangeable, and the external to internal diameter rate is not optimal; It is unsuitable for unusual anatomies and can cause some bronchi to collapse (Puma et al., 2000; Chin et al., 2008). However, silicone stents are all removable and maintain their tolerance and safety in the long term, and they are strong enough to withstand external pressures. In addition, they can be easily customized by cutting and are less expensive than other types (Puma et al., 2000; Folch and Keyes, 2018).
Today, researchers developed biodegradable stents, which are not removed in cases where a temporary stent is needed (Wu et al., 2019); for example, a magnesium airway stent is one type of biodegradable Stent (Xue et al., 2019).
A study by Li et al. (2021) has reported that airway stent with silver nanoparticles/PCL nanocomposites decreases tissue granulation, which is one of the side effects of stenting.
Mencattelli et al. (2021) in their research, provide a patient-specific stent using nitinol and a type of photo-curable polymer. Developing 3D-printed mini-stents and drug-delivery stents are novel ways to produce an airway stent (Sabath and Ost, 2018).
Stenting may lead to complications; for example, about 15% of patients suffer mucus compaction regardless of the type of Stent. Granulation and displacement are other important dilemmas for using stents. Stent displacement is the cause of 20% of stenting failures. Increasing the pressure of expansion of the Stent can reduce the risk of migration but cause tissue granulation (Freitag et al., 1994).
Before stenting, the patient’s condition should be monitored. Prolonged stenting may exacerbate the problems. CT scanning to evaluate tracheal conditions before surgery helps to choose the correct length and diameter of the Stent. The Stent must be sterilized before being placed in the body to prevent infection. Fluoroscopy, Flexible bronchoscopy to clean the accumulated mucus, and Laser to treat tissue granulation can also be used as ancillary treatment. 4–6 weeks after the operation, a CT scan can be used to monitor the patient’s condition (Dumon, 1990; Bolliger et al., 2002; Chin et al., 2008; Breen and Dutau, 2009; Freitag et al., 2017; Liu et al., 2020).
Silicone is the general representative of a family of polymers based on silicon (Selmanowitz and Orentreich, 1977). Silicon is used for a compound in which silicon atoms are bonded to oxygen atoms. Silicon atoms are usually connected with chemical or phenyl inert groups (Moretto et al., 2000).
In general, silicone rubber is divided into three categories: HTV (high-temperature vulcanization), RTV (room-temperature vulcanization), and LSR (liquid-silicone rubber vulcanized at high temperature). RTV silicone rubber is divided into two categories, one component and two-component. Rtv1 is cured when exposed to air humidity, and rtv2 has two components that are cured by mixing them (Kopylov et al., 2011). Metal catalysts such as tin or platinum are used for complete curing and improving properties (Moretto et al., 2000).
Various properties of silicone rubber, such as heat resistance, chemical stability, electrical insulation, wear resistance, transparency, flame resistance, and gas permeability, make it widely used in various industries. Silicones are generally biocompatible and widely used to manufacture catheters, shunts, blood oxygen generators, heart bypass machines, valves, and plastic surgery. Although it is possible to decompose silicone by reacting with chemical catalysts, the hydrophobic nature of silicone leads to its minimal reaction with body fluids. Despite these properties, pure silicone has insufficient mechanical properties for many applications due to the weak interaction and flexibility between the main chains in the silicone matrix (Moretto et al., 2000; Colas and Curtis, 2004; Wu et al., 2016; Ji et al., 2019). Various nanoparticles can be used to reinforce silicone; for example, graphene nanoparticles can improve thermal conductivity (Yang et al., 2018); Carbon black increases electrical conductivity; titanium dioxide improves the dielectric constant, and barium sulfate improves radiopacity (Colas and Curtis, 2004).
Nano silica is an inorganic nanoparticle commonly used to improve the mechanical properties of silicone rubber. Silica is uniformly distributed in the silicone rubber matrix and leads to an increase in tensile strength, tear limit, and hardness (Yan et al., 2015; Wu et al., 2016).
The effect of the addition of Nano-silica to silicone rubber has been investigated in various studies. In other research, surface-treated Nano-silica was added to improve the Silicone Elastomer’s properties. The research shows that adding Nano-silica has improved mechanical properties, including tensile strength and shore hardness (Zayed et al., 2014).
Another research shows that adding 3% by weight of Nano-silica to silicone rubber nanocomposite has improved the hydrophobic properties and corrosion resistance (Ammar et al., 2016).
In another research, adding Nano-silica to silicone rubber has been introduced as an attractive approach to improve the hydrophobic properties of outdoor insulations (Nazir et al., 2016).
The main target of this research is to synthesize silicone stents reinforced with silica nanoparticles. For this purpose, a sheep trachea was chosen to place a target stent. The mold-making process has been selected based on the design variables. Simultaneously with the mold-making process, nanocomposites are synthesized by adding different percentages of nanoparticles to achieve the best percentage for conducting a pilot animal study.
The production of a silicone stent with Nano-silica includes three fundamental steps. The first step includes synthesizing silica–silicone nanocomposite, the second step includes stent designing, and the third step is fabricating an airway stent by vacuum injection molding (Figure 1).
Medical grade RTV2 silicone was exploited to synthesize nanocomposite. This type of silicone usually consists of a polydimethylsiloxane polymer chain with a vinyl end group and platinum catalyst (Freitag et al., 1994). Two components of silicone have been combined with equal ratios.
Two groups of Silica nanoparticles have been chosen as target inclusions to improve silica-silicone nanocomposites’ mechanical and surface properties. Silica particles can be added during or after the silicone polymerization. Silica fillers drastically increase viscosity and affect the crystallization rate of low-curing temperature silicones (Moretto et al., 2000).
For silicone reinforcement, 15–20 nm hydrophilic Nano-silica and 20–35 nm hydrophobic nano-silica have been used (United States NANO Company). The material was prepared with 1wt%, 3wt%, and 5wt% of hydrophilic and hydrophobic Nano-silica. Each of the two silicone components was strengthened separately to prevent an excessive increase in viscosity.
Due to the low volume fraction of Nano-silica, it is difficult to have perfect dispersion of Nano-silica in the silicone matrix. Therefore, a high shear mixer with a speed of 700 rpm ensures perfect dispersion and distribution of the nanoparticle in the matrix. A negative pressure of 0.9 bar is applied to the solution to remove bubbles caused by stirring.
One factor affecting the composite’s quality is nanoparticle dispersion and distribution. SEM analysis was exploited to evaluate nanoparticle dispersions.
Add Nano-silica to silicone leads to an increase in hardness. The hardness of pure silicone is measured and equal to 36 shore A, and the addition of nanoparticles, regardless of hydrophilicity and hydrophobicity, increases hardness.
Before molding, it is necessary to determine the percentage of adding Nano-silica and the suitable hydrophobicity for making stents. Several small and similar samples were prepared to test hydrophobicity, shore A hardness, and nanoparticle distribution. Also, dumbbell shape specimens were prepared for tensile testing according to the ASTM D412-Die C standard (Figure 2).
FIGURE 2. Tensile test specimens (left) and shore A, hydrophobicity and nanoparticle distribution analysis specimens (right).
Tensile test specimens were made and tested according to the ASTM D412-Die C standard by a universal machine designed and manufactured by Khallagh Sanat Atiyeh Peyman Company.
Static, advancing, and receding contact angles and surface free energy parameters have been measured using CAG-20, Jikan Co., and JikanAssistant software Ver. 7.3.2.
The contact angle of diiodomethane has been measured as a nonpolar liquid to calculate the surface free energy. The program uses the advancing contact angles to calculate the surface free energy, and the OWRK algorithm is selected.
Characterization results in this research are comparative, and the data collection process was not statistical.
One of the effective parameters in the performance of a stent is stent radial strength. When force is applied to the distal end of the Stent, the lumen collapses with relatively lower forces. For this reason, it is suggested that the length of the Stent should be at least 5 mm longer than the occlusion unless the end of the Stent is reinforced (Freitag et al., 1995). In this case, the length of the Stent was 50 mm. The stents made in this study will be tested in a pilot study on sheep.
For designing a target stent, the diameter of the four sheep trachea was measured (Figure 3) to determine the approximate diameter of the Stent. For this purpose, every single trachea has been cut into four segments, and a digital caliper has measured the diameter of both ends of each section. The background of the research shows that for proper stability of the Stent in the respiratory tract, the diameter of the Stent should be 10% bigger than the diameter of the trachea (Ratnovsky et al., 2015). For this case, a diameter of 20 mm and a thickness of 2 mm were adopted to maintain strength. These values are taken based on the average values obtained from the measurement in consultation with a veterinarian. Three rows of studs were considered to prevent migration. Gold markers were placed under some of the studs to improve visibility under radiography.
FIGURE 3. Four tracheas of sheep were cut to measure the mean diameter for designing sheep airway stents.
After determining the appropriate Nano-silica in terms of hydrophobicity and its addition percentage, the Stent is molded. Two silicone components were mixed in equal proportions and completely mixed with a high-shear impeller for molding. Then, bubbles were removed from the mixture to prevent air bubbles inside the Stent. Silicone was molded under a vacuum, and after 24 h, the mold was opened, and the Stent was removed.
After analyzing the results of the performed tests, silicone with 1wt%. Hydrophobic and 3% wt. Hydrophilic Nano-silica was selected as the target material. The final Stent was obtained by injection molding (Figure 4).
FIGURE 4. Fabricated stents by vacuum injection molding (Middle row of studs includes gold markers).
The method of making silicone stents in this research is injection molding; therefore, the appropriate viscosity of the material plays a significant role in the molding and the final Stent. The samples with 5wt% hydrophobic and hydrophilic Nano-fillers were discarded due to their high viscosity and difficulty in molding. In addition, the addition of nanoparticles leads to a decrease in the transparency of silicone. The hydrophobicity of the material decreases the risk of interaction between the Stent and tissue. The samples of 1wt% and 3wt% Nano-silica have a close Young’s modulus.
The sample with 1wt% hydrophobic Nano-silica has a lower viscosity, and the molding procedure is simple. The sample with 3wt% hydrophilic Nano-silica has good hydrophobic and mechanical properties, but the molding procedure is difficult. Gold markers were exploited in the stents to improve radiopacity under X-ray imaging.
All samples were characterized using SEM analysis to estimate the quality of dispersion and distribution of the silica nanoparticles.
As an example, two samples of 3wt%. Hydrophilic and 3wt%. Hydrophobic were analyzed by SEM (Figure 5). The result of both samples shows that the nanoparticles are well dispersed in the silicone matrix without agglomerations and confirm the synthesis procedure. Due to the low amount of reinforcement additive to synthesize the raw materials of the stent, the total cost of the stent does not change significantly.
FIGURE 5. Distribution of 3wt% hydrophobic nano silica (left) and 3wt% hydrophilic nano silica (right) in a silicone matrix.
The results of the shore A hardness test, tensile test, and hydrophobicity analysis are given below: Variation of shore A due to the addition of silica nanoparticles is shown in Table 1.
Tensile test specimens were made according to the ASTM D412-Die C standard. Due to the very high viscosity and adhesion of the 5% hydrophilic sample, it was impossible to get bubbles out or mold it. Therefore, this sample was removed from the test process. The tensile test diagram for 37°C and strain rate (1 mm per minute) test conditions are shown in (Figure 6).
Tensile test results show that in samples with the same weight percentage, the reinforcing of hydrophilic specimens was better than that of hydrophobic specimens. According to the stress-strain diagram, it is clear that the addition of nanoparticles leads to a significant improvement in the strength of silicone. Increasing the weight percentage of nanoparticles improves the mechanical properties but also leads to an increase in viscosity.
The contact angle was measured in silicone samples with different percentages of Nano silica. Both water and diiodomethane were used as mediums (Figure 7).
Silicone is hydrophobic, and the water contact angle on the used silicone is 104o. According to Cassie–Baxter and Wenzel’s theories, roughening the surface in the nanoscale increases the contact angle. As such, adding nanoparticles should cause the contact angle to increase, pending they swim to the top and stay on the surface.
Hydrophilic silica nanoparticles have no or small affinity to the RTV polymer and move up to the surface during the polymerization. As such, they create roughness on the surface and, as such, increase the contact angle. For example, by adding 1wt% and 3wt% hydrophilic silica to the polymer, the contact angle increases from 104° to 110° (Figure 7). However, excess hydrophilic silica has the opposite effect and the contact angle declines. The reason is that hydrophilic silica nanoparticles eventually cover a good portion of the polymer surface.
Despite hydrophilic silica nanoparticles, the hydrophobic ones have an affinity to the vinyl ends of the polymer. As such, they remain seated inside the polymer body during polymerization and cannot swim to the top. As such, adding hydrophobic silica nanoparticles has a small effect on the contact angle. For example, adding 1wt% and 3wt% hydrophobic silica nanoparticles has minimally affected the contact angle.
However, increasing the hydrophobic silica nanoparticles increases the contact angle as some particles move to the surface, contributing to the surface roughness (Figure 8).
The surface free energy values and the polar and dispersive components are shown in Table 2. In fact, by increasing the hydrophilic silica nanoparticles, the polar component of the surface free energy increases, which proves the presence of hydrophilic silica nanoparticles on the surface.
A pilot study was conducted to test a newly developed nanocomposite stent in this research. Sufficient radial force is one of the essential features of airway stents. We have used an in-house setup to measure the radial force of the stents, and our results confirmed the applicability of the stent for clinical application. In this study, two bred sheep were selected for the pilot. An industrially equipped livestock farm with sufficient facilities was used to keep the sheep. The sheep received the vaccine per the schedule 3 months before the stenting (Table 3).
For the first time, a new and low-risk stenting procedure was developed to perform the stenting process in sheep; the details of the pilot animal study were published in the M.D. dissertation performed in our research group (Frahani, 2022). The sheep underwent stenting operations under the ethical guidelines for research in animal science. An anesthesiologist and an experienced veterinarian were present during the operation. Furthermore, surgery was performed in the operating room, equipped with all facilities, including an anesthesia machine, cold light, bronchoscope, Etc. (Figure 8).
Hydrophobic silica nanoparticles improve the resistance and mechanical properties of Stent. The hydrophobic properties of the stent surface can decrease plaque mucus in the tubular Stent. The pilot sheep were stented with a new method of stenting (Maryam Mazraehei Farahani et al., 2022). Two sheep were examined using 3D-virtual CT, bronchoscopy and multiple biopsies from the erosion (Maryam Mazraehei Farahani et al., 2022). After stenting both sheep, a bronchoscopy was performed to confirm the position and condition of the stents (Figure 9).
Nowadays, airway stents are widely used to treat respiratory tract diseases. Despite the complications like stent migration and mucus formation, silicone stents are a safe and cost-effective choice for treating many airway diseases. Due to the extensive use of silicone stents in treating tracheal diseases and the advantages of this type of stents, the fabrication of silicone stent whit Nano-silica was the subject of this study. To evaluate the improvement of mechanical properties comparatively, amounts of 1wt%, 3wt%, and 5wt%. Hydrophobic and hydrophilic Nano-silica were added to pure silicone.
This research shows that increasing the volume fraction of silica nanoparticles increases the elastic modulus and hardness. The increase in properties in the hydrophobic type is more than in the hydrophilic type due to better distribution and dispersion of Nano filler in the silicone matrix. For low volume fraction (less than 3wt%), Hydrophilic Nano-silica particles move to the surface and consequently increase the roughness and hydrophobicity of samples. Increasing the volume fraction to 5wt% decreases hydrophobicity due to the surface agglomeration of nanoparticles. For low-volume fractions (less than 3wt%), hydrophobic Nano-silica particles disperse in the matrix and do not affect the hydrophobicity properties. Due to the surface agglomeration of nanoparticles, Increasing the volume fraction to 5wt% increases hydrophobicity. Various characterization techniques such as hardness, tensile strength, hydrophobicity, and SEM were exploited to characterize the based materials for the Stent.
Gold markers are placed in one row of studs to improve radiopacity. A pilot animal test was performed on two sheep for 2 months To study in vivo performance quality of the fabricated stents (Maryam Mazraehei Farahani et al., 2022).
Finally, the newly developed stents have better hydrophobic properties than pure silicone ones. Hydrophobic properties improve the quality of the Stent due to the decrease of bacteria and mucus deposition.
The original contributions presented in the study are included in the article/Supplementary Material, further inquiries can be directed to the corresponding author.
The animal study was reviewed and approved by Research Ethics Committees of Imam Khomeini Hospital Complex- Tehran University of Medical Sciences, Tehran, Iran.
ZM: methodology, conceptualization, investigation, writing original draft, visualization, resources, implementation. MF: methodology, conceptualization, investigation, writing original draft, visualization, resources, pilot study. MaB: methodology, validation, writing—review and editing, resources, supervision. FC: conceptualization, review and editing, validation, methodology. A-MK: methodology, implementation, validation, writing—review and editing, pilot study. AK: intervention, pilot study, implementation, methodology MoB: methodology, validation, writing—review and editing, resources, supervision, project administration. All authors contributed to the article and approved the submitted version.
The authors declare that the research was conducted in the absence of any commercial or financial relationships that could be construed as a potential conflict of interest.
All claims expressed in this article are solely those of the authors and do not necessarily represent those of their affiliated organizations, or those of the publisher, the editors and the reviewers. Any product that may be evaluated in this article, or claim that may be made by its manufacturer, is not guaranteed or endorsed by the publisher.
Ammar, S., Ramesh, K., Vengadaesvaran, B., Ramesh, S., and Arof, A. K. (2016). A novel coating material that uses nano-sized SiO2 particles to intensify hydrophobicity and corrosion protection properties. Electrochimica Acta 220, 417–426. doi:10.1016/j.electacta.2016.10.099
Bolliger, C. T., Mathur, P. N., Beamis, J. F., Becker, H. D., Cavaliere, S., Colt, H., et al. (2002). ERS/ATS statement on interventional pulmonology: Chairmen: C.T. Bolliger, P.N. Mathur. Eur. Respir. J. 19, 356–373. doi:10.1183/09031936.02.00204602
Breen, D. P., and Dutau, H. (2009). On-site customization of silicone stents: Towards optimal palliation of complex airway conditions. Respiration 77, 447–453. doi:10.1159/000205396
Chin, C. S., Litle, V., Yun, J., Weiser, T., and Swanson, S. J. (2008). Airway stents. Ann. Thorac. Surg. 85, S792–S796. doi:10.1016/j.athoracsur.2007.11.051
Colas, A., and Curtis, J. (2004). Silicone biomaterials: History and chemistry. Biomaterials Sci. Introd. Mater. Med. 2, 80–85.
Dahlqvist, C., Ocak, S., Gourdin, M., Dincq, A. S., Putz, L., and d'Odémont, J. P. (2016). Fully covered metallic stents for the treatment of benign airway stenosis. Can. Respir. J. 2016, 1–7. doi:10.1155/2016/8085216
Dumon, J. F. (1990). A dedicated tracheobronchial stent. Chest 97, 328–332. doi:10.1378/chest.97.2.328
Folch, E., and Keyes, C. (2018). Airway stents. Ann. Cardiothorac. Surg. 7, 273–283. doi:10.21037/acs.2018.03.08
Frahani, M. M. (2022). Implementation of the pilot animal study for air way silicone stent. Tehran: Tehran university of Medical Science.
Freitag, L., Eicker, K., Donovan, T. J., and Dimov, D. (1995). Mechanical properties of airway stents. J. Bronchology and Interventional Pulmonol. 2, 270–278. doi:10.1097/00128594-199510000-00003
Freitag, L., Eicker, R., Linz, B., and Greschuchna, D. (1994). Theoretical and experimental basis for the development of a dynamic airway stent. Eur. Respir. J. 7, 2038–2045. doi:10.1183/09031936.94.07112038
Freitag, L., Gordes, M., Zarogoulidis, P., Darwiche, K., Franzen, D., Funke, F., et al. (2017). Towards individualized tracheobronchial stents: Technical, practical and legal considerations. Respiration 94, 442–456. doi:10.1159/000479164
Guibert, N., Saka, H., and Dutau, H. (2020). Airway stenting: Technological advancements and its role in interventional pulmonology. Respirology 25, 953–962. doi:10.1111/resp.13801
Huang, S., Xu, J., An, Z., Yuan, P., Xu, H., Lv, W., et al. (2018). Clinical assessment of airway stent placement in patients with malignant airway lesions. J. Thorac. Dis. 10, 3277–3288. doi:10.21037/jtd.2018.06.01
Ion, R., Cabon, G., Gordin, D. M., Ionica, E., Gloriant, T., and Cimpean, A. (2021). Endothelial cell responses to a highly deformable titanium alloy designed for vascular stent applications. J. Funct. biomaterials 12, 33. doi:10.3390/jfb12020033
Ji, J., Ge, X., Pang, X., Liu, R., Wen, S., Sun, J., et al. (2019). Synthesis and characterization of room temperature vulcanized silicone rubber using methoxyl-capped MQ silicone resin as self-reinforced cross-linker. Polym. (Basel) 11, 1142. doi:10.3390/polym11071142
Kopylov, V., Kostyleva, E., Kostylev, I., and Koviazin, A. (2011). Silica fillers for silicone rubber. Int. Polym. Sci. Technol. 38, 35–47. doi:10.1177/0307174x1103800408
Li, Z., Jiao, D., Zhang, W., Ren, K., Qiu, L., Tian, C., et al. (2021). Antibacterial and antihyperplasia polylactic acid/silver nanoparticles nanofiber membrane-coated airway stent for tracheal stenosis. Colloids Surf. B Biointerfaces 206, 111949. doi:10.1016/j.colsurfb.2021.111949
Liu, L., Kong, J., and Georg, C. (2020). Recent advances in airway stenting. Shanghai Chest 4, 6. doi:10.21037/shc.2019.11.02
Marquette, C. H., Mensier, E., Copin, M. C., Desmidt, A., Freitag, L., Witt, C., et al. (1995). Experimental models of tracheobronchial stenoses: A useful tool for evaluating airway stents. Ann. Thorac. Surg. 60, 651–656. doi:10.1016/0003-4975(95)00460-3
Maryam Mazraehei Farahani, A. M. K., Kiani, A., Kazemizadeh, H., and Baghani, M. (2022). Developing an innovative low-risk interventional approach for stenting trachea. Interactive CardioVascular and Thoracic Surgery.
Mehta, A. C., and Dasgupta, A. (1999). Airway stents. Clin. chest Med. 20, 139–151. doi:10.1016/s0272-5231(05)70132-5
Mencattelli, M., Mondal, A., Miale, R., Van Story, D., Peine, J., Li, Y., et al. (2021). Vivo molding of airway stents. Adv. Funct. Mater 31, 2010525. doi:10.1002/adfm.202010525
Montgomery, W. W. (1965). T-tube tracheal stent. Archives otolaryngology 82, 320–321. doi:10.1001/archotol.1965.00760010322023
Moretto, H. H., Schulze, M., and Wagner, G. (2000). Silicones. Ullmann's Encyclopedia of Industrial Chemistry. Wiley. doi:10.1002/14356007.a24_057
Nazir, M. T., Phung, B. T., and Hoffman, M. (2016). Performance of silicone rubber composites with SiO2 micro/nano-filler under AC corona discharge. IEEE Trans. Dielectr. Electr. Insulation 23, 2804–2815. doi:10.1109/tdei.2016.7736840
Oki, M., and Saka, H. (2013). New dedicated bifurcated silicone stent placement for stenosis around the primary right carina. Chest 144, 450–455. doi:10.1378/chest.12-2834
Puma, F., Farabi, R., Urbani, M., Santoprete, S., Daddi, N., Di Meo, A., et al. (2000). Long-term safety and tolerance of silicone and self-expandable airway stents: An experimental study. Ann. Thorac. Surg. 69, 1030–1034. doi:10.1016/s0003-4975(00)01092-4
Ratnovsky, A., Regev, N., Wald, S., Kramer, M., and Naftali, S. (2015). Mechanical properties of different airway stents. Med. Eng. Phys. 37, 408–415. doi:10.1016/j.medengphy.2015.02.008
Sabath, B. F., and Ost, D. E. (2018). Update on airway stents. Curr. Opin. Pulm. Med. 24, 343–349. doi:10.1097/mcp.0000000000000486
Saito, Y. (2004). “Endobronchial stents: Past, present, and future,” in Seminars in respiratory and critical care medicine: Copyright© (Seventh Avenue, New: Thieme Medical Publishers, Inc), 375–380.
Selmanowitz, V. J., and Orentreich, N. (1977). Medical-grade fluid silicone: A monographic review. Dermatol. Surg. 3, 597–611. doi:10.1111/j.1524-4725.1977.tb00366.x
Sökücü, S. N., Özdemir, C., Tural Önür, S., Dalar, L., and Altın, S. (2020). Comparison of silicon and metallic bifurcated stents in patients with malignant airway lesions. Clin. Respir. J. 14, 198–204. doi:10.1111/crj.13114
Wang, J., Boutin, K. G., Abdulhadi, O., Personnat, L. D., Shazly, T., Langer, R., et al. (2013). Fully biodegradable airway stents using amino alcohol-based poly(ester amide) elastomers. Adv. Healthc. Mater 2, 1329–1336. doi:10.1002/adhm.201200348
Wang, J., Song, C., Xiao, Y., and Liu, B. (2018). In vivo and in vitro analyses of the effects of a novel high-nitrogen low-nickel coronary stent on reducing in-stent restenosis. J. Biomaterials Appl. 33, 64–71. doi:10.1177/0885328218773306
Wu, J., Lee, B., Saha, P., and N Kumta, P. (2019). A feasibility study of biodegradable magnesium-aluminum-zinc-calcium-manganese (AZXM) alloys for tracheal stent application. J. Biomaterials Appl. 33, 1080–1093. doi:10.1177/0885328218824775
Wu, L., Wang, X., Ning, L., Han, J., Wan, Z., and Lu, M. (2016). Improvement of silicone rubber properties by addition of nano-SiO2 particles. J. Appl. Biomater. Funct. Mater 14 (1), e11–e14. doi:10.5301/jabfm.5000298
Xu, C., Ma, Y., Huang, H., Ruan, Z., and Li, Y. (2022). A review of woven tracheal stents: Materials, structures, and application. J. Funct. biomaterials 13, 96. doi:10.3390/jfb13030096
Xue, B., Liang, B., Yuan, G., Zhu, L., Wang, H., Lu, Z., et al. (2019). A pilot study of a novel biodegradable magnesium alloy airway stent in a rabbit model. Int. J. Pediatr. Otorhinolaryngol. 117, 88–95. doi:10.1016/j.ijporl.2018.10.047
Yan, F., Zhang, X., Liu, F., Li, X., and Zhang, Z. (2015). Adjusting the properties of silicone rubber filled with nanosilica by changing the surface organic groups of nanosilica. Compos. Part B Eng. 75, 47–52. doi:10.1016/j.compositesb.2015.01.030
Yang, B., Zhang, S. H., Zou, Y. F., Ma, W. S., Huang, G. J., and Li, M. D. (2018). Improving the thermal conductivity and mechanical properties of two-component room temperature vulcanized silicone rubber by filling with hydrophobically modified SiO2-graphene nanohybrids. Chin. J. Polym. Sci. 37, 189–196. doi:10.1007/s10118-019-2185-4
Zayed, S. M., Alshimy, A. M., and Fahmy, A. E. (2014). Effect of surface treated silicon dioxide nanoparticles on some mechanical properties of maxillofacial silicone elastomer. Int. J. Biomaterials 2014, 1–7. doi:10.1155/2014/750398
Keywords: silicone-silica nanocomposites, airway stent, hydrophobicity, sheep trachea, stent migration
Citation: Morad Hasely Z, Farahani MM, Baniassadi M, Chini F, Kajbafzadeh A-M, Kiani A and Baghani M (2023) Design and fabrication of silicone-silica nanocomposites airway stent. Front. Mater. 10:1114981. doi: 10.3389/fmats.2023.1114981
Received: 03 December 2022; Accepted: 15 May 2023;
Published: 31 May 2023.
Edited by:
Hannes C. Schniepp, College of William & Mary, United StatesReviewed by:
Amal Bouich, Universitat Politècnica de València, SpainCopyright © 2023 Morad Hasely, Farahani, Baniassadi, Chini, Kajbafzadeh, Kiani and Baghani. This is an open-access article distributed under the terms of the Creative Commons Attribution License (CC BY). The use, distribution or reproduction in other forums is permitted, provided the original author(s) and the copyright owner(s) are credited and that the original publication in this journal is cited, in accordance with accepted academic practice. No use, distribution or reproduction is permitted which does not comply with these terms.
*Correspondence: Moastafa Baghani, baghani@ut.ac.ir
Disclaimer: All claims expressed in this article are solely those of the authors and do not necessarily represent those of their affiliated organizations, or those of the publisher, the editors and the reviewers. Any product that may be evaluated in this article or claim that may be made by its manufacturer is not guaranteed or endorsed by the publisher.
Research integrity at Frontiers
Learn more about the work of our research integrity team to safeguard the quality of each article we publish.