- 1CNOOC Research Institute Co., Ltd., Beijing, China
- 2Exploration Business Department of PetroChina Southwest Oil and Gas Field Company, Chengdu, China
- 3School of New Energy and Materials, Southwest Petroleum University, Chengdu, China
Active alumina (Al2O3) is one of the carrier materials used for the purification of automobile exhaust. The crystal transformation of active Al2O3 is due to the high temperature of the automobile exhaust port, which greatly reduces the specific surface area and affects the catalytic efficiency. To improve the high-temperature stability of active Al2O3, this study synthesized Al2O3 via the gel-sol method. The influence of rare Earth and alkaline Earth metal doping modification on the high-temperature stability of activated Al2O3 was investigated. The specific surface areas were analyzed using Brunauer–Emmett–Teller, X-ray diffraction, Fourier-transform infrared spectroscopy, Scanning electron microscopy, and transmission electron microscopy to explore the effects and mechanism of different contents of La, Ce, and Ba on active Al2O3. The results showed that suitable single doping of La, Ce, and Ba inhibited the phase transition of Al2O3 and improved the high-temperature stability of active Al2O3. When 1% of La and Ba were mixed and doped into active Al2O3, the specific surface area of the sample after aging at 1,100°C for 4 h was 119.2512 m2/g. X-ray diffraction analysis revealed the agglomeration of Ba atoms inside the alumina and that the incorporated La atoms accelerated the agglomeration and promoted the formation of BaAl2O4.
1 Introduction
China is the world’s largest producer and consumer of motor vehicles (Ministry of Environmental Protection of the People’s Republic of China, 2020). In 2019, the total emission of four pollutants from motor vehicles in China was initially calculated to be 16.038 million tons. These four pollutants are the main source of air pollution in the large and medium-sized cities of China. Engine external purification technology is one of the effective strategies for treating automobile exhaust, which minimizes the emission of pollutants (Tu and Ming, 2013; Deng and Zhou, 2020). The off-machine purification technology converts the harmful substances in automobile exhaust into other harmless or less harmful substances through catalysis (Wang et al., 2017). The efficiency of the catalysis can be improved by dispersing a catalyst on the surface of activated alumina (Azizi et al., 2016; Feng et al., 2019; Yadav et al., 2021). The large specific surface area of activated alumina provides sufficient attachment sites for the catalyst to maintain good dispersion, thereby improving catalytic efficiency (Lin et al., 2021). However, the exhaust port temperature of automobile engine is usually up to 800°C–1,000°C. When the temperature exceeds 1,000°C, the crystal transformation of active Al2O3 will occur, resulting in a substantial reduction of specific surface area, which seriously affects the catalytic efficiency (Xu et al., 2022). Therefore, the improvement of the high-temperature stability of the active Al2O3 is urgent and crucial.
Studies have shown that the activated alumina synthesized via the sol-gel method has a controllable grain size, uniform internal pores, and better performance. The synthesis method is simple and safe (Hosseini et al., 2011; Aghamohammadi et al., 2017). However, the pure activated alumina prepared via the sol-gel method has poor high-temperature stability; thus, it is necessary to improve its high-temperature stability. The addition of a high-temperature stabilizer to alumina can improve its high-temperature stability. A study has shown that the high specific surface area of activated alumina can be maintained by doping La to inhibit the crystal transformation of Al2O3 at 1,200°C (Wei et al., 2005). In addition, studies have revealed that a strong two-phase symbiosis can be formed between CeO2 and Al2O3 in the process of synthesizing CeO2-Al2O3, thus making CeO2 and Al2O3 to be evenly distributed in the symbiotic process, hindering the agglomeration and crystal transformation of oxides, and improving the stability of γ-Al2O3 under high-temperature conditions (Li et al., 2010). The above studies showed that the doping of rare Earth and alkaline Earth metal could improve the high-temperature stability of active Al2O3. The active Al2O3 carriers doped with alkaline Earth and rare Earth metals (La, Ce, and Ba) were synthesized via a sol-gel method to explore the influence of various element dopings on the active Al2O3 prepared via a sol-gel method. The influence of rare Earth and alkaline Earth metal doping on the high-temperature stability of active Al2O3 and its mechanism was studied using Brunauer–Emmett–Teller (BET), X-ray diffraction, Fourier-transform infrared spectroscopy, Scanning electron microscopy, and transmission electron microscopy.
2 Materials and methods
2.1 Materials
The materials used in the experiment were as follows: aluminum nitrate (AR), ethanol (AR), propylene oxide (AR), ammonia (concentration of 15%–28%), lanthanum nitrate (AR), cerium nitrate (AR) and barium hydroxide (AR). All chemicals were purchased from the Chengdu KeLong Chemical Reagent Plant. The deionized water used in this study was produced in the laboratory. The formula for synthesizing activated alumina is shown in Table 1. The dosages of lanthanum nitrate, cerium nitrate, and barium hydroxide used in the experiment are presented in Tables 2–Tables 4, respectively.
2.2 Preparation of modified Al2O3
Aluminum nitrate, lanthanum nitrate, cerium nitrate, and barium hydroxide were added to the mixed solution of ethanol and water. For the mixed doping sample, the two-phase mixture was prepared according to the two-phase ratio of 1:1 and the total addition was 1%, and the three-phase mixture sample was doped according to the three-phase ratio of 1:1:1 and the total addition was 1%. The mixed solution was continuously stirred until all particles were completely dissolved in the solution. Propylene oxide was slowly added to the beaker containing the mixed solution. Then, the mixed solution was stirred at high speed for 5–6 min to ensure propylene oxide was evenly distributed in the solution. Afterward, ammonia water was slowly dropped into the beaker and stirred for 10–11 min until the solution became colorless and transparent. Then the mixed solution in the beaker was allowed to stand at room temperature, aging for 24 h, to form a wet gel. The obtained wet gel was placed into an oven and dried at 60°C for 24 h. Afterward, the dried gel was transferred into a resistance furnace and calcined at 550°C for 4 h at a heating rate of 5°C/min. Then, the calcined product was cooled to obtain modified Al2O3. Then, the heat treatment aging test was performed at 1,100°C for 4 h, and the changes in specific surface area and phase were investigated.
2.3 Characterization of samples
The X-ray diffractometer (XRD, DX-2700B) was used to test the diffraction pattern of the synthesized activated alumina. The scanning step was 0.04°, and the scanning angle 2θ ranged from 5° to 80°.
The specific surface area of the synthesized activated alumina was measured by a fully automatic physical adsorption instrument (BET, Micromeritics ASAP2460). Before the measurement, the sample was dried at 300°C for 4 h to remove the water and air in the sample, and then the nitrogen adsorption experiment was performed at 77 K. BET was used to calculate the specific surface area.
The molecular structure and chemical bonds of the synthesized activated alumina were analyzed using a Fourier transform infrared spectrometer (FT-IR, NICOLET 6700). The sample was mixed with potassium bromide at a mass ratio of 1:100 and ground evenly in an agate mortar. The samples obtained after mixing were pressed into thin sheets and tested in the range of 4,000–400 cm−1.
The microstructure and pore distribution of the synthesized alumina were examined using a scanning electron microscope (SEM, ZEISS EV0 MA15) and transmission electron microscope (TEM, ZEISS Libra 200 FE). A small amount of ground sample was added to anhydrous ethanol and ultrasonically dispersed for 30 min. Then one drop of ethanol mixed solution was gently dropped onto a clean glass sheet using a dropper. After the ethanol was volatilized, the sample on the glass plate was collected with conductive adhesive and sprayed with gold. Afterward, the sprayed sample was examined using SEM. In addition, one drop of ethanol was added to the mixture onto the copper gate, and the sample was examined using TEM after ethanol volatilization.
3 Results and discussion
3.1 Specific surface area analysis
Considering the special application performance as catalyst support, the specific surface area of Al2O3 prepared under different conditions was tested. Figure 1 shows the specific surface area of pure activated alumina and samples doped with La, Ce, and Ba calcined at 550°C and aged at 1,100°C for 4 h. The specific surface areas of pure synthesized Al2O3 obtained after its calcination and high-temperature aging at 550°C and 1,100°C were 298.5764 and 21.3124 m2/g, respectively, indicating that the specific surface area of Al2O3 after high-temperature aging was significantly reduced. The specific surface area of all modified samples was smaller than that of pure Al2O3 after calcination at 550°C. When the high-temperature stabilizers La, Ce, and Ba were added to the sample, the specific surface area of the sample showed a downward trend with increasing content. After the sample with 1% of La, Ce, and Ba contents was aged at a high temperature of 1,100°C, the specific surface area became the largest. When the contents of La, Ce, and Ba were more than 1%, the specific surface area of the sample decreased in a “cliff-like” manner, and the specific surface area of the sample doped with La significantly decreased. It is worth noting that when the specific surface area of Ce-2 and Ba-8 changes significantly, this is because the addition of Ce-2 and Ba-8 has a significant effect on the size of primary Al2O3 crystallization particles, thus affecting the pore structure. Overall, the modification effect of Ba was the best, and the specific surface area of all the samples was higher than that of pure Al2O3 after they were aged at a high temperature of 1,100°C.The specific surface area of “La + Ba” mixed sample after high-temperature aging at 1,100°C for 4 h was the largest (119.2512 m2/g). The high-temperature stability of other mixed-doped samples has not been further improved. The specific surface area of the other groups after high-temperature aging at 1,100°C was lower than that of the samples when each component was doped separately, indicating that there was an interaction between the doped elements.
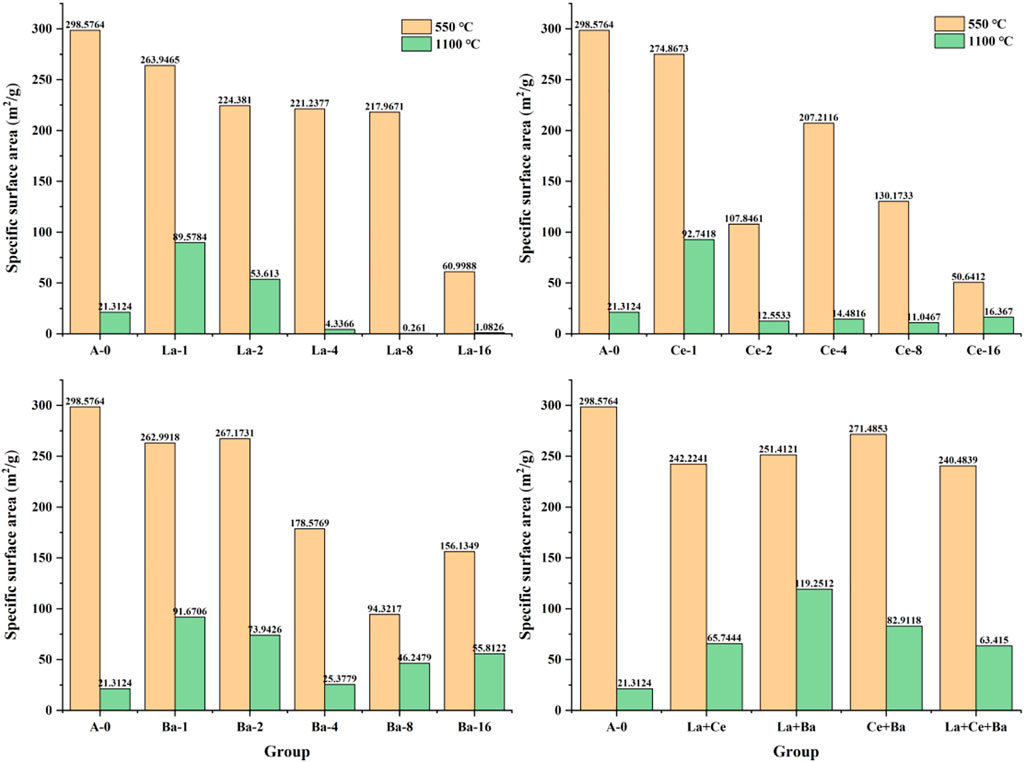
FIGURE 1. Specific surface area of pure activated alumina, single doping modified alumina and mixed doping-modified alumina.
3.2 Phase analysis
Figure 2 shows the XRD pattern of pure Al2O3 calcined at 550°C (Figure 2A) and aged at 1,100°C for 4 h (Figure 2B). After the pure Al2O3 was calcined at 550°C, only the diffraction characteristic peak of γ-Al2O3 (PDF # 10-0425) was observed in the spectrum. After the sample was aged at a high temperature of 1,100°C, only the diffraction characteristic peak of α-Al2O3 (PDF # 82-1467) was observed in the spectrum, indicating that γ-Al2O3 transformed into α-Al2O3 after high-temperature treatment at 1,100°C, thus decreasing the specific surface area of pure Al2O3.
Figure 3 shows the XRD pattern of La-doped samples calcined at 550°C (Figure 3A) and aged at 1,100°C for 4 h (Figure 3B). As shown in Figure 3A, only diffraction characteristic peaks of γ-Al2O3 (PDF#10-0425) and La2O3 (PDF#74-2430) were observed in the spectrum after the calcination of the samples at 550°C. No significant decrease was observed in the diffraction peaks of La2O3 as La content increased. This phenomenon can be attributed to low calcination temperature and crystallization degree of La2O3. As shown in Figure 3B, γ-Al2O3 (PDF#10-0425) (Chen et al., 2018), La2O3 (PDF#74-2430), and LaAlO3 (PDF#74-2430) were observed in the sample after it was aged at 1,100°C. The diffraction characteristic peak at 1,100°C was sharper, indicating that high temperature could promote the formation and growth of crystals.
When the content of La was less than 4%, the intensity of the characteristic peak of γ-Al2O3 gradually weakened, and the diffraction characteristic peak corresponding to the (311) crystal plane shifted to the left because the introduction of La element led to the distortion of the Al2O3 lattice (Gong et al., 2001). With the increased La content, La reacted with activated alumina to form LaAlO3 (Jing et al., 2020); thus, the corresponding characteristic peak intensity gradually increased. When the content of La was more than 4%, the characteristic peak of LaAlO3 gradually increased, and the BET test results showed that the corresponding specific surface area was less than 2 m2/g, which was due to the thermally stable product of La atoms covering the surface of Al2O3 (BETTMAN et al., 1989).
Figure 4 shows the XRD patterns of Ce-doped samples after calcination at 550°C (Figure 4A) and aging at 1,100°C for 4 h (b). After the calcination of Ce-doped samples at 550°C, the characteristic peaks of γ-Al2O3 (PDF#10-0425) and CeO2 (PDF#78-0694) are presented in Figure 4A. The result showed that the diffraction characteristic peak of CeO2 was significantly enhanced with increasing Ce content. When the Ce content was 16%, a clear diffraction characteristic peak appeared, indicating that the crystallization degree of CeO2 in alumina increased; Figure 4B shows the characteristic peak of α-Al2O3 (PDF82-1467) after aging the samples at 1,100°C.
The addition of Ce inhibited the transformation of γ-Al2O3 to α-Al2O3. The crystallization degree of CeO2 was related to the content of CeO2. The crystallization degree of CeO2 increased when the content of CeO2 was below 8%. By comparing the samples with Ce content of 1, 2, and 4%, the diffraction characteristic peaks corresponding to (511) of γ-Al2O3 containing Ce content of 2% and 4% increased significantly, indicating that Ce element had the effect of inducing Al2O3 lattice distortion. Compared with the spectrum at 550°C, the crystallinity of CeO2 crystal was higher with the increase in temperature under the same dosage condition, indicating that high temperature contributed to the formation of CeO2. The above results show that Ce atoms mainly exist in the form of CeO2, and the presence of Al2O3 does not interfere with the formation of CeO2 (Lu et al., 2003; Pang et al., 2020).
Figure 5 shows the XRD pattern of Ba-doped samples aged at 550°C (Figure 5A) and 1,100°C (Figure 5B) for 4 h. After calcination of Ba-doped samples at 550°C, the characteristic peaks of γ-Al2O3 (PDF#10-0425), BaO (PDF#74-1228), and BaCO3 (PDF#71-2394) are shown in Figure 5A. After the aging of Ba-doped samples at 1,100°C, the characteristic peaks of γ-Al2O3 (PDF#10-0425), BaO (PDF#74-1228), and BaAl2O4 (PDF#72-0387) are shown in Figure 5B. The result showed BaCO3 decomposed after it was aged at 1,100°C. When the Ba content was less than 4%, the characteristic peak intensity of γ-Al2O3 gradually decreased with the increasing Ba content. A weak characteristic peak of BaAl2O4 was observed at 4% addition of Ba, which indicated that Ba2+ existed in the lattice gap of alumina after the decomposition of BaCO3 (Chen et al., 2021), resulting in the distortion of alumina lattice and the interaction with alumina to form BaAl2O4 (Jing et al., 2021). At the same time, compared with the XRD patterns of samples with 1% and 4% Ba content, the characteristic peaks corresponding to γ-Al2O3 (311) and (222) rapidly disappeared. When the Ba content was 4%, the corresponding characteristic peaks disappeared, but (440) and (400) still exhibited sharp diffraction characteristic peaks, which further indicated that the introduction of Ba atoms led to the distortion of alumina crystals. When the Ba content was more than 4%, the characteristic peak of BaAl2O4 appeared in the sample, and the characteristic peak of α-Al2O3 (PDF#82-1467) was not observed. The BaAl2O4 and α-Al2O3 were both hexagonal close-packed structures. Only the characteristic peak of BaAl2O4 appeared after the sample was calcined at a high temperature, indicating that the crystal structure of the former was more stable. The addition of Ba inhibited the transformation of γ-Al2O3 to α-Al2O3. The specific surface area of the sample with 16% of Ba content was much higher than that of La and Ce samples with the same content owing to a large specific surface area of BaAl2O4 (Yang et al., 2015; Yadav and Sharma, 2019).
Figure 6 shows the XRD pattern of the samples calcined at 550°C (Figure 6A) and aged at 1,100°C for 4 h (Figure 6B), with the sample containing 1% content of La, Ce, and Ba. The characteristic peaks of γ-Al2O3 (PDF#10-0425), La2O3 (PDF#74-2430), and CeO2 (PDF#78-0694) after calcination at 550°C are shown in Figure 6A. Most of the aluminas retained their amorphous structures after calcination. After the samples were aged at 1,100°C, the characteristic peaks of α-Al2O3 (PDF#82-1467), γ-Al2O3 (PDF#10-0425), LaAlO3 (PDF#85-0848), CeO2 (PDF#78-0694), and BaAl2O4 (PDF#72-0387) are shown in Figure 6B. After La and Ce were co-doped, the characteristic peak of LaAlO3 containing 1% content of La was observed, and the characteristic peak of CeO2 was significantly enhanced, indicating the existence of an interaction between La and Ce (Tang et al., 2018), which could promote the formation of LaAlO3 and CeO2. After the incorporation of Ba and Ce, α-Al2O3 diffraction peaks appeared, indicating that the inhibition of Ba and Ce on the phase transition of Al2O3 is interfered with each other, which may be related to the electron arrangement of the them. After La and Ba were doped, the diffraction characteristic peaks of BaAl2O4 were observed in the spectrum, and the diffraction characteristic peaks of (311) and (222) corresponding to γ-Al2O3 were more visible than those of single doping of Ba. This phenomenon indicated that the introduction of Ba atoms distorted the alumina crystal, and the doped La atoms could induce the distortion and promote the formation of BaAl2O4. The XRD patterns of “La + Ce” doped with three elements were similar to that of “La + Ce” doped. However, the diffraction characteristic peak of α-Al2O3 was more visible; as a result, the specific surface area of this group was the lowest, indicating mutual interference between the elements when the three elements were doped, which reduced the inhibitory effect of the crystal transformation of alumina.
3.3 Infrared spectroscopic analysis
Based on the above discussion results, this section discusses the functional group changes on the surface of Al2O3 before and after doping and modification with La + Ba As shown in Figure 7, the corresponding infrared spectra of each sample are mainly the vibration peaks of -OH and Al-O (Xu et al., 2022). Among them, the stretching vibration at 3,450 and 1,644 cm−1 corresponds to that of -OH, and the vibration at 1,030 cm−1 corresponds to that of Al-O. Studies have shown that the essence of the sintering phase transition process of Al2O3 is its dehydroxylation process (Wu et al., 2021). For the sample treated at 550°C, the peak area of La + Ba doped modified Al2O3 sample is larger at 3,450 cm−1, and the strength value is higher. This means that the modified sample has a higher content of γ-Al2O3. In the sample treated at 1,100°C, the diffraction peak area at 3,450 cm−1 is smaller, which indicates that the transition from γ-Al2O3 to α-Al2O3 is irreversible.
Compared with the spectra of modified samples containing La + Ba after aging at 1,100°C, the peak areas of undoped samples at 3,450 and 1,644 cm−1 were smaller, indicating that the modified samples retained more -OH, that is, more γ-Al2O3. The results of the infrared analysis showed that the incorporation of La + Ba could hinder the crystal transformation of alumina, which was consistent with the XRD test results. Numerous Al-O vibration peaks at 1,101, 826, 665, 558, and 462 cm−1 were observed in the fingerprint region (Hiremath et al., 2020), indicating the formation of the Al-O-Al bond and the corresponding oxygen ion vacancies in the structure (Feng et al., 2011; Belekar and Dhoble, 2018; Li et al., 2020; Yang et al., 2020). La and Ba atoms affected the diffusion and rearrangement of O2−and Al3+ by occupying the corresponding lattice vacancies (Arai and Machida, 1996; Ozawa and Nishio, 2016).
3.4 Morphological observation
Figure 8 shows the SEM image of the sample after aging at 1,100°C for 4 h. The samples before and after aging were tested using TEM to better compare the microstructure difference before and after aging. Figure 9 shows the TEM image of the sample before and after aging. Under the condition of 500 times magnification, the pure Al2O3 synthesized using the sol-gel method had an irregular polyhedron shape, and the surface was mainly flat and smooth, with only a few depression marks, and the diameter was about 3–8 μm (Figure 8). The Al2O3 modified by doping had a rough surface, with clear gaps and folds, and more holes. The size of the holes was larger than that of the pure Al2O3. As shown in Figure 8, the TEM image of pure Al2O3 before aging was fog-like. The interior of the pure Al2O3 mainly contained fine particles, and each particle was evenly distributed, with abundant pores between the particles. TEM images revealed cracks in the samples after aging, and there were agglomerations between the particles, indicating that the pores of Al2O3 were reduced during the transformation from γ phase to α phase after high-temperature aging, thus significantly decreasing specific surface area. The observed cracks were attributed to different orientations between grains. The La and Ba-modified samples displayed thick dark lines in the TEM images before aging, and other samples were similar to the TEM images of pure Al2O3 before aging. By comparing the TEM images after aging, the pores in the samples doped with La and Ba were reduced. However, the agglomerated products of the modified samples were significantly larger than those of pure Al2O3 samples after calcination, indicating that the transition from γ phase to α phase was insignificant.
4 Conclusion
In this study, active Al2O3 was synthesized via a sol-gel method. La, Ce, and Ba were used as high-temperature stabilizing additives. The effects and mechanism of different contents of La, Ce, Ba, and mixed doping on active Al2O3 were investigated.
1) La, Ce, and Ba inhibited the crystal transformation from γ-Al2O3 to α-Al2O3, and the incorporation of 1% Ba exhibited an excellent inhibitory effect on the crystal transformation. The addition of La and Ba atoms to Al2O3 distorted the Al2O3 crystal. The crystal structure of BaAl2O4 was more stable than that of α-Al2O3. The O atoms in Al2O3 preferentially combined with Ce atoms to form CeO2.
2) The co-doping of La and Ba exhibited the best inhibitory effect on the crystal transformation of active Al2O3. XRD analysis results revealed a synergistic effect between La and Ce, which promoted the formation of LaAlO3 and CeO2. Ba atoms caused the distortion of alumina crystal, while the doped La atoms accelerated the distortion and promoted the formation of BaAl2O4. The infrared analysis results showed that the modified sample retained more γ-Al2O3. SEM analysis revealed the rough surface of active Al2O3 and gaps on the surface after the mixed modification of the sample with La and Ba. TEM analysis results showed that La and Ba inhibited the crystal transformation of active Al2O3.
Data availability statement
The raw data supporting the conclusion of this article will be made available by the authors, without undue reservation.
Author contributions
ZW: Conceptualization, investigation, writing-original draft, data curation. LJ: Validation, resources, writing—review and editing, supervision. QD: Validation, formal analysis, investigation, data curation. QG: Formal analysis, project administration. JC: Formal analysis, project administration. XC: Methodology, conceptualization.
Funding
The authors appreciate the support of the Sichuan Science and Technology Program (2021YFQ0045 and 2021YFSY0056) and the Cnooc’s preliminary project: Feasibility study of Wenchang 9-7 oilfield (2021PFS-05). This study was financially supported by 111 Project, No. D18016.
Acknowledgments
The authors would also like to thank the Advanced Cementing Materials Research Center of SWPU for their kind assistance with the experiments.
Conflict of interest
Authors ZW and LJ were employed by the company CNOOC Research Institute Co., Ltd. and author QD was employed by the company Exploration Business Department of PetroChina Southwest Oil and Gas Field Company.
The remaining authors declare that the research was conducted in the absence of any commercial or financial relationships that could be construed as a potential conflict of interest.
Publisher’s note
All claims expressed in this article are solely those of the authors and do not necessarily represent those of their affiliated organizations, or those of the publisher, the editors and the reviewers. Any product that may be evaluated in this article, or claim that may be made by its manufacturer, is not guaranteed or endorsed by the publisher.
References
Aghamohammadi, S., Haghighi, M., Maleki, M., and Rahemi, N. (2017). Sequential impregnation vs. sol-gel synthesized Ni/Al2O3 -CeO2 nanocatalyst for dry reforming of methane: Effect of synthesis method and support promotion. Molecular Catalysis 431, 39–48. doi:10.1016/j.mcat.2017.01.012
Arai, H., and Machida, M. (1996). Thermal stabilization of catalyst supports and their application to high-temperature catalytic combustion. Applied Catalysis A: General 138, 161–176. doi:10.1016/0926-860x(95)00294-4
Azizi, Y., Kambolis, A., Boréave, A., Giroir-Fendler, A., Retailleau-Mevel, L., Guiot, B., et al. (2016). NOx abatement in the exhaust of lean-burn natural gas engines over Ag-supported γ-Al2O3 catalysts. Surface Science 646, 186–193. doi:10.1016/j.susc.2015.10.020
Belekar, R. M., and Dhoble, S. J. (2018). Activated Alumina Granules with nanoscale porosity for water defluoridation. Nano-Structures Nano-Objects 16, 322–328. doi:10.1016/j.nanoso.2018.09.007
Bettman, M. C. R. E., Otto, K., et al. (1989). Dispersion studies on the system of La2O3/γ-Al2O3. J. Catal. 117 (2), 447–454. doi:10.1016/0021-9517(89)90354-0
Chen, X., Lin, J., Zheng, Y., Zhan, Y., Zhang, W., Xiao, Y., et al. (2021). Catalytic methane oxidation performance over Pd/γ-Al2O3 catalyst optimized by the synergy of phosphorus and MOx (M = La, Ba and Zr). Fuel 299, 120933. doi:10.1016/j.fuel.2021.120933
Chen, X., Zheng, Y., Huang, F., Xiao, Y., Cai, G., Zhang, Y., et al. (2018). Catalytic activity and stability over nanorod-like ordered mesoporous phosphorus-doped alumina supported palladium catalysts for methane combustion. ACS Catal. 8 (12), 11016–11028. doi:10.1021/acscatal.8b02420
Deng, S., and Zhou, J. (2020). Research progress of automobile exhaust pollution control technology. Chemical Design Newsletter 46 (8), 213–214. in Chinese.
Feng, G., Huo, C., Li, Y., Wang, J., and Jiao, H. (2011). Structures and energies of iron promoted γ-Al2O3 surface: A computational study. Chemical Physics Letters 510 (4-6), 224–227. doi:10.1016/j.cplett.2011.05.035
Feng, Z., Ren, Q., Peng, R., Mo, S., Zhang, M., Fu, M., et al. (2019). Effect of CeO2 morphologies on toluene catalytic combustion. Catalysis Today 332, 177–182. doi:10.1016/j.cattod.2018.06.039
Gong, C. M., Lin, Z. E., Gao, S. J., et al. (2001). Study on the preparation chemistry of new high-temperature and high-surface alumina materials Ⅳ. The influence of lanthanum composition on the preparation of high-surface-area Al2O3 by sol-gel method. Journal of Fuel Chemistry and Technology 1, 76–79. in Chinese.
Hiremath, V., Shiferraw, B. T., and Seo, J. G. (2020). MgO insertion endowed strong basicity in mesoporous alumina framework and improved CO2 sorption capacity. Journal of CO2 Utilization 42, 101294. doi:10.1016/j.jcou.2020.101294
Hosseini, Z., Taghizadeh, M., and Yaripour, F. (2011). Synthesis of nanocrystalline γ-Al2O3 by sol-gel and precipitation methods for methanol dehydration to dimethyl ether. Journal of Natural Gas Chemistry 20 (2), 128–134. doi:10.1016/s1003-9953(10)60172-7
Jing, Y., Cai, Z., Liu, C., Toyao, T., Maeno, Z., Asakura, H., et al. (2020). Promotional effect of La in the three-way catalysis of La-loaded Al2O3-supported Pd catalysts (Pd/La/Al2O3). ACS Catalysis 10 (2), 1010–1023. doi:10.1021/acscatal.9b03766
Jing, Y., Wang, G., Ting, K. W., Maeno, Z., Oshima, K., Satokawa, S., et al. (2021). Roles of the basic metals La, Ba, and Sr as additives in Al2O3-supported Pd-based three-way catalysts. Journal of Catalysis 400, 387–396. doi:10.1016/j.jcat.2021.06.016
Li, B., Li, S., Wang, Y., Zhang, W., and Lin, B. (2010). Intergrowth effects in CeO2-Y-Al2O3 mixed oxides. Chinese Journal of Catalysis 31 (5), 528–534. doi:10.1016/s1872-2067(09)60067-x
Li, L., Gan, Y., Lu, Z., XiaohuYu, , Qing, S., Gao, Z., et al. (2020). The effects of Fe, Co and Ni doping in CuAl2O4 spinel surface and bulk: A dft study. Applied Surface Science 521, 146478. doi:10.1016/j.apsusc.2020.146478
Lin, C., Yang, Z., Pan, H., Cui, J., Lv, Z., liu, X., et al. (2021). Ce-introduced effects on modification of acidity and Pt electronic states on Pt-Sn/γ-Al2O3 catalysts for catalytic reforming. Applied Catalysis A: General 617, 118116. doi:10.1016/j.apcata.2021.118116
Lu, W., Long, J., and Tian, H. (2003). Effects of lanthanum and cerium modification on the properties of alumina. Journal of Catalysis 24 (8), 574–578. doi:10.3321/j.issn:0253-9837.2003.08.005
Ministry of Environmental Protection of the People's Republic of China (2020). China mobile source environmental management annual report. Beijing, China: Ministry of Environmental Protection of the People's Republic of China. in Chinese.
Ozawa, M., and Nishio, Y. (2016). Thermal stability and microstructure of catalytic alumina composite support with lanthanum species. Applied Surface Science 380, 288–293. doi:10.1016/j.apsusc.2015.12.246
Pang, Y., Wu, Y., Chen, Y., Luo, F., and Chen, J. (2020). Degradation effect of Ce/Al2O3 catalyst on pyrolysis volatility of pine. Renewable energy 162, 134–143. doi:10.1016/j.renene.2020.07.125
Tang, M., Liu, K., Roddick, D. M., and Fan, M. (2018). Enhanced lattice oxygen reactivity over Fe2O3/Al2O3 redox catalyst for chemical-looping dry (CO2) reforming of CH4: Synergistic La-Ce effect. Journal of Catalysis 368, 38–52. doi:10.1016/j.jcat.2018.09.022
Tu, L. Q., and Ming, J. (2013). Research on purification and treatment technology of automobile exhaust. Jiangsu Science & Technology Information 18, 67. in Chinese. doi:10.3969/j.issn.1004-7530.2013.18.034
Wang, B., Wang, G. L., Sun, L. Z., et al. (2017). Summary of performance improvement of activated alumina coating in automobile exhaust gas purifier. Hebei College of Industry and Technology 34 (4), 282–286. in Chinese.
Wei, Q., Chen, Z.-X., Wang, Z.-H., Hao, Y. L., Zou, J. X., and Nie, Z. R. (2005). Effect of La, Ce, Y and B addition on thermal stability of unsupported alumina membranes. Journal of Alloys and Compounds 387 (1-2), 292–296. doi:10.1016/j.jallcom.2004.06.081
Wu, Q., Yan, J., Jiang, M., Dai, Q., Wu, J., Ha, M. N., et al. (2021). Phosphate-assisted synthesis of ultrathin and thermally stable alumina nanosheets as robust Pd support for catalytic combustion of propane. Applied Catalysis B: Environmental 286, 119949. doi:10.1016/j.apcatb.2021.119949
Xu, H., Zhang, C., Cai, J., Wang, J., Liu, K., and Cheng, X. (2022). Synthesis and characterization of activated alumina with high thermal stability by a low-heat solid-phase precursor method. Microporous Mesoporous Mater 337, 111921. doi:10.1016/j.micromeso.2022.111921
Yadav, M., and Sharma, Y. C. (2019). Transesterification of used vegetable oil using BaAl2O4 spinel as heterogeneous base catalyst. Energy Conversion and Management 198, 111795. doi:10.1016/j.enconman.2019.111795
Yadav, S., Mittal, A., Sharma, S., Kumari, K., and Kumar, N. (2021). Highly efficient Ag2O loaded ZnO/Al2O3 coupled catalyst and its photocatalytic application. Inorganic Chemistry Communications 130, 108738. doi:10.1016/j.inoche.2021.108738
Yang, G., Li, Y., and Men, Y. (2015). Synergistic catalysis effect of Mn-promoted BaAl2O4 catalysts on catalytic performance for soot combustion. Catalysis Communications 69, 202–206. doi:10.1016/j.catcom.2015.06.020
Keywords: activated alumina, sol-gel, rare Earth metals, alkaline Earth metals, doping modification
Citation: Wu Z, Jiang L, Dong Q, Gao Q, Cai J and Cheng X (2023) Effect and mechanism of rare Earth and alkaline Earth metals on the high-temperature stability of activated alumina. Front. Mater. 10:1103590. doi: 10.3389/fmats.2023.1103590
Received: 21 November 2022; Accepted: 09 January 2023;
Published: 19 January 2023.
Edited by:
Jianxin Yi, University of Science and Technology of China, ChinaReviewed by:
Sufang He, Kunming University of Science and Technology, ChinaGang Feng, Nanchang University, China
Copyright © 2023 Wu, Jiang, Dong, Gao, Cai and Cheng. This is an open-access article distributed under the terms of the Creative Commons Attribution License (CC BY). The use, distribution or reproduction in other forums is permitted, provided the original author(s) and the copyright owner(s) are credited and that the original publication in this journal is cited, in accordance with accepted academic practice. No use, distribution or reproduction is permitted which does not comply with these terms.
*Correspondence: Xiaowei Cheng, Y2hlbmd4d0Bzd3B1LmVkdS5jbg==