- 1Fourth Department of Gynecologic Oncology, Hunan Cancer Hospital/The Affiliated Cancer Hospital of Xiangya School of Medicine, Central South University, Changsha, China
- 2School of Chemistry and Chemical Engineering, Hunan University of Science and Technology, Xiangtan, China
- 3Hunan Veterinary Medicine and Feed Supervision Institute, Changsha, China
The cytokine interferon gamma (IFN-γ) and doxorubicin mono-therapy has been approved by the Food and Drug Administration (FDA) for the treatment of tumors. The importance of IFN-γ in the immune system lies in its immunomodulatory effects, and the importance of doxorubicin in antitumor therapy lies in inhibiting RNA and DNA synthesis. In this work, the role of IFN-γ in the antitumor activity in combination with doxorubicin was investigated. Meanwhile, IFN-γ was used as a vehicle to load doxorubicin over immunotherapy and chemotherapy for synergistic therapy. IFN-γ/doxorubicin complex nanoparticles were prepared by a fusion method with a size of approximately 13 nm and a low polydispersity index. The doxorubicin release profile was analyzed with different pH ranges, and it showed an enhanced release in acidic pH. The ability of IFN-γ/doxorubicin complex nanoparticles to induce human ovarian carcinoma cell (Skov 3) apoptosis was evaluated by the cytotoxicity test. The cellular uptake of IFN-γ/doxorubicin complex nanoparticles was time-dependent, and the IFN-γ/doxorubicin complex nanoparticles showed a higher apoptosis efficiency than free doxorubicin by flow cytometry analysis and fluorescence imaging. This work bridged IFN-γ with doxorubicin to utilize their potential for antitumor activities, opening new avenues for their use in clinical settings.
Introduction
IFN-γ is thought to be a pleiotropic cytokine with antiviral, anti-proliferative, and immunomodulatory functions for a long time (García et al., 2019). IFN-γ plays a central role in the progression of a variety of inflammatory and autoimmune diseases (Dufour et al., 2018). Growing IFN-γ production is also associated with rheumatoid arthritis. The complex effects of IFN-γ are due to its extremely pleiotropic nature. Moreover, it has played an important role in the antitumor effect (Perry et al., 2018). IFN-γ has a positive impact on antitumor responses by creating an inflamed tumor microenvironment which is a prognostic biomarker for immunotherapy (Blankenstein and Qin, 2003). Indeed, its effect on APCs is in a large part responsible for the induction of strong cytotoxic T-cell responses against tumor (Castro et al., 2019). IFN-γ is considered one of the main antitumor cytokines. Numerous antitumor effects of IFN-γ have been described (Kursunel and Esendagli, 2016). IFN-γ provides the dualistic role in the regulation of tumor progression (Jorgovanovic et al., 2020). The inflammatory regulation of IFN-γ in tumor immune surveillance can be executed by directly inducing the inhibitory molecule expression, such as PDL-1, on tumor cells. IFN-γ directly increases apoptosis of tumor cells by inducing MHC class I expression, APC priming, Th1 induction, and reducing proliferation and angiogenesis (Pulido et al., 2020). IFN-γ could activate JAK-STAT1-caspase signaling and facilitate transcription and synthesis of caspase 3 and caspase 7, which further initiated apoptotic processes in cancer cells (Bhattarai et al., 2016; Yu et al., 2017). IFN-γ could also decrease the number of endothelial cells by downregulating the expression of vascular endothelial growth factor A, inducing blood vessel destruction and promoting tumor tissue necrosis (Xu et al., 2019).
Doxorubicin is a small molecule therapeutic agent that exerts cytotoxic effects on tumor cells by inhibiting topoisomerase II, intercalating DNA, and generating free radicals (Schürmann et al., 2021). Also, many articles support that doxorubicin causes consequential lipid peroxidation, calcium dysregulation, and intervention in energy transfer (Quagliariello et al., 2019; Syahputra et al., 2022). Therefore, doxorubicin is an effective agent used commonly to treat a wide range of human malignant neoplasms, including a variety of solid tumors, breast cancer, acute lymphoblastic leukemia, pediatric leukemia, lung cancer, lymphomas, and several metastatic cancers, but easily leads to systemic toxicities in the heart, brain, and kidney and short circulation half-life (Yousefpour et al., 2019). Therefore, its use is limited by maximum dose, owing to its chronic toxicity and other side effects (Chen et al., 2021).
IFN-γ and doxorubicin can be exploited for nano-drug formulations and allow for selective targeting in tumors and enhancing the antitumor effect (Yan et al., 2020). IFN-γ easily binds to tumor cells and helps drug better penetrate into the tumor cells. Doxorubicin possesses hydrophobic character, which can also be adjusted by IFN-γ decoration (Lv et al., 2018; Yin et al., 2018). In addition, the size of complex is beneficial to increasing the accumulation of drugs in the tumor site by the enhanced permeability and retention (EPR) effects associated with the leakiness of the solid tumor vasculature (Gong et al., 2021). Furthermore, IFN-γ has the long half-life which can extend the biological half-life of doxorubicin and minimize toxicity against normal cells. IFN-γ and doxorubicin work together to maximize the antitumor effect (Rogers et al., 2007; Zhang et al., 2022). This evidence prompted us to investigate the function of IFN-γ in the antitumor activity in combination with doxorubicin (Lv et al., 2018; Yin et al., 2018).
Herein, we reported a facile and versatile method to formulate doxorubicin using IFN-γ to obtain high anticancer efficiency and high biocompatibility (Scheme 1). The conjugation of IFN-γ and doxorubicin only involves one processing step, which could not alter the structure and conformation of IFN-γ. The size and drug loading of these complexes are evaluated in detail. These complexes are used to initiate apoptosis in Skov 3, verified by cell viability, flow cytometry, and cell imaging. This report provides a future therapeutic strategy based on the IFN-γ and doxorubicin complex.
Methods
Skov 3 cells, derived from human ovarian carcinoma, were cultured in RPMI 1640 (Sigma), 10% fetal bovine serum (Gibco), 100 units/mL penicillin (Beyotime Biotechnology (Shanghai, China)), and 100 μg/ml streptomycin (Beyotime Biotechnology (Shanghai, China)), as described previously (Chen et al., 2021). Doxorubicin was purchased from Beyotime Biotechnology (Shanghai, China). IFN-γ, Annexin V-FITC Apoptosis Detection Kit, Terminal Deoxynucleotidyl-Transferase–Mediated dUTP Nick End-Labeling (TUNEL) Apoptosis Detection Kit (FITC), and 4′,6-diamidino-2-phenylindole (DAPI) were purchased from Shanghai WellBio Technology Co., Ltd. (Shanghai, China)
IFN-γ (100 mg) and doxorubicin (10 mg) were mixed in PBS solutions and stirred for 24 h at room temperature. Hydrophobic interactions possibly drove IFN-γ and doxorubicin to aggregate in aqueous solution. Then, the mixture was washed with PBS (1.5 ml*3) in an Amicon centrifugal filter (molecular weight cutoff = 10 kDa; Millipore, United States) to remove unloaded doxorubicin. The final loading capacity of doxorubicin in IFN-γ was 7.1 wt% measured by using a fluorometer (Thermo Scientific Fluoroskan Ascent FL), according to the doxorubicin standard curve, and the encapsulation efficiency was 71%. The hydrodynamic diameter of IFN-γ/doxorubicin was measured by dynamic light scattering (DLS, Malvern ZEN 3600 Instrument). The morphology of IFN-γ/doxorubicin was measured by transmission electron microscopy (TEM, Thermo Fisher Scientific, Talos F200i Instrument).
Cell viability was evaluated by Cell Counting Kit-8 (CCK-8) (Dojin Laboratories, Kumamoto, Japan). Skov 3 cells were plated at a density of 1.5 × 104 per well in 96-well plates for 12 h. Then, the cells were treated with IFN-γ/doxorubicin complex nanoparticles (0.1, 0.25, 0.5, 1, 2.5, 5, 10, and 20 μg/ml), doxorubicin (7.1, 17.75, 35.5, 71, 177.5, 355, 710, and 1420 ng/ml), and IFN-γ (0.1, 0.25, 0.5, 1, 2.5, 5, 10, and 20 μg/ml). After 24 h, 10 µl of CCK-8 solution was added to each well, and then the cells were incubated for 2 h at 37°C, 5% CO2. The absorbance was determined by using a TECAN infinite M200 plate reader (Tecan, Mechelen, Belgium) at 450 nm.
Flow cytometry was performed to analyze the cell apoptosis of IFN-γ/doxorubicin complex nanoparticles. Skov 3 cells were set up with the control and IFN-γ/doxorubicin complex nanoparticle groups (10, 0.71, and 10 μg/ml). Each group was harvested after 6, 12, and 24 h of culture, respectively, washed with PBS buffer three times, and re-suspended in binding buffer. The cells were stained with Annexin V-FITC Apoptosis Detection Kit according to the manufacturer’s instructions. The Skov 3 cells were immediately evaluated by flow cytometry.
TUNEL immunohistochemistry was performed to analyze cell apoptosis. Skov 3 cells were set up with the control and IFN-γ/doxorubicin complex nanoparticle groups (10, 0.71, and 10 μg/ml). Each group was harvested after 6, 12, and 24 h of culture, respectively. Each group was washed with PBS buffer three times to remove IFN-γ, doxorubicin, and IFN-γ/doxorubicin complex nanoparticles. Then, the TUNEL apoptosis assay was performed according to the manufacturer’s instructions. The Skov 3 cells were immediately observed by the fluorescence photomicrograph.
Results and Discussion
As shown in Figure 1, the mean diameters of IFN-γ and doxorubicin-loaded IFN-γ complex were 10 and 13 nm, respectively. The sizes of doxorubicin-loaded IFN-γ slightly increased, when compared to those of the blank IFN-γ, especially contributing to the doxorubicin intercalation into IFN-γ. A large amount of doxorubicin was intercalated in IFN-γ. The driving force is mainly non-covalent force, including the hydrophobic force between IFN-γ and doxorubicin and π–π stacking force between doxorubicin and hydrogen bonding. Therefore, IFN-γ exhibited rather high doxorubicin loading (7.1 wt%) and encapsulation efficiencies. The morphology of IFN-γ/doxorubicin complex was observed by TEM. The diameter results from TEM were basically identical to the results of hydrodynamic diameter. As shown in Figure 2, IFN-γ and IFN-γ/doxorubicin complex characterized the same dot morphology. The IFN-γ/doxorubicin complex showed a larger diameter (≈10 nm) than free IFN-γ, which ensured the loading of doxorubicin.
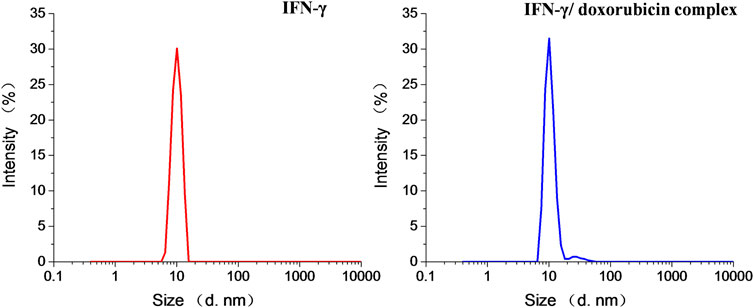
FIGURE 1. Intensity hydrodynamic diameters of IFN-γ and the IFN-γ/doxorubicin complex measured by DLS.
The release properties of doxorubicin from the IFN-γ complex were investigated in pH ranges 4.5, 6.5, and 7.4 to simulate the acidic tumor microenvironment and normal physiological environment (Figure 3). The cumulative percentage of the doxorubicin release profile from IFN-γ was investigated over 80 h. Then, 40% of doxorubicin was released after 2 h in pH 4.5, and the amount of released doxorubicin gradually increased (50, 60, and 80% after 5, 8, and 24 h, respectively). The more acidic the pH was, the faster the release rate was. The release of doxorubicin could be accelerated in the acidic tumor environment, which favors doxorubicin accumulation in tumor sites. The doxorubicin release in the acidic environment exhibited a burst behavior initially in the first 24 h, followed by a sustained release. The doxorubicin release in pH 7.4 exhibited a slow release behavior (30% after 80 h), which reduced drug leakage in the normal physiological environment.
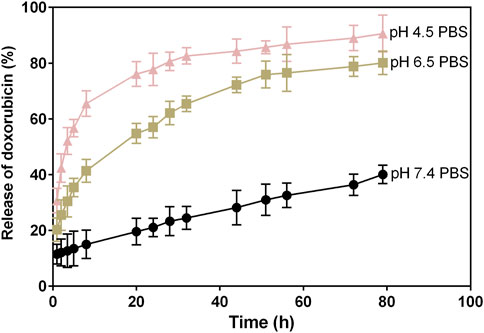
FIGURE 3. In vitro release of doxorubicin from the IFN-γ/doxorubicin complex in pH 4.5, 6.5, and 7.4.
IFN-γ is well described as a helpful modulator of cancer cell function, and doxorubicin’s use has still been limited due to its high toxicity and non-targeting. To evaluate the enhanced cancer cell therapy of the IFN-γ/doxorubicin complex, the cell viability was investigated on the IFN-γ/doxorubicin complex and their respective controls via CCK-8 analysis. Representative histogram profiles and dot plots for the antitumor effect by different concentrations of IFN-γ/doxorubicin complex are shown in Figure 4. IFN-γ treatment, doxorubicin treatment, and IFN-γ/doxorubicin complex treatment were efficient at inhibiting Skov 3 cells. But IFN-γ/doxorubicin complex treatment enhanced the optical density to a higher extent than IFN-γ or doxorubicin treatment (Figure 4A). Responsively, IFN-γ/doxorubicin complex treatment significantly decreased the cell viability of Skov 3, when compared to IFN-γ or doxorubicin treatment (Figure 4B). The half maximal inhibitory concentration (IC50) value of IFN-γ/doxorubicin complex treatment was 10 μg/ml. The significant difference of cell viability was found in the IFN-γ/doxorubicin complex group. As expected, Skov 3 cells were in response to the distinct treatment of the IFN-γ/doxorubicin complex. These results suggested that the incorporation of IFN-γ and doxorubicin contributed to cancer cell death and tumor regression, which subsequently led to IFN-γ–mediated antitumor effects.
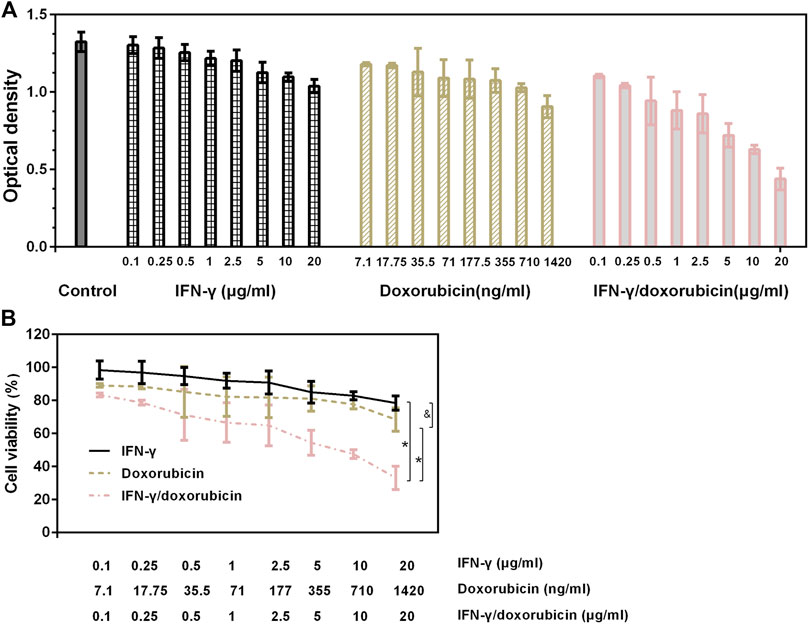
FIGURE 4. In vitro cytotoxicity of IFN-γ, doxorubicin, and IFN-γ/doxorubicin complex at different concentrations. Cells were incubated for 24 h with IFN-γ, doxorubicin, and the IFN-γ/doxorubicin complex. (A) Optical density of each group after CCK-8 treatment. (B) Corresponding cell viability of each group. Data are presented as mean ± SEM, n = 6. *p < 0.05 and not significant, as compared with the IFN-γ/doxorubicin complex group.
The interaction of IFN-γ and doxorubicin would change cell viability to a higher level. The first promising results indicated that IFN-γ and doxorubicin could be used as a combined antitumor agent and explored the discovery of its apoptotic effects on cancer cells. In order to further evaluate the IFN-γ/doxorubicin complex’s ability to inhibit tumor cell growth, the cell apoptosis ability of Skov 3 cells treated with the IFN-γ/doxorubicin complex and their respective controls was investigated by flow cytometry. The cells were treated with IFN-γ, doxorubicin, and IFN-γ/doxorubicin complex at a concentration of 10 μg/ml at different times. At 6 h, the apoptosis rate of Skov 3 treated with IFN-γ and doxorubicin, respectively, was low, as compared to that of Skov 3 treated with the IFN-γ/doxorubicin complex (Figure 5). It was found that the apoptotic cells treated with the IFN-γ/doxorubicin complex distinctly increased, and the apoptosis rate was up to 42.03%, which was significantly higher than that of IFN-γ and doxorubicin treatment over 12 h. After 24 h, the apoptotic rate of IFN-γ/doxorubicin complex increased to 65.19% prior to that of IFN-γ and doxorubicin alone. As expected, the IFN-γ/doxorubicin complex could efficiently facilitate the apoptosis of Skov 3, suggesting that the IFN-γ/doxorubicin complex co-interacted with tumor cells and increased the cytotoxicity against tumor cells.
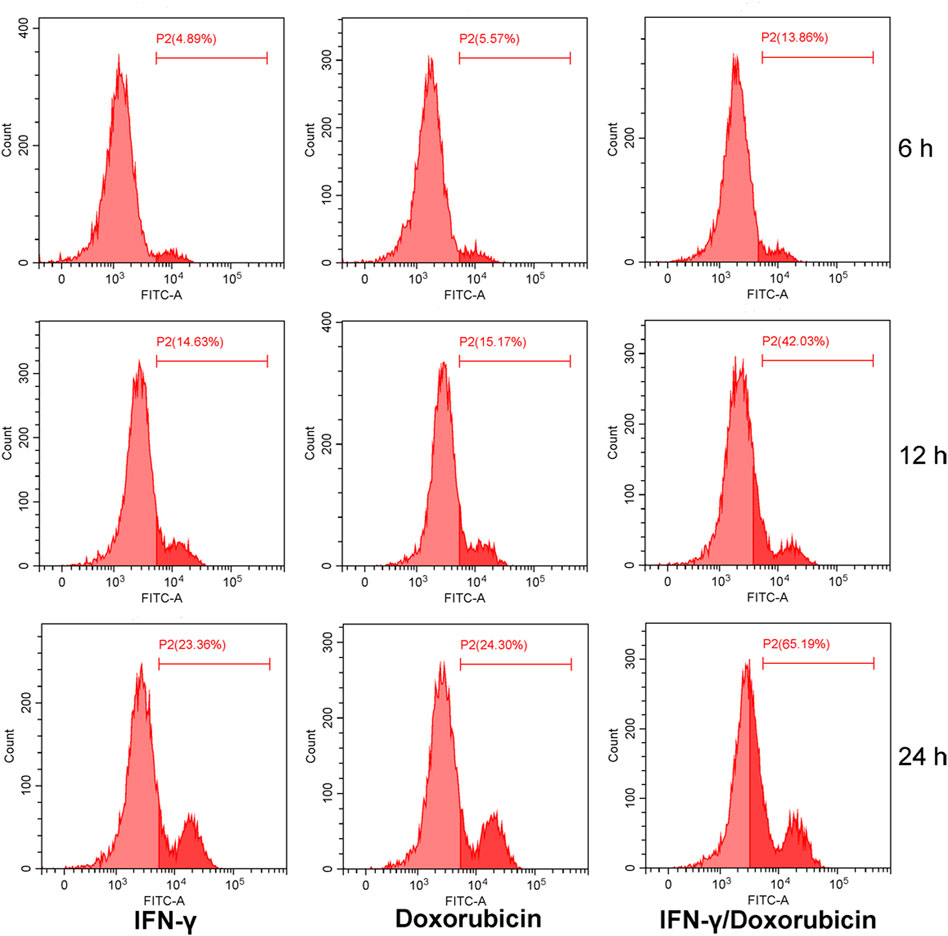
FIGURE 5. Cell apoptosis analysis of IFN-γ (10 μg/ml), doxorubicin (0.71 μg/ml), and the IFN-γ/doxorubicin complex (10 μg/ml). Cells were incubated for different times with IFN-γ, doxorubicin, and the IFN-γ/doxorubicin complex at 37 °C and stained with Annexin V-FITC.
IFN-γ is a key cytokine in tumor immunology, which is proposed to be a promoter that promotes apoptosis. Herein, the expression levels of apoptotic effect were analyzed by fluorescence imaging. The green fluorescence showed the apoptotic cells and was clearly located in the blue fluorescence when stained with DAPI, as shown in Figure 6. The apoptotic cells were not obvious after the treatment of IFN-γ and doxorubicin, respectively, while IFN-γ/doxorubicin complex treatment was upregulated at 6 h. Accordingly, the green fluorescence was more obvious after the treatment of IFN-γ/doxorubicin complex, indicating that the IFN-γ/doxorubicin complex had a sustained tumor growth inhibition feature. After 24 h treatment, the green fluorescence of all three groups increased with the extent of the incubation time. The results suggested that a long-time treatment was helpful for cell apoptosis for all three groups. Meanwhile, the IFN-γ/doxorubicin complex contributed to the high intracellular content of the drug. Importantly, the IFN-γ/doxorubicin complex group showed the most significant apoptotic effect, which could be explained by the enhanced green fluorescence of TUNEL in Skov 3 cells. The strongest green fluorescence in the IFN-γ/doxorubicin complex group indicated that IFN-γ could deliver the largest amount of doxorubicin to Skov 3 and perform the synergistic effect with doxorubicin. IFN-γ had the long half-life which could extend the biological half-life of doxorubicin and maximize toxicity against tumor cells.
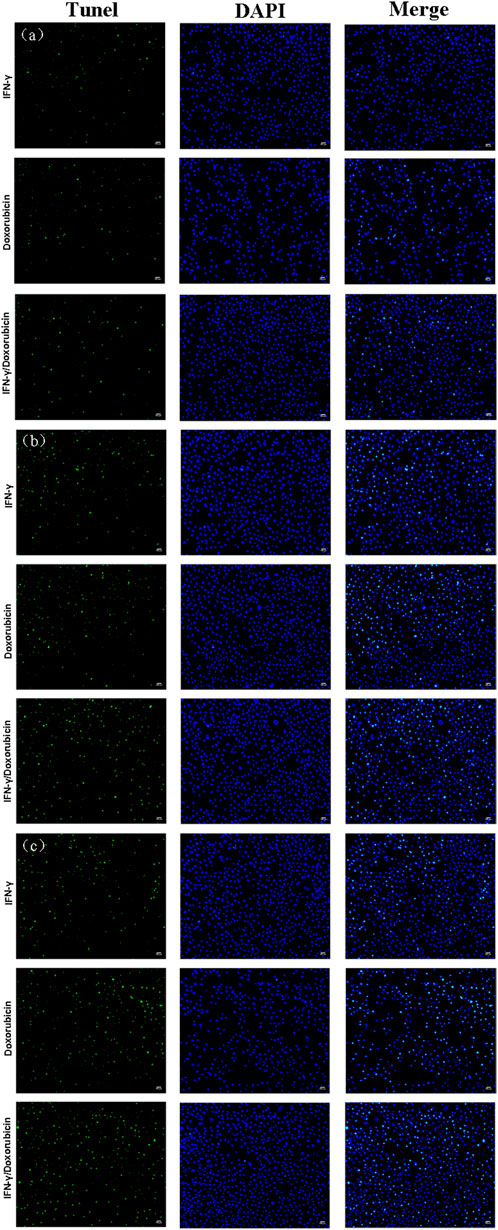
FIGURE 6. TUNEL analysis of Skov 3 cells incubated with IFN-γ (10 μg/ml), doxorubicin (0.71 μg/ml), and the IFN-γ/doxorubicin complex (10 μg/ml) for 6 h (A), 12 h (B), and 24 h (C) at 37 °C, followed by incubation with the TUNEL analysis reagent (green) to stain apoptotic cells and DAPI (blue) to stain cell nuclei. Scale bars: 100 μm.
Conclusion
In this study, doxorubicin was successfully intercalated into IFN-γ with a loading capacity of 7.1 wt% and a size of 13 nm. The IFN-γ/doxorubicin complex exhibited facile drug release preferentially in the lower pH environments, such as cancer tissues, for cell apoptosis. Although IFN-γ could disrupt Skov 3 cell growth and lead to cell death, it could help deliver doxorubicin and accelerate cell apoptosis. The IFN-γ/doxorubicin complex showed the strongest effects in killing tumor cells. This study represented the preliminary research in an effort to combine IFN-γ with doxorubicin to modulate tumor cells and enhance chemotherapy. It is also important to fully understand antitumor combination interactions between IFN-γ and doxorubicin. Next, exploring the exclusivity of the IFN-γ/doxorubicin relationship serves to the discovery of new therapeutic targets for cancer treatment.
Data Availability Statement
The original contributions presented in the study are included in the article/Supplementary Material, and further inquiries can be directed to the corresponding author.
Author Contributions
All authors listed have made a substantial, direct, and intellectual contribution to the work and approved it for publication.
Funding
This project was financially supported by the Scientific Research Fund of Hunan Provincial Education Department (Grant No. 19C0774), the Natural Science Foundation of Hunan Province, China (Grant No. 2020JJ5156), and the Student Research and Innovation Program of Hunan University of Science and Technology (Grant Nos. YZ2143 and ZZ2109).
Conflict of Interest
The authors declare that the research was conducted in the absence of any commercial or financial relationships that could be construed as a potential conflict of interest.
Publisher’s Note
All claims expressed in this article are solely those of the authors and do not necessarily represent those of their affiliated organizations, or those of the publisher, the editors, and the reviewers. Any product that may be evaluated in this article, or claim that may be made by its manufacturer, is not guaranteed or endorsed by the publisher.
References
Bhattarai, S., Ghannam, K., Krause, S., Benveniste, O., Marg, A., de Bruin, G., et al. (2016). The Immunoproteasomes Are Key to Regulate Myokines and MHC Class I Expression in Idiopathic Inflammatory Myopathies. J. Autoimmun. 75, 118–129. doi:10.1016/j.jaut.2016.08.004
Blankenstein, T., and Qin, Z. (2003). The Role of IFN-γ in Tumor Transplantation Immunity and Inhibition of Chemical Carcinogenesis. Curr. Opin. Immunol. 15 (2), 148–154. doi:10.1016/s0952-7915(03)00007-4
Castro, F., Pinto, M. L., Almeida, R., Pereira, F., Silva, A. M., Pereira, C. L., et al. (2019). Chitosan/poly(γ-glutamic Acid) Nanoparticles Incorporating IFN-γ for Immune Response Modulation in the Context of Colorectal Cancer. Biomater. Sci. 7 (8), 3386–3403. doi:10.1039/c9bm00393b
Chen, J., Liu, H., Li, X., Li, J., Tang, R., Deng, Z., et al. (2021). Dually Acid- and GSH-Triggered Bis(β-Cyclodextrin) as Drugs Delivery Nanoplatform for Effective Anticancer Monotherapy. Nanotechnology 32 (14), 145714. doi:10.1088/1361-6528/abd7b1
Dufour, A., Bellac, C. L., Eckhard, U., Solis, N., Klein, T., Kappelhoff, R., et al. (2018). C-Terminal Truncation of IFN-γ Inhibits Proinflammatory Macrophage Responses and Is Deficient in Autoimmune Disease. Nat. Commun. 9 (1), 2416. doi:10.1038/s41467-018-04717-4
García, J. R., Quirós, M., Han, W. M., O'Leary, M. N., Cox, G. N., Nusrat, A., et al. (2019). IFN-γ-tethered Hydrogels Enhance Mesenchymal Stem Cell-Based Immunomodulation and Promote Tissue Repair. Biomaterials 220, 119403. doi:10.1016/j.biomaterials.2019.119403
Gong, Z., Zhou, B., Liu, X., Cao, J., Hong, Z., Wang, J., et al. (2021). Enzyme-Induced Transformable Peptide Nanocarriers with Enhanced Drug Permeability and Retention to Improve Tumor Nanotherapy Efficacy. ACS Appl. Mat. Interfaces 13 (47), 55913–55927. doi:10.1021/acsami.1c17917
Jorgovanovic, D., Song, M., Wang, L., and Zhang, Y. (2020). Roles of IFN-γ in Tumor Progression and Regression: a Review. Biomark. Res. 8 (1), 49. doi:10.1186/s40364-020-00228-x
Kursunel, M. A., and Esendagli, G. (2016). The Untold Story of IFN-γ in Cancer Biology. Cytokine & Growth Factor Rev. 31, 73–81. doi:10.1016/j.cytogfr.2016.07.005
Lv, Q., He, C., Quan, F., Yu, S., and Chen, X. (2018). DOX/IL-2/IFN-γ Co-loaded Thermo-Sensitive Polypeptide Hydrogel for Efficient Melanoma Treatment. Bioact. Mater. 3 (1), 118–128. doi:10.1016/j.bioactmat.2017.08.003
Perry, C. J., Muñoz-Rojas, A. R., Meeth, K. M., Kellman, L. N., Amezquita, R. A., Thakral, D., et al. (2018). Myeloid-targeted Immunotherapies Act in Synergy to Induce Inflammation and Antitumor Immunity. J. Exp. Med. 215 (3), 877–893. doi:10.1084/jem.20171435
Pulido, M., Chamorro, V., Romero, I., Algarra, I., S-Montalvo, A., Collado, A., et al. (2020). Restoration of MHC-I on Tumor Cells by Fhit Transfection Promotes Immune Rejection and Acts as an Individualized Immunotherapeutic Vaccine. Cancers 12 (6), 1563. doi:10.3390/cancers12061563
Quagliariello, V., Coppola, C., Mita, D. G., Piscopo, G., Iaffaioli, R. V., Botti, G., et al. (2019). Low Doses of Bisphenol A Have Pro-inflammatory and Pro-oxidant Effects, Stimulate Lipid Peroxidation and Increase the Cardiotoxicity of Doxorubicin in Cardiomyoblasts. Environ. Toxicol. Pharmacol. 69, 1–8. doi:10.1016/j.etap.2019.03.006
Rogers, K. M. A., Thomas, M., Galligan, L., Wilson, T. R., Allen, W. L., Sakai, H., et al. (2007). Cellular FLICE-Inhibitory Protein Regulates Chemotherapy-Induced Apoptosis in Breast Cancer Cells. Mol. cancer Ther. 6 (5), 1544–1551. doi:10.1158/1535-7163.mct-06-0673
Schürmann, L., Schumacher, L., Roquette, K., Brozovic, A., and Fritz, G. (2021). Inhibition of the DSB Repair Protein RAD51 Potentiates the Cytotoxic Efficacy of Doxorubicin via Promoting Apoptosis-Related Death Pathways. Cancer Lett. 520, 361–373. doi:10.1016/j.canlet.2021.08.006
Syahputra, R. A., Harahap, U., Dalimunthe, A., Nasution, M. P., and Satria, D. (2022). The Role of Flavonoids as a Cardioprotective Strategy against Doxorubicin-Induced Cardiotoxicity: A Review. Molecules 27 (4), 1320. doi:10.3390/molecules27041320
Xu, Y., Zhang, X., Wang, Y., Pan, M., Wang, M., and Zhang, J. (2019). A VEGFR2-MICA Bispecific Antibody Activates Tumor-Infiltrating Lymphocytes and Exhibits Potent Anti-tumor Efficacy in Mice. Cancer Immunol. Immunother. 68 (9), 1429–1441. doi:10.1007/s00262-019-02379-9
Yan, J., Chen, J., Zhang, N., Yang, Y., Zhu, W., Li, L., et al. (2020). Mitochondria-targeted Tetrahedral DNA Nanostructures for Doxorubicin Delivery and Enhancement of Apoptosis. J. Mat. Chem. B 8 (3), 492–503. doi:10.1039/c9tb02266j
Yin, Y., Hu, Q., Xu, C., Qiao, Q., Qin, X., Song, Q., et al. (2018). Co-delivery of Doxorubicin and Interferon-γ by Thermosensitive Nanoparticles for Cancer Immunochemotherapy. Mol. Pharm. 15 (9), 4161–4172. doi:10.1021/acs.molpharmaceut.8b00564
Yousefpour, P., Ahn, L., Tewksbury, J., Saha, S., Costa, S. A., Bellucci, J. J., et al. (2019). Conjugate of Doxorubicin to Albumin‐Binding Peptide Outperforms Aldoxorubicin. Small 15 (12), 1804452. doi:10.1002/smll.201804452
Yu, T., Zuo, Y., Cai, R., Huang, X., Wu, S., Zhang, C., et al. (2017). SENP1 Regulates IFN-Γ−stat1 Signaling through STAT3−SOCS3 Negative Feedback Loop. J. Mol. Cell Biol. 9 (2), 144–153. doi:10.1093/jmcb/mjw042
Keywords: IFN-γ, doxorubicin, antitumor, synergistic therapy, nanoparticles
Citation: Yi R, Lv W, Zheng S, Zhang N, Zhang Y, Yang K, Huang T, Yang Y, Chu H and Chen J (2022) IFN-γ/Doxorubicin Complex Nanoparticles for Enhancing Therapy in the Context of Human Ovarian Carcinoma. Front. Mater. 9:944930. doi: 10.3389/fmats.2022.944930
Received: 16 May 2022; Accepted: 24 June 2022;
Published: 25 July 2022.
Edited by:
Jianhua Zou, National University of Singapore, SingaporeReviewed by:
Dengfeng Zou, Guilin Medical University, ChinaLingjun Tong, National University of Singapore, Singapore
Jianwei Zhu, Nanjing Tech University, China
Copyright © 2022 Yi, Lv, Zheng, Zhang, Zhang, Yang, Huang, Yang, Chu and Chen. This is an open-access article distributed under the terms of the Creative Commons Attribution License (CC BY). The use, distribution or reproduction in other forums is permitted, provided the original author(s) and the copyright owner(s) are credited and that the original publication in this journal is cited, in accordance with accepted academic practice. No use, distribution or reproduction is permitted which does not comply with these terms.
*Correspondence: Jian Chen, Y2hlbmppYW56aGl5YW9AMTYzLmNvbQ==