- 1Department of Orthopedics Combined TCM with Western Medicine, Honghui Hospital, Xi’an Jiaotong University, Xi’an, China
- 2Department of Orthopaedics, Honghui Hospital, Xi’an Jiaotong University, Xi’an, China
- 3Medical School of Yan’an University, Yan’an, China
Micro/nanostructured TiO2, ion-doped TiO2, and heterojunction TiO2 composite photocatalysts have low toxicity, high biocompatibility, and high photocatalytic and antibacterial activities and have broad applications in the fields of photocatalytic, antibacterial, and orthopedic implants. The photocatalytic and antibacterial activities of TiO2 and TiO2-based photocatalysts depend on their preparation methods. In this review, the preparation methods of TiO2, ion-doped TiO2, and heterojunction TiO2 composite photocatalysts and their effects on photocatalytic and antibacterial activities were reviewed. Based on the excellent physical and chemical properties of TiO2, ion-doped TiO2, and heterojunction TiO2-based photocatalysts, their applications in the field of orthopedic implants were reviewed. Meanwhile, the development trend of the photocatalyst in the fields of photocatalysis, bacteriostasis, and medicine was prospected. The purpose of this review was to point out the direction for further study on photocatalytic and antibacterial activities and related applications of TiO2 and TiO2-based photocatalysts.
1 Introduction
The development of global economy has brought comfortable material living conditions and various conveniences to the people of the world, but at the same time, it has caused great pressure on the environment (Tiwari et al., 2022). Dyes are closely related to the clothes people wear, the paper they use, and the packaging of food and medicine. The use of these dyes discharges a large amount of organic dye wastewater into rivers, resulting in water pollution (Chow et al., 2021; Feng et al., 2021; Liu et al., 2022a). The most effective method to kill the pollution of organic dye is at the source, to solve the pollution of organic dye wastewater. Therefore, the development of new technical means to degrade organic dyes has become an urgent task to solve water pollution and has received extensive attention from all over the world.
In order to solve the problem of environmental pollution, the world’s developed countries associated together at all costs to solve this problem. Through unremitting efforts, many effective means have been developed to solve the problem of water pollution (Wang et al., 2019; Wang et al., 2020a; Wang et al., 2020b; Shifa Wang et al., 2020; He et al., 2021a; Wang et al., 2021a; Cheng et al., 2021; Mateo et al., 2021; Saheed et al., 2021; Han et al., 2022; He et al., 2022), including 1) the thermal catalysis technique driven by thermal energy; 2) the electrocatalytic technology driven by electricity; 3) the photocatalysis technique driven by light energy; 4) the ultrasonic catalytic technology driven by mechanical energy; 5) the biodegradation technology, which uses microbial degradation; 6) adsorption techniques, using physical or chemical properties or diffusion; and 7) the new catalytic technology using heat, light, electricity, and other methods. Among these catalytic technologies, the photocatalytic technology is a green catalytic technology that uses sunlight to achieve degradation of organic dyes. In order to realize the effective degradation of organic dyes, the selection of a suitable photocatalyst is very important.
Among many photocatalysts, TiO2 photocatalysts have the longest research history (Fujishima and Rao, 1997). TiO2 has three phases: tetragonal, orthogonal, and monoclinic phases. It has an optical band gap of 3.2 eV, can respond to ultraviolet light, and is a good ultraviolet photocatalyst, which has a wide range of applications in the industrial degradation of organic dyes (Wu and Yu, 2004). Meanwhile, it has high antibacterial activity and also has a wide range of applications in the fields of photocatalytic and antibacterial implants (Skorb et al., 2008). However, due to its large optical band gap value, it is difficult to respond to visible light, and the visible light occupies the majority of sunlight, which limits its application in the field of photocatalysis or photocatalytic and antibacterial implants (Dalton et al., 2002). Therefore, researchers have adopted many methods to improve the photocatalytic and antibacterial activities of TiO2. 1) TiO2 photocatalysts with a special defect structure were synthesized under extreme conditions. TiO2 photocatalysts synthesized under such extreme conditions have a high specific surface area, which introduces a large number of defects and makes it have a high surface active site, thus enhancing its photocatalytic and antibacterial activities (Qu and Kroes, 2007; Li et al., 2017; Li et al., 2021a). 2) An atom with a similar radius to the Ti atom is selected for doping, and the optical band gap value of TiO2 is reduced to achieve the purpose of responding to visible light. This method allows the atom to be doped to occupy the position of the Ti atom, but not many atoms can be doped. If the synthesis method is not chosen correctly, it can easily form composites (Colón et al., 2006; Cong et al., 2007; Zaleska, 2008). 3) Special preparation technology to construct a special heterojunction structure or surface-modified TiO2 photocatalysts to enhance the photocatalytic or antibacterial activity of TiO2 photocatalysts was developed (Chen et al., 2010; Bai et al., 2015; Martins et al., 2016). This method does not change the optical band gap value of TiO2, and it combines one or more excellent physical and chemical properties of semiconductors so that the new TiO2-based photocatalyst combined together has enhanced or novel physical and chemical properties.
In this study, the preparation methods of TiO2, ion-doped TiO2, and TiO2-based composite photocatalysts are reviewed, and the effects of preparation methods on the physicochemical properties of TiO2-based photocatalysts are summarized. Meanwhile, the photocatalytic and bacteriostatic activities of TiO2, ion-doped TiO2, and TiO2-based composite photocatalysts in degradation of organic pollutants and antibacterials were reviewed. Based on the review of photocatalytic and antibacterial activities of TiO2-based photocatalysts, the photocatalytic and antibacterial mechanisms and application of TiO2-based photocatalysts in orthopedic implants were summarized. This review puts forward the future development direction of TiO2-based photocatalysts and provides corresponding technical reference for expanding the study of other photocatalysts.
2 Preparation of Micro/Nanostructured TiO2-Based Photocatalysts
The photocatalytic and bacteriostatic activities of the micro/nanostructured TiO2-based photocatalyst strongly depend on the preparation method (Nakata and Fujishima, 2012; Daghrir et al., 2013; Guo et al., 2019). TiO2-based photocatalysts synthesized by using different preparation methods may have the advantages of large specific surface area, large surface defect concentration, and high charge carrier transfer and separation efficiency, thus greatly improving the photocatalytic and antibacterial activities of the system (Zhang et al., 1998; Choi et al., 2010).
2.1 Preparation of Micro/Nanostructured TiO2 Photocatalysts
With the rapid development of technology, the preparation method of TiO2 has also been in rapid development. But, really summed up, there are only two methods: physical and chemical methods. Physical methods mainly include the solid phase sintering method, vacuum evaporation, vapor phase transfer deposition, and sputtering method. Pure TiO2 prepared by using the physical method is mainly in the form of a film, and as a photocatalyst, it has the disadvantages of having a small contact area and low photocatalytic efficiency. Chemical methods mainly include the gas-phase and liquid-phase preparation method. The commonly used gas-phase methods for the preparation of TiO2 include gas fuel combustion, gas phase oxidation, and the atmospheric microwave plasma gas-phase method. The liquid phase methods include the sol–gel method, inorganic salt hydrolysis method, solvothermal method, micro-emulsion method, hydrolysis, hydrothermal method, HCl–water volatilization-assisted precipitation method, and polyacrylamide gel method (Macwan et al., 2011; Yu et al., 2009; Fan et al., 2009). Ren et al. (2022) prepared the TiO2 nanoparticles by using the HCl–water volatilization-assisted precipitation method, which exhibit high photocatalytic activity for the degradation of methyl orange. Figure 1 shows the preparation flow chart of pure TiO2 nanoparticles prepared by using the HCl–water volatilization-assisted precipitation method. TiO2 nanoparticles prepared by using this method have a one-dimensional structure, which greatly enhances its specific surface area and improves its photocatalytic activity. The polyacrylamide gel method is also a common method used to prepare TiO2 nanoparticles. The nanoparticles prepared by this method are uniformly dispersed with almost no adhesion agglomeration and are widely used in the preparation of metal oxide semiconductor materials and multiple heterojunction composite semiconductor materials (Xian et al., 2013; Gao et al., 2021a; Gao et al., 2021b; He et al., 2021b; Li et al., 2021b; Liang et al., 2021; Tang et al., 2022a; Liu et al., 2022b; Fu et al., 2022; Gao et al., 2022; Wang et al., 2022). Lu et al. (2022) prepared the TiO2 nanotube photocatalysts, which exhibit high photocatalytic activity for the degradation of patulin in simulated juice. This special structure enables TiO2 to achieve new applications in the degradation of cold drugs.
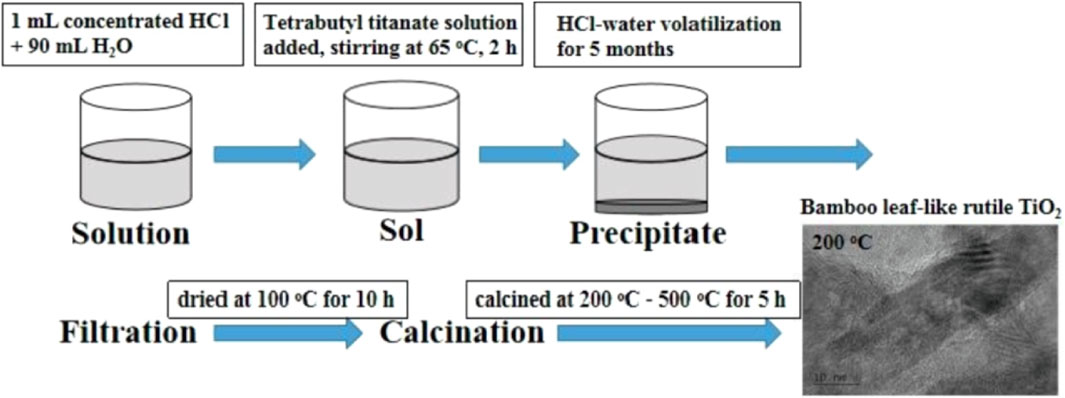
FIGURE 1. Preparation flow chart of pure TiO2 nanoparticles prepared by the HCl–water volatilization-assisted precipitation method. Adapted from Ren et al. (2022). Copyright © 2021 Elsevier B.V.
2.2 Preparation of Micro/Nanostructured Ion-Doped TiO2 Photocatalysts
Ion doping mainly includes metal ion doping and non-metal ion doping. Metal ion doping mainly introduces metal ions such as alkali metals, transition metals, and rare earth metals into the crystal structure of pure TiO2, making metal ions become the receiver pole of photogenerated electrons or the defect that can capture photogenerated electrons, thus improving the separation of the photogenerated charge carrier and correspondingly improving the photocatalytic capacity of photocatalysts. Non-metallic ion doping generally involves doping B, N, C, S, and other non-metallic elements close to O elements into TiO2 photocatalysts. It is generally believed that the hybridization of p orbital and 2p orbital of O in the non-metal leads to an upward shift in the valence band width of TiO2, which reduces the band gap width and enables TiO2 to absorb visible light.
The key to the synthesis of ion-doped TiO2 is to control the composition and content of doped ions and element substitution for the position of Ti. Researchers usually adopt physical and chemical methods to enhance the photocatalytic activity of TiO2 by doping metal ions or non-metal ions in the lattice position of Ti or modifying the surface of TiO2. Generally, it is easy to modify TiO2 with metal ions, and the photocatalytic and bacteriostatic activities of TiO2 can be greatly improved by chemical experiments. Ellouzi et al. (2021) synthesized silver-doped TiO2 nanoparticles by the glucose-assisted ball-milling method, which exhibit high photocatalytic activity for efficient photodegradation of rhodamine B. The preparation flow chart of silver-doped TiO2 nanoparticles prepared by using the glucose-assisted ball-milling method is shown in Figure 2. As can be seen from the figure, it is easier to modify Ag particles to the surface of TiO2 nanoparticles by the glucose-assisted ball-milling method, thus improving the uniformity of the entire sample particles. Venkatachalam et al. prepared Mg2+- and Ba2+-doped TiO2 nanoparticles by using the sol–gel method (Venkatachalam et al., 2007). The band gap value of nano-TiO2 doped with Mg2+ and Ba2+ is higher than that of pure nano-TiO2, but the photocatalytic activity of 4-chlorophenol degradation is higher than that of pure nano-TiO2 and commercial Degussa P25. Hu et al. (2022) synthesized Ni-doped TiO2 nanotubes by using the anodic oxidation method, which exhibit high electrocatalytic activity for the degradation of phenol wastewater. Zhou et al. (2019) prepared a photocatalyst doped with six different types of rare earth (RE) ions (La, Ce, Pr, Nd, Eu, or Gd) by using the microwave hydrothermal treatment method. The results showed that the structure and chemical properties of nanocomposites are related to the radius of Re3+. Since the radius of Re3+ is much larger than that of Ti4+, Ti4+ can replace Re3+ in the lattice of Re2O3 in the form of Ti3+, and it produces charge imbalance due to its small ionic radius.
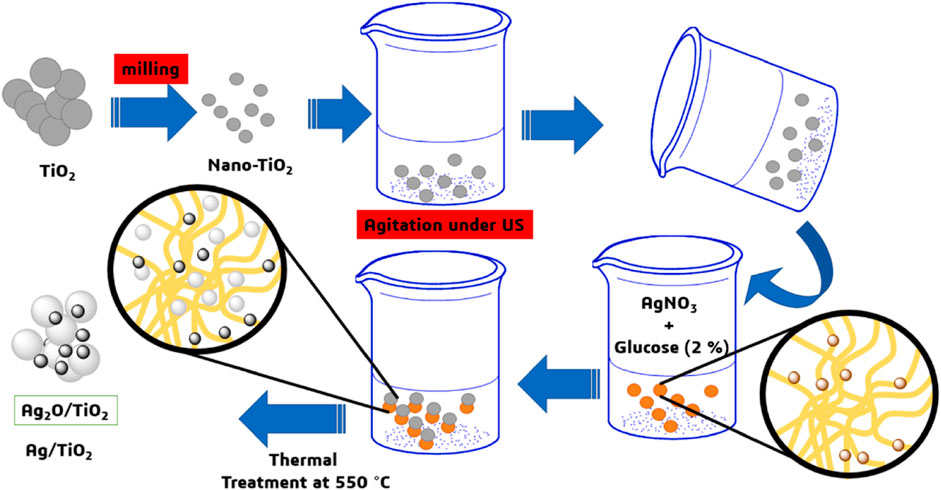
FIGURE 2. Preparation flow chart of silver-doped TiO2 nanoparticles prepared by the glucose-assisted ball-milling method. Adapted from Ellouzi et al. (2021). Copyright © 2021 Elsevier B.V.
Boningari et al. (2018) successfully prepared N-doped TiO2 by using a new single-step flame spraying method. The results showed that the N atom is effectively doped into the crystal structure of TiO2. The combination of the N atom and TiO2 crystal structure changed the electronic band structure of TiO2, formed a new middle gap energy state N 2p band in the O 2p valence band, reduced the band gap width of TiO2, and transferred the optical absorption of TiO2 to the visible region. The results showed that the N-doped TiO2 improved TiO2’s solar energy utilization rate. Zhang et al. (2015) continuously deposited TiO2 using monodispersed cationic polystyrene microspheres as templates by using the directional self-assembly method and then removed cationic polystyrene microspheres by calcination at 450°C to obtain C-doped hollow TiO2. Under visible light irradiation, C-doped hollow anatase TiO2 has better photocatalytic activity than commercial P25.
2.3 Preparation of Micro/Nanostructured TiO2-Based Composite Photocatalysts
The TiO2-based composite photocatalysts mainly show two states: one is the formation of heterojunction, and the other is mixed together in a simple way. Semiconductor heterostructures are usually constructed by coupling two or more semiconductors with different band gap widths to widen the light response range of wide-gap semiconductors and improve the separation efficiency of photogenerated electron hole pairs. If two or more semiconductor materials are simply combined, a simple one-step synthesis can be performed by many chemical processes. When two or more semiconductor materials are combined in a heterojunction, special preparation methods are required to couple them together. Some special morphologies, such as the hierarchical structure and core-shell structure, were constructed to promote the separation of photogenerated electron holes from the built-in electric field, thus improving the photocatalytic efficiency of the photocatalyst.
There are many methods for heterostructure construction, including the sol–gel method, electrostatic spinning method, hydrothermal method, solvothermal method, spin-coating method, and facile solid–liquid adsorption template technology, followed by calcination process. Table 1 shows the preparation method of micro/nanostructured TiO2-based composite photocatalysts. Based on the different uses of TiO2 composite photocatalyst, different methods can be used to regulate and synthesize heterojunction composites with different morphologies. Wang et al. (2021b) prepared SiO2-TiO2@PDMS by the spray method, which exhibited excellent repellent effect toward organic dye droplets and good mechanical stability. Xu et al. (2022) synthesized the core-shell C@TiO2 microspheres by using a three-step method, which exhibit high electrochemical property. Figure 3 shows the preparation flow chart of core-shell C@TiO2 microspheres prepared by using a three-step method. Uniform core-shell C@TiO2 microspheres can be obtained by this method. However, in order to increase the photocatalytic or bacteriostatic activity of TiO2-based composite photocatalysts, defects, impurity levels, and transport carrier of charge carriers should be introduced into the composite. As the companion of TiO2 heterojunction, the selection of the other half plays a crucial role in the photocatalytic and antibacterial activities of TiO2 catalysts. Improper selection of another semiconductor may result in the reduced photocatalytic or bacteriostatic activity of the entire system. Therefore, the preparation method is only one of the important factors affecting the photocatalytic and antibacterial activities of TiO2-based composite photocatalysts.
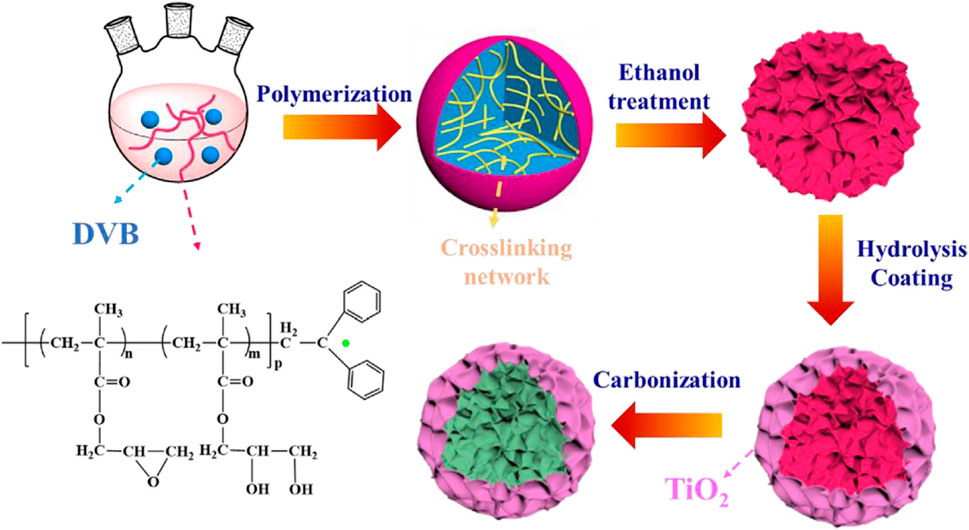
FIGURE 3. Preparation flow chart of core-shell C@TiO2 microspheres prepared by a three-step method. Adapted from Xu et al. (2022). Copyright © 2020 Elsevier Ltd.
3 Photocatalytic Activity of Micro/Nanostructured TiO2-Based Photocatalysts
3.1 Photocatalytic Activity of Micro/Nanostructured TiO2 Photocatalysts
The photocatalytic activity of TiO2 strongly depends on its preparation method. TiO2 with different morphologies can be obtained by using different preparation methods, including nanoparticles, nanoflowers, nanosheets, nanoribbons, microspheres, and nanotrees. For pure phase TiO2, it can only respond to ultraviolet light and can degrade different kinds of dyes, drugs, and some pollutants that are difficult to degrade. Due to the small composition of ultraviolet light in natural light, this greatly limits the application of TiO2 photocatalyst in the field of photocatalysis. The recombination rate of photogenerated carriers in TiO2 is much higher than its mobility, which makes the photogenerated carriers to migrate to the surface of TiO2 and participate in very few photocatalytic reactions. At present, commonly used TiO2 photocatalysts are mainly powdered nanoparticles, which makes it difficult to separate, recycle, and reuse from liquid after the photocatalytic reaction. On the one hand, it causes waste of photocatalysts; on the other hand, it is easy to cause secondary pollution, which limits its large-scale use. However, as a catalyst for industrial use, TiO2 has contributed a lot.
For pure phase TiO2 catalysts, many researchers used special preparation methods to construct defects or special morphology to enable TiO2 to respond to visible light, thus expanding the application of TiO2 photocatalyst in the field of photocatalysis. Xie et al. (2021) synthesized the TiO2 nanobelts by a molten salt method with the NaBH4 or H2/Ar atmosphere and exhibits high photocatalytic activity. Figure 4 shows the SEM images of TiO2 nanobelts, TiO2 nanobelts calcined at 400°C for 3 h in air, TiO2 nanobelts with 30 mg NaBH4, and TiO2 nanobelts annealed at 400°C for 3 h under a H2/Ar atmosphere. TiO2 nanobelts have different concentrations of oxygen vacancies and special electronic structures through different treatment methods, thus enhancing the transfer and separation efficiency of electrons and holes in TiO2 and finally achieving the purpose of enhancing the photocatalytic activity of TiO2 nanoribbons. Therefore, a variety of extreme conditions are used to construct TiO2 surface defects, regulate the electronic structure of TiO2, and enable it to have high oxidation or reduction capacity, thus achieving the purpose of degrading dyes. However, this approach often has the disadvantages of high equipment requirements, high cost, and unsafe, and the concentration of surface defects cannot be accurately controlled, which further limits the development of this technology. Kaushik et al. (2019) transformed 2D TiO2 to mesoporous hollow 3D TiO2 spheres by using the solvothermal strategy followed by thermal treatment, which exhibit high photocatalytic and antibacterial activities. This strategy provides a new idea for the follow-up study of TiO2.
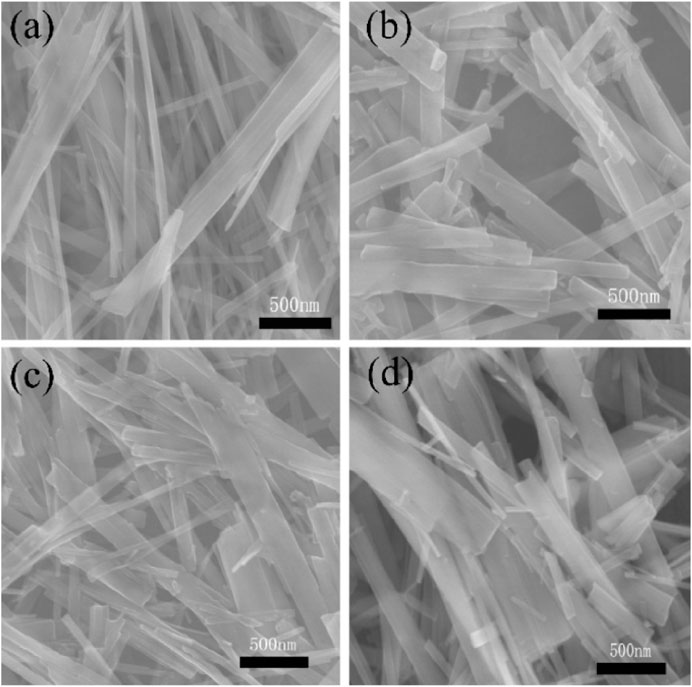
FIGURE 4. SEM images of (A) TiO2 nanobelts, (B) TiO2 nanobelts calcined at 400°C for 3 h in air, (C) TiO2 nanobelts with 30 mg NaBH4, and (D) TiO2 nanobelts annealed at 400°C for 3 h under a H2/Ar atmosphere. Adapted from Xie et al. (2021). Copyright © 2021 Capital Normal University. Published by Elsevier B.V.
3.2 Photocatalytic Activity of Micro/Nanostructured Ion-Doped TiO2 Photocatalysts
Ion doping is one of the effective ways to enhance the photocatalytic activity of the TiO2 photocatalyst. The optical band gap value of TiO2 can be effectively improved by doping different ions. The reduction of its optical band gap value enables it to respond to visible light, expanding its application in the field of photocatalysis. The ultimate goal is to prevent the recombination of electron–hole pairs inside TiO2 so that electrons react with dyes in its conduction band, and the holes in the valence band react with dyes to generate non-toxic and harmless products. Ion doping is divided into metal doping and non-metal doping, and metal doping is mainly performed by doping rare earth ions and transition metal ions (Santos et al., 2022). As for noble metal ions, they are easy to exist in the elemental form, so TiO2 is mostly modified on the surface of noble metal particles to enhance the photocatalytic activity of TiO2 (Phromma et al., 2022). The photocatalytic activity of ion-doped TiO2 is also affected by the surface morphology, oxygen vacancy, electronic structure, and optical band gap value.
Unlike metal ion doping, non-metal ion–doped TiO2 can be sintered at high temperature to remove non-metal ions. Therefore, it is necessary to control the appropriate sintering temperature to keep non-metal ions. This will bring a new problem: the content of non-metallic ions cannot be controlled. However, non-metallic ion doping does solve the shortcoming that TiO2 cannot respond to visible light, which enables it to have high visible light photocatalytic activity. Zhu et al. (2022b) reported that the C-modified and N-doped TiO2 photocatalysts prepared by the hydrothermal method using tetrabutyl titanate (TBOT) and feathers of chicken as the precursor exhibit high photocatalytic activity for the degradation of tetracycline hydrochloride under visible light irradiation. Figure 5 shows the photocatalytic mechanism diagram of C-modified and N-doped TiO2 photocatalysts. Carbon acts as a carrier of charge carriers in the whole system, separating photogenerated electrons and holes in space. Nitrogen doping can make TiO2 respond to visible light.
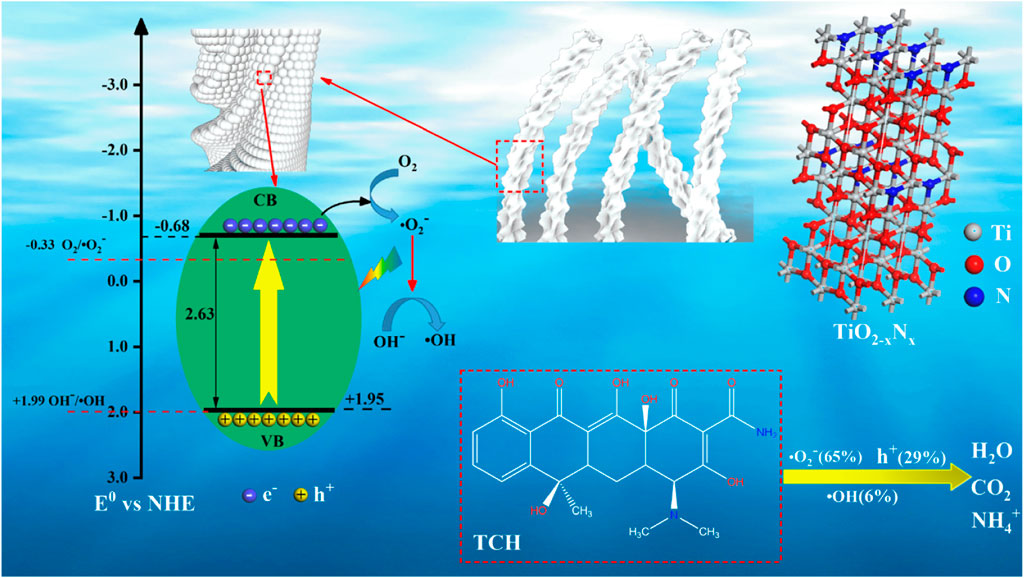
FIGURE 5. Photocatalytic mechanism diagram of C-modified and N-doped TiO2 photocatalysts. Adapted from Zhu et al. (2022b). Copyright © 2022 Elsevier B.V.
3.3 Photocatalytic Activity of Micro/Nanostructured TiO2-Based Composite Photocatalysts
TiO2 composite photocatalysts can overcome the shortcoming of uncontrollable doping content of non-metal ions. Combining the advantages of two or more kinds of semiconductor materials, the whole system has physical and chemical properties that a single component does not have at the same time, which is the key to the design of TiO2-based composite photocatalysts (Perales-Martínez et al., 2015; Hao et al., 2016; Sotelo-Vazquez et al., 2017). This design method will not only change the optical band gap value of TiO2 but also will introduce surface defects or oxygen vacancies at the interface of the two semiconductors. Therefore, the study of the interface characteristics of two or more semiconductors is conducive to insight into the photocatalytic mechanism of TiO2-based complex photocatalyst. In addition to metal oxides that can form heterojunctions with TiO2, some polymers and sulfides can also form heterojunctions with TiO2 in special ways to enhance the photocatalytic activity of TiO2 (Vorontsov et al., 2001; Liao et al., 2010; Antoniadou et al., 2011; Riaz et al., 2015). Therefore, there are many kinds of TiO2-based photocatalysts, but the ultimate goal is to make TiO2 exhibit high visible light photocatalytic activity or show new physical and chemical properties.
To summarize, there are four main ways to form a heterojunction (Schuettfort et al., 2009; Low et al., 2017; Guo et al., 2021): one is that the conduction band of a semiconductor is located in the conduction band and valence band of another semiconductor. The other is that the conduction band and valence band of one kind of semiconductor are located in the interior of another kind of semiconductor. Third, the conduction band and valence band of the two kinds of semiconductor are completely separated. Fourth, there is no conduction band and valence band between metal particles and semiconductor heterojunction. Tang et al. (2022b) reported that the b-TiO2 @MoS2 photocatalysts synthesized by an ultrasound technique coupled with the sol–gel method at low temperature exhibit high visible light photocatalytic activity. A photocatalytic mechanism diagram of b-TiO2 @MoS2 photocatalysts is shown in Figure 6. As can be seen from the figure, the valence band of MoS2 is located inside the conduction band and valence band of TiO2. Such a structure facilitates the relaxation of electrons from the more negative conduction band of MoS2 to the conduction band of TiO2, and the transition of holes from the more positive valence band of TiO2 to the valence band of MoS2, thus promoting the separation of electrons and holes. At present, the construction of special heterojunction TiO2-based composite photocatalysts has become the most popular effective way to enhance the photocatalytic activity of TiO2 photocatalysts.
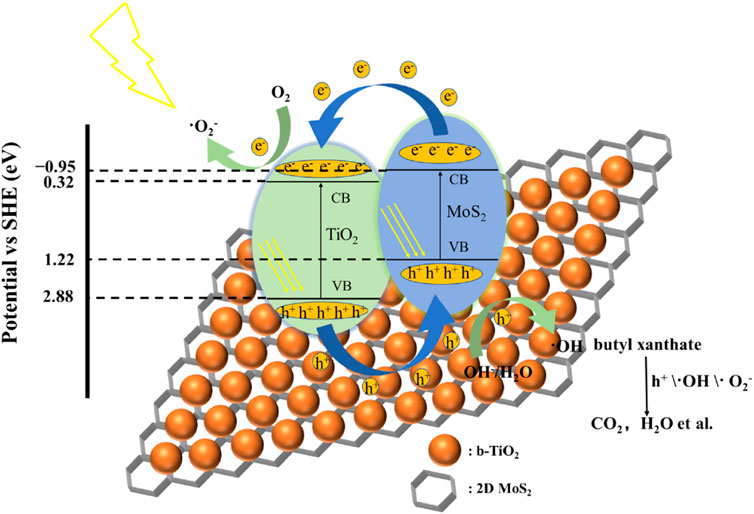
FIGURE 6. Photocatalytic mechanism diagram of b-TiO2 @MoS2 photocatalysts. Adapted from Tang et al. (2022b). Copyright © 2022 Elsevier B.V.
4 Antibacterial Activity of Micro/Nanostructured TiO2-Based Photocatalysts
4.1 Antibacterial Activity of Micro/Nanostructured TiO2 Photocatalysts
The high antibacterial properties of orthopedic implants are an effective way to avoid infection during surgery, which will reduce the incidence of amputation or death due to infection. Generally, antibacterial materials can be divided into natural antibacterial materials, organic antibacterial materials, and inorganic antibacterial materials (Li et al., 2020). Inorganic antibacterial materials can be divided into two categories: one is the prepared antibacterial materials containing copper, silver, zinc, and other metal antibacterial ions; the second is photocatalytic antibacterial materials represented by TiO2; such inorganic antibacterial materials can play an antibacterial role under the condition of ultraviolet light irradiation, water, or oxygen (Chen et al., 2017; Wang et al., 2020c). TiO2 is a widely studied orthopedic implant material with high photocatalytic antibacterial activity. Titanium dioxide has become the most common photocatalytic antibacterial material because of its low toxicity, high safety, and no irritation to skin. Silver antibacterial materials take about 24 h to exert the antibacterial effect, while the oxide ingots only take about an hour. Moreover, the antibacterial effect of the dioxide ingot is carried out through photocatalysis, and it itself will not be consumed in the antibacterial process so that the antibacterial material of dioxide ingot has a more lasting antibacterial performance.
The antibacterial mechanism of titanium dioxide is similar to its photocatalytic mechanism, and the resulting hydroxyl radicals can react with biological macromolecules, damage the structure of biological cells, and then lead to cell death. Figure 7 shows the photocatalytic bactericidal process of TiO2 photocatalysts. Bacteria eventually mineralize H2O, CO2, and other small molecular organics under the action of TiO2 (Ge et al., 2019). Similarly, the antibacterial activity of TiO2 is affected by its morphology, surface defects, oxygen vacancy concentration, and electronic structure (Mazare et al., 2016). At the same time, the crystal type of titanium dioxide also has a great influence on its antibacterial activity. TiO2 has high antibacterial activity under ultraviolet light irradiation, but TiO2 is powerless under visible light irradiation due to the limitation of its own optical band gap value. Therefore, researchers are committed to improving the photocatalytic antibacterial mechanism of TiO2 through ion doping and heterostructure construction.
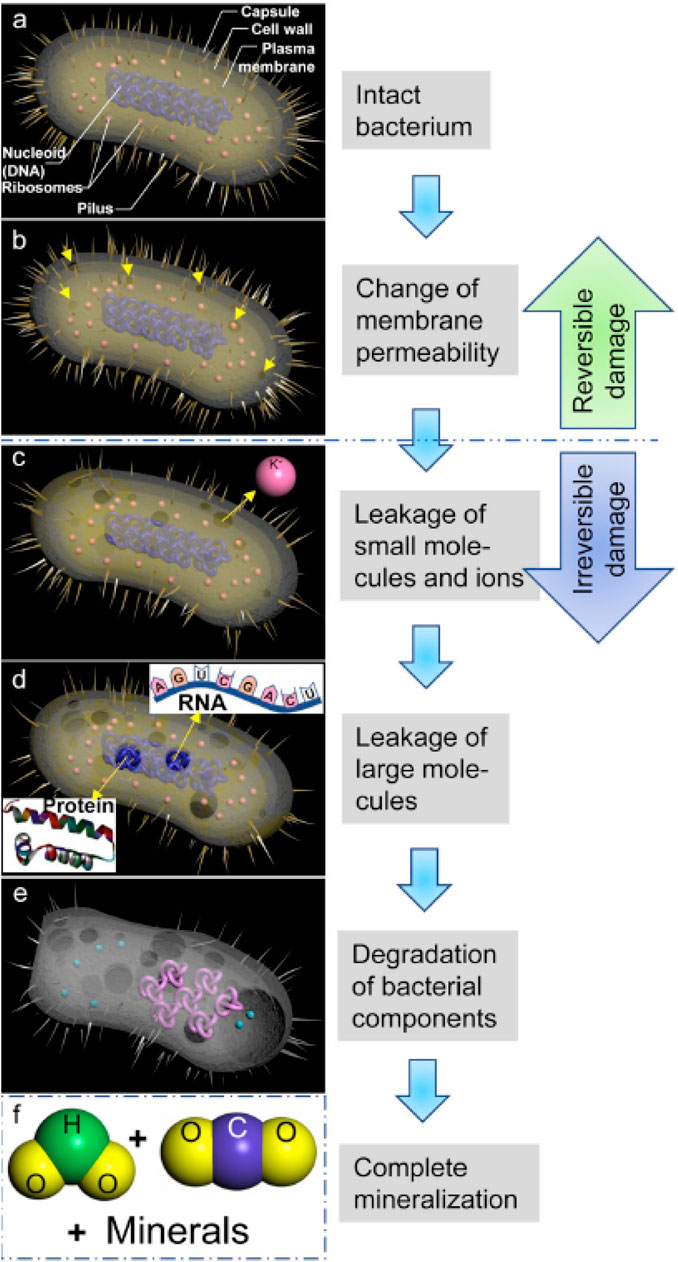
FIGURE 7. Photocatalytic bactericidal process of TiO2 photocatalysts. Adapted from Ge et al. (2019). Copyright © 2020 Production and hosting by Elsevier B.V. (A) An intact bacterium; (B) Bacterial oxidized by TiO2 photocatalysis; (C) The bacterium leak small molecules and ions; (D) Leakage of higher molecular weight components; (E) Degradation of bacterial internal components; (F) The bacterium was completely mineralized.
4.2 Antibacterial Activity of Micro/Nanostructured Ion-Doped TiO2 Photocatalysts
In the process of using the antibacterial activity of titanium dioxide, the photoresponse range of titanium dioxide is narrow, and the photoelectron–hole pair is easy to compound. To solve such problems, TiO2 photocatalytic materials can be modified structurally or on the surface so as to increase the light response range. This modification process can also reduce the recombination probability of carriers and improve the quantum efficiency, thus enhancing the photocatalytic bacteriostatic effect of TiO2. Recently, the application of TiO2 in the field of antibacterial materials has achieved remarkable results. With the continuous development and utilization of antibacterial performance of the TiO2 photocatalyst, glass, ceramics, metal products, coatings, fibers, plastics, and other antibacterial products can be produced by plating on the surface of materials or doping in other materials (Mori, 2005; Tung and Daoud, 2011).
Ion-doped TiO2 photocatalysts have been widely used in the bacteriostatic field. Similar to the photocatalytic activity of the TiO2 photocatalyst, ion-doped TiO2 can be divided into metal ion doping and non-metal ion doping when studying the antibacterial activity of ion-doped TiO2. Zhang et al. (2020) reported that the Y-doped TiO2 photocatalysts prepared by the plasma electrolytic oxidation method exhibit high bacteriostatic activity. Figure 8 shows the preparation flow chart and antibacterial mechanism of Y-doped TiO2 photocatalysts. The result shows that the Y-doped TiO2 photocatalysts possess excellent bacteriostatic activity against Staphylococcus aureus and Escherichia coli and outstanding biocompatibility and antibacterial capacity. Yadav et al. (2014) prepared nickel-doped TiO2 nanoparticles by using the sol–gel method, which show the high photocatalytic inactivation and lower recombination rate of photogenerated charge carriers. Similarly, non-metallic doping can also greatly improve the antibacterial activity of TiO2. Cao et al. (2014) reported that the N-doped TiO2 thin films coated on stainless steel brackets exhibit high antibacterial activity against Lactobacillus acidophilus and Candida albicans.
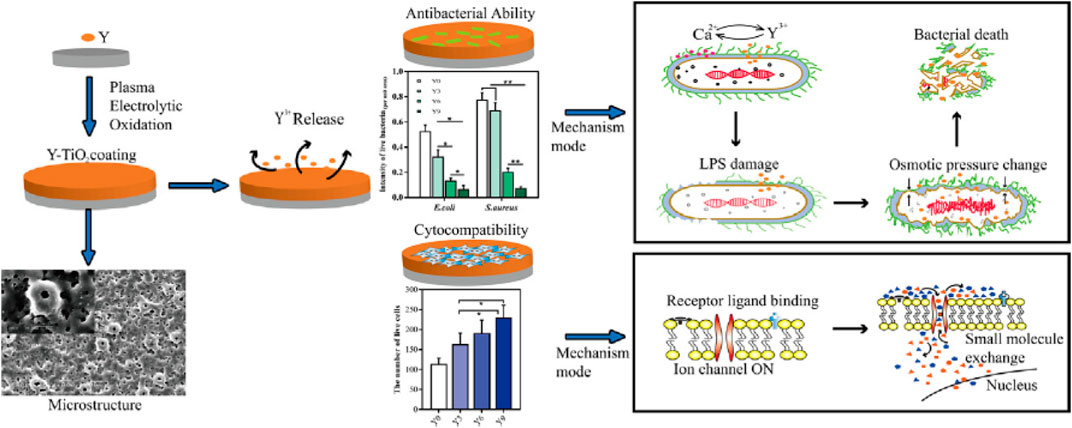
FIGURE 8. Preparation flow chart and antibacterial mechanism of Y-doped TiO2 photocatalysts. Adapted from Zhang et al. (2020). Copyright © 2020 Published by Elsevier Ltd.
4.3 Antibacterial Activity of Micro/Nanostructured TiO2-Based Composite Photocatalysts
The construction of heterojunction will greatly improve the surface oxygen vacancy concentration and interface characteristics of TiO2 so that it has a high antibacterial activity and a potential application prospect in orthopedic implants. Similar to the study on the photocatalytic activity of TiO2, its antibacterial activity has received unprecedented attention. The construction of heterojunction can form microspheres, mesoporous structures, layered structures, nanotubes, nanosheets, nanoribbons, nanotrees, and various flower-like structures (Wu et al., 2014; Lou et al., 2019; Murthy, 2019). These special morphologies will make TiO2-based composite photocatalysts have high antibacterial activity. Combining the advantages of a variety of semiconductors will expand the application of TiO2 in different fields, which is the absolute advantage of heterogeneous structure construction (Ma et al., 2015). Therefore, TiO2-based heterojunction composite photocatalysts are widely favored by researchers.
Gnanasekaran et al. (2021) reported that p-n junction TiO2/CuO heterojunction composite photocatalysts synthesized by the sol–gel method, followed by chemical precipitation methods, exhibit high photocatalytic and antibacterial activities. Figure 9A shows the antibacterial activity of p-n junction TiO2/CuO heterojunction composite photocatalysts. The results indicate that the p-n junction TiO2/CuO heterojunction composite photocatalysts possess high bacteriostatic activity. The antibacterial mechanism of the p-n junction TiO2/CuO heterojunction composite photocatalysts is shown in Figure 9B. The conduction band and valence band of CuO are completely located inside TiO2. However, due to the spatial separation of charge carriers, CuO and CuO have high transfer and separation efficiency, thus enhancing the antibacterial activity of TiO2. At present, researchers focus on how to apply the prepared TiO2-based heterojunction composite photocatalysts to orthopedic implants, hoping to move the research of TiO2 from laboratory to practical application.
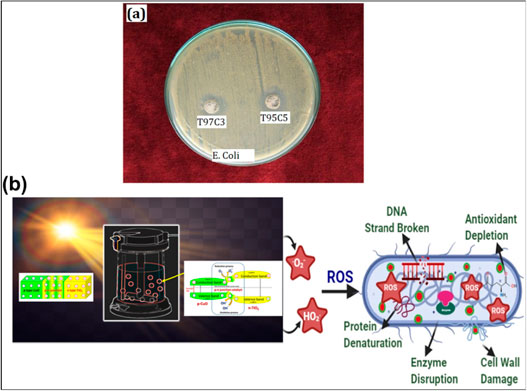
FIGURE 9. (A) Antibacterial activity and (B) antibacterial mechanism of the p-n junction TiO2/CuO heterojunction composite photocatalysts. Adapted from Gnanasekaran et al. (2021). Copyright © 2021 Elsevier Ltd.
5 TiO2-Based Photocatalysts for Application in Orthopedic Implants
5.1 TiO2 Photocatalysts for Application in Orthopedic Implants
Biomaterials implanted in the body will induce a series of reactions, including postoperative infection, inflammatory reaction (acute inflammatory reaction and chronic inflammatory reaction), and fibrous tissue hyperplasia, which can prolong the treatment period and increase the treatment cost of patients at one level or lead to amputation or even death of patients due to infection (Anderson, 1993; Lin et al., 2014; Zhang et al., 2021). The mechanism of infection around orthopedic implants is that bacteria adhere to the implant surface and form biofilms. Deposition, adhesion, and growth are three main processes of biofilm formation. Therefore, orthopedic implants need to have high biocompatibility and bacteriostatic activity. TiO2 has low toxicity, high biocompatibility, and high bacteriostatic activity, so it can be used as orthopedic implants in the medical field.
Blendinger et al. (2021) deposited TiO2 thin films on the orthopedic implant material polyetheretherketone (PEEK) by plasma enhanced atomic layer deposition, which exhibit high biocompatibility and osteogenic properties. Figure 10 shows the deposition mechanism and SEM images of TiO2 thin films. This method provides a new idea for studying the biocompatibility and bacteriostatic properties of TiO2 orthopedic implants. Antibiotics such as vancomycin, gentamicin, amoxicillin, and berberine were used in the study of pit bacteria coating on the titanium implant surface, among which gentamicin and vancomycin were the most commonly used. Popat et al. (2007) prepared TiO2 nanotubes by using the anodic oxidation method and then loaded gentamicin into TiO2 nanotubes. Experimental results showed that the gentamicin-loaded TiO2 nanotubes could inhibit bacterial reproduction with good biocompatibility, but the release of antibiotics was faster. Due to the limitation of physical and chemical properties of pure TiO2, its application in orthopedic implants is limited. In order to break through this limitation, ion doping and heterostructure construction are necessary effective methods to expand the application of TiO2 in orthopedic implants.
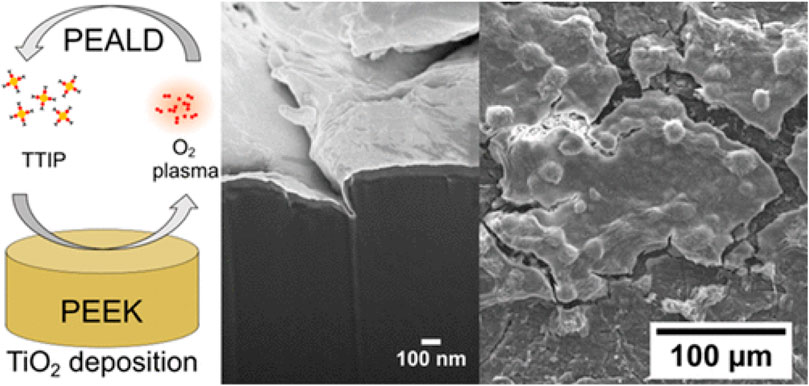
FIGURE 10. Deposition mechanism and SEM images of TiO2 thin films. Adapted from Blendinger et al. (2021). Copyright © 2021 The Authors. Published by the American Chemical Society.
5.2 Ion-Doped TiO2 Photocatalysts for Application in Orthopedic Implants
In antibacterial agents, silver antibacterial agents have a wide range of antibacterial ability; can fight against Gram-negative bacteria, fungi, and even viruses; have low cytotoxicity, good stability, and low effective concentration; and are not easy to produce drug resistance and other advantages. Compared with eukaryotic cells, silver has greater toxicity to prokaryotic cells, so silver has excellent inhibition of microbial growth and proliferation and low cytotoxicity to biological somatic cells. Nanoscale silver particles have a high specific surface area, high contact probability with bacteria, and strong chemical activity. Atoms on the surface of silver particles are easy to bond with other chemical groups, and their antibacterial effect is much stronger than that of micron silver particles. Therefore, Ag-doped TiO2 can improve the antibacterial activity and biocompatibility of TiO2, which can be used in orthopedic implants. Zhao et al. (2013) used the anodic oxidation method and hydrothermal method in titanium implant surface preparation of Ag- and Sr-doped TiO2 nanotubes, by changing the process parameters for different structures of TiO2 nanotubes, as well as the precursor concentration change of Ag and Sr, and antibacterial performance and characterization of the biological activity of TiO2 was improved. According to the results, TiO2 coatings doped with Ag and Sr have good antibacterial activity and cytocompatibility. Amin Yavari et al. (2016) prepared TiO2 nanotubes on the surface of 3D-printed porous titanium, then loaded the nanotubes with Ag nanoparticles, obtained a composite coating loaded with silver antibacterial factors on the surface of porous titanium, and studied the killing ability of silver ions on Staphylococcus aureus.
Lv et al. (2021) reported that the Zn-modified TiO2 photocatalysts prepared by the micro-arc oxidation technique exhibit high cytocompatibility and bactericidal ability for orthopedic implants. Figure 11 shows the pathological photographs of Zn-modified TiO2 photocatalysts. The result indicates that the Zn-modified TiO2 coating produced by the micro-arc oxidation technique benefits the application in the orthopedic implanted devices. Due to technical limitations, ion-doped TiO2 is rarely used in orthopedic implants. At the same time, this is due to the fact that simple particles in the air are easy to be oxidized or easy to dissolve in water solution and release metal ions, resulting in high concentration of silver ions and fine toxicity. The aforementioned problems also occur when the elemental particles are combined or doped with TiO2, thus limiting the application of ion-doped TiO2 photocatalysts in orthopedic implants.
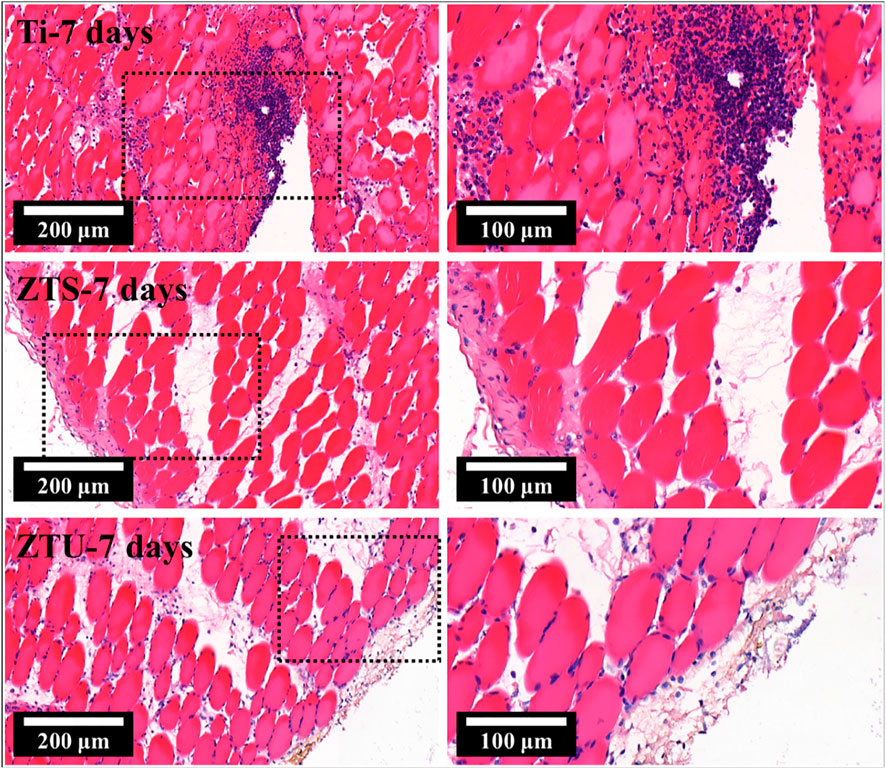
FIGURE 11. Pathological photographs of Zn-modified TiO2 photocatalysts. Adapted from Lv et al. (2021). Copyright © 2021 Elsevier B.V.
5.3 TiO2-Based Composite Photocatalysts for Application in Orthopedic Implants
In order to overcome two or many kinds of antibacterial materials used alone, materials with excellent antibacterial properties, by putting other excellent antibacterial materials and two titanic oxide composite photocatalytic antibacterial materials, not only make it photocatalytic and antibacterial under strong light conditions but also in the dark under the condition of using other antibacterial effects of antibacterial materials (Etacheri et al., 2013; Sood et al., 2016; Mousa et al., 2021). The synergistic antibacterial effect of inorganic antibacterial materials was obtained. On the other hand, compared with traditional linear, blocky, spherical and tubular materials, multistage structural materials have better antibacterial effect because of their close contact with cells. The construction of multiple heterojunction TiO2 composite photocatalysts can be effectively applied in the field of orthopedic implants.
Vandana et al. (2021) reported that the TiO2-Nb2O5 nanoporous mixed metal oxide bone implant materials were assessed by using in vitro and in vivo methods and exhibit high biocompatibility. The result indicates that the TiO2-Nb2O5 nanoporous mixed metal oxide bone implant materials are non-cytotoxic, non-hemolytic, and biocompatible. Figure 12 shows the orthopedic applications for the TiO2-Nb2O5 nanoporous mixed metal oxide bone implant materials. The results suggesting that the TiO2-Nb2O5 nanoporous mixed metal oxide bone implant materials can be used safely for orthopedic applications. A variety of heterojunction composite TiO2-based photocatalysts are widely used in the field of orthopedic implants. Therefore, its research is a very popular topic but also a fruitful topic. With the development of science and technology, the application of TiO2-based composite photocatalysts in orthopedic implants will develop rapidly.
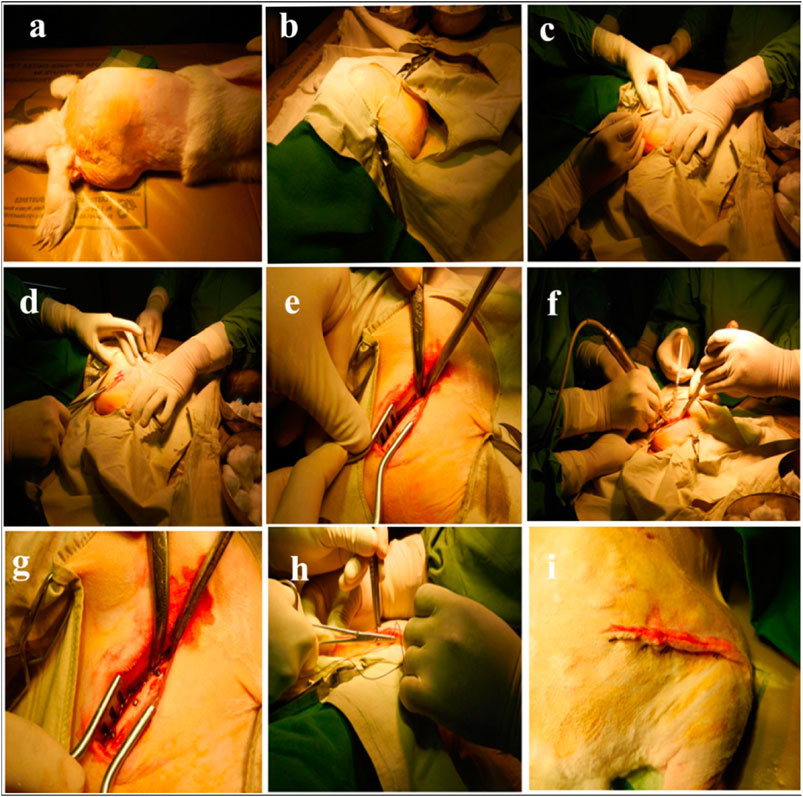
FIGURE 12. Orthopedic applications for the TiO2-Nb2O5 nanoporous mixed metal oxide bone implant materials. Adapted from Vandana et al. (2021). Copyright © 2020 Elsevier B.V. (A, B) Animals that lie on their side, (C, D) The exposed femoral cortex, (E) Three holes spaced 1 cm apart, (F, G) Material implanted, (H, I) Suture with sterile stitches.
6 Conclusion and Outlook
6.1 Conclusion
TiO2 with three crystal structures has the characteristics of non-toxic and excellent physical and chemical properties and has been applied in many fields. Due to the large optical band gap value of TiO2 and the influence of its particle size, crystal structure, and crystal defect on the photocatalytic and antibacterial activities of TiO2, research of single-component TiO2 in photocatalytic and medical fields is greatly limited. Therefore, using special means to develop metal ion–doped and non-metal ion–doped TiO2 photocatalysts can expand the light response range of TiO2 so that TiO2 has an important application in the field of photocatalysis and bacteriostatic activities. Meanwhile, the development of heterojunction TiO2-based composite photocatalysts can overcome the shortcomings of non-metal ion–doped TiO2 and obtain novel physical and chemical properties so that the heterojunction TiO2-based composite photocatalyst has broad application prospects in a variety of fields. It is worth noting that TiO2, ion-doped TiO2, and heterojunction TiO2-based composite photocatalysts have low toxicity, high biocompatibility, high photocatalytic activity, and antibacterial activity, enabling them with unprecedented applications in orthopedic implants. Although TiO2 and TiO2-based photocatalysts have been widely used in the fields of photocatalysis and antibacterial and orthopedic implants, related technologies are still developing, which makes them have potential application prospects in many fields.
6.2 Outlook
The shortcomings of TiO2 and TiO2-based photocatalysts are being overcome by researchers, which have been applied in various fields. Nevertheless, there are still many topics worthy of study in the fields of photocatalysis, bacteriostasis, and orthopedic implants. 1) With the continuous development of science and technology, people are not only satisfied with the use of TiO2 and TiO2-based photocatalysts to degrade organic dyes but also toward more complex fields such as insoluble and difficult-to-degrade pollutants and drug degradation. 2) To develop special preparation methods to synthesize TiO2 and TiO2-based photocatalysts with a special defective structure and improve the application of the catalyst in the fields of photocatalysis, bacteriostasis, and orthopedic implants. 3) The TiO2-based photocatalyst combines excellent optical, magnetic, mechanical, and electrical properties of semiconductor materials and makes the properties of the system with special optical, magnetic, mechanical, or electrical properties and the internal connection mechanism of photocatalytic and bacteriostatic activities and aforementioned properties a topic worth studying. 4) The combination of ion-doped TiO2 and heterojunction TiO2-based composite photocatalysts will produce new physical and chemical properties, which is also a topic worthy of study.
Author Contributions
All authors listed have made a substantial, direct, and intellectual contribution to the work and approved it for publication.
Conflict of Interest
The authors declare that the research was conducted in the absence of any commercial or financial relationships that could be construed as a potential conflict of interest.
Publisher’s Note
All claims expressed in this article are solely those of the authors and do not necessarily represent those of their affiliated organizations, or those of the publisher, the editors, and the reviewers. Any product that may be evaluated in this article, or claim that may be made by its manufacturer, is not guaranteed or endorsed by the publisher.
References
Abrinaei, F., and Aghabeygi, S. (2022). Optimization on Preparation Conditions to Improve the Nonlinear Optical Response of ZnO/TiO2/ZrO2 Ternary Nanocomposites under Continuous-Wave Laser Irradiation. Optik 255, 168720. doi:10.1016/j.ijleo.2022.168720
Amin Yavari, S., Loozen, L., Paganelli, F. L., Bakhshandeh, S., Lietaert, K., Groot, J. A., et al. (2016). Antibacterial Behavior of Additively Manufactured Porous Titanium with Nanotubular Surfaces Releasing Silver Ions. ACS Appl. Mat. Interfaces 8 (27), 17080–17089. doi:10.1021/acsami.6b03152
Anderson, J. M. (1993). Chapter 4 Mechanisms of Inflammation and Infection with Implanted Devices. Cardiovasc. Pathol. 2 (3), 33–41. doi:10.1016/1054-8807(93)90045-4
Antoniadou, M., Daskalaki, V. M., Balis, N., Kondarides, D. I., Kordulis, C., and Lianos, P. (2011). Photocatalysis and Photoelectrocatalysis Using (CdS-ZnS)/TiO2 Combined Photocatalysts. Appl. Catal. B Environ. 107 (1-2), 188–196. doi:10.1016/j.apcatb.2011.07.013
Bai, S., Liu, H., Sun, J., Tian, Y., Chen, S., Song, J., et al. (2015). Improvement of TiO2 Photocatalytic Properties under Visible Light by WO3/TiO2 and MoO3/TiO2 Composites. Appl. Surf. Sci. 338, 61–68. doi:10.1016/j.apsusc.2015.02.103
Blendinger, F., Seitz, D., Ottenschläger, A., Fleischer, M., and Bucher, V. (2021). Atomic Layer Deposition of Bioactive TiO2 Thin Films on Polyetheretherketone for Orthopedic Implants. ACS Appl. Mat. Interfaces 13 (3), 3536–3546. doi:10.1021/acsami.0c17990
Boningari, T., Inturi, S. N. R., Suidan, M., and Smirniotis, P. G. (2018). Novel One-step Synthesis of Nitrogen-Doped TiO2 by Flame Aerosol Technique for Visible-Light Photocatalysis: Effect of Synthesis Parameters and Secondary Nitrogen (N) Source. Chem. Eng. J. 350, 324–334. doi:10.1016/j.cej.2018.05.122
Cao, S., Liu, B., Fan, L., Yue, Z., Liu, B., and Cao, B. (2014). Highly Antibacterial Activity of N-Doped TiO2 Thin Films Coated on Stainless Steel Brackets under Visible Light Irradiation. Appl. Surf. Sci. 309, 119–127. doi:10.1016/j.apsusc.2014.04.198
Chen, C., Cai, W., Long, M., Zhou, B., Wu, Y., Wu, D., et al. (2010). Synthesis of Visible-Light Responsive Graphene Oxide/TiO2 Composites with P/n Heterojunction. ACS Nano 4 (11), 6425–6432. doi:10.1021/nn102130m
Chen, R., Han, Z., Huang, Z., Karki, J., Wang, C., Zhu, B., et al. (2017). Antibacterial Activity, Cytotoxicity and Mechanical Behavior of Nano-Enhanced Denture Base Resin with Different Kinds of Inorganic Antibacterial Agents. Dent. Mat. J. 36 (6), 693–699. doi:10.4012/dmj.2016-301
Cheng, L., Huang, D., Zhang, Y., and Wu, Y. (2021). Preparation and Piezoelectric Catalytic Performance of HT-Bi2MoO6 Microspheres for Dye Degradation. Adv. Powder Technol. 32 (9), 3346–3354. doi:10.1016/j.apt.2021.07.021
Choi, S. K., Kim, S., Lim, S. K., and Park, H. (2010). Photocatalytic Comparison of TiO2 Nanoparticles and Electrospun TiO2 Nanofibers: Effects of Mesoporosity and Interparticle Charge Transfer. J. Phys. Chem. C 114 (39), 16475–16480. doi:10.1021/jp104317x
Chow, L. K. M., Ghaly, T. M., and Gillings, M. R. (2021). A Survey of Sub-inhibitory Concentrations of Antibiotics in the Environment. J. Environ. Sci. 99, 21–27. doi:10.1016/j.jes.2020.05.030
Colón, G., Maicu, M., Hidalgo, M. S., and Navío, J. A. (2006). Cu-doped TiO2 Systems with Improved Photocatalytic Activity. Appl. Catal. B Environ. 67 (1-2), 41–51. doi:10.1016/j.apcatb.2006.03.019
Cong, Y., Zhang, J., Chen, F., and Anpo, M. (2007). Synthesis and Characterization of Nitrogen-Doped TiO2 Nanophotocatalyst with High Visible Light Activity. J. Phys. Chem. C 111 (19), 6976–6982. doi:10.1021/jp0685030
Daghrir, R., Drogui, P., and Robert, D. (2013). Modified TiO2 for Environmental Photocatalytic Applications: A Review. Ind. Eng. Chem. Res. 52 (10), 3581–3599. doi:10.1021/ie303468t
Dalton, J. S., Janes, P. A., Jones, N. G., Nicholson, J. A., Hallam, K. R., and Allen, G. C. (2002). Photocatalytic Oxidation of NO X Gases Using TiO 2 : a Surface Spectroscopic Approach. Environ. Pollut. 120 (2), 415–422. doi:10.1016/s0269-7491(02)00107-0
Ellouzi, I., Bouddouch, A., Bakiz, B., Benlhachemi, A., and Abou Oualid, H. (2021). Glucose-assisted Ball Milling Preparation of Silver-Doped Biphasic TiO2 for Efficient Photodegradation of Rhodamine B: Effect of Silver-Dopant Loading. Chem. Phys. Lett. 770, 138456. doi:10.1016/j.cplett.2021.138456
Etacheri, V., Michlits, G., Seery, M. K., Hinder, S. J., and Pillai, S. C. (2013). A Highly Efficient TiO2-xCx Nano-Heterojunction Photocatalyst for Visible Light Induced Antibacterial Applications. ACS Appl. Mat. Interfaces 5 (5), 1663–1672. doi:10.1021/am302676a
Fan, X., Liang, X., Guo, G., Wang, R., and Li, J. (2009). “Preparation of Nano-TiO2 Powder by Polyacrylamide Gel Method,” in Second International Conference on Smart Materials and Nanotechnology in Engineering, Weihai China, 20 October 2009 (Weihai, China: International Society for Optics and Photonics), 749362. doi:10.1117/12.840137
Feng, S., Yan, X., Sun, H., Feng, Y., and Liu, H. X. (2021). Intelligent Driving Intelligence Test for Autonomous Vehicles with Naturalistic and Adversarial Environment. Nat. Commun. 12 (1), 1–14. doi:10.1038/s41467-021-21007-8
Fu, M., Yang, J., Luo, W., Tian, Q., Li, Q., Zhao, Z., et al. (2022). Preparation of Gd2Zr2O7 Nanopowders by Polyacrylamide Gel Method and Their Sintering Behaviors. J. Eur. Ceram. Soc. 42 (4), 1585–1593. doi:10.1016/j.jeurceramsoc.2021.12.038
Fujishima, A., and Rao, T. N. (1997). Recent Advances in Heterogeneous TiO2 Photocatalysis. J. Chem. Sci. 109 (6), 471–486. doi:10.1007/bf02869207
Gao, H. J., Wang, S. F., Fang, L. M., Sun, G. A., Chen, X. P., Tang, S. N., et al. (2021). Nanostructured Spinel-type M(M = Mg, Co, Zn)Cr2O4 Oxides: Novel Adsorbents for Aqueous Congo Red Removal. Mater. Today Chem. 22, 100593. doi:10.1016/j.mtchem.2021.100593
Gao, H., Tang, S., Chen, X., Yu, C., Wang, S., Fang, L., et al. (2021). Facile Synthesis of Cobalt Tungstate with Special Defect Structure with Enhanced Optical, Photoluminescence, and Supercapacitive Performances. Russ. J. Phys. Chem. A 95 (2), S288–S295. doi:10.1134/s0036024421150103
Gao, H., Wang, S., Wang, Y., Yang, H., Wang, F., Tang, S., et al. (2022). CaMoO4/CaWO4 Heterojunction Micro/nanocomposites with Interface Defects for Enhanced Photocatalytic Activity. Colloids Surfaces A Physicochem. Eng. Aspects 642, 128642. doi:10.1016/j.colsurfa.2022.128642
Gao, Y., and Wang, T. (2021). Preparation of Ag2O/TiO2 Nanocomposites by Two-step Method and Study of its Degradation of RHB. J. Mol. Struct. 1224, 129049. doi:10.1016/j.molstruc.2020.129049
Ge, X., Ren, C., Ding, Y., Chen, G., Lu, X., Wang, K., et al. (2019). Micro/nano-structured TiO2 Surface with Dual-Functional Antibacterial Effects for Biomedical Applications. Bioact. Mater. 4, 346–357. doi:10.1016/j.bioactmat.2019.10.006
Gnanasekaran, L., Pachaiappan, R., Kumar, P. S., Hoang, T. K. A., Rajendran, S., Durgalakshmi, D., et al. (2021). Visible Light Driven Exotic P (CuO) - N (TiO2) Heterojunction for the Photodegradation of 4-chlorophenol and Antibacterial Activity. Environ. Pollut. 287, 117304. doi:10.1016/j.envpol.2021.117304
Guo, L., Chen, Y., Ren, Z., Li, X., Zhang, Q., Wu, J., et al. (2021). Morphology Engineering of Type-II Heterojunction Nanoarrays for Improved Sonophotocatalytic Capability. Ultrason. Sonochemistry 81, 105849. doi:10.1016/j.ultsonch.2021.105849
Guo, Q., Zhou, C., Ma, Z., and Yang, X. (2019). Fundamentals of TiO 2 Photocatalysis: Concepts, Mechanisms, and Challenges. Adv. Mat. 31 (50), 1901997. doi:10.1002/adma.201901997
Han, X., Sun, M., Chai, X., Li, J., Wu, Y., and Sun, W. (2022). Progress in Synthesis and Photocatalytic Activity of MAl2O4(M=Mg, Sr, Ba) Based Photocatalysts. Front. Mat. 9, 40. doi:10.3389/fmats.2022.845664
Hao, R., Wang, G., Tang, H., Sun, L., Xu, C., and Han, D. (2016). Template-free Preparation of Macro/mesoporous G-C 3 N 4/TiO 2 Heterojunction Photocatalysts with Enhanced Visible Light Photocatalytic Activity. Appl. Catal. B Environ. 187, 47–58. doi:10.1016/j.apcatb.2016.01.026
He, Z., Siddique, M. S., Yang, H., Xia, Y., Su, J., Tang, B., et al. (2022). Novel Z-Scheme In2S3/Bi2WO6 Core-Shell Heterojunctions with Synergistic Enhanced Photocatalytic Degradation of Tetracycline Hydrochloride. J. Clean. Prod. 339, 130634. doi:10.1016/j.jclepro.2022.130634
He, Z., Yang, H., Su, J., Xia, Y., Fu, X., Kang, L., et al. (2021). Polyacrylamide Gel Synthesis and Photocatalytic Performance of CuCo2O4 Nanoparticles. Mater. Lett. 288, 129375. doi:10.1016/j.matlet.2021.129375
He, Z., Yang, H., Su, J., Xia, Y., Fu, X., Wang, L., et al. (2021). Construction of Multifunctional Dual Z-Scheme Composites with Enhanced Photocatalytic Activities for Degradation of Ciprofloxacin. Fuel 294, 120399. doi:10.1016/j.fuel.2021.120399
Hu, C., Zhao, Q., Zang, G.-L., Luo, J.-T., and Liu, Q. (2022). Preparation and Characterization of a Novel Ni-Doped TiO2 Nanotube-Modified Inactive Electrocatalytic Electrode for the Electrocatalytic Degradation of Phenol Wastewater. Electrochimica Acta 405, 139758. doi:10.1016/j.electacta.2021.139758
Jiang, J., Chen, Z., Wang, P., Gu, P.-Y., Liu, J., Zhang, Z., et al. (2022). Preparation of Black Hollow TiO2 Nanotube-Coated PDA@Ag2S Heterostructures for Efficient Photocatalytic Reduction of Cr(VI). J. Solid State Chem. 307, 122865. doi:10.1016/j.jssc.2021.122865
Jiang, X., Peng, Z., Gao, Y., You, F., and Yao, C. (2021). Preparation and Visible-Light Photocatalytic Activity of Ag-Loaded TiO2@Y2O3 Hollow Microspheres with Double-Shell Structure. Powder Technol. 377, 621–631. doi:10.1016/j.powtec.2020.09.030
Kale, D. P., Deshmukh, S. P., Shirsath, S. R., and Bhanvase, B. A. (2020). Sonochemical Preparation of Multifunctional rGO-ZnS-TiO2 Ternary Nanocomposite and its Application for CV Dye Removal. Optik 208, 164532. doi:10.1016/j.ijleo.2020.164532
Kaushik, R., Vineeth Daniel, P., Mondal, P., and Halder, A. (2019). Transformation of 2-D TiO2 to Mesoporous Hollow 3-D TiO2 Spheres-Comparative Studies on Morphology-dependent Photocatalytic and Anti-bacterial Activity. Microporous Mesoporous Mater. 285, 32–42. doi:10.1016/j.micromeso.2019.04.068
Li, D., Gao, X., Huang, X., Liu, P., Xiong, W., Wu, S., et al. (2020). Preparation of Organic-Inorganic Chitosan@silver/sepiolite Composites with High Synergistic Antibacterial Activity and Stability. Carbohydr. Polym. 249, 116858. doi:10.1016/j.carbpol.2020.116858
Li, G., Sun, Y., Zhang, Q., Gao, Z., Sun, W., and Zhou, X. (2021). Ag Quantum Dots Modified Hierarchically Porous and Defective TiO2 Nanoparticles for Improved Photocatalytic CO2 Reduction. Chem. Eng. J. 410, 128397. doi:10.1016/j.cej.2020.128397
Li, J., Li, F., Zhu, X., Lin, D., Li, Q., Liu, W., et al. (2017). Colossal Dielectric Permittivity in Hydrogen-Reduced Rutile TiO2 Crystals. J. Alloys Compd. 692, 375–380. doi:10.1016/j.jallcom.2016.09.044
Li, J., Wang, S., Sun, G., Gao, H., Yu, X., Tang, S., et al. (2021). Facile Preparation of MgAl2O4/CeO2/Mn3O4 Heterojunction Photocatalyst and Enhanced Photocatalytic Activity. Mater. Today Chem. 19, 100390. doi:10.1016/j.mtchem.2020.100390
Li, Z., He, J., Ma, H., Zang, L., Li, D., Guo, S., et al. (2021). Preparation of Heterogeneous TiO2/g-C3n4 with a Layered Mosaic Stack Structure by Use of Montmorillonite as a Hard Template Approach: TC Degradation, Kinetic, Mechanism, Pathway and DFT Investigation. Appl. Clay Sci. 207, 106107. doi:10.1016/j.clay.2021.106107
Liang, S., Wang, C., Li, Y., Xu, M., and Jia, H. (2021). Microfluidic Fabrication of ZrO2 Microspheres Using Improved External Gelation Aided by Polyacrylamide Networks. Ceram. Int. 47 (15), 21576–21581. doi:10.1016/j.ceramint.2021.04.169
Liao, G., Chen, S., Quan, X., Chen, H., and Zhang, Y. (2010). Photonic Crystal Coupled TiO2/Polymer Hybrid for Efficient Photocatalysis under Visible Light Irradiation. Environ. Sci. Technol. 44 (9), 3481–3485. doi:10.1021/es903833f
Lin, T.-h., Tamaki, Y., Pajarinen, J., Waters, H. A., Woo, D. K., Yao, Z., et al. (2014). Chronic Inflammation in Biomaterial-Induced Periprosthetic Osteolysis: NF-Κb as a Therapeutic Target. Acta biomater. 10 (1), 1–10. doi:10.1016/j.actbio.2013.09.034
Liu, H., Huo, W., Zhang, T. C., Ouyang, L., and Yuan, S. (2022). Photocatalytic Removal of Tetracycline by a Z-Scheme Heterojunction of Bismuth Oxyiodide/exfoliated G-C3n4: Performance, Mechanism, and Degradation Pathway. Mater. Today Chem. 23, 100729. doi:10.1016/j.mtchem.2021.100729
Liu, H., Wang, S., Gao, H., Yang, H., Wang, F., Chen, X., et al. (2022). A Simple Polyacrylamide Gel Route for the Synthesis of MgAl2O4 Nanoparticles with Different Metal Sources as an Efficient Adsorbent: Neural Network Algorithm Simulation, Equilibrium, Kinetics and Thermodynamic Studies. Sep. Purif. Technol. 281, 119855. doi:10.1016/j.seppur.2021.119855
Lou, S., Zhao, Y., Wang, J., Yin, G., Du, C., and Sun, X. (2019). Ti‐Based Oxide Anode Materials for Advanced Electrochemical Energy Storage: Lithium/Sodium Ion Batteries and Hybrid Pseudocapacitors. Small 15 (52), 1904740. doi:10.1002/smll.201904740
Low, J., Yu, J., Jaroniec, M., Wageh, S., and Al-Ghamdi, A. A. (2017). Heterojunction Photocatalysts. Adv. Mat. 29 (20), 1601694. doi:10.1002/adma.201601694
Lu, X., Yue, Z., and Peng, B. (2022). Preparation of TiO2-Nanotube-Based Photocatalysts and Degradation Kinetics of Patulin in Simulated Juice. J. Food Eng. 323, 110992. doi:10.1016/j.jfoodeng.2022.110992
Lv, Y., Sun, S., Zhang, X., Lu, X., and Dong, Z. (2021). Construction of Multi-Layered Zn-Modified TiO2 Coating by Ultrasound-Auxiliary Micro-arc Oxidation: Microstructure and Biological Property. Mater. Sci. Eng. C 131, 112487. doi:10.1016/j.msec.2021.112487
Ma, S., Zhan, S., Jia, Y., and Zhou, Q. (2015). Superior Antibacterial Activity of Fe3O4-TiO2 Nanosheets under Solar Light. ACS Appl. Mat. Interfaces 7 (39), 21875–21883. doi:10.1021/acsami.5b06264
Macwan, D. P., Dave, P. N., and Chaturvedi, S. (2011). A Review on Nano-TiO2 Sol-Gel Type Syntheses and its Applications. J. Mater Sci. 46 (11), 3669–3686. doi:10.1007/s10853-011-5378-y
Martins, N. C. T., Ângelo, J., Girão, A. V., Trindade, T., Andrade, L., and Mendes, A. (2016). N-doped Carbon Quantum dots/TiO2 Composite with Improved Photocatalytic Activity. Appl. Catal. B Environ. 193, 67–74. doi:10.1016/j.apcatb.2016.04.016
Mateo, D., Cerrillo, J. L., Durini, S., and Gascon, J. (2021). Fundamentals and Applications of Photo-Thermal Catalysis. Chem. Soc. Rev. 50 (3), 2173–2210. doi:10.1039/D0CS00357C
Mazare, A., Totea, G., Burnei, C., Schmuki, P., Demetrescu, I., and Ionita, D. (2016). Corrosion, Antibacterial Activity and Haemocompatibility of TiO 2 Nanotubes as a Function of Their Annealing Temperature. Corros. Sci. 103, 215–222. doi:10.1016/j.corsci.2015.11.021
Mori, K. (2005). Photo-Functionalized Materials Using Nanoparticles: Photocatalysis [Translated]†. Kona 23, 205–214. doi:10.14356/kona.2005023
Mousa, H. M., Alenezi, J. F., Mohamed, I. M. A., Yasin, A. S., Hashem, A.-F. M., and Abdal-hay, A. (2021). Synthesis of TiO2@ZnO Heterojunction for Dye Photodegradation and Wastewater Treatment. J. Alloys Compd. 886, 161169. doi:10.1016/j.jallcom.2021.161169
Murthy, H. (2019). Nanoarchitectures as Photoanodes. Interfacial Eng. Funct. Mater. Dye‐Sensitized Sol. Cells 7, 35–77. doi:10.1002/9781119557401.ch3
Nakata, K., and Fujishima, A. (2012). TiO2 Photocatalysis: Design and Applications. J. Photochem. Photobiol. C Photochem. Rev. 13 (3), 169–189. doi:10.1016/j.jphotochemrev.2012.06.001
Perales-Martínez, I. A., Rodríguez-González, V., Lee, S.-W., and Obregón, S. (2015). Facile Synthesis of InVO4/TiO2 Heterojunction Photocatalysts with Enhanced Photocatalytic Properties under UV-Vis Irradiation. J. Photochem. Photobiol. A Chem. 299, 152–158. doi:10.1016/j.jphotochem.2014.11.021
Phromma, S., Wutikhun, T., Kasamechonchung, P., Sattayaporn, S., Eksangsri, T., and Sapcharoenkun, C. (2022). Effects of Ag Modified TiO2 on Local Structure Investigated by XAFS and Photocatalytic Activity under Visible Light. Mater. Res. Bull. 148, 111668. doi:10.1016/j.materresbull.2021.111668
Popat, K. C., Eltgroth, M., LaTempa, T. J., Grimes, C. A., and Desai, T. A. (2007). Decreased Staphylococcus epidermis Adhesion and Increased Osteoblast Functionality on Antibiotic-Loaded Titania Nanotubes. Biomaterials 28 (32), 4880–4888. doi:10.1016/j.biomaterials.2007.07.037
Qu, Z.-w., and Kroes, G.-J. (2007). Theoretical Study of Stable, Defect-free (TiO2)n Nanoparticles with N = 10−16. J. Phys. Chem. C 111 (45), 16808–16817. doi:10.1021/jp073988t
Ren, Y. W., Guo, Y., Zhao, D. Y., Wang, H. L., Wang, N., Jiang, W. W., et al. (2022). Preparation and Characterization of Rutile TiO2 Nanoparticles by HCl-Water Volatilization-Assisted Precipitation Method. J. Cryst. Growth 577, 126410. doi:10.1016/j.jcrysgro.2021.126410
Riaz, U., Ashraf, S. M., and Kashyap, J. (2015). Role of Conducting Polymers in Enhancing TiO2-Based Photocatalytic Dye Degradation: A Short Review. Polymer-Plastics Technol. Eng. 54 (17), 1850–1870. doi:10.1080/03602559.2015.1021485
Saheed, I. O., Oh, W. D., and Suah, F. B. M. (2021). Chitosan Modifications for Adsorption of Pollutants - A Review. J. Hazard. Mater. 408, 124889. doi:10.1016/j.jhazmat.2020.124889
Santhoshkumar, P., Shaji, N., Sim, G. S., Nanthagopal, M., and Lee, C. W. (2022). Towards Environment-Friendly and Versatile Energy Storage Devices: Design and Preparation of Mesoporous Li4Ti5O12-TiO2 Nano-Hybrid Electrode Materials. Appl. Surf. Sci. 583, 152490. doi:10.1016/j.apsusc.2022.152490
Santos, E., Catto, A. C., Peterline, A. F., and Avansi Jr, W. (2022). Transition Metal (Nb and W) Doped TiO2 Nanostructures: The Role of Metal Doping in Their Photocatalytic Activity and Ozone Gas-Sensing Performance. Appl. Surf. Sci. 579, 152146. doi:10.1016/j.apsusc.2021.152146
Schuettfort, T., Nish, A., and Nicholas, R. J. (2009). Observation of a Type II Heterojunction in a Highly Ordered Polymer−Carbon Nanotube Nanohybrid Structure. Nano Lett. 9 (11), 3871–3876. doi:10.1021/nl902081t
Shifa Wang, S., Gao, H., Sun, G., Wang, Y., Fang, L., Yang, L., et al. (2020). Synthesis of Visible-Light-Driven SrAl2O4-Based Photocatalysts Using Surface Modification and Ion Doping. Russ. J. Phys. Chem. 94 (6), 1234–1247. doi:10.1134/s003602442006031x
Skorb, E. V., Antonouskaya, L. I., Belyasova, N. A., Shchukin, D. G., Möhwald, H., and Sviridov, D. V. (2008). Antibacterial Activity of Thin-Film Photocatalysts Based on Metal-Modified TiO2 and TiO2: In2O3 Nanocomposite. Appl. Catal. B Environ. 84 (1-2), 94–99. doi:10.1016/j.apcatb.2008.03.007
Sood, S., Mehta, S. K., Sinha, A. S. K., and Kansal, S. K. (2016). Bi 2 O 3/TiO 2 Heterostructures: Synthesis, Characterization and Their Application in Solar Light Mediated Photocatalyzed Degradation of an Antibiotic, Ofloxacin. Chem. Eng. J. 290, 45–52. doi:10.1016/j.jphotochem.2019.04.02810.1016/j.cej.2016.01.017
Sotelo-Vazquez, C., Quesada-Cabrera, R., Ling, M., Scanlon, D. O., Kafizas, A., Thakur, P. K., et al. (2017). Evidence and Effect of Photogenerated Charge Transfer for Enhanced Photocatalysis in WO3/TiO2 Heterojunction Films: A Computational and Experimental Study. Adv. Funct. Mat. 27 (18), 1605413. doi:10.1002/adfm.201605413
Sun, S., Ding, H., Wang, J., Li, W., and Hao, Q. (2020). Preparation of a Microsphere SiO2/TiO2 Composite Pigment: The Mechanism of Improving Pigment Properties by SiO2. Ceram. Int. 46 (14), 22944–22953. doi:10.1016/j.ceramint.2020.06.068
Tang, S., Gao, H., Wang, S., Yu, C., Chen, X., Liu, H., et al. (2022). Temperature Dependence of the Phase Transformation and Photoluminescence Properties of Metastable ZnWO4 Nano-Phosphors with High UV Absorption and VIS Reflectance. Russ. J. Phys. Chem. 96 (3), 515–526. doi:10.1134/s0036024422030220
Tang, Z., Xu, L., Shu, K., Yang, J., and Tang, H. (2022). Fabrication of TiO2 @MoS2 Heterostructures with Improved Visible Light Photocatalytic Activity. Colloids Surfaces A Physicochem. Eng. Aspects 642, 128686. doi:10.1016/j.colsurfa.2022.128686
Tiwari, I., Sharma, P., and Nebhani, L. (2022). Polybenzoxazine - an Enticing Precursor for Engineering Heteroatom-Doped Porous Carbon Materials with Applications beyond Energy, Environment and Catalysis. Mater. Today Chem. 23, 100734. doi:10.1016/j.mtchem.2021.100734
Tung, W. S., and Daoud, W. A. (2011). Self-cleaning Fibers via Nanotechnology: a Virtual Reality. J. Mat. Chem. 21 (22), 7858–7869. doi:10.1039/c0jm03856c
Vandana, U., Nancy, D., Sabareeswaran, A., Remya, N. S., Rajendran, N., and Mohanan, P. V. (2021). Biocompatibility of Strontium Incorporated Ceramic Coated Titanium Oxide Implant Indented for Orthopaedic Applications. Mater. Sci. Eng. B 264, 114954. doi:10.1016/j.mseb.2020.114954
Venkatachalam, N., Palanichamy, M., and Murugesan, V. (2007). Sol-gel Preparation and Characterization of Alkaline Earth Metal Doped Nano TiO2: Efficient Photocatalytic Degradation of 4-chlorophenol. J. Mol. Catal. A Chem. 273 (1-2), 177–185. doi:10.1016/j.molcata.2007.03.077
Vorontsov, A. V., Savinov, E. V., Davydov, L., and Smirniotis, P. G. (2001). Photocatalytic Destruction of Gaseous Diethyl Sulfide over TiO2. Appl. Catal. B Environ. 32 (1-2), 11–24. doi:10.1016/s0926-3373(01)00127-8
Wang, C. Y., Makvandi, P., Zare, E. N., Tay, F. R., and Niu, L. N. (2020). Advances in Antimicrobial Organic and Inorganic Nanocompounds in Biomedicine. Adv. Ther. 3 (8), 2000024. doi:10.1002/adtp.202000024
Wang, S., Gao, H., Fang, L., Hu, Q., Sun, G., Chen, X., et al. (2021). Synthesis of Novel CQDs/CeO2/SrFe12O19 Magnetic Separation Photocatalysts and Synergic Adsorption-Photocatalytic Degradation Effect for Methylene Blue Dye Removal. Chem. Eng. J. Adv. 6, 100089. doi:10.1016/j.ceja.2021.100089
Wang, S., Gao, H., Yu, X., Tang, S., Wang, Y., Fang, L., et al. (2020). Nanostructured SrTiO3 with Different Morphologies Achieved by Mineral Acid-Assisted Hydrothermal Method with Enhanced Optical, Electrochemical, and Photocatalytic Performances. J. Mater Sci. Mater Electron 31 (20), 17736–17754. doi:10.1007/s10854-020-04328-0
Wang, S., Tang, S., Yang, H., Wang, F., Yu, C., Gao, H., et al. (2022). A Novel Heterojunction ZnO/CuO Piezoelectric Catalysts: Fabrication, Optical Properties and Piezoelectric Catalytic Activity for Efficient Degradation of Methylene Blue. J. Mater Sci. Mater Electron 33 (9), 7172–7190. doi:10.1007/s10854-022-07899-2
Wang, S., Wang, Y., Gao, H., Li, J., Fang, L., Yu, X., et al. (2020). Synthesis and Characterization of BaAl2O4: Ce and Mn-Ce- Co-doped BaAl2O4 Composite Materials by a Modified Polyacrylamide Gel Method and Prediction of Photocatalytic Activity Using Artificial Neural Network (ANN) Algorithm. Optik 221, 165363. doi:10.1016/j.ijleo.2020.165363
Wang, S., Wei, X., Gao, H., and Wei, Y. (2019). Effect of Amorphous Alumina and α-alumina on Optical, Color, Fluorescence Properties and Photocatalytic Activity of the MnAl2O4 Spinel Oxides. Optik 185, 301–310. doi:10.1016/j.ijleo.2019.03.147
Wang, X., Ding, H., Sun, S., Zhang, H., Zhou, R., Li, Y., et al. (2021). Preparation of a Temperature-Sensitive Superhydrophobic Self-Cleaning SiO2-TiO2@PDMS Coating with Photocatalytic Activity. Surf. Coatings Technol. 408, 126853. doi:10.1016/j.surfcoat.2021.126853
Wu, J.-J., and Yu, C.-C. (2004). Aligned TiO2 Nanorods and Nanowalls. J. Phys. Chem. B 108 (11), 3377–3379. doi:10.1021/jp0361935
Wu, Y., Yan, K., Xu, G., Yang, C., and Wang, D. (2021). Facile Preparation of Super-oleophobic TiO2/SiO2 Composite Coatings by Spraying Method. Prog. Org. Coatings 159, 106411. doi:10.1016/j.porgcoat.2021.106411
Wu, Z., Wu, Q., Du, L., Jiang, C., and Piao, L. (2014). Progress in the Synthesis and Applications of Hierarchical Flower-like TiO2 Nanostructures. Particuology 15, 61–70. doi:10.1016/j.partic.2013.04.003
Wu, Z., Zhao, Y., Chen, X., Guo, Y., Wang, H., Jin, Y., et al. (2022). Preparation of Polymeric Carbon nitride/TiO2 Heterostructure with NH4Cl as Template: Structural and Photocatalytic Studies. J. Phys. Chem. Solids 164, 110629. doi:10.1016/j.jpcs.2022.110629
Xian, T., Yang, H., Di, L. J., Chen, X. F., and Dai, J. F. (2013). Polyacrylamide Gel Synthesis and Photocatalytic Properties of TiO2 Nanoparticles. J. Sol-Gel Sci. Technol. 66 (2), 324–329. doi:10.1007/s10971-013-3013-x
Xiang, J., Liu, H., Na, R., Wang, D., Shan, Z., and Tian, J. (2020). Facile Preparation of Void-Buffered Si@TiO2/C Microspheres for High-Capacity Lithium Ion Battery Anodes. Electrochimica Acta 337, 135841. doi:10.1016/j.electacta.2020.135841
Xie, D., Wang, Y., Yu, H., Yang, X., Geng, S., and Meng, X. (2021). Molten-salt Defect Engineering of TiO2(B) Nanobelts for Enhanced Photocatalytic Hydrogen Evolution. JCIS Open 4, 100031. doi:10.1016/j.jciso.2021.100031
Xu, J., Liu, Z., Wang, J., Liu, P., Ahmad, M., Zhang, Q., et al. (2022). Preparation of Core-Shell C@TiO2 Composite Microspheres with Wrinkled Morphology and its Microwave Absorption. J. Colloid Interface Sci. 607, 1036–1049. doi:10.1016/j.jcis.2021.09.038
Yadav, H. M., Otari, S. V., Bohara, R. A., Mali, S. S., Pawar, S. H., and Delekar, S. D. (2014). Synthesis and Visible Light Photocatalytic Antibacterial Activity of Nickel-Doped TiO2 Nanoparticles against Gram-Positive and Gram-Negative Bacteria. J. Photochem. Photobiol. A Chem. 294, 130–136. doi:10.1016/j.jphotochem.2014.07.024
Yu, J., Wang, W., Cheng, B., and Su, B.-L. (2009). Enhancement of Photocatalytic Activity of Mesporous TiO2 Powders by Hydrothermal Surface Fluorination Treatment. J. Phys. Chem. C 113 (16), 6743–6750. doi:10.1021/jp900136q
Zhang, B., Li, B., Gao, S., Li, Y., Cao, R., Cheng, J., et al. (2020). Y-doped TiO2 Coating with Superior Bioactivity and Antibacterial Property Prepared via Plasma Electrolytic Oxidation. Mater. Des. 192, 108758. doi:10.1016/j.matdes.2020.108758
Zhang, D., Chen, Q., Shi, C., Chen, M., Ma, K., Wan, J., et al. (2021). Dealing with the Foreign‐Body Response to Implanted Biomaterials: Strategies and Applications of New Materials. Adv. Funct. Mat. 31 (6), 2007226. doi:10.1002/adfm.202007226
Zhang, S., Guo, W., Liu, N., Xia, C., Wang, H., and Liang, C. (2022). In Situ preparation of MAO/TiO2 Composite Coating on WE43 Alloy for Anti-corrosion Protection. Vacuum 197, 110835. doi:10.1016/j.vacuum.2021.110835
Zhang, Y., Zhao, Z., Chen, J., Cheng, L., Chang, J., Sheng, W., et al. (2015). C-doped Hollow TiO2 Spheres: In Situ Synthesis, Controlled Shell Thickness, and Superior Visible-Light Photocatalytic Activity. Appl. Catal. B Environ. 165, 715–722. doi:10.1016/j.apcatb.2014.10.063
Zhang, Z., Wang, C. C., Zakaria, R., and Ying, J. Y. (1998). Role of Particle Size in Nanocrystalline TiO2-Based Photocatalysts. J. Phys. Chem. B 102 (52), 10871–10878. doi:10.1021/jp982948
Zhao, L., Wang, H., Huo, K., Zhang, X., Wang, W., Zhang, Y., et al. (2013). The Osteogenic Activity of Strontium Loaded Titania Nanotube Arrays on Titanium Substrates. Biomaterials 34 (1), 19–29. doi:10.1016/j.biomaterials.2012.09.041
Zhou, F., Yan, C., Sun, Q., and Komarneni, S. (2019). TiO2/Sepiolite Nanocomposites Doped with Rare Earth Ions: Preparation, Characterization and Visible Light Photocatalytic Activity. Microporous Mesoporous Mater. 274, 25–32. doi:10.1016/j.micromeso.2018.07.031
Zhu, G., Yang, X., Liu, Y., Zeng, Y., Wang, T., and Yu, H. (2022). One-pot Synthesis of C-Modified and N-Doped TiO2 for Enhanced Visible-Light Photocatalytic Activity. J. Alloys Compd. 902, 163677. doi:10.1016/j.jallcom.2022.163677
Keywords: TiO2, heterojunction, photocatalytic activity, antibacterial activity, orthopedic implants
Citation: Qi L, Guo B, Lu Q, Gong H, Wang M, He J, Jia B, Ren J, Zheng S and Lu Y (2022) Preparation and Photocatalytic and Antibacterial Activities of Micro/Nanostructured TiO2-Based Photocatalysts for Application in Orthopedic Implants. Front. Mater. 9:914905. doi: 10.3389/fmats.2022.914905
Received: 07 April 2022; Accepted: 20 April 2022;
Published: 23 May 2022.
Edited by:
Hua Yang, Lanzhou University of Technology, ChinaReviewed by:
Yi Li, China Academy of Space Technology, ChinaZuming He, Changzhou University, China
Tao Xian, Qinghai Normal University, China
Copyright © 2022 Qi, Guo, Lu, Gong, Wang, He, Jia, Ren, Zheng and Lu. This is an open-access article distributed under the terms of the Creative Commons Attribution License (CC BY). The use, distribution or reproduction in other forums is permitted, provided the original author(s) and the copyright owner(s) are credited and that the original publication in this journal is cited, in accordance with accepted academic practice. No use, distribution or reproduction is permitted which does not comply with these terms.
*Correspondence: Binghua Guo, 395128339@qq.com