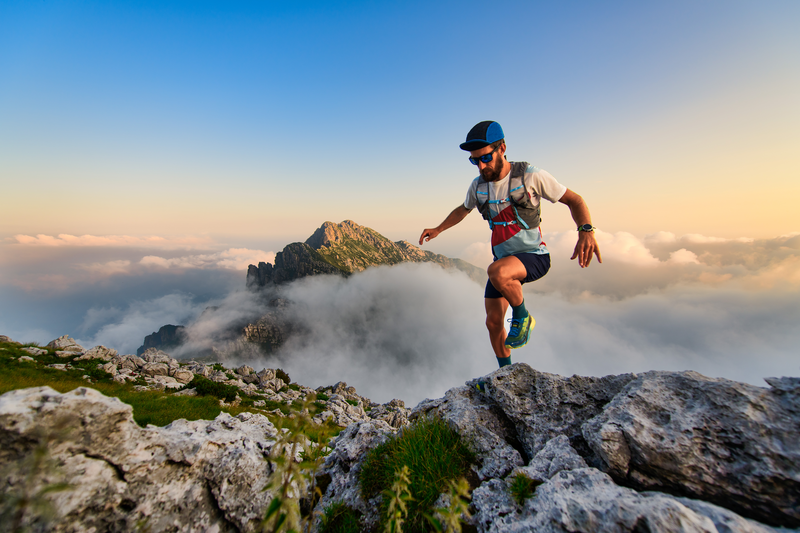
95% of researchers rate our articles as excellent or good
Learn more about the work of our research integrity team to safeguard the quality of each article we publish.
Find out more
OPINION article
Front. Mater. , 27 June 2022
Sec. Biomaterials
Volume 9 - 2022 | https://doi.org/10.3389/fmats.2022.912685
Research is continuously searching for new knowledge niches or technical developments that could have a societal impact and game-changing fundamental findings. Since the biomedical field entangles several disciplines, a particular problem is tackled from different approaches which helps to develop new solutions that can crystallize in biomedical devices with applications in diagnosis, therapy or theranostics. Certainly, the properties of the materials under study govern the scope of the biomedical research, e.g., quantum dots (QDs) and its fluorescence properties for imaging and detection or magnetic nanoparticles (NPs) for magnetic resonance imaging (MRI) and hyperthermia. In this sense, elastin-like polypeptides (ELPs) are not different and their capability to assemble into nanoparticles by themselves has naturally attracted most of the attention for their use in biomedical devices. Therefore, less efforts have been made in their application in NP synthetic procedures. ELPs are genetically engineered repetitive proteins derived from tropoelastin. Their thermoresponsive properties allows the formation of supramolecular structures by phase separation in a reversible fashion. In aqueous solvents below their transition temperature (Tt) they are solubilized, while above Tt they acquire hydrophobic character and collapse causing the segregation. This reversible conformational change from random coil to primarily type-II β-turns leads to the formation of a coacervates (Löwik et al., 2010). The Tt or lower critical solution temperature (LCST) is defined by the hydrophilic/hydrophobic balance of the amino acid sequence (VPGXG), were X can be any residue except proline (Varanko et al., 2020). This property has been widely used for recombinant protein solubilization and purification in which the protein of interest is elongated with an ELP polypeptide and then subjected to an inverse transition cycling (ITC) procedure to separate the target protein from the lysate products (Fletcher et al., 2019). Since the building blocks of ELPs are natural amino acids, their biocompatibility, biodegradability, and non-immunogenicity make them even more attractive for applications in tissue engineering or nanomedicine (Jenkins et al., 2021). One of the first examples of their application in the nanomedicine field was carried out by Chilkoti laboratory, where the introduction of a cysteine-containing segment allowed the conjugation of a doxorubicin derivative through a pH cleavable linker (Andrew MacKay et al., 2009). This drug-polypeptide conjugate was able to form 100 nm nanoparticles and deliver their chemotherapeutic payload in a murine cancer model, inducing an almost completely tumor regression after a single dose. ELPs engineering also can serve as a straightforward way to incorporate protein-based targeting agents or encapsulate the protein of interest with high efficiency (van Oppen et al., 2019; Pille et al., 2021).
Since inorganic nanoparticle synthetic processes are varied but needed for physiological stabilization in biomedical applications, one may expect that ELPs have been widely applied as NP coatings. Instead, best examples of organic-inorganic hybrid materials based on elastin-like polypeptides found in the fabrication of biomedical devices are minded for applications as tissue engineering, hydrogels actuators, cardiovascular implants or antimicrobial materials between others (Anh et al., 2013; Wang et al., 2013; Mbundi et al., 2021). The main approach to build hybrid NP-based biomedical devices is to perform a conjugation of the ELP to the surface of the nanoparticle but also ligand exchange, hydrophobic interactions or physical immobilization have been used in a post-synthetic route (Ta et al., 2017; Zhang et al., 2018; Alvisi et al., 2021). In the other hand, regarding the design of ELPs to stabilize or synthesize NPs only a few examples arise. Fahmi et al. reported the successful stabilization of CdSe NPs to form fibers in aqueous media through the incorporation of glutamic acid as a gest residue in the ELP to coordinate Cd (II) ions prior to their precipitation with NaHSe Fahmi et al. (2010). In parallel Alvarez-Rodriguez and coworkers carried out the generation of ELP-stabilized gold nanoparticles through cysteine residues in one pot for their use as stimuli responsive optical sensors and detectors for biological applications Alvarez-Rodriguez et al. (2010). More complex structures have been recently achieved by Zhou et al. by coupling the biomineralization of iron oxide NPs with further biosilicification triggered by lysine residues which delivers a green route to obtain magnetic thermoresponsive nanohybrids in one pot Zhou et al. (2021). Another example of ELPs opportunities in synthetic procedures is the incorporation of specific protein domains other than targeting moieties able to trigger the biomineralization of the assemblies. Mimicking the silica formation in diatoms, the elongation of ELPs with silaffin domains as triggers of the formation of silica structures led to discrete sub-100 nm diameter hybrid ELP-silica particles Han et al. (2015). This way, researchers have unveiled that the ELP tunability is highly versatile and useful for building biomedical devices, especially the ones with stimuli-responsive behavior that allow to control their performance in a precise manner.
Generally, two main routes are used to build stable nanoparticles: their surface modification/stabilization after the NP growth (post-synthetic procedure) or the introduction of the stabilizing agents that also take part in the NP synthesis (in situ stabilization). Therefore, two key features of ELPs that can actively contribute to the NP synthetic procedures: their guest residue flexibility, and solubility in organic solvents. The control over polypeptide sequence allows to choose the guest residues to match the desired ELP properties besides their Tt, e.g., net charge or metal binding capabilities. Simultaneously, their lack of secondary structure and organic solvent solubility expand their use to synthetic procedures that most of the proteins cannot participate, due to poor solubility or unreversible precipitation that hinders a proper refolding. Thus, ELPs are an excellent platform to exploit the properties of inorganic nanoparticles into biomedical devices.
The introduction of specific residues in fixed locations but also nature-inspired sequences make ELPs capable to program organic–inorganic interactions to form hybrid structures (Figure 1). This is especially important when only the combination of both components can address the specific needs of an application, besides an expansion of the synthetic portfolio which is indeed valuable. Curiously, this concept has been applied to a much lesser extent in ELP-templated synthesis for the fabrication of physiologically stable hybrid nanoparticles, employing ELPs as an active part of the synthetic procedure. As presented before, this methodology is not only capable to address the stabilization of the nanoparticles but also their biocompatibility, which is a major concern in the use of some heavy metal nanoparticles in biomedical field. Furthermore, the control of NP size and its properties has been done already with peptides or engineered proteins (Li et al., 2021; Uribe et al., 2021). These strategies reveal how guest residues as tyrosine, cysteine or tryptophan are capable to drive reduction, condensation or coordination of species that guide the formation of 1D nanostructures, as well as NPs stabilization.
FIGURE 1. The variety of guest residues that can be tailored in the polypeptide sequence enrich the capabilities of ELPs, bringing many opportunities for their use in nanoparticle synthesis (left). Summative scheme to gather the background, current status and future developments in nanoparticle synthesis through ELPs.
Aside the chemical diversity of the guest residues and motifs that can be engineered into ELPs they are essentially random coils that can be solubilized in organic solvents with different polarities. (Verheul et al., 2018; Zhao et al., 2020) This property has been exploited by researchers not only to perform ELPs purification but also for the fabrication of biomedical devices through processes as electrospinning or 3D bioprinting. (Putzu et al., 2019; Dai et al., 2021) Specially revealing is the work carried out by Elsharkawy and colleges where ELPs are used to control the biomineralization of apatite nanocrystals by tuning the disorder–order ratio. Elsharkawy et al. (2018) Despite the aqueous green synthetic routes are preferred, solubility in organic media can lead a good approach to build materials that cannot be fabricated in water. (Zhang et al., 2013; Desai et al., 2016) This solubility performance of ELPs could allow them to act as stabilizers in organic solvents during the synthesis of the NPs from metal salts but also as transfer agents from an immiscible organic phase to an aqueous media in a straightforward way, which eases the post-synthetic treatment and therefore benefit its further industrial applications. Again, added to the solubility, the diversity in the guest residues available by protein engineering make ELPs useful for metal coordination or surface stabilization in organic media. These features are indeed key aspects in the synthesis of nanoparticles using metal alkoxides by non-aqueous sol–gel chemistry or thermal decomposition of organometallic precursors. New advances in sol–gel chemistry avoiding calcination which includes alternative heating processes and lower-temperature routes are extensively reviewed (Niederberger, 2007; Danks et al., 2016) and have to be adapted to allow the use of polypeptides in this synthetic processes. The advantages of this sort of pathways are the enhanced control over the kinetics of the reactions and therefore the crystallization of the nanoparticles.
In summary, despite the versatility showed by ELPs as an active part of different synthetic procedures the state or the art has been mostly focused on the use of ELPs to assemble into NPs or in combination with small molecules, polymers, or post-synthetic modification nanoparticles. Only a few examples are found in the literature were ELPs drive the formation or stabilization of NP surface during the synthesis in aqueous media. Hence, there is still room for the expansion of the methods and approaches to build new NP-based biomedical devices. Biomineralization is an exciting route to build many different nanoarchitectures, especially due to the growing evidence around the role that disordered proteins may play in building well-defined structures in addition to ELPs sequence versatility. Moreover, the secondary structure adopted by ELPs inside coacervate phase lays out the question about their capability to contribute with directionality to the nanocrystal growth, as showed for other mineralization processes mention above. Biomimetic routes can also be carried out in organic matrices that allow to control the organic-inorganic interactions. In the other hand, after a thoroughly literature review no examples of the use of ELPs as surface stabilizers or structure-directing agents in non-aqueous solvents were found. (Portehault et al., 2018) The rational design of ELPs in combination with synthetic procedures in organic solvents may lead to new synthetic approaches that can simplify or circumvent certain processes at purification or production, which in turn will benefit the pipelines of NP-based biomedical devices fabrication and support their application feasibility. Since the interests in enlarging the portfolio of inorganic nanostructures are increasing, the exploration of novel compositions or synthetic approaches may retrieve original properties or discover new behaviors of the designed nano-objects.
EG carried out the literature review, drafting, analysis, and final version of the whole manuscript.
This project has received funding from the European Union’s Horizon 2020 research and innovation programme under the Marie Skłodowska-Curie grant agreement No. 101029446—Healthcare applications of Stimuli-Responsive ElastinLike Polypeptide Nanocarriers (HSRELPNANO).
The author declares that the research was conducted in the absence of any commercial or financial relationships that could be construed as a potential conflict of interest.
All claims expressed in this article are solely those of the authors and do not necessarily represent those of their affiliated organizations, or those of the publisher, the editors and the reviewers. Any product that may be evaluated in this article, or claim that may be made by its manufacturer, is not guaranteed or endorsed by the publisher.
The author acknowledges the TU/e Biomedical Engineering Department, in particular Prof. Jan C. M. van Hest for his mentorship and support in the development of HSRELPNANO project and Duc H. T. Le for sharing his laboratory experience.
Alvarez-Rodriguez, R., Alonso, M., Girotti, A., Reboto, V., and Rodriguez-Cabello, J. C. (2010). One-pot Synthesis of pH and Temperature Sensitive Gold Clusters Mediated by a Recombinant Elastin-like Polymer. Eur. Polym. J. 46, 643–650. doi:10.1016/j.eurpolymj.2009.12.022
Alvisi, N., Gutiérrez-Mejía, F. A., Lokker, M., Lin, Y.-T., de Jong, A. M., van Delft, F., et al. (2021). Self-Assembly of Elastin-like Polypeptide Brushes on Silica Surfaces and Nanoparticles. Biomacromolecules 22, 1966–1979. doi:10.1021/acs.biomac.1c00067
Andrew MacKay, J., Chen, M., McDaniel, J. R., Liu, W., Simnick, A. J., and Chilkoti, A. (2009). Self-assembling Chimeric Polypeptide-Doxorubicin Conjugate Nanoparticles that Abolish Tumours after a Single Injection. Nat. Mater 8, 993–999. doi:10.1038/nmat2569
Anh, T. T. H., Xing, M., Le, D. H. T., Sugawara-Narutaki, A., and Fong, E. (2013). Elastin-based Silver-Binding Proteins with Antibacterial Capabilities. Nanomedicine 8, 567–575. doi:10.2217/nnm.13.47
Dai, M., Belaïdi, J.-P., Fleury, G., Garanger, E., Rielland, M., Schultze, X., et al. (2021). Elastin-like Polypeptide-Based Bioink: A Promising Alternative for 3D Bioprinting. Biomacromolecules 22, 4956–4966. doi:10.1021/acs.biomac.1c00861
Danks, A. E., Hall, S. R., and Schnepp, Z. (2016). The Evolution of 'sol-Gel' Chemistry as a Technique for Materials Synthesis. Mat. Horiz. 3, 91–112. doi:10.1039/C5MH00260E
Desai, M. S., Wang, E., Joyner, K., Chung, T. W., Jin, H.-E., and Lee, S.-W. (2016). Elastin-Based Rubber-like Hydrogels. Biomacromolecules 17, 2409–2416. doi:10.1021/acs.biomac.6b00515
Elsharkawy, S., Al-Jawad, M., Pantano, M. F., Tejeda-Montes, E., Mehta, K., Jamal, H., et al. (2018). Protein Disorder-Order Interplay to Guide the Growth of Hierarchical Mineralized Structures. Nat. Commun. 9. doi:10.1038/s41467-018-04319-0
Fahmi, A., Pietsch, T., Bryszewska, M., Rodríguez-Cabello, J. C., Koceva-Chyla, A., Arias, F. J., et al. (2010). Fabrication of CdSe-Nanofibers with Potential for Biomedical Applications. Adv. Funct. Mat. 20, 1011–1018. doi:10.1002/adfm.200902013
Fletcher, E. E., Yan, D., Kosiba, A. A., Zhou, Y., and Shi, H. (2019). Biotechnological Applications of Elastin-like Polypeptides and the Inverse Transition Cycle in the Pharmaceutical Industry. Protein Expr. Purif. 153, 114–120. doi:10.1016/j.pep.2018.09.006
Han, W., MacEwan, S. R., Chilkoti, A., and López, G. P. (2015). Bio-inspired Synthesis of Hybrid Silica Nanoparticles Templated from Elastin-like Polypeptide Micelles. Nanoscale 7, 12038–12044. doi:10.1039/C5NR01407G
Jenkins, I. C., Milligan, J. J., and Chilkoti, A. (2021). Genetically Encoded Elastin‐Like Polypeptides for Drug Delivery. Adv. Healthc. Mater. 10, 2100209–2100218. doi:10.1002/adhm.202100209
Li, X., Jian, M., Sun, Y., Zhu, Q., and Wang, Z. (2021). The Peptide Functionalized Inorganic Nanoparticles for Cancer-Related Bioanalytical and Biomedical Applications. Molecules 26, 3228–3230. doi:10.3390/molecules26113228
Löwik, D. W. P. M., Leunissen, E. H. P., van den Heuvel, M., Hansen, M. B., and van Hest, J. C. M. (2010). Stimulus Responsive Peptide Based Materials. Chem. Soc. Rev. 39, 3394. doi:10.1039/b914342b
Mbundi, L., González-Pérez, M., González-Pérez, F., Juanes-Gusano, D., and Rodríguez-Cabello, J. C. (2021). Trends in the Development of Tailored Elastin-like Recombinamer-Based Porous Biomaterials for Soft and Hard Tissue Applications. Front. Mat. 7, 1–27. doi:10.3389/fmats.2020.601795
Niederberger, M. (2007). Nonaqueous Sol-Gel Routes to Metal Oxide Nanoparticles. Acc. Chem. Res. 40, 793–800. doi:10.1021/ar600035e
Pille, J., Aloi, A., Le, D. H. T., Vialshin, I., Laar, N., Kevenaar, K., et al. (2021). Pathway‐Dependent Co‐Assembly of Elastin‐Like Polypeptides. Small 17, 2007234. doi:10.1002/smll.202007234
Portehault, D., Delacroix, S., Gouget, G., Grosjean, R., and Chan-Chang, T.-H. -C. (2018). Beyond the Compositional Threshold of Nanoparticle-Based Materials. Acc. Chem. Res. 51, 930–939. doi:10.1021/acs.accounts.7b00429
Putzu, M., Causa, F., Parente, M., González de Torre, I., Rodriguez-Cabello, J. C., and Netti, P. A. (2019). Silk-ELR Co-recombinamer Covered Stents Obtained by Electrospinning. Regen. Biomater. 6, 21–28. doi:10.1093/rb/rby022
Ta, D. T., Vanella, R., and Nash, M. A. (2017). Magnetic Separation of Elastin-like Polypeptide Receptors for Enrichment of Cellular and Molecular Targets. Nano Lett. 17, 7932–7939. doi:10.1021/acs.nanolett.7b04318
Uribe, K. B., Guisasola, E., Aires, A., López-Martínez, E., Guedes, G., Sasselli, I. R., et al. (2021). Engineered Repeat Protein Hybrids: The New Horizon for Biologic Medicines and Diagnostic Tools. Acc. Chem. Res. 54, 4166–4177. doi:10.1021/acs.accounts.1c00440
van Oppen, L. M. P. E., Pille, J., Stuut, C., van Stevendaal, M., van der Vorm, L. N., Smeitink, J. A. M., et al. (2019). Octa-arginine Boosts the Penetration of Elastin-like Polypeptide Nanoparticles in 3D Cancer Models. Eur. J. Pharm. Biopharm. 137, 175–184. doi:10.1016/j.ejpb.2019.02.010
Varanko, A. K., Su, J. C., and Chilkoti, A. (2020). Elastin-Like Polypeptides for Biomedical Applications. Annu. Rev. Biomed. Eng. 22, 343–369. doi:10.1146/annurev-bioeng-092419-061127
Verheul, R., Sweet, C., and Thompson, D. H. (2018). Rapid and Simple Purification of Elastin-like Polypeptides Directly from Whole Cells and Cell Lysates by Organic Solvent Extraction. Biomater. Sci. 6, 863–876. doi:10.1039/c8bm00124c
Wang, E., Desai, M. S., and Lee, S.-W. (2013). Light-Controlled Graphene-Elastin Composite Hydrogel Actuators. Nano Lett. 13, 2826–2830. doi:10.1021/nl401088b
Zhang, H., Chen, J., Xiao, C., Tao, Y., and Wang, X. (2018). A Multifunctional Polypeptide via Ugi Reaction for Compact and Biocompatible Quantum Dots with Efficient Bioconjugation. Bioconjugate Chem. 29, 1335–1343. doi:10.1021/acs.bioconjchem.8b00072
Zhang, J., Yuan, Z.-F., Wang, Y., Chen, W.-H., Luo, G.-F., Cheng, S.-X., et al. (2013). Multifunctional Envelope-type Mesoporous Silica Nanoparticles for Tumor-Triggered Targeting Drug Delivery. J. Am. Chem. Soc. 135, 5068–5073. doi:10.1021/ja312004m
Zhao, Y., Singh, M. K., Kremer, K., Cortes-Huerto, R., and Mukherji, D. (2020). Why Do Elastin-like Polypeptides Possibly Have Different Solvation Behaviors in Water-Ethanol and Water-Urea Mixtures? Macromolecules 53, 2101–2110. doi:10.1021/acs.macromol.9b02123
Keywords: elastin-like polypeptides, drug delivery, nanomedicine, nanoparticles, protein engineering, polymers, self-assembly
Citation: Guisasola E (2022) Broadening Approaches in Nanoparticle Synthesis Through Elastin-Like Polypeptides. Front. Mater. 9:912685. doi: 10.3389/fmats.2022.912685
Received: 04 April 2022; Accepted: 13 June 2022;
Published: 27 June 2022.
Edited by:
Guocheng Wang, Shenzhen Institutes of Advanced Technology (CAS), ChinaReviewed by:
Jiao Jiao Li, University of Technology Sydney, AustraliaCopyright © 2022 Guisasola. This is an open-access article distributed under the terms of the Creative Commons Attribution License (CC BY). The use, distribution or reproduction in other forums is permitted, provided the original author(s) and the copyright owner(s) are credited and that the original publication in this journal is cited, in accordance with accepted academic practice. No use, distribution or reproduction is permitted which does not comply with these terms.
*Correspondence: Eduardo Guisasola, ZS5qLmd1aXNhc29sYUB0dWUubmw=
Disclaimer: All claims expressed in this article are solely those of the authors and do not necessarily represent those of their affiliated organizations, or those of the publisher, the editors and the reviewers. Any product that may be evaluated in this article or claim that may be made by its manufacturer is not guaranteed or endorsed by the publisher.
Research integrity at Frontiers
Learn more about the work of our research integrity team to safeguard the quality of each article we publish.