- 1Department of Environmental Engineering, Mersin University, Mersin, Turkey
- 2Department of Pharmaceutical Microbiology, Faculty of Pharmacy, Mersin University, Mersin, Turkey
- 3Food Processing Programme, Technical Science Vocational School, Mersin University, Mersin, Turkey
- 4College of Engineering, Prince Mohammad Bin Fahd University, Al Khobar, Saudi Arabia
- 5Department of Mechanical Engineering, Jyothi Engineering College, Thrissur, India
- 6Department of Physics, Zhejiang Normal University, Jinhua, China
Currently, nanotechnology and nanoparticles have been quickly emerged and have gained the attention of scientists due to their massive applications in environmental sectors. Nanotechnology also encompasses the ability to design, characterize, manufacture, and implement nano-sized structures. Today, metal oxide nanoparticles stand out in industrial applications in various fields of applied nanotechnology. Among metal oxide nanoparticles, iron oxide nanoparticles (FeO-NPs) are one of the widely used NPs. Green chemistry-based nanoparticles production is one of the most interesting topics in recent years. In the present study, we used vermicomposting leachate to synthesize FeO-NPs. First, vermicomposting leachate (VCL) was produced and then FeO-NPs was obtained from ferric chloride salt. FeO-NPs was characterized by scanning electron microscopy with energy dispersive X-ray spectroscopy (SEM/EDX) and X-ray diffraction (XRD). Additionally, the antioxidant activities of FeO-NPs synthesized from vermicomposting leachate (VCL-FeO-NPs) were evaluated by DPPH scavenging activity. The highest DPPH activities of VCL-FeO-NPs at 200 mg/L concentration were 93.54%. In addition, the nanoparticles showed significant DNA nuclease activity. The antimicrobial activities of VCL-FeO-NPs were studied in micro dilution methods and it exhibited moderate antimicrobial activity through Gr +ve, Gr −ve, and fungi. The nanoparticles showed more effective microbial cell inhibition activity against E. coli. Also, biofilm inhibition results were detected against S. aureus and P. aeruginosa were 66.05% and 67.29%, respectively.
Introduction
Nanotechnology (NT) and nanoscience is one of the remarkable areas of science dealing with using the structure of nanoscale (Kakakhel et al., 2021). NT is used in the medical field, pharmacology, optics, electronics, food industry, textiles, wastewater treatment, agriculture, (Azarang et al., 2014; Fernández et al., 2016). In addition, NT is used extensively in antimicrobial, catalysis and toxicity science (Potbhare et al., 2019; Umekar et al., 2021; Yabalak et al., 2022). The nanoparticles (NPs) are found in different types based on by their chemical structure. Nevertheless, some processes of nanoparticle’s synthesis do not accord economically with their biological aim. Such as, till now, many methods have been used for the synthesis of FeO-NPs. Mostly microorganisms and chemical methods have been used to synthesize FeO-NPs; however, the chemical methods have been considered as ecotoxic (Arslan et al., 2022). Therefore, the present study was aimed to choose biological methods using plant for the fabrication of FeO-NPs. This green process for NPs synthesis is non-toxic, eco-friendly, non-harmful for human health (Ghotekar et al., 2021; Priya et al., 2021). So far, this biotechnology became an important branch followed by nanotechnology (Mohanpuria et al., 2008). Biological synthesis is pretty cheap, an environmentally friendly and safe method (Latha et al., 2018). In biological synthesis, bacteria, fungi, algae, and different sources of plants can be used (Nanda, et al., 2018). For example, Potbhare et al. (2020a) fabricated silver nanoparticles (Ag NPs) on the surface of graphene oxide (GO) nanosheets as a multifunctional antibacterial material by using Pseudomonas aeruginosa biomass extract. Antibacterial action of the synthesized Ag-GO NPs was tested against bacterial species E. coli, Staphylococcus aureus, and Bacillus subtilis. The results revealed that Ag-GO NPs showed effective antibacterial performance against E. coli. In another study, Ag-NPs was synthesized by Rhizoctonia solani (R. solani) fungi with particle size of 10–20 nm (Potbhare et al., 2020b). AgNPs were found as an effective antibacterial agent against S. aureus. Chaudhary et al. (2021) demonstrated the fabrication of uniformly decorated zinc oxide (ZnO) on reduced graphene oxide (ZnO-rGO) nanocomposites using Sesbania bispinosa. The nanocomposites were used as photocatalyst for methylene blue (MB) dye-degradation and they supplied 85% dye degradation efficiency towards MB dye under UV–Visible light irradiation within 70 min.
In recent years, researchers have used various plant extracts to produce metal and metal oxide nanoparticles such as manganese dioxide (MnO2) (Hashem et al., 2018), zinc oxide (ZnO) (Suresh et al., 2015), iron oxides (FeO, Fe2O3, Fe3O4, FeOOH, Fe0 (Karunakaran et al., 2018), tin oxide (SnO2), lead oxide (PbO2), silver (Tavaf et al., 2015), gold, copper oxide (CuO), palladium (Kalaiselvi et al., 2015), cerium (IV) oxide, cerium dioxide, titanium oxide, ZnCuFe nanoparticle, cobalt ferrite (CoFe2O4), aluminum oxide (Al2O3), magnesium (Mg), and sulfur nanoparticles (Salem et al., 2016). Iron oxide has been one of the extensively investigated transition metal oxides due to its important variable oxidation states, low cost, environmental friendly nature, crystal structures, and magnetic properties (Devi et al., 2019).
Vermicomposting leachate can be also used for synthesis of nanoparticles. The digestion of organic material by earthworms results in the production of vermicomposting (Chaoui et al., 2003). Water is constantly sprayed during vermicomposting process on account of obtain the required moisture level in the reactor. Vermicomposting process also generates leachate due to the delivery of water by microorganisms throughout the separation of organic material (Gutiérrez-Miceli et al., 2008). The surplus water that leaches out is usually familiar as vermicomposting leachate or worm bed leachate. Vermicomposting leachate is very valuable and can be exploited liquid fertilizer due to its loud concentration of plant nutrition’s and the existence of fulvic and humic acids (Gutiérrez-Miceli et al., 2008). However, if the resulting leachate is discharged to the environment, it may reason pollution, exclusively if the site is close to the groundwater source (Katheem Kiyasudeen et al., 2016). Therefore, various methods should be developed for their evaluation.
The implementation of vermicomposting in the recycling of organic and animal wastes has acquired admission in the last two decades (Cambardella et al., 2003; Reynolds 2004). Studies on utilizing vermicomposting leachate focused on their fertilizer properties (Arancon et al., 2005; Gutiérrez-Miceli et al., 2011; Tharmaraj et al., 2011; Vasantharaj et al., 2017; García-Gómez et al., 2008). So far, different types of wastes such as cow manure, cow dung and green forages, vegetable waste have been utilized in studies of vermicomposting leachate.
In this study, we focused on the synthesis of FeO-NPs using the green approach and examined their DPPH activity, DNA cleavage ability, antimicrobial activity, cell viability inhibition, antimicrobial photodynamic therapy, and biofilm inhibition properties. As far as our knowledge, vermicomposting leachate has been used for the first time to synthesize FeO-NPs. The present work has highlighted the easy to scale up method for the fabrication of FeO-NPs and also contributed to the development of less expensive FeO-NPs in the field of antimicrobial activity. Moreover, the prepared FeO-NPs from vermicomposting leachate were characterized using SEM, EDX, and XRD.
Materials and Methods
Materials and Chemicals
Hydrochloric acid (HCl, ACS reagent, 37%), iron (III) chloride (FeCl3, reagent grade, 97%) and sodium hydroxide [NaOH, reagent grade, ≥98%, pellets (anhydrous)] were purchased from Sigma Aldrich. FeCl3 salt was utilized as the iron source. The utilized chemicals and reagents of this research were analytical grade. Distilled water used in all experiments was obtained from a two-stage Millipore Direct-Q3UV purification system.
Vermicomposting Leachate Production
Four test beds (40 cm height × 40 cm length × 25 cm depth) were used to obtain vermicomposting leachate. In order to protect the vermicomposting against the sun and rain, the experimental beds were carried out in an indoor environment with an average temperature of 20–25°C, which does not receive direct sunlight. Cow manure and fruit pulp were composted in a thermophilic environment for 2 months, with mechanically turned over every 15 days (Juarez et al., 2015). Cow dung and fruit pulp with 80% moisture content, after a 2-month composting period, worms (Eisenia fetida) were left in four test beds in the composted cow manure and fruit pulp. The composite leaks were obtained from the leachate collection tanks during the 2-month vermicomposting process.
Iron Oxide Nanoparticles Synthesis
The vermicomposting leachate collected from four beds was used by filtering through a funnel with a filter paper to remove the solids. The experimental steps for preparation of VCL-FeO-NPs is shown in Figure 1. 0.1 M FeCl3 (400 ml) was prepared and mixed with 100 ml vermicomposting leachate. The mixture was incubated (Microtest MCI 120 incubator) at 70°C for 24 h and the resulting precipitate was separated by centrifugation (Hettich Mikro 22R) at 6,000 rpm for 5 min. NPs was washed with double distilled water and ethanol for five times followed by drying in oven at 80°C.
Iron Oxide Nanoparticles Characterization
The SEM-EDX technique was used to characterize the synthesized FeO-NPs. The surface morphology of NPs was studied using scanning electron microscopy (SEM). (Germany’s Zeiss Supra 55). The composition of the elements presents on FeO-NPs surfaces was quantified using energy dispersive X-ray spectroscopy (EDX). The XRD measurements were carried out using via X-ray diffraction device (XRD, Bruker, D8 Venture) with 2θ scan range from 20 to 90° at a scan rate of 2°/min.
DPPH Activity
The DPPH activity was utilized to discover the antioxidant activity of VCL-FeO-NPs and the method was applied as previously reported by Salih Ağırtaş et al. (2015). Firstly, 250 µl of VCL-FeO-NPs prepared in five different concentrations (range from 12.5 to 200 mg/ml) and ascorbic acid and trolox used as standard were taken and put into test tubes. The 1,000 µl of DPPH methanol solution was then added to the test tubes, vortexed to form a homogeneous mixture, and then incubated in the dark for 30 min. Later, the absorbance was detected using spectrophotometer at 517 nm and the percent activity was calculated according to the formula below (1).
Abscontrol: absorbance value of Ascorbic acid and Trolox, Abssample: the absorbance value of the DPPH and test compounds.
DNA Cleavage Ability
Plasmid DNA was used to investigate the impact of newly synthesized VCL-FeO-NPs on DNA. Evaluation of the method was carried out by electrophoresis. The method is principally based on treating different concentrations of VCL-FeO-NPs with E. coli plasmid DNA. Briefly, prepared in three different concentrations of VCL-FeO-NPs (125, 250 and 500 mg/L) and 0.1 μg/μl supercoiled plasmid DNA were mixed and incubated at 37°C for 60 min. At the end of the time, loading dye was joined to the mixture and thoroughly blended and then the mixture was loaded onto agarose gel. Moreover, electrophoresis process was carried out at 120 V for 1 h. Later, the gel was lightened with UV lamp and photographed.
Antimicrobial Activity
The antimicrobial activity of the prepared VCL-FeO-NPs was verified using the standard microdilution method. To investigate the antibacterial activity of VCL-FeO-NPs, several types of Gram −ve (Pseudomonas aeruginosa, Escherichia coli, and Legionella pneumophila subsp. pneumophila), Gram +ve (Staphylococcus aureus, Enterococcus faecalis, and Enterococcus hirae) bacteria and yeast (Candida tropicalis, Candida parapisilosis) were utilized. The strains were grown overnight before microdilution experiment. Two-fold serial dilutions of VCL-FeO-NPs were done for the study. Later, the strains were added to the microplate-wells. Next plates left for 24 h incubation at 37 ± 2 C. MIC was described as the lowest concentration of VCL-FeO-NPs capable of inhibiting microbial growth up to 99%.
Bacterial Viability Inhibition Test and Antimicrobial Photodynamic Therapy
Cell viability inhibition and antimicrobial photodynamic therapy study was also performed to evaluate the inhibition activity of cellular viability of newly synthesized VCL-FeO-NPs. The strain used for this was a Gr −ve E. coli (ATCC 10536) strain. The preliminary preparation stage for the test procedure of E. coli was explained in detail in our previous study (Gonca et al., 2021). The prepared E. coli to be used in both bacterial viability inhibition and antibacterial photodynamic therapy studies. The prepared E. coli was treated with VCL-FeO-NPs. Then it was allowed to incubate for 60 min and when the incubation was finished, the dilution step and the cultivation step were performed and incubated at 37°C for 24 h. Finally, the colonies were counted. Finally, Inhibition percentages were calculated according to the Eq. 2 below by counting the colonies. In addition, in the antimicrobial photodynamic therapy study, the method in the microbial cell viability study was applied after the compounds were exposed to LED light for 20 min. Ultimately, the colonies were counted and the inhibition activity computed utilizing with Eq. 2.
Inhibition Activity of Biofilm Formation
Biofilm inhibition study was performed as another parameter to evaluate the antimicrobial activity of newly synthesized VCL-FeO-NPs. S. aureus (Gr +ve) and P. aeruginosa (Gr −ve) were used in the biofilm inhibition study. The details of the study are given in our previous study (Gonca et al., 2021). In summary, VCL-FeO-NPs was prepared at three different concentrations into a 24 well plate and then, the microorganisms were inoculated into the respective wells and incubated. The wells without VCL-FeO-NPs were used as a positive control wells. When incubation time was expired the wells were drained and washed distilled water. Afterwards, the plate was kept in an oven until dry. After the wells dried, crystal violet (CV) dye was added and left for 60 min. The CV was then removed and the plates were gently washed. Afterwards, ethanol was added to the wells and absorbance values were measured. Biofilm inhibition activity was computed in accordance with the Eq. 3 shown below
Results and Discussion
Characterization of Iron Oxide Nanoparticles
Morphological properties of FeO-NPs obtained from vermicomposting leachate are examined using SEM microscopy. It was observed that FeO-NPs induced are highly agglomerated with distinct particle sizes. The results also showed that FeO-NPs were an irregular and nonuniform semi-spherical shape (Figure 2). The rougher surface was observed, as well as an apparent sticking behavior due to the capping agent on the surface. SEM analysis depicted that FeO-NPs were smaller than 100 nm. It can be clearly seen that the peak of Fe was prominent among another element which indicates the presence of Fe in the NPs (Figure 3). Other elements like oxygen, carbon, and chlorine were also observed which indicated the effective role of organic materials as a capping agent. Similar results were reported by Aisida et al. (2021).
XRD technique was also exploited to obtain more detailed information about FeO-NPs crystallography. A series of predominant peaks observed in XRD were indexing the purity and crystalline nature of the synthesized FeO-NPs (Figure 4). The reflections in the given XRD pattern were identified as iron oxide. The XRD patterns matched with that of Fe-NPs synthesized using avocado fruit rind aqueous extract (Kamaraj et al., 2019).
DPPH Radical Scavenging Activity
As it is known, free radicals are necessary to kill microorganisms, activate enzymes and hormones, and produce energy. However, overproduction of free radicals causes many diseases related to oxidative stress such as oxidative stress-related neurological disorder, cardiac arrest, and cancer cell growth in humans. Antioxidants, which are called radical scavengers, normally regulate free radicals in the human body (Stephen Inbaraj and Chen, 2020). In this study, the DPPH method was used to investigate the free radical scavenging activity of FeO-NPs produced using compost leachate. The DPPH method is a commonly used technique for determining antioxidant activity. The color of the DPPH solution, which has a purple color, changes towards yellow in the presence of antioxidant substances in the environment. The DPPH activity results of VCL-FeO-NPs and also of ascorbic acid and trolox used as standard are shown in the Figure 5. DPPH activity results of newly synthesized VCL-FeO-NPs were 38.88%, 48.49%, 61.83%, 86.51%, and 93.54% at 12.5, 25, 50, 100, and 200 mg/L concentrations, respectively. DPPH activity results of Ascorbic acid and Trolox at the same concentrations were in the range from 84.12% to 100.00% and 86.39%–100.00%, respectively. Also, as can be clearly seen in the Figure 5, the free radical scavenging activity increased in parallel with the concentration. The free radical scavenging activity of the surface reaction between DPPH radical and VCL-FeO-NPs might be due to an electron shift in oxygen at the radical’s surface (Mirza et al., 2018). Antioxidant activities of FeO-NPs were also investigated in studies conducted by different researchers. Some of these studies are as follows. Chavan et al. (2020) synthesized FeO-NPs using Blumea eriantha DC extract (BEDC). They studied that of BEDC-FeO-NPs DPPH activity and reported that it was showed scavenging activity as 17.25 ± 1.19% at 50 μg ml−1 concentration. Dowlath et al. (2021) synthesized FeO-NPs in two ways including GS (green synthesis) and CS (chemical synthesis) and investigated the antioxidant activity of both FeO-NPs by DPPH method. They found that GS FeO-NPs and CS FeO-NPs showed 79.99 ± 0.92% and 15.11 ± 0.65% DPPH inhibition activity at 100 μg/ml concentration, respectively. Singh et al. (2020) used Coriandrum sativum leaf extract to synthesize iron oxide nanoparticles and reported that it showed DPPH activity with ranged from 32.54 to 84.28% at different concentrations (50–250 µg). Kumar et al. (2020) synthesized Citrus paradisi peel extract-mediated FeO-NPs and reported that scavenging activity of the CPPE-FeO-NPs was 26.52% at 100 μg the quantity of FeO-NPs. DPPH activity results obtained in the presented study were found to have better results compared to the above-mentioned studies. Therefore, FeO-NPs synthesized using compost leachate seem to be the good candidate for be antioxidant agent because of its good antioxidant potential.
DNA Cleavage Ability
The bio-efficiency of most antimicrobial and anticancer agents is frequently connected to its capability to interact with DNA. Such compounds can cause damage to the structure of DNA in cancer and microbial cells as well as induce cellular death by apoptosis/necrosis (El-ghamry et al., 2021) and these DNA damages may have been caused by single oxygen radicals (Chouke et al., 2022). One of the studies carried out in the presented study was the DNA cleavage study. For this, the effect of VCL-FeO-NPs on E. coli pBR 322 plasmid DNA was investigated by agarose gel electrophoresis method. To investigate the effect of VCL-FeO-NPs on plasmid DNA, DNA was treated with 3 different (125, 250, and 500 mg/L) concentrations of NPs. The results of the DNA cleavage study are given in the Figure 6. Possible results in DNA cleavage study are as follows; 1) Single strand break in plasmid DNA and transition from Form I to Form II. 2) Occurrence of double strand break in plasmid DNA and transition from Form I to Form III. 3) Another expected situation is the complete degradation of DNA by exposure to the substances (VCL-FeO-NPs for this study). When faced with the situation in the 3rd option, no band is observed in the gel because the DNA is divided into small pieces. In the presented study, there is no band in the Figure 6 because the DNA was completely fragmented. That is, the synthesized nanoparticle is highly effective on DNA and its effect on DNA is a very valuable result when considered from a pharmacological point of view. It has also been shown by studies that nanoparticles cause damage to DNA. El-ghamry et al. (2021) studied on nanoparticle-size metal complexes and showed its DNA cleavage ability. Gur et al. (2022) synthesized that biogenic zinc oxide nanoparticles and they showed that its DNA damage increases depending on the concentration. As a result, newly synthesized VCL-FeO-NPs can be used as a chemical nuclease agent after further studies.
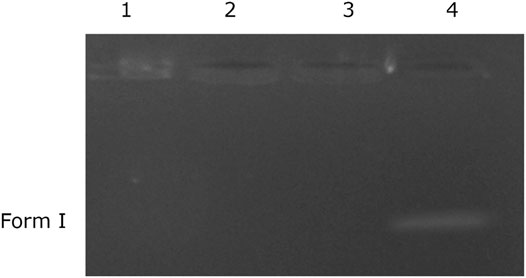
FIGURE 6. DNA cleavage of iron oxide nanoparticle. Lane 1, pBR 322 DNA + 125 mg/L of Fe nanoparticle; Lane 2, pBR 322 DNA + 250 mg/L Fe nanoparticle; Lane 3, pBR 322 DNA + 500 mg/L of Fe nanoparticle; Lane 4, pBR 322 DNA.
Antimicrobial Activity
In the present study, the antimicrobial capability of the synthesized VCL-FeO-NPs were investigated through microdilution processes. The antimicrobial activity results are given in Table 1. Ampicillin and Flucozanole used as controls for bacterial and fungal strains, respectively. VCL-FeO-NPs demonstrated lower antimicrobial activity than controls. Accordingly, the MIC values of the nanoparticle are 128 μg/ml against E. hirae and S. aureus and 256 μg/ml against E. coli, P. aeruginosa, L. pneumophila subsp. pneumophila, E. fecalis, C. parapisilosis, and C. tropicalis. According to our antimicrobial activity results, Gr +ve bacteria including E. hirae and S. aureus were found to be more sensitive to FeO-NPs. This situation can be explained as follows; because of that in Gr +ve bacteria formed of a thick peptidoglycan layer comparison to the Gram −ve bacteria is perhaps an effective degree of contact between nanoparticles and organisms due to its small size (Vitta et al., 2020). Previous reports also showed that higher antimicrobial activity of FeO-NPs on Gram +ve bacteria as compared to Gram −ve ones (Shaker Ardakani et al., 2021). Moreover, antimicrobial activities of iron nanoparticles against different microorganisms have been reported in various studies. Some of these studies are as follows; Salem et al. (2019) biosynthesized Fe3O4 NPs using aqueous extracts of seaweed Colpomenia sinuosa and Pterocladia capillacea and they reported that synthesized Fe3O4 NPs exhibited wide spectrum of antibacterial potency against the growth of Gram −ve and Gram +ve. Chavan et al. (2020) synthesized FeO-NPs using alcoholic Blumea eriantha DC plant extract, and they found that it showed antibacterial activity against S. aureus, B. subtilis, B. cereus and E. coli. Vasantharaj et al. (2019) synthesized FeO-NPs using Ruellia tuberosa leaf aqueous extract and studied their antimicrobial activity. They finally reported that while it exhibited better antimicrobial activity against E. coli and Klebsiella pneumoniae, it was less active against S. aureus. According to our antimicrobial activity results, it is seen that the synthesized NPs has an antimicrobial effect on the strains studied. Therefore, we think that it can be used in various fields as an antimicrobial active substance after further studies.
Biofilm Inhibition Activity
Multidrug resistance of microorganisms results is not only from structure transformation or gene changing of planktonic bacteria, but also from their ability to form biofilms. Combating biofilm formation is one of the important strategies in the development of new antibacterial agents (Srinivasan et al., 2021; Li et al., 2020). In this respect, nanoparticles may emerge as promising new effective substances. In the study, the biofilm inhibition activity of VCL-FeO-NPs was also investigated against S. aureus and P. aeruginosa. Biofilm inhibition activity results are given in Figure 7. Accordingly, as the concentration of VCL-FeO-NPs increased against both bacteria, the biofilm inhibition also increased. Biofilm inhibition activity results were 33.81%, 53.66%, and 66.05% for S. aureus and 41.11%, 55.50%, and 67.29% for P. aeruginosa at 125 mg/L, 250 mg/L, and 500 mg/L concentrations, respectively. The antimicrobial activity of metal and metal oxide NPs is thought to be mediated by the release of reactive oxygen species (ROS) and effective metal-ions from and its increased surface-to-volume ratios (Khalid et al., 2019). Antibiofilm effect of nanoparticles against various microorganisms has been demonstrated in many studies. Some of these studies are as follows; Salari et al. (2018) reported that Fe3O4-NPs inhibited biofilm formation of Candida spp. Kamble and Shinde (2018) reported that synthesized AgNPs using Curcuma longa showed potent anti-biofilm activity against S. aureus and S. pneumoniae. Pham et al. (2019) reported that synthesized and characterized iron NPs inhibited of biofilm formation of P. aeruginosa and this inhibition raised as the FeOOH-NP concentration increased. It seems that, these results ensure to anti-biofilm activity of a new synthesized VCL-FeO-NPs, contributing to the research for an efficient material to fight S. aureus and P. aeruginosa infections resulted from biofilm generation.
Bacterial Viability Effect and Antimicrobial Photodynamic Therapy
In the study, another method used to investigate the antimicrobial activity of CT-FeO-NPs were cell viability inhibition. E. coli bacteria were used for this in the method. The results of cell viability inhibition study are shown in the Figure 8. As seen Figure 8, cell viability inhibition results of VCL-FeO-NPs were 94.3%, 98.7%, and 99.99% at 125 mg/L, 250 mg/L, and 500 mg/L concentrations, respectively. According to our results, we think that the possible antimicrobial activity is similar to that of Fe2O3NPs. The Fe2O3 NPs breaks the bacterial cell membrane and consequently the potential of membrane turn into unsteady and the active transport collapsed. Contemporaneously, Fe2O3 NPs enter via the impaired membrane and inactivate the enzyme as well as disrupt the DNA, which conduces to death of the bacterial cell. (Ahmad et al., 2020). Also, antimicrobial photodynamic therapy study was performed by exposing the compounds prepared as mentioned above to LED light for 20 min (Figure 9). As a result, inhibition rates of antimicrobial photodynamic therapy were determined as 99.99%, 100%, and 100% at 125, 250, and 500 mg/L, respectively. The production of ROS, which damages the protein and DNA of bacteria, is another possible mechanism for the antimicrobial activity of iron NPs, and after exposure to light the antimicrobial effect may have occurred as mentioned (Vasantharaj et al., 2019; Vitta et al., 2020). In addition to these, it is well known that metal oxide nanoparticles can create the formation of ROS in microbial cells. These ROS molecules especially can cause deterioration of the membrane structure (Chaudhary et al., 2019). According to cell viability and antimicrobial photodynamic therapy results, newly synthesized FeO-NPs seems to be quite effective on E. coli bacteria. After further studies, we can say that NPs can be used as antimicrobial agent.
Conclusion
Vermicomposting leachate was used to synthesize FeO-NPs. The highest DPPH activities of VCL-FeO-NPs at 200 mg/L concentration were 93.54%. In addition, the nanoparticle showed significant DNA nuclease activity. The antimicrobial activity of VCL-FeO-NPs showed moderate antimicrobial activity against Gram positive, Gram negative, and fungi. However, the nanoparticles showed more effective microbial cell inhibition activity against E. coli. Also, biofilm inhibition results were detected against S. aureus and P. aeruginosa were 66.05% and 67.29%, respectively. It clearly indicated that green synthesized FeO-NPs showed good biological properties, which could be used to produce and formulate new drug and biomedical applications. In addition, we hope that the results of our study will inspire readers to explore new avenues for the synthesis of FeO-NPs of various morphologies for future antimicrobial applications in nanomedicine. Overall, these results suggest that green synthesis of FeO-NPs has potential as an operative, low-cost, and beneficial process for many scientific and technical applications in future, including environmental applications.
Data Availability Statement
The original contributions presented in the study are included in the article/supplementary material, further inquiries can be directed to the corresponding authors.
Author Contributions
HA, SG, ZI, SÖ, and MY: Concept, Data Curation, Investigation, Writing—Original Draft. ND, BD, GA: Conceptualization, Writing—Original Draft, Formal analysis, Review and; Editing.
Conflict of Interest
The authors declare that the research was conducted in the absence of any commercial or financial relationships that could be construed as a potential conflict of interest.
Publisher’s Note
All claims expressed in this article are solely those of the authors and do not necessarily represent those of their affiliated organizations, or those of the publisher, the editors and the reviewers. Any product that may be evaluated in this article, or claim that may be made by its manufacturer, is not guaranteed or endorsed by the publisher.
References
Ahmad, W., Khan, A. U., Shams, S., Qin, L., Yuan, Q., Ahmad, A., et al. (2020). Eco-Benign Approach to Synthesize Spherical Iron Oxide Nanoparticles: A New Insight in Photocatalytic and Biomedical Applications. J. Photochem. Photobiol. B Biol. 205, 111821. doi:10.1016/j.jphotobiol.2020.111821
Aisida, S. O., Ugwu, K., Akpa, P. A., Nwanya, A. C., Nwankwo, U., Bashir, A. K. H., et al. (2021). Synthesis and Characterization of Iron Oxide Nanoparticles Capped with Moringa oleifera: The Mechanisms of Formation Effects on the Optical, Structural, Magnetic and Morphological Properties. Mater. Today Proc. 36, 214–218. doi:10.1016/j.matpr.2020.03.167
Arancon, N. Q., Edwards, C. A., Bierman, P., Metzger, J. D., and Lucht, C. (2005). Effects of Vermicomposts Produced from Cattle Manure, Food Waste and Paper Waste on the Growth and Yield of Peppers in the Field. Pedobiologia 49, 297–306. doi:10.1016/j.pedobi.2005.02.001
Arslan, H., Eskikaya, O., Bilici, Z., Dizge, N., and Balakrishnan, D. (2022). Comparison of Cr(VI) Adsorption and Photocatalytic Reduction Efficiency Using Leonardite Powder. Chemosphere 300, 134492. doi:10.1016/j.chemosphere.2022.134492
Azarang, M., Shuhaimi, A., Yousefi, R., Moradi Golsheikh, A., ve Sookhakian, M., and Sookhakian, M. (2014). Synthesis and Characterization of ZnO NPs/Reduced Graphene Oxide Nanocomposite Prepared in Gelatin Medium as Highly Efficient Photo-Degradation of MB. Ceram. Int. 40, 10217–10221. doi:10.1016/j.ceramint.2014.02.109
Cambardella, C. A., Richard, T. L., and Russell, A. (2003). Compost Mineralization in Soil as a Function of Composting Process Conditions. Eur. J. Soil Biol. 39, 117–127. doi:10.1016/s1164-5563(03)00027-x
Chaoui, H. I., Zibilske, L. M., and Ohno, T. (2003). Effects of Earthworm Casts and Compost on Soil Microbial Activity and Plant Nutrient Availability. Soil Biol. Biochem. 35 (2), 295–302. doi:10.1016/s0038-0717(02)00279-1
Chaudhary, R. G., Bhusari, G. S., Tiple, A. D., Rai, A. R., Somkuvar, S. R., Potbhare, A. K., et al. (2019). Metal/Metal Oxide Nanoparticles: Toxicity, Applications, and Future Prospects. Curr. Pharm. Des. 25, 4013–4029. doi:10.2174/1381612825666191111091326
Chaudhary, R. G., Potbhare, A. K., Aziz, S. K. T., Umekar, M. S., Bhuyar, S. S., and Mondal, A. (2021). Phytochemically Fabricated Reduced Graphene Oxide-ZnO NCs by Sesbania bispinosa for Photocatalytic Performances. Mater. Today Proc. 36, 756–762. doi:10.1016/j.matpr.2020.05.821
Chavan, R. R., Bhinge, S. D., Bhutkar, M. A., Randive, D. S., Wadkar, G. H., Todkar, S. S., et al. (2020). Characterization, Antioxidant, Antimicrobial and Cytotoxic Activities of Green Synthesized Silver and Iron Nanoparticles Using Alcoholic Blumea eriantha DC Plant Extract. Mater. Today Commun. 24 (May), 101320. doi:10.1016/j.mtcomm.2020.101320
Chouke, P. B., Potbhare, A. K., Meshram, N. P., Rai, M. M., Dadure, K. M., Chaudhary, K., et al. (2022). Bioinspired NiO Nanospheres: Exploring In Vitro Toxicity Using Bm-17 and L. Rohita Liver Cells, DNA Degradation, Docking, and Proposed Vacuolization Mechanism. ACS Omega 7, 6869–6884. doi:10.1021/acsomega.1c06544
Devi, H. S., Boda, M. A., Shah, M. A., Parveen, S., and Wani, A. H. (2019). Green Synthesis of Iron Oxide Nanoparticles Using Platanus orientalis Leaf Extract for Antifungal Activity. Green Process Synth. 8, 38–45. doi:10.1515/gps-2017-0145
Dowlath, M. J. H., Musthafa, S. A., Mohamed Khalith, S. B., Varjani, S., Karuppannan, S. K., Ramanujam, G. M., et al. (2021). Comparison of Characteristics and Biocompatibility of Green Synthesized Iron Oxide Nanoparticles with Chemical Synthesized Nanoparticles. Environ. Res. 201 (June), 111585. doi:10.1016/j.envres.2021.111585
El-ghamry, M. A., Nassir, K. M., Elzawawi, F. M., Aziz, A. A. A., and Abu-El-Wafa, S. M. (2021). Novel Nanoparticle-Size Metal Complexes Derived from Acyclovir. Spectroscopic Characterization, Thermal Analysis, Antitumor Screening, and DNA Cleavage, as Well as 3D Modeling, Docking, and Electrical Conductivity Studies. J. Mol. Struct. 1235, 130235. doi:10.1016/j.molstruc.2021.130235
Fernández, J. G., Fernandez-Baldo, A. M., Berni, E., Cami, G., Duran, N., Raba, J., et al. (2016). Production of Silver Nanoparticles Using Yeasts and Evaluation of Their Antifungal Activity against Phyto Pathogenic Fungi. Process Biochem. 51 (9), 1306–1313. doi:10.1016/j.procbio.2016.05.021
García-Gómez, R. C., Dendooven, L., and Gutiérrez-Miceli, F. A. (2008). Vermicomposting Leachate (Worm Tea) as Liquid Fertilizer for Maize (Zea mays L.) Forage Production. Asian J. Plan. Sci. 7 (4), 360–367. doi:10.3923/ajps.2008.360.367
Ghotekar, S., Pansambal, S., Bilal, M., Pingale, S. S., and Oza, R. (2021). Environmentally Friendly Synthesis of Cr2O3 Nanoparticles: Characterization, Applications and Future Perspective ─ a Review. Case Stud. Chem. Environ. Eng. 3, 100089. doi:10.1016/j.cscee.2021.100089
Gonca, S., Arslan, H., Isik, Z., Özdemir, S., and Dizge, N. (2021). The Surface Modification of Ultrafiltration Membrane with Silver Nanoparticles Using Verbascum thapsus Leaf Extract Using Green Synthesis Phenomena. Surfaces Interfaces 26, 101291. doi:10.1016/j.surfin.2021.101291
Gur, T., Meydan, I., Seckin, H., Bekmezci, M., and Sen, F. (2022). Green Synthesis, Characterization and Bioactivity of Biogenic Zinc Oxide Nanoparticles. Environ. Res. 204, 111897. doi:10.1016/j.envres.2021.111897
Gutiérrez-Miceli, F. A., Llaven, M. A. O., Nazar, P. M., Sesma, B. R., Álvarez-Solís, J. D., and Dendooven, L. (2011). Optimization of Vermicompost and Worm-Bed Leachate for the Organic Cultivation of Radish. J. Plant Nutr. 34 (11), 1642–1653. doi:10.1080/01904167.2011.592561
Gutierrezmiceli, F., Garciagomez, R., Rinconrosales, R., Abudarchila, M., Mariaangela, O., Cruz, M., et al. (2008). Formulation of a Liquid Fertilizer for Sorghum (Sorghum bicolor (L.) Moench) Using Vermicompost Leachate. Bioresour. Technol. 99 (14), 6174–6180. doi:10.1016/j.biortech.2007.12.043
Hashem, A. M., Abuzeid, H., Kaus, M., Indris, S., Ehrenberg, H., Mauger, A., et al. (2018). Green Synthesis of Nanosized Manganese Dioxide as Positive Electrode for Lithium-Ion Batteries Using Lemon Juice and Citrus Peel. Electrochimica Acta 262, 74–81. doi:10.1016/j.electacta.2018.01.024
Juárez, A. L., González, A. R., Piña, N. R., González, R. G. G., Pacheco, I. T., Velázquez, R. V. O., et al. (2015). Vermicompost Leachate as a Supplement to Increase Tomato Fruit Quality. J. Soil Sci. Plant Nutr. 15 (1), 46–59. doi:10.4067/s0718-95162015005000005
Kakakhel, M. A., Wu, F., Sajjad, W., Zhang, Q., Khan, I., Ullah, K., et al. (2021). Long-Term Exposure to High-Concentration Silver Nanoparticles Induced Toxicity, Fatality, Bioaccumulation, and Histological Alteration in Fish (Cyprinus carpio). Environ. Sci. Eur. 33, 14. doi:10.1186/s12302-021-00453-7
Kalaiselvi, A., Roopan, S. M., Madhumitha, G., Ramalingam, C., and Elango, G. (2015). Synthesis and Characterization of Palladium Nanoparticles Using Catharanthus roseus Leaf Extract and its Application in the Photo-Catalytic Degradation. Spectrochimica Acta Part A Mol. Biomol. Spectrosc. 135, 116–119. doi:10.1016/j.saa.2014.07.010
Kamaraj, M., Kidane, T., Muluken, K. U., and Aravind, J. (2019). Biofabrication of Iron Oxide Nanoparticles as a Potential Photocatalyst for Dye Degradation with Antimicrobial Activity. Int. J. Environ. Sci. Technol. 16, 8305–8314. doi:10.1007/s13762-019-02402-7
Kamble, S. P., and Shinde, K. D. (2018). Anti-Biofilm Activity Against Gram-Positive Bacteria by Biologically Synthesized Silver Nanoparticles Using Curcuma longa. Pharm. Nanotechnol. 6 (3), 165–170. doi:10.2174/2211738506666180629142001
Karunakaran, S., Ramanujam, S., and Gurunathan, B. (2018). Green Synthesised Iron And Iron-Based Nanoparticle in Environmental and Biomedical Application: - A Review. IET nanobiotechnol. 12 (8), 1003–1008. doi:10.1049/iet-nbt.2018.5048
Katheem Kiyasudeen, S., Ibrahim, M. H., Quaik, S., and Ismail, S. A. (2016). Introduction to Organic Wastes and its Management. Appl. Environ. Sci. Eng. A Sustain. Future, 1–21. doi:10.1007/978-3-319-24708-3_1
Khalid, H. F., Tehseen, B., Sarwar, Y., Hussain, S. Z., Khan, W. S., Raza, Z. A., et al. (2019). Biosurfactant Coated Silver and Iron Oxide Nanoparticles with Enhanced Anti-Biofilm and Anti-Adhesive Properties. J. Hazard. Mater. 364, 441–448. doi:10.1016/j.jhazmat.2018.10.049
Kumar, B., Smita, K., Galeas, S., Sharma, V., Guerrero, V. H., Debut, A., et al. (2020). Characterization and Application of Biosynthesized Iron Oxide Nanoparticles Using Citrus paradisi Peel: A Sustainable Approach. Inorg. Chem. Commun. 119 (May), 108116. doi:10.1016/j.inoche.2020.108116
Latha, D., Sampurnam, S., Arulvasu, C., Prabu, P., Govindaraju, K., and ve Narayanan, V. (2018). Biosynthesis and Characterization of Gold Nanoparticle from Justicia adhatoda and its Catalytic Activity”, Mater. Today Proc., C. 5, Sayı 2, Ss. 8968–8972, Li W, Wei W, Wu X, Zhao Y, Dai H. The Antibacterial and Antibiofilm Activities of Mesoporous Hollow Fe3O4 Nanoparticles in an Alternating Magnetic Field. Biomater. Sci. 8 (16), 4492–4507. doi:10.1016/j.matpr.2017.12.337
Li, W., Wei, W., Wu, X., Zhao, Y., and Dai, H. (2020). The Antibacterial and Antibiofilm Activities of Mesoporous Hollow Fe3O4 Nanoparticles in an Alternating Magnetic Field. Biomater. Sci. 8 (16), 4492–4507. doi:10.1039/D0BM00673D
Mirza, A. U., Kareem, A., Nami, S. A. A., Khan, M. S., Rehman, S., Bhat, S. A., et al. (2018). Biogenic Synthesis of Iron Oxide Nanoparticles Using Agrewia optiva and Prunus persica Phyto Species: Characterization, Antibacterial and Antioxidant Activity. J. Photochem. Photobiol. B Biol. 185 (April), 262–274. doi:10.1016/j.jphotobiol.2018.06.009
Mohanpuria, P., Rana, N. K., and Yadav, S. K. (2008). Biosynthesis of Nanoparticles: Technological Concepts and Future Applications. J Nanopart. Res. 10, 507–517. doi:10.1007/s11051-007-9275-x
Nanda, A., Nayak, B. K., and Krishnamoorthy, M. (2018). Antimicrobial Properties of Biogenic Silver Nanoparticles Synthesized from Phylloplane Fungus, Aspergillus tamarii. Biocatal. Agric. Biotechnol. 16, 225–228. doi:10.1016/j.bcab.2018.08.002
Pham, D. T. N., Khan, F., Phan, T. T. V., Park, S.-k., Manivasagan, P., Oh, J., et al. (2019). Biofilm Inhibition, Modulation of Virulence and Motility Properties by FeOOH Nanoparticle in Pseudomonas aeruginosa. Braz J. Microbiol. 50 (3), 791–805. doi:10.1007/s42770-019-00108-z
Potbhare, A. K., Chaudhary, R. G., Chouke, P. B., Yerpude, S., Mondal, A., Sonkusare, V. N., et al. (2019). Phytosynthesis of Nearly Monodisperse CuO Nanospheres Using Phyllanthus reticulatus/Conyza bonariensis and its Antioxidant/Antibacterial Assays. Mater. Sci. Eng. C 99, 783–793. doi:10.1016/j.msec.2019.02.010
Potbhare, A. K., Umekar, M. S., Chouke, P. B., Bagade, M. B., Tarik Aziz, S. K., Abdala, A. A., et al. (2020a). Bioinspired Graphene-Based Silver Nanoparticles: Fabrication, Characterization and Antibacterial Activity. Mater. Today Proc. 29, 720–725. doi:10.1016/j.matpr.2020.04.212
Potbhare, A. K., Chouke, P. B., Mondal, A., Thakare, R. U., Mondal, S., Chaudhary, R. G., et al. (2020b). Rhizoctonia solani Assisted Biosynthesis of Silver Nanoparticles for Antibacterial Assay. Mater. Today Proc. 29, 939–945. doi:10.1016/j.matpr.2020.05.419
Priya, N., Kaur, K., and Sidhu, A. K. (2021). Green Synthesis: An Eco-Friendly Route for the Synthesis of Iron Oxide Nanoparticles. Front. Nanotechnol. 3, 655062. doi:10.3389/fnano.2021.655062
Reynolds, J. (2004). “The Status of Earthworm Biogeography, Diversity, and Taxonomy in North America Revisited with Glimpses into the Future,” in Earthworm Ecology, 2nd End. Editor C. A. Edwards (Boca Raton FL: CRC Press LLC), 63–74. doi:10.1201/9781420039719.ch4
Salari, S., Sadat Seddighi, N., and Ghasemi Nejad Almani, P. (2018). Evaluation of Biofilm Formation Ability in Different Candida Strains and Anti-Biofilm Effects of Fe3 O4 -NPs Compared with Fluconazole: An In Vitro Study. J. de Mycol. Médicale 28 (1), 23–28. doi:10.1016/j.mycmed.2018.02.007
Salem, N. M., Albanna, L. S., and Awwad, A. M. (2016). Green Synthesis of Sulfur Nanoparticles Using Punica granatum Peels and the Effects on the Growth of Tomato by Foliar Spray Applications. Environ. Nanotechnol. Monit. Manag. 6, 83–87. doi:10.1016/j.enmm.2016.06.006
Salem, D. M. S. A., Ismail, M. M., and Aly-Eldeen, M. A. (2019). Biogenic Synthesis and Antimicrobial Potency of Iron Oxide (Fe3O4) Nanoparticles Using Algae Harvested from the Mediterranean Sea, Egypt. Egypt. J. Aquatic Res. 45 (3), 197–204. doi:10.1016/j.ejar.2019.07.002
Salih Ağırtaş, M., Karataş, C., and Özdemir, S. (2015). Synthesis of Some Metallophthalocyanines with Dimethyl 5-(Phenoxy)-Isophthalate Substituents and Evaluation of Their Antioxidant-Antibacterial Activities. Spectrochimica Acta Part A Mol. Biomol. Spectrosc. 135, 20–24. doi:10.1016/j.saa.2014.06.139
Shaker Ardakani, L., Alimardani, V., Tamaddon, A. M., Amani, A. M., and Taghizadeh, S. (2021). Green Synthesis of Iron-Based Nanoparticles Using Chlorophytum comosum Leaf Extract: Methyl Orange Dye Degradation and Antimicrobial Properties. Heliyon 7 (2), e06159. doi:10.1016/j.heliyon.2021.e06159
Singh, K., Chopra, D. S., Singh, D., and Singh, N. (2020). Optimization and Ecofriendly Synthesis of Iron Oxide Nanoparticles as Potential Antioxidant. Arabian J. Chem. 13 (12), 9034–9046. doi:10.1016/j.arabjc.2020.10.025
Srinivasan, R., Santhakumari, S., Poonguzhali, P., Geetha, M., Dyavaiah, M., and Xiangmin, L. (2021). Bacterial Biofilm Inhibition: A Focused Review on Recent Therapeutic Strategies for Combating the Biofilm Mediated Infections. Front. Microbiol. 12, 676458. doi:10.3389/fmicb.2021.676458
Stephen Inbaraj, B., and Chen, B.-H. (2020). An Overview on Recent In Vivo Biological Application of Cerium Oxide Nanoparticles. Asian J. Pharm. Sci. 15, 558–575. doi:10.1016/j.ajps.2019.10.005
Suresh, D., Nethravathi, P. C., Rajanaika, H., Nagabhushana, H., Sharma, S. C., et al. (2015). Green Synthesis of Multifunctional Zinc Oxide (ZnO) Nanoparticles Using Cassia fistula Plant Extract and Their Photodegradative, Antioxidant and Antibacterial Activities. Mater. Sci. Semicond. Process. 31, 446–454. doi:10.1016/j.mssp.2014.12.023
Tavaf, Z., Tabatabaei, M., Khalafi-Nezhad, A., Panahi, F., and Hosseini, A. (2015). Green Synthesis of Silver Nanoparticles by Reduced Glycated Casein Adducts: Assessment of Their Antibacterial and Antioxidant Activity Against Streptococcus mutans. Eur. J. Integr. Med. 7, 294–302. doi:10.1016/j.eujim.2015.02.001
Tharmaraj, K., Ganesh, P., Kolanjinathan, K., Suresh Kumar, R., and Anandan, A. (2011). Influence of Vermicompost and Vermiwash on Physico Chemical Properties of Rice Cultivated Soil. Curr. Bot. 2 (3), 18–21.
Umekar, M. S., Bhusari, G. S., Potbhare, A. K., Mondal, A., Kapgate, B. P., Desimone, M. F., et al. (2021). Bioinspired Reduced Graphene Oxide Based Nanohybrids for Photocatalysis and Antibacterial Applications. Curr. Pharm. Biotechnol. 22, 1759–1781. doi:10.2174/1389201022666201231115826
Vasantharaj, K., Jerold, M., Deepanraj, B., Velan, M., and Sivasubramanian, V. (2017). Assessment of a Sulfidogenic System Utilizing Microalgal Biomass of Chlorella Pyrenoidosa as an Electron Donor: Taguchi Based Grey Relational Analysis. Int. J. Hydrogen Energy 42, 26545–26554. doi:10.1016/j.ijhydene.2017.08.001
Vasantharaj, S., Sathiyavimal, S., Senthilkumar, P., LewisOscar, F., and Pugazhendhi, A. (2019). Biosynthesis of Iron Oxide Nanoparticles Using Leaf Extract of Ruellia tuberosa: Antimicrobial Properties and Their Applications in Photocatalytic Degradation. J. Photochem. Photobiol. B Biol. 192, 74–82. doi:10.1016/j.jphotobiol.2018.12.025
Vitta, Y., Figueroa, M., Calderon, M., and Ciangherotti, C. (2020). Synthesis of Iron Nanoparticles from Aqueous Extract of Eucalyptus robusta Sm and Evaluation of Antioxidant and Antimicrobial Activity. Mater. Sci. Energy Technol. 3, 97–103. doi:10.1016/j.mset.2019.10.014
Yabalak, E., Mahmood Al-Nuaimy, M. N., Saleh, M., Isik, Z., Dizge, N., and Balakrishnan, D. (2022). Catalytic Efficiency of Raw and Hydrolyzed Eggshell in the Oxidation of Crystal Violet and Dye Bathing Wastewater by Thermally Activated Peroxide Oxidation Method. Environ. Res. 212, 113210. doi:10.1016/j.envres.2022.113210
Keywords: antimicrobial activity, antioxidant activity, biofilm inhibition, iron oxide nanoparticles, microbial cell viability, vermicomposting leachate
Citation: Arslan H, Gonca S, Isik Z, Özdemir S, Yalvac M, Dizge N, Deepanraj B and Ashraf GA (2022) Iron Oxide Nanoparticles Synthesis From Vermicomposting Leachate and its Antioxidant Activities. Front. Mater. 9:912066. doi: 10.3389/fmats.2022.912066
Received: 03 April 2022; Accepted: 02 May 2022;
Published: 16 June 2022.
Edited by:
Muhammad Aamer Mehmood, Government College University, Faisalabad, PakistanReviewed by:
Jebaraj Sargunam Azariah, Jubail Industrial College, Saudi ArabiaRatiram Gomaji Chaudhary, Seth Kesarimal Porwal College, India
Sumit Yadav, Universiti Malay, Malaysia
Copyright © 2022 Arslan, Gonca, Isik, Özdemir, Yalvac, Dizge, Deepanraj and Ashraf. This is an open-access article distributed under the terms of the Creative Commons Attribution License (CC BY). The use, distribution or reproduction in other forums is permitted, provided the original author(s) and the copyright owner(s) are credited and that the original publication in this journal is cited, in accordance with accepted academic practice. No use, distribution or reproduction is permitted which does not comply with these terms.
*Correspondence: Balakrishnan Deepanraj, YmFidWRlZXBhbkBnbWFpbC5jb20=; Ghulam Abbas Ashraf, Z2FfcGh5QHlhaG9vLmNvbQ==