- 1State Key Laboratory of High Performance Civil Engineering Materials, Nanjing, China
- 2Sobute New Materials Co. Ltd., Nanjing, China
- 3Jiangsu Research Institute of Building Science, Nanjing, China
- 4The Second Construction Co. LTD of China Construction Eighth Engineering Division, Nanjing, China
Integral hydrophobic treatment is the most effective method to prolong the service life of the concrete by resisting the transport of water containing aggressive ions. The hydrophobicity phenomenon of cementitious materials with carboxylic acid type admixture has been presented, and experimental studies, including water adsorption, amount of hydration products, and pore system, are conducted to reveal the hydrophobicity mechanism. It is reported that cementitious materials containing hydrophobic admixture present excellent water resistance ability, and the experimental results of nitrogen adsorption isotherm show that carboxylic acid type admixture addition increases pore volume and surface area of cement pastes. In addition, the fractal geometry theory is applied to reveal the relation between the pore structure and the permeability coefficient of cement pastes with a hydrophobic agent. In conclusion, the high tortuosity fractal dimension and the hydrophobicity of the pore surface result in water resistance when the cementitious system is mixed with a new type of hydrophobic admixture.
1 Introduction
Reinforced concrete composites have been proved to be the most suitable basic material for infrastructures in corrosive environments, including ocean, saline soil, and chemical plant, all containing erosion ions (Jakobsen et al., 2016). Generally, concrete presents porous material characteristics without hydrophobicity such that fluid could easily transport into the interior of concrete. When erosion fluid penetrates concrete, cementitious materials and steel reinforcement are attacked by chloride and sulfate ions in fluid, reducing the service life of structures. In view of this, resisting water with aggressive ions by modifying the permeability of concrete materials can be one of the most effective methods to improve the durability of the concrete structure (Tittarelli and Moriconi, 2011; Chen et al., 2019; Song et al., 2017; Liu et al., 2018; Song et al., 2019; Tittarelli and Moriconi, 2010; Wang et al., 1450; Dehghanpoor Abyaneh et al., 2013; Jin et al., 2020). The concrete modified by a hydrophobic agent to reduce the permeability attracts extensive attention, including the integral and surface modification method (Liu et al., 2017; Song et al., 2017; Li et al., 2019; Tonelli et al., 2019). Based on the observed phenomenon that the concrete matrix modified by surface treatment cannot resist erosion ion transportation after surface peels and cracks occur, the concrete matrix itself modified by a hydrophobic agent shows longer water resistance ability and better durability. It has been reported in different studies (Wang et al., 1450) that stearic salt, natural oils, and saline can be utilized as hydrophobic agents. In a recent study (Liu et al., 2019), the carboxylic acid polymer was synthesized to improve the water-resistance ability of concrete by integral to improve the durability of concrete but without revealing a clear mechanism.
There are some supposed theoretical models to analyze the hydrophobicity of concrete with wax emulsions or lattices (Rixom and Mailvaganam, 2001). It is generally considered that the pores generated after the hydration process were coated with a layer of hydrophobic molecular or coalesced emulsion particles. However, this proposed theory has not been proven further by experimental methods. When the hydrophobic admixture is added to the concrete matrix, the hydrophobic molecular will be inserted in hydration products (Li and Liu, 2018), which modifies water transportation channels. The characterization of a pore includes the pore volume, pore diameter, tortuosity, and the pore surface state.
The influences of a new hydrophobic admixture type on the hydration process and surface relaxation of pores (Zhang et al., 2021a) have been studied by experimental methods, showing that flaky carboxylic calcium crystals, which changed the water transportation channel and increased the water resistance, were generated in the middle of hydration products when various dosages of the hydrophobic admixture were added to cement pastes. The relaxation of the pore surface investigated by the NMR method further presents that the pore surface was not coated by a layer of hydrophobic molecular. Therefore, the increased water resistance ability of cementitious materials containing a new hydrophobic admixture type can be caused by changes in the pore type and tortuosity (Cheng et al., 2019; Cheng et al.,2020; Liu and Zhang, 2021). It is necessary to study pore characteristics and tortuosity of cementitious materials with a carboxylic acid hydrophobic agent to reveal the hydrophobicity mechanism.
This study used the carboxylic acid hydrophobic admixture to explore the pore characteristics of cementitious material. The experimental research containing water adsorption, hydration degree, and pore system is conducted, whereas the fractal geometry method is utilized to analyze the hydrophobicity mechanism of cementitious material.
2 Raw Materials and Experimental Program
2.1 Raw Materials
The cement pastes consist of the ordinary Portland cement provided by China United Cement Co., LTD. and deionized water. The oxide compositions and the mineral phases of cement measured by X-ray photoelectron spectroscopy and X-ray diffraction are presented in Table 1. The new hydrophobic agent type used in research is carboxylic acid salt, provided by Jiangsu Sobute New Materials Co., Ltd. The characteristics of hydrophobic admixture are shown by Liu et al. (2019).
2.2 Experimental Methods
2.2.1 Steady Water Transportation Property
According to the Chinese standard JC 474-2008, the mortar specimens mixed with different amounts of the hydrophobic agent were prepared. Cement pastes are prepared with a water-to-cement ratio of 0.35, which minimizes the bleeding effect. The steady water transportation properties were measured to preset the hydrophobicity of mortars.
2.2.2 XRD Analysis and Hydration Degree
In order to analyze and quantify the amounts of hydration products, cement pastes were prepared in a glass bottle, which holds the same ratio of water to cement with mortar because the water transportation channels exist in cement pastes. In addition, all hydration processes of paste specimens were ceased by soaking in isopropanol for 48 h. Samples were crushed in a ball mill and manually through an 80 μm square-mesh sieve after being placed in a vacuum drying oven for 48 h at 20°C. X-ray diffraction analysis (Bruker D8, Bruker) is utilized to quantify the hydration products combined with TOPAS 4.0 Rietveld analysis, scanning from 5 to 70° with a 0.02 o/s rate. The hydration degree was measured by the chemical-bound water method: the temperature was directly heated up to 105°C to exclude nonchemical bound water and then burn to1,050°C at a constant weight and the mass loss between 105°C and 1,050°C was the chemically bound water content.
2.2.3 Brunauer–Emmett–Teller Analysis
The cement paste specimens for nitrogen adsorption and desorption tests (Quadrasorb 2 MP, Anton Paar) were prepared by being grounded manually using a 150 and 80 μm square-mesh sieve after ball mill crush analyzer and tested at 77 K. The surface area was obtained from the adsorption isotherm ranging from 0.05 to 1.0 relative pressure using the BJH method.
3 Results and Discussions
3.1 Effect of Hydrophobic Admixture on Steady Water Transportation
The steady water transportation process mainly decides the durability of concrete in most service environments. The addition of the hydrophobic agent will affect the water transportation process, which can be used to evaluate their hydrophobicity ability. Figure 1 shows the influence of hydrophobic agent addition amount on the steady water transportation of mortars. It has been observed that mortars mixed with a hydrophobic agent show a significantly higher water-resistance ability than the reference sample without admixture. When the addition amount of hydrophobic admixture increases from 0% to 0.6%, the water adsorption rate decreases from 0.029%/min to 0.0092%/min. In addition, the water adsorption rate of mortars slightly reduces from 0.0082%/min to 0.0062%/min when the amount increases to 0.9% and 1.2%. It can be concluded that if more than 0.6% of the hydrophobic agent is added to the mortar, the water-resistance ability can improve by more than 70%, indicating that a small amount of the hydrophobic admixture can significantly enhance the water-resistance ability of cementitious materials. From this point of view, the addition of a new hydrophobic agent type can reduce the corrosion rate caused by erosion ions.
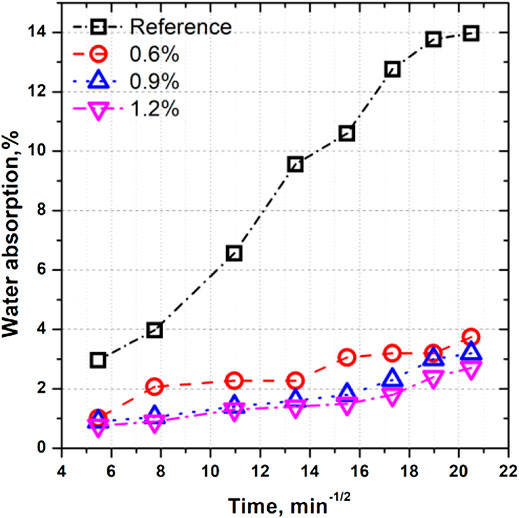
FIGURE 1. Steady water transportation process of mortars mixed with different amounts of hydrophobic agents.
3.2 Pore Structure of Cementitious Materials Containing a Hydrophobic Agent
Nitrogen sorption–desorption isotherms for cement pastes mixed with hydrophobic admixture at various relative pressure are shown in Figure 2. On the basis of previous results (Rouquerol et al., 2014), all of the nitrogen sorption–desorption isotherms for cement pastes show IV type curve with a hysteresis loop, which shows monolayer and multilayer adsorption in mesopores (2 nm < φ < 50 nm) and macropores (50 nm < φ < 200 nm), respectively. It should be noted that there is no limiting adsorption at high relative pressure (P/P0), suggesting that the samples are made of plate particles giving rise to slit pores (Sing, 1985; Shafaei et al., 2019). In Figure 2, samples added with hydrophobic admixture obviously present more nitrogen adsorbents adsorption ability than the reference sample. Similarly, the results also can be observed in the process of nitrogen desorption. Furthermore, the adsorbed quantity of nitrogen increases with the increase in the hydrophobic addition when P/P0 ranges from 0.05 to 1.0. It should be noted that the hysteresis loop shape shows little changes when various amounts of hydrophobic admixture are added.
Figure 3 reports cumulative pore volumes for cement pastes containing various dosages of hydrophobic admixture at 28 days. Generally, the cumulative pore volume of reference cement paste is lower than those containing various dosages of hydrophobic admixture. It can be inferred that the capillary pores will show a similar phenomenon corresponding to a higher porosity. More specifically, the reference cement paste exhibited 0.0225 cm3/g pore volume, whereas the samples incorporating 0.6%, 0.9%, and 1.2% of the hydrophobic agent showed 0.028, 0.029, and 0.031 cm3/g, exhibiting that carboxylic acid salt addition causes an increase in the porosity of cement pastes. The effect can be explained by the generation of the calcium carboxylate in the interior of the C-S-H gel, as proven by Zhang et al. (2021b). Calcium carboxylate crystals are generated in the pore solution at the moment of interaction between calcium ion and hydrophobic admixture, whereas the C-S-H gel is generated on the calcium carboxylate crystal surface. It should be noted that the amount of C-S-H is obviously larger than calcium carboxylate crystals, the calcium carboxylate crystals present the charging properties, and the hydration products are accumulated loosely. It can be seen in the results that inserting calcium carboxylate into C-S-H gel causes an increase in the pore volume.
Figure 4 shows the pore size distribution of samples containing various dosages of hydrophobic admixture. According to pore structure analysis (Jennings, 2000a; Juenger and Jennings, 2001; Shafaei et al., 2019), it can be observed that the size between 0.5 and 10 nm is the typical C-S-H gel pore. It is evident that hydrophobic admixture addition will increase the gel pore volume. Figure 4 shows that the highest pore volume distribution changed from 30 nm for reference neat cement paste to around 20 nm for cementitious materials containing various dosages of hydrophobic admixture. As shown in Figure 5, on the basis of the BJH pore calculation model, pore surface areas of samples with different amounts of carboxylic acid salt were 15.38, 19.54, 20.60, and 21.85 m2/g. It is similar that the pore volume of samples containing various dosages of the hydrophobic admixture shows the same trend with surface area. The total volume increases with the larger amount of the hydrophobic agent. It can be inferred that the hydrophobic admixture addition can lose the microstructure density of hydrated cementitious materials. However, the average pore diameter for the cement composite sample at 28 days exhibits a slightly different trend. The average pore diameter of samples decreases with hydrophobic admixture amount. It can be concluded that hydrophobic admixture addition could increase the porosity and reduce the pore diameter.
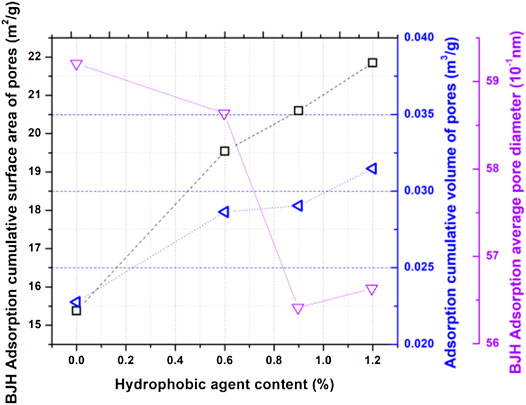
FIGURE 5. Effects of carboxylic acid salt addition amounts on the surface area of pores, volume of pores, and average pore diameter for cement composite samples.
3.3 Hydration Degree and Products of Cement Pastes Containing Hydrophobic Admixture
In order to analyze the hydrophobicity mechanism of cementitious materials with carboxylic acid hydrophobic admixture, the impacts of the hydrophobic agent on hydration products are studied. XRD patterns of cementitious materials mixed with different amounts of the hydrophobic agent are exhibited in Figure 6A, showing that the diffraction intensity of the reference sample is obviously similar to that of cementitious materials mixed with different amounts of the hydrophobic agent. It should be noted that the peak intensity of Ca(OH)2 and calcium carboxylate slightly increases at 19°. After the quantitative analysis of TOPAS, it can be founded in Figure 6B that crystal product amounts such as CH and Aft + AFm show similar results in reference samples and cement pastes containing hydrophobic. The C-S-H amounts in samples containing a hydrophobic agent increases slightly compared to reference samples. It can be concluded that the addition of a hydrophobic agent has little influence on the hydration products of cementitious materials.
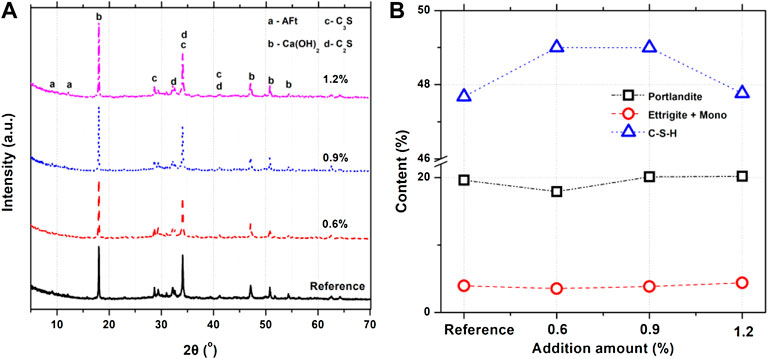
FIGURE 6. (A) XRD patterns and (B) comparison of three main hydration product amounts of cement composites containing various dosages of hydrophobic admixture.
3.4 Effect of Hydrophobic Agent on C-S-H Surface Area
According to experimental results of cement composite surface area obtained by nitrogen sorption isotherm, the main hydration products include high-density C-S-H and low-density C-S-H (abbreviated as HD C-S-H and LD C-S-H). When the spherical structure unit of C-S-H is considered, the high-density C-S-H is unseen by nitrogen, whereas the LD C-S-H can be seen. The C-S-H structure model proposed by Jennings (Jennings, 2000a; Jennings, 2000b) exhibits that very little amounts of gel pores in HD C-S-H are accessible to nitrogen adsorption. On the contrary, the pores in low-density C-S-H are available by the nitrogen sorption measurement. Based on this fact, The LD C-S-H mass ratio can be defined as follows:
where
However, the mass ratio of low-density calcium silicate hydrates to total gel weight can also be calculated by the other empirical formula as follows:
where
The hydration degree is obtained by the non-evaporable water experimental tests, whose results are shown in table 2. The hydration degree is calculated by dividing the non-evaporable water percent by the fully hydrated percent obtained from the reference study.

TABLE 2. Chemically bound water of hydrated cement pastes containing hydrophobic agent after 28 days.
Table 3 shows that the hydration degree is obtained by the ordinary heating method, and the real water-to-cement ratio is used in Eq. 2 to calculate the ratio of the low-density calcium silicate hydrate. In Eq. 1,

TABLE 3. Calculation results of calcium silicate hydrate properties of hydrated cement pastes containing hydrophobic agent after 28 days.
3.5 Pore Structure Fractal Characteristics of Cementitious Pastes Containing Hydrophobic Agent
The pore system is the channel of water transportation in the material. In order to clarify the influence of hydrophobic admixture on the permeability of the cementitious material, the pore system should be performed by theoretical analysis. Fractal geometry has been utilized to quantitatively characterize the geometric and structural properties of pore surfaces in cementitious materials (Pfeifer and Avnir, 1984). In the nitrogen adsorption isotherm, the nitrogen was adsorbed on the pore surface in a multilayer adsorption way. The single-probe method always obtains surface fractal dimension by the FHH theory (Frenkel, 1946; Hill, 1952; Jovanović, 1969). In the adsorption process of nitrogen in the cementitious material, nitrogen adsorption is determined by the external relative pressure. According to the theory proposed by Pfeifer et al. (Pfeifer and Cole, 1990; Pfeifer et al., 1991; Ismail and Pfeifer, 1994), the fractal dimension of cementitious can be calculated from the nitrogen sorption as follows:
where
The fractal characteristics of cementitious materials containing different dosages of hydrophobic admixture are investigated by the single-probe method. The plots of
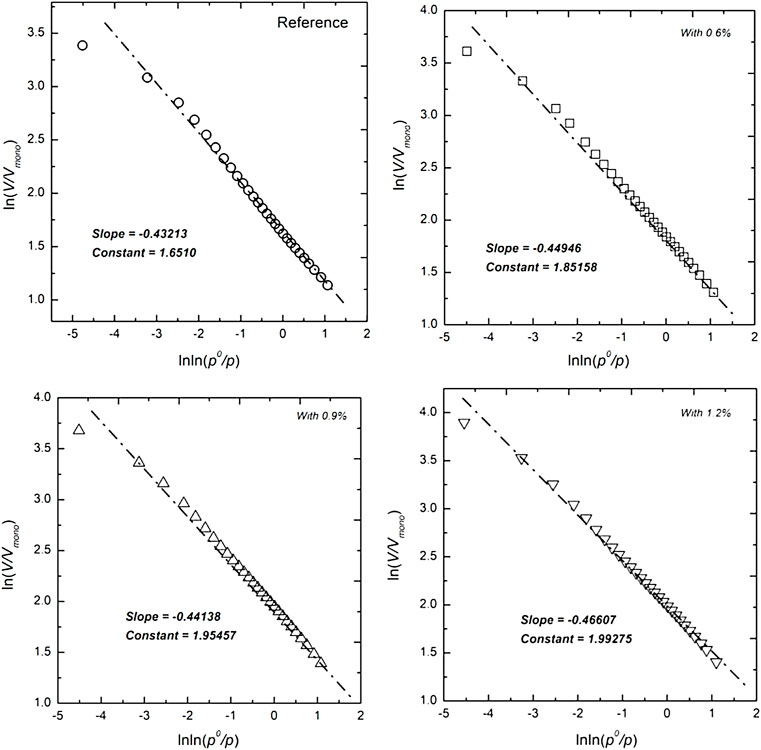
FIGURE 7. Reconstruction curves of ln(V/Vmono) versus lnln(po/p) from the nitrogen sorption isotherms from the cementitious materials containing different amounts of the hydrophobic agent.

TABLE 4. The fractal characteristics of hydrated cement pastes containing hydrophobic agent after 28 days.
3.6 The Relation Between Permeability and Water Adsorption
Assuming that a bundle of tortuous pores in cementitious material follows the fractal scaling law, the Hagen–Poiseuille equation and Darcy’s law are combined to derive the analytical formula for effective permeability k (Yu and Cheng, 2002) as follows:
where
In the cross-section, the pores are regarded as spheres with different diameters
Combining Eqs 7, 5,
It is difficult to measure the tortuosity fractal dimension in cementitious materials. The tortuosity fractal dimension is correlated to the surface area of low-density calcium silicate hydrates, and the tortuosity fractal dimension of the reference sample is estimated as 1.0 in this research, as shown in Table 4. The porosity is estimated by dividing the pore volume by the sample volume in the nitrogen adsorption measurement. The calculation results of effective permeability are shown in Table 4. Generally, it can be observed that hydrophobic agent addition reduces effective permeability. When the calculation results of effective permeability are compared with the water adsorption, it is shown in Figure 8 that the effective permeability is relatively consistent with water adsorption, but the difference in effective permeability between the sample with 0.6% hydrophobic agent and the reference sample is not obvious. Water adsorption ability of cementitious materials mixed with various dosages of carboxylic acid salts reduces to a 30% level of plain cement pastes. On the contrary, the effective permeability of cementitious materials containing different dosages of hydrophobic admixture just reduces to 80% of the reference sample.
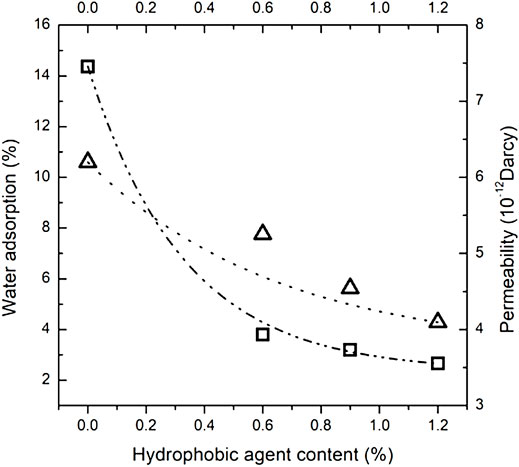
FIGURE 8. The tested water adsorption of mortars and the calculated permeability of cementitious materials with the same dosages of hydrophobic admixture.
According to the above discussion, the high tortuosity fractal dimension caused by surface area change of low-density C-S-H plays an important role in effective permeability. However, because inconsistency exists between effective permeability and water adsorption, the hydrophobic admixture addition reduces the irregularity and the roughness of the pore surface of cementitious materials. There must be another water resistance force in the pore. Zhang et al. (2021b) found that calcium carboxylate crystals are prior to generating in the cement pastes and adsorb on the surface of C-S-H. These products increase the contact angle reported by Zhang et al. (2021a), resulting in force at the entrance of the pores acting to keep water out.
4 Conclusion
The influence of carboxylic acid type hydrophobic agent on steady water transportation is studied by experiments. The hydrophobic admixture performs the excellent water repellence of mortars obviously increasing with hydrophobic agent addition amounts. In addition, the pore structure is investigated by nitrogen adsorption isotherm, and results show that the addition of hydrophobic admixture increases pore volume and surface area. Based on the C-S-H model and fractal geometry theory, the relation between pore structure and permeability of cement composite is analyzed. It is concluded that the high tortuosity fractal dimension caused by surface area change of low-density C-S-H plays a vital role in effective permeability and irregularity, and the roughness reduction of pore surface achieves hydrophobicity of cementitious materials (Hao et al., 2021).
Data Availability Statement
The datasets presented in this article are not readily available. Requests to access the datasets should be directed to bXVzb25nQGNuanNqay5jbg==.
Author Contributions
HZ: conceptualization, methodology, investigation, writing—original draft. SM: methodology, investigation, writing—review and editing, funding acquisition. JC: resources, methodology, investigation, writing—review and editing. JH: writing—review and editing, project administration. Wang Jiawei: investigation, writing—review and editing. YZ: investigation, writing—review and editing.
Conflict of Interest
HZ, YZ, SM, JC, JH, and JL were employed by Sobute New Materials Co. Ltd. YZ is employed by The Second Construction Co. LTD of China Construction Eighth Engineering Division.
The remaining author declares that the research was conducted in the absence of any commercial or financial relationships that could be construed as a potential conflict of interest.
Publisher’s Note
All claims expressed in this article are solely those of the authors and do not necessarily represent those of their affiliated organizations or those of the publisher, the editors, and the reviewers. Any product that may be evaluated in this article, or claim that may be made by its manufacturer, is not guaranteed or endorsed by the publisher.
Acknowledgments
We wish to acknowledge the financial support from the National Key R&D Program of China (no. 2021YFB2601000), National Natural Science Foundation of China (no. 5210081817), Key R&D Program of Guangdong Province (no. 2019B111106002), and Opening Project of State Key Laboratory of Green Building Materials (no. 2021GBM04).
References
Chen, J., Zhang, Y., Hou, D., Yu, J., Zhao, T., and Yin, B. (2019). Experiment and Molecular Dynamics Study on the Mechanism for Hydrophobic Impregnation in Cement-Based Materials: A Case of Octadecane Carboxylic Acid. Constr. Build. Mater. 229 (Dec.30), 116871. doi:10.1016/j.conbuildmat.2019.116871
Cheng, L. A., Wang, F., and Zhang, M. (2020). Modelling of 3D Microstructure and Effective Diffusivity of Fly Ash Blended Cement Paste. Cem. Concr. Compos., 110, 103586. doi:10.1016/j.cemconcomp.2020.103586
Cheng, L. A., Chen, Q. B., Rq, A., Zl, A., Hq, C., and Yz, C. (2019). Numerical Prediction of Effective Diffusivity in Hardened Cement Paste between Aggregates Using Different Shapes of Cement Powder. Constr. Build. Mat. 223, 806–816. doi:10.1016/j.conbuildmat.2019.06.125
Dehghanpoor Abyaneh, S., Wong, H. S., and Buenfeld, N. R. (2013). Modelling the Diffusivity of Mortar and Concrete Using a Three-Dimensional Mesostructure with Several Aggregate Shapes. Comput. Mater. Sci. 78 (5), 63–73. doi:10.1016/j.commatsci.2013.05.024
Hao, Z., Song, M., Jingshun, C., and Ruixing, C. (2021). The Impact of Carboxylic Acid Type Hydrophobic Agent on Compressive Strength of Cementitious Materials. Constr. Build. Mat. 291, 125264. doi:10.1016/j.conbuildmat.2021.123315
Hill, T. L. (1952). Theory of Physical Adsorption. Adv. Catal., 211–258. doi:10.1016/s0360-0564(08)60615-x
Ismail, I. M. K., and Pfeifer, P. (1994). Fractal Analysis and Surface Roughness of Nonporous Carbon Fibers and Carbon Blacks. Langmuir 10 (5), 1532–1538. doi:10.1021/la00017a035
Jakobsen, U. H., De Weerdt, K., and Geiker, M. R. (2016). Elemental Zonation in Marine Concrete. Cem. Concr. Res. 85, 12–27. doi:10.1016/j.cemconres.2016.02.006
Jaroniec, M. (1990). Evaluation of the Fractal Dimension of Microporous Activated Carbons. Fuel 69 (12), 1573–1574. doi:10.1016/0016-2361(90)90211-8
Jennings, H. M. (2000). A Model for the Microstructure of Calcium Silicate Hydrate in Cement Paste. Cem. Concr. Res. 30 (1), 101–116. doi:10.1016/s0008-8846(99)00209-4
Jennings, T. (2000). A Model for Two Types of Calcium Silicate Hydrate in the Microstructure of Portland Cement Pastes. Cem. Concr. Res. 30, 855–863. doi:10.1016/s0008-8846(99)00209-4
Jin, Z., Chang, H., Du, F., Zhao, T., Jiang, Y., and Chen, Y. (2020). Influence of SAP on the Chloride Penetration and Corrosion Behavior of Steel Bar in Concrete. Corros. Sci. 171, 108714. doi:10.1016/j.corsci.2020.108714
Jovanović, D. S. (1969). Physical Adsorption of Gases. Colloid Polym. Sci. 235 (1), 1203–1213. doi:10.1007/BF01542530
Juenger, M. C. G., and Jennings, H. M. (2001). The Use of Nitrogen Adsorption to Assess the Microstructure of Cement Paste. Cem. Concr. Resz.31(6):883-892. doi:10.1016/S0008-8846(01)00493-8
Li, F., and Liu, J. (2018). An Experimental Investigation of Hydration Mechanism of Cement with Silicane. Constr. Build. Mater. 166, 684–693. doi:10.1016/j.conbuildmat.2018.01.164
Li, Y., Gou, L., Wang, H., Wang, Y., Zhang, J., Li, N., et al. (2019). Fluorine-free Superhydrophobic Carbon-Based Coatings on the Concrete. Mater. Lett. 244 (JUN.1), 31–34. doi:10.1016/j.matlet.2019.01.149
Liu, C., and Zhang, M. (2021). Effect of Curing Temperature on Hydration, Microstructure and Ionic Diffusivity of Fly Ash Blended Cement Paste: A Modelling Study. Constr. Build. Mater. 297 (9), 123834. doi:10.1016/j.conbuildmat.2021.123834
Liu, J., Cai, J., Shi, L., Liu, J., Zhou, X., Mu, S., et al. (2018). The Inhibition Behavior of a Water-Soluble Silane for Reinforcing Steel in 3.5% NaCl Saturated Ca(OH)2 Solution. Constr. Build. Mat. 189, 95–101. doi:10.1016/j.conbuildmat.2018.08.151
Liu, J., Mu, S., Cai, J., and Jiang, Q. (2019). Preparation and Performance Evaluation of Cement Hydration Responsive Nanomaterial. J. Build. Struct. 40 (1), 181–187. doi:10.14006/j.jzjgxb.2019.01.021
Liu, P., Gao, Y., Wang, F., Yang, J., Yu, X., Zhang, W., et al. (2017). Superhydrophobic and Self-Cleaning Behavior of Portland Cement with Lotus-leaf-like Microstructure. J. Clean. Prod. 156 (jul.10), 775–785. doi:10.1016/j.jclepro.2017.03.211
Pfeifer, P., and Avnir, D. (1984). Chemistry in Noninteger Dimensions between Two and Three. I. Fractal Theory of Heterogeneous Surfaces. J. Chem. Phys. 80 (7), 3558–3562. doi:10.1063/1.447307
Pfeifer, P., and Cole, M. (1990). Fractals in Surface Science: Scattering and Thermodynamics of Adsorbed Films II New. J. de Physique I 14, 221–232. doi:10.1007/978-3-642-73902-6_10
Pfeifer, P., Kenntner, J., and Cole, M. W. (1991). Fundamentals of Adsorption.Springer US: Philadelphia, USA. doi:10.1007/978-1-4613-1375-5
Rixom, R., and Mailvaganam, N. P. (2001). Chemical Admixtures for Concrete, Cement, Concrete and Aggregates, Portland Cement Association: Skokie, IL, USA 23 (2), 86–98.
Rouquerol, F., Rouquerol, J., and Sing, K. (2014). Adsorption by Powders and Porous Solids. Amsterdam, Netherlands: Elseiver doi:10.1016/B978-0-12-598920-6.X5000-3
Shafaei, D., Yang, S., Berlouis, L., and Minto, J. (2019). Multiscale Pore Structure Analysis of Nano Titanium Dioxide Cement Mortar Composite. Mater. Today Commun. 22, 100779. doi:10.1016/j.mtcomm.2019.100779
Sing, K. Sw. (1985). Reporting Physisorption Data for Gas/solid Systems with Special Reference to the Determination of Surface Area and Porosity (Recommendations 1984). Pure Appl. Chem. 57 (4):603-619. doi:10.1351/pac198557040603
Song, J., Li, Y., Xu, W., Liu, H., and Lu, Y. (2019). Inexpensive and Non-fluorinated Superhydrophobic Concrete Coating for Anti-icing and Anti-corrosion. J. Colloid Interf. Sci. 541, 84–92. doi:10.1016/j.jcis.2019.01.014
Song, J., Zhao, D., Han, Z., Xu, W., Lu, Y., Liu, X., et al. (2017). Super-robust Superhydrophobic Concrete. J. Mat. Chem. A 5, 14542. doi:10.1039/c7ta03526h
Taylor, H.F. W., (1998). Cement Chemistry, Chemistry for Engineers, London, UK: Thomas Telford 2.doi:10.1142/9781860949982_0010
Tittarelli, F., and Moriconi, G. (2011). Comparison between Surface and Bulk Hydrophobic Treatment against Corrosion of Galvanized Reinforcing Steel in Concrete. Cem. Concr. Res. 41 (6), 609–614. doi:10.1016/j.cemconres.2011.03.011
Tittarelli, F., and Moriconi, G. (2010). The Effect of Silane-Based Hydrophobic Admixture on Corrosion of Galvanized Reinforcing Steel in Concrete. Corros. Sci. 52, 2958–2963. doi:10.1016/j.corsci.2010.05.008
Tonelli, M., Peppou-Chapman, S., Ridi, F., and Neto, C. (2019). Effect of Pore Size, Lubricant Viscosity, and Distribution on the Slippery Properties of Infused Cement Surfaces. J. Phys. Chem. C 123, 2987–2995. doi:10.1021/acs.jpcc.8b11221
Wang, F., Lei, S., Ou, J., and Li, W. Effect of PDMS on the Waterproofing Performance and Corrosion Resistance of Cement Mortar. Appl. Surf. Sci. 507, 145016. doi:10.1016/j.apsusc.2019.145016
Yu, B., and Cheng, P. (2002). A Fractal Permeability Model for Bi-dispersed Porous Media. Int. J. Heat Mass Transf. 45 (14), 2983–2993. doi:10.1016/s0017-9310(02)00014-5
Zhang, H., Mu, S., Cai, J., and Chen, R. (2021). The Impact of Carboxylic Acid Type Hydrophobic Agent on Compressive Strength of Cementitious Materials. Constr. Build. Mater. 291, 123315. doi:10.1016/j.conbuildmat.2021.123315
Keywords: hydrophobicity, pore structure, fractal geometry, permeability, cement paste
Citation: Zhang H, Zhou Y, Mu S, Cai J, Hong J, Liu J and Zhao Y (2022) Pore Structure and Permeability of Cementitious Materials Containing a Carboxylic Acid Type Hydrophobic Agent. Front. Mater. 9:907638. doi: 10.3389/fmats.2022.907638
Received: 31 March 2022; Accepted: 26 April 2022;
Published: 14 June 2022.
Edited by:
Xiangming Zhou, Brunel University London, United KingdomReviewed by:
Peng Zhang, National Natural Science Foundation of China, ChinaZhiyong Liu, Southeast University, China
Copyright © 2022 Zhang, Zhou, Mu, Cai, Hong, Liu and Zhao. This is an open-access article distributed under the terms of the Creative Commons Attribution License (CC BY). The use, distribution or reproduction in other forums is permitted, provided the original author(s) and the copyright owner(s) are credited and that the original publication in this journal is cited, in accordance with accepted academic practice. No use, distribution or reproduction is permitted which does not comply with these terms.
*Correspondence: Song Mu, bXVzb25nQGNuanNqay5jbg==; Hao Zhang, emhhbmdoYW9AY25qc2prLmNu