- 1Guangxi Beitou Transportation Maintenance Technology Group Co.,LTD, Nanning, China
- 2Guangxi Transportation Science and Technology Group CO., LTD., Nanning, China
The high-temperature rheological properties of rubberized asphalt and mixture were evaluated by frequency scanning, repeated creep recovery (RCR) test, temperature sweep test, Hamburg wheel tracking test (HWTT). Based on the Pearson correlation coefficient, the correlation of the mixture gradation and asphalt characteristics with the high-temperature stability of the mixture was analyzed. Finally, the gray correlation theory was applied to analyze the evaluation indexes of the high-temperature rheological properties of rubberized asphalt and mixture. The results show that the asphalt-stone ratio and the fractal dimension (Dc) of coarse aggregate have a significant correlation with the high-temperature performance of the mixture, and the mixture with a smaller asphalt-stone ratio and a higher percentage of coarse aggregate has a better high-temperature performance. The correlation degree of softening point, viscous stiffness modulus and permanent deformation with rubber-asphalt mixture is higher than 0.7, and are significantly higher than those of rotational viscosity at 180°C. Therefore, we recommend the use of permanent deformation, softening point, and viscous stiffness modulus to evaluate the high-temperature performance of rubberized asphalt mixture.
1 Introduction
As a mature environmentally-friendly pavement material, rubber modified asphalt has been widely used in China for decades. It shows advantages in noise reduction and skid resistance and boosts excellent high and low temperature performances, especially high-temperature performance, and anti-fatigue property (He et al., 2014; Zhou et al., 2014; Ma et al., 2021). At present, the evaluation indexes for the high-temperature performance of rubber modified asphalt are still similar to those for matrix asphalt and conventional polymer modified asphalt. South Africa, the United States, and Chile all use rotational viscosity at 177 °C (He, 2019) and China adopts rotational viscosity at 180 °C stipulated in crumb rubber modified Asphalt for Highway Engineering (JT/T 798–2019) (Industry standards of Transportation Department of the People ' s Republic of China, 2011a). However, 177°C–180°C is a range of mixing temperature during construction and does not fit the actual pavement operating temperature. When the rubberized asphalt is at 180°C, a solid-liquid two-phase separation state is formed inside, which is quite different from the phase structure of rubberized asphalt in the working temperature range. Therefore, it is difficult to evaluate the high-temperature rutting resistance of the road surface. In addition, in the high-content rubber modified asphalt, the rotor movement may be blocked due to rubber powder accumulation, resulting in large result variability (He, 2019). To sum up, it is necessary to compare the evaluation indexes of high-temperature performance of rubber modified asphalt and propose an evaluation index that is highly correlated to the rutting resistance of the road surface to guide the construction.
At present, high-temperature performance evaluation indexes of rubberized asphalt have been extensively studied. Navarro (Navarro et al., 2005) studied the high-temperature performance of rubberized asphalt through dynamic shear rheometer (DSR) frequency scanning and rotational viscosity test and found that mixing rubber powder could significantly improve the temperature sensitivity and then the high-temperature performance of asphalt. Ding et al. (2019) and Hu et al. (2022) studied the performance of recycled asphalt concrete with stable crumb rubberized asphalt (SCRA) binder and foamed warm mix asphalt with crumb rubber, and found that the high and low temperature properties of the test samples were significantly improved after adding rubber. Shenoy (2001) concluded that the DSR rutting factor proposed in the SHRP plan was not an applicable index for grading evaluation of modified asphalts after high-temperature performance research on different polymer modified asphalts. They proposed an improved rutting factor G∗(sinδ)−9 to avoid the influence of the small phase angle of the modified asphalt on its high-temperature performance. Yang et al. (2010) studied the correlation between the dynamic stability of rubberized asphalt mixture and indicators of the corresponding modified asphalt, such as the rotational viscosity at 177°C, softening point, and penetration. It was found that the rotational viscosity at 177°C of the modified asphalt was significantly correlated to the dynamic stability of the mixture. Hu et al. (2018) tested the four components of different rubber modified asphalt based on multivariate regression analysis and gray correlation analysis to analyze the correlation between the proportion of the four components and each index. The results showed that the 60°C complex elastic modulus and the zero shear viscosity (ZSV) obtained by the DSR testing had a correlation coefficient as high as 0.99 with four components of asphalt. Li et al. (2015) studied the influence of rubber powder content and matrix asphalt type on the performance of rubber modified asphalt through conventional performance tests and DSR testing. Li also analyzed the applicability of evaluation indexes and concluded that penetration degree is not appropriate for the performance evaluation of rubber modified asphalt while the softening point is suitable. Huang et al. (2010) investigated the influence of rubber powder property, asphalt-stone ratio, porosity, etc., on the high-temperature performance of mixture through the wheel rutting test and found that rubber powder content and asphalt-stone ratio had more significant impacts.
In previous studies, various indexes were used to evaluate the high-temperature performance of rubberized asphalt and mixture. However, consistent results of asphalt and mixture have rarely been reported, making it difficult to characterize the high-temperature performance of actual road surfaces with asphalt evaluation indexes used in engineering. Therefore, in this paper, rubberized asphalt and mixtures with different viscosity grades were prepared based on the rotational viscosity at 180°C and their high-temperature rheological properties were analyzed using frequency scanning, repeated creep recovery (RCR), and temperature sweep tests. Furthermore, the correlation between indicators, including the fractal dimension of rubberized asphalt and mixture and high-temperature stability of three types of asphalt mixtures (SMA, ARAC, and AC) was analyzed by Pearson correlation. Finally, the gray correlation degree of the high-temperature performance evaluation index of asphalt and mixture was analyzed. It is expected that this study can guide the selection of reasonable evaluation indexes for rubberized asphalt pavement construction in the future.
2 Raw Material and Tests
2.1 Raw Materials
The rubberized asphalt with different viscosity grades (about 1 Pa·s, 3 Pa·s, 5 Pa·s, and 7 Pa·s) was prepared according to rotational viscosity at 180°C. Esso matrix asphalt and Maoming matrix asphalt (referred to as A and B) were selected as raw materials, and 30–100 mesh rubber powder under different dosing regimens was used as the modifier. For simplification, asphalt samples were expressed in the form of asphalt type-rubber powder content-rotational viscosity at 180°C. For example, A-20%-1 represents the Esso modified rubberized asphalt with a rubber powder content of 20% and a rotational viscosity of 1 Pa·s at 180°C. The performance indexes of raw materials, rubber powder, and modified asphalt are shown in Tables 1–3.
2.2 Test Methods
2.2.1 Rheological Property Test
In this paper, the Bohlin cvo100D-ADS type DSR produced by Malvern, United Kingdom, was used to study the rheological properties of asphalt samples. The test temperature range of this instrument is 5°C–95 °C, the frequency range is 10 μHz–100 Hz, and the shear rate range is 0.1 μrad/s-320 rad/s, and the strain range is 1%–100%. In this paper, frequency scanning, temperature sweep, and RCR tests were conducted to evaluate the rheological properties.
2.2.1.1 Frequency Scanning Test.
The DSR frequency scanning was performed to explore the stress-resistant behavior of asphalt when vehicle loads were moving on the asphalt pavement at different speeds. In this paper, the change of complex viscosity with stress frequency from 0.001 to 10 Hz at 60°C was used to indicate the anti-deformation ability of rubber modified asphalt. Additionally, based on the Carreau model and the Cross model (He, 2019), ZSV of different asphalt samples was derived for subsequent correlation evaluation of asphalt indexes.
2.2.1.2 Repeated Creep Recovery Test.
The RCR test was proposed by Bahia et al. (2001). It aims to simulate the behavior of asphalt in the development of permanent deformation of the mixture when the pavement is under the traffic load. In this paper, the DSR instrument was applied to load the sample for 1 s and unload the sample for 9 s under a stress of 30 Pa and the cycle was repeated 100 times. The stress change of the sample was recorded. Finally, the Burgers model (Lin and Fan, 2018) was used to fit the obtained stress and deformation data, and the viscous stiffness modulus (
2.2.1.3 Temperature Sweep Test.
The rutting factor G∗/sinδ in the DSR temperature sweep is one of the important indexes to evaluate the high-temperature performance of asphalt. Since the phase angle of rubber modified asphalt has a low phase angle, its temperature sweep test was carried out at 64°C–82°C. Furthermore, the improved rutting factor G∗(sinδ)−9 based on the study of Shenoy (2001) was compared with the conventional rutting factor.
2.2.2 Hamburg Wheel Tracking Test
Hamburg wheel tracking test (HWTT) reflects the high-temperature rutting resistance of asphalt mixture through the stress-strain behavior of the mixture sample against deformation under repeated load. The rutting deformation rate (RDr) and the creep slope (CS) were used to evaluate the results of the HWTT. The physical meaning of RDr is the amount of rutting deformation per hour and a larger RDr indicates worse high-temperature rutting resistance. The RDr index includes the maximum rutting depth, which can compare the high-temperature rutting resistance among asphalt mixtures simply and effectively. CS represents the rutting development speed, which is reflected in the rut development curve as the reciprocal of the slope in a certain stage, and its physical meaning is the number of loadings to produce 1 mm deformation.
3 Test Results
3.1 Rheological Property Test Results
3.1.1 Frequency Scanning Test Results
The relationship between frequency and complex viscosity obtained by frequency scanning of different rubber modified asphalt samples at 60 °C is shown in Figure 1.
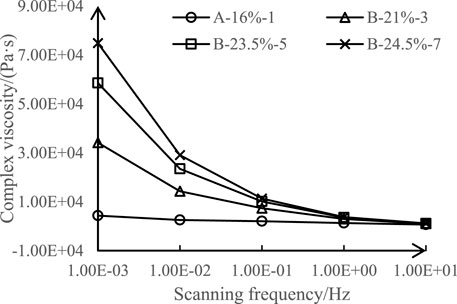
FIGURE 1. The relationship between frequency and complex viscosity obtained by frequency scanning of different rubber modified asphalt samples.
As shown in Figure 1, the increase of the complex viscosity gradually slows down with the progressive increase in rotational viscosity. When the frequency is 0.001 Hz, the complex viscosity of the B-21%-3 sample is 686.4% higher than that of the A-16%-1 sample, while the complex viscosity of the B-24.5%-7 sample is only 27.8% higher than that of the B-23.5%-5 sample. However, the gap decreases significantly as the scanning frequency increases. When the scanning frequency is 10 Hz, the complex viscosity of the B-21%-3 sample is 56.9% higher than that of the A-16%-1 sample, while the complex viscosity of the B-24.5%-7 sample is only 6.4% higher than that of the B-23.5%-5 sample. It is shown that under low-frequency stress scanning, the complex viscosity and the rotational viscosity have large evaluation differences.
Since rubberized asphalt is easily affected by stress frequency, its viscosity evaluation index is also susceptible to the interference of stress frequency. In this paper, ZSV derived based on the Carreau model and the Cross model (He, 2019) was adopted as an evaluation index of rubberized asphalt. The variation of ZSV of different modified asphalt samples is shown in Figure 2.
ZSV increases with the progressive increase of rotational viscosity. The linear fitting shows that the correlation coefficient between ZSV derived based on the two models and rotational viscosity are 0.9866 and 0.9955, respectively, indicating strong correlations. Furthermore, the fitting slope of the Carreau model is greater than that of the Cross model, indicating that the Carreau model is more sensitive to asphalt viscosity changes.
3.1.2 Repeated Creep Recovery Test Results
The trends of the viscous stiffness modulus (
It can be seen from Figure 3 that as the rotation viscosity progressively increases, the viscous stiffness modulus (
3.1.3 Temperature Sweep Test
In this paper, the rutting factor G∗/sinδ and the improved rutting factor G∗(sinδ)−9 were used to evaluate the asphalt samples. The results are shown in Figure 4.
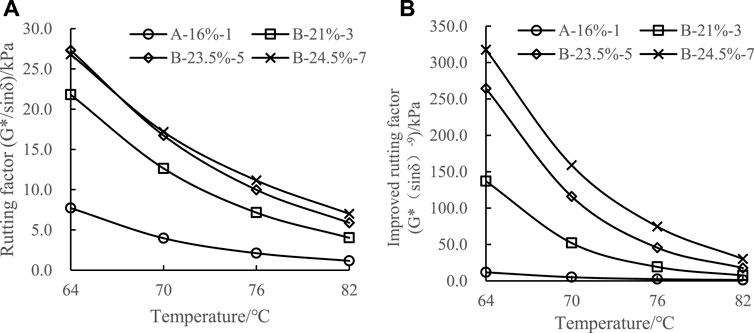
FIGURE 4. The trend of two rutting factors of different modified asphalt. (A) Conventional rutting factor (B) Improved rutting factor.
As shown in Figure 4, the basic trends of the two indexes are the same. However, the difference among improved rutting factor G∗(sinδ)−9 is larger than that among conventional rutting factor G∗/sinδ. The temperature sweep curves of B-24.5%-7 and B-23.5%-5 in Figure 4A almost overlap while curves in Figure 4B do not. With the rising temperature, the decrease in the improved rutting factor G∗(sinδ)−9 is more significant than that of the conventional rutting factor G∗/sinδ. For example, the improved rutting factor of B-24.5%-7 sample at 82°C decreases by 95.8% compared with that at 64°C. In contrast, the conventional rutting factor G∗/sinδ decreases by 83.4%, suggesting that the improved rutting factor G∗(sinδ)−9 is more sensitive to changes in temperature and asphalt rotational viscosity.
3.2 Hamburg Wheel Tracking Test
In this paper, RDr and CS were adopted to evaluate the high-temperature rutting resistance of different modified rubberized asphalt mixtures with three gradations (SMA-13, ARAC-13, and AC-13). The sieve passing rates of these gradations are shown in Table 4, and the performance index of the mixture is shown in Table 5.
4 Test Result Analysis
4.1 Correlation Analysis of Factors Affecting the High-Temperature Performance of the Mixture
4.1.1 Analysis of Gradation Characteristics Based on Fractal Theory
The correlations between factors including gradation type, rotational viscosity, rubber powder content, asphalt-stone ratio, and porosity, and two indexes (RDr and CS) in HWTT were analyzed. In this way, the influencing factors for the high-temperature performance of the mixture were ranked to guide the engineering construction. For the simplicity of the characteristics analysis of the overall gradation and coarse and fine gradations, the gradation was described based on the fractal dimension theory. Fractal dimension theory is an emerging subject that quantitatively describes the complexity and space-filling capacity of geometry. In addition, the theory can describe the asphalt mixtures, which are characterized by heterogeneity, nonlinearity, irregularity, and ambiguity, especially aggregate gradation with self-similarity (Yang et al., 2006).
The double logarithmic scatter plots of three gradation curves are drawn based on the fractal theory, as shown in Figure 5. The least square method was applied to perform linear regression to obtain the overall fractal dimension (D) of the gradation. Then, only the parts of the gradation curve with a value above or below 4.75 mm were taken to calculate the gradation fractal dimensions of coarse and fine aggregates (Dc and Df), respectively. The fractal indexes of the three degradations are shown in Table 6, and the changing trend is shown in Figure 6.
1) R2 in the regression equation represents the fitting degree between the actual gradation and the gradation modeled based on the fractal theory. Figure 6 shows that the fractal characteristics of the three types of gradation are all strongly correlated. AC has the strongest correlation, and SMA has the weakest correlation. This phenomenon is because SMA gradation is discontinuous and its continuous granular material system with fractal characteristics breaks into multiple small fractal systems due to the lack of aggregates in a certain particle size range.
2) Figure 6 shows that the D and Df values of SMA are higher than those of ARAC and AC, which are continuous gradations. Figure 6 and the aggregate proportion of each gradation in Table 5 indicate that as the proportion of the coarse aggregate in the gradation increases, the D and Df values increase while the Dc value decreases. This result suggests that the fractal dimension can effectively characterize the difference in the proportion of particle size of different gradations. Therefore, in the following part, the Pearson correlation analysis between the characteristics of the overall gradation and coarse and fine aggregates represented by D, Df, and Dc, and the rutting performance indexes of the mixture in HWTT were conducted.
4.1.2 Correlation Analysis
The Pearson correlation analysis between fractal dimensions, rubber powder content, asphalt-stone ratio, and porosity of the three gradation types and RDr and CS obtained from HWTT was performed. The results are shown in Table 7.
It can be seen from Table 7 that.
1) Among the influencing factors, asphalt-stone ratio, porosity, and Df are all positively correlated with RDr and CS, indicating that the larger the three factors, the worse the high-temperature performance of the mixture. While the other influencing factors are all negatively correlated with the two indexes in HWTT, indicating that when their values are large, the high-temperature performance of the mixture is excellent.
2) The asphalt-stone ratio and Dc have significant effects on the high-temperature performance of the mixture, and the absolute values of the correlation coefficients are higher than 0.7. The remaining factors in the order of influence are the rubber powder content, rotational viscosity at 180°C, porosity, D, and Df. The asphalt-stone ratio of the mixture needs to be determined by aggregate characteristics and gradation characteristics together and cannot be controlled artificially. Therefore, to improve the high-temperature performance of the mixture, the priority is controlling the proportion of the coarse aggregate in the gradation. Then the rubber powder content needs to be controlled to enhance the asphalt viscosity.
4.2 Gray Correlation Analysis for Performance Index of Asphalt and Index of Hamburg Wheel Tracking Test
4.2.1 Calculation Method for Gray Correlation Analysis
The gray correlation analysis of indexes of general performance and rheological performance and the indicators of HWTT was performed to obtain the index which has the strongest correlation with the indicator.
Gray correlation analysis theory (Tan and Deng, 1995), proposed by Chinese scholar Prof. Deng Julong in 1982, is an important part of gray system theory. It has been widely used in many scientific research fields. The basic idea of gray correlation analysis theory is to judge whether the relationship is close according to the similarity of the geometry of the sequence curve. Curves close to each other indicate a strong correlation between the corresponding sequences. Gray relational analysis theory is applicable whether the sample size has regularity or not. The calculation is easy, and the quantitative results agree with the qualitative analysis results. In practical applications, the degree of the influence of factors on the results is generally represented by the gray correlation degree. A value closer to 1 indicates a higher influence degree. The calculation process is as follows:
1 The reference sequence that characterizes the system (i.e., the result)and the comparison sequence that affects the system features (i.e., the influencing factor) are determined. The two sequences are characterized by X0(k),k = 1,2 … m and Xi(k),k = 1,2 … m,i = 1,2 … n, respectively, where m is the number of results corresponding to one factor, and n is the number of factors.
2 According to Eq. 1, the original data are non-dimensionalized:
where
3 The difference sequence is calculated according to Eq. 2:
Where
4 The gray correlation number is calculated according to Eq. 3:
where
5 The gray correlation degree is calculated according to Eq. 4:
where
4.2.2 Gray Correlation Analysis
The data of the same kind of modified asphalt with different gradations in Table 5 are equalized (since the deformation of the A-16%-1 asphalt mixture reaches 1,000 mm, resulting in the abnormal indexes, this set of data is excluded from the analysis). RDr and CS in HWTT are taken as reference sequences. The softening point, rotational viscosity at 180 °C in the conventional test, the ZSV of the Cross model and Carreau model obtained by frequency scanning in the rheological property test, the viscous stiffness modulus (
The correlation analysis is performed on the data in Table 8. The correlation between the two reference sequences is shown in Table 9.
As shown in Table 9, the ranking of asphalt performance indexes in terms of the gray correlation degree with RDr and CS in HWTT is the same: permanent deformation > softening point > viscous stiffness modulus > rutting factor > rotational viscosity > ZSV (Cross model) > improved rutting factor > ZSV (Caren model). The permanent deformation has a correlation degree larger than 0.8 with both RDr and CS, indicating that it is strongly correlated with the high-temperature rutting resistance of the asphalt mixture. Additionally, the correlations between the softening point and viscous stiffness modulus and the two indexes in HWWT are all higher than 0.7, which indicates a strong correlation. Therefore, the use of the permanent deformation and viscous stiffness modulus in the repeated creep test is recommended for the evaluation of the high-temperature performance of rubberized asphalt mixture. Compared with rotational viscosity in conventional tests, they correlate better with the high-temperature stability of the mixture. The softening point also shows an excellent correlation with the high-temperature performance in conventional tests and has a priority over rotational viscosity.
5 Conclusion
1) The correlation coefficients of eigenvalues based on fractal dimension theory are all greater than 0.9, which indicates that fractal dimension theory is suitable for characterization of gradation characteristics. Based on the correlation analysis of the influencing factors of the high temperature performance of the mixture, it can be seen that the asphalt-stone ratio and the fractal dimension of coarse aggregate Dc have significant indigenous effects on the high temperature performance of the mixture. Therefore, gradation has the greatest impact on the high temperature stability of rubberized asphalt mixture.
2) The grey correlation degree was used to analyze the indexes of rubberized asphalt binder and hamburger rutting test results. It was found that there was no significant correlation between rotational viscosity and other high temperature rheological indexes and rubberized asphalt mixture. The correlation coefficient between the permanent deformation, viscous stiffness modulus and softening of the repeated creep test and the results of the Hamburg rutting test is greater than 0.7, showing a significant indigenous correlation, which can effectively characterize the high-temperature performance of rubberized asphalt binder. These three indicators are recommended as high-temperature evaluation indexes of rubberized asphalt binder.
Data Availability Statement
The raw data supporting the conclusion of this article will be made available by the authors, without undue reservation.
Author Contributions
CX is the experimental designer and executor of this research, completing data analysis and writing the first draft of the paper; JL guides data analysis, thesis writing and revision; LZ, TR, HL, and JC participated in the experimental design and analysis of experimental results, and the paper was revised. All authors have read and agreed to the final text.
Conflict of Interest
Authors CX, JL, TR, HL, and JC were employed by Guangxi Beitou Transportation Maintenance Technology Group Co., LTD. Author LZ was employed by Guangxi Transportation Science and Technology Group CO., LTD.
Publisher’s Note
All claims expressed in this article are solely those of the authors and do not necessarily represent those of their affiliated organizations, or those of the publisher, the editors and the reviewers. Any product that may be evaluated in this article, or claim that may be made by its manufacturer, is not guaranteed or endorsed by the publisher.
References
Bahia, H. U., Hanson, D. I., and Zeng, M. (2001). Characterization of Modified Asphalt Binders in Superpave Mix Design[R]. Washington DC, United States: Transportation Research Board.
Ding, X., Chen, L., Ma, T., Ma, H., Gu, L., Chen, T., et al. (2019). Laboratory Investigation of the Recycled Asphalt Concrete with Stable Crumb Rubber Asphalt Binder. Constr. Build. Mater. 203 (APR.10), 552–557. doi:10.1016/j.conbuildmat.2019.01.114
He, L. P., Shen, A. Q., and Xie, C. (2014). Orthogonal Test of Rubber Asphalt Binder Performance[J]. J. Chang’an University:Natural(Science Ed. 34 (1), 7–12. doi:10.19721/j.cnki.1671-8879.2014.01.002
He, L. P. (2019). Study on Viscoelastic Properties and High Temperature Performance of Rubber Asphalt Based on DMA method[D]. Xi’an, China: Chang’an University.
Hu, J., Ma, T., Yin, T., and Zhou, Y. (2022). Foamed Warm Mix Asphalt Mixture Containing Crumb Rubber: Foaming Optimization and Performance Evaluation. J. Clean. Prod. 333, 130085. doi:10.1016/j.jclepro.2021.130085
Hu, S. S., Qin, R. P., and Li, H. (2018). Application of Rubber Asphalt Performance Testing Technology[J]. Acta Mater. Compos. Sin. 35 (8), 14. doi:10.13801/j.cnki.fhclxb.20170905.002
Huang, W. D., Wang, W., and Huang, Y. (2010). Test on Influencing Factors of High Temperature Stability of Rubber Asphalt Mixture[J]. J. Tongji Univ. Sci. 38 (7). doi:10.3969/j.issn.0253-374x.2010.07.015
Industry standards of Transportation Department of the People ' s Republic of China (2011). Asphalt Rubber for Highway Engineering: JT/T 798-2019 [S]. Beijing, China: china communications press.
Industry standards of Transportation Department of the People ' s Republic of China (2011). Standard Test Methods of Bitumen and Bituminous Mixtures for Highway Engineering: JTG E20-2011[S]. Beijing, China: china communications press.
Li, X. Y., Ping, L., and Wang, H. N. (2015). Performance Test of Rubber Asphalt Based on Domestic and Foreign Test Methods[J]. J. Transp. Eng. 15 (1), 8. doi:10.3969/j.issn.1671-1637.2015.01.002
Lin, J. T., and Fan, L. (2018). High Temperature Performance Evaluation of Asphalt Based on MSCR Test and Burgers Model Analysis[J]. J. Highw. Transp. Res. Dev. 35 (6), 8. doi:10.3969/j.issn.1002-0268.2018.06.004
Ma, T., Chen, C. L., and Zhang, Y. (2021). Development of Rubber Powder Used in Asphalt Modification Technology[J]. China J. Highw. Transp. 34 (10), 16. doi:10.19721/j.cnki.1001-7372.2021.10.001
Navarro, F. J., Partal, P., Martínez-Boza, F., and Gallegos, C. (2005). Influence of Crumb Rubber Concentration on the Rheological Behavior of a Crumb Rubber Modified Bitumen. Energy fuels. 19 (5), 1984–1990. doi:10.1021/ef049699a
Shenoy, A. (2001). Unifying Asphalt Rheological Data Using the Material's Volumetric-Flow Rate. J. Mat. Civ. Eng. 13 (4), 260–273. doi:10.1061/(asce)0899-1561(2001)13:4(260)
Tan, X. R., and Deng, J. L. (1995). Grey Correlation Analysis : a New Method for Multivariate Statistical Analysis[J]. Stat. Res. 3, 3. doi:10.19343/j.cnki.11-1302/c.1995.03.011
Yang, G., Huang, W. D., and Li, Y. W. (2010). Experimental Study on High Temperature Performance Evaluation Index of Rubber Asphalt Mixture[J]. J. Build. Mater. 13 (6), 6. doi:10.3969/j.issn.1007-9629.2010.06.010
Yang, Q., Guo, Z. Y., and Chen, L. P. (2006). Fractal Characteristics Analysis of Graded Crushed Stone and its Application in Pavement Engineering[J]. J. Build. Mater. 9 (4), 5. doi:10.3969/j.issn.1007-9629.2006.04.008
Keywords: rubberized asphalt, high-temperature performance, rheological property, correlation analysis, gray correlation analysis
Citation: Xie C, Luo J, Zeng L, Ren T, Liu H and Chen J (2022) Research on Evaluation Index of High Temperature Performance of Rubberized Asphalt Binder. Front. Mater. 9:904087. doi: 10.3389/fmats.2022.904087
Received: 25 March 2022; Accepted: 20 April 2022;
Published: 20 May 2022.
Edited by:
Hui Yao, Beijing University of Technology, ChinaReviewed by:
Tao Ma, Southeast University, ChinaSiyu Chen, Southeast University, China
Jue Li, Chongqing Jiaotong University, China
Copyright © 2022 Xie, Luo, Zeng, Ren, Liu and Chen. This is an open-access article distributed under the terms of the Creative Commons Attribution License (CC BY). The use, distribution or reproduction in other forums is permitted, provided the original author(s) and the copyright owner(s) are credited and that the original publication in this journal is cited, in accordance with accepted academic practice. No use, distribution or reproduction is permitted which does not comply with these terms.
*Correspondence: Cheng Xie, 45902900@qq.com