- 1Public Education Department, Anhui Universtiy of Arts, Hefei, China
- 2School of Instrument Science and Opto-Electronics Engineering, Hefei University of Technology, Hefei, China
- 3School of Energy Materials and Chemical Engineering, Hefei University, Hefei, China
Sports and physical fitness play important roles in people’s daily life. By sticking to exercise we can better enjoy a healthy life. Scientific and accurate monitoring of sports training and individual physical condition is important. However, many challenges still need to be solved, such as the complex design of measurement devices, the external power supplies, inaccurate measurement data, and unscientific measurement methods. Recently, the TENG-based self-powered real-time measurement sensors have attracted much more attention due to their unique advantages such as wearable features, simple construction, a wide choice of materials, and operation without external power supply. They can measure a wide range of real-time data such as haptics, velocity, pressure, and dynamic movement. In addition, they can also be made into a human–machine interactive device to provide athletes with a scientific approach to training. In this review, the working principle of TENGs is summarized and clarified. Moreover, the applications of the TENG in the areas of tactical strategy in sports, individual sports training, and physical state monitoring are also reviewed. Finally, the challenges and development opportunities faced by the TENG are also discussed and summarized.
1 Introduction
With the gradual improvement in the quality of life, many electronic devices have replaced human labor, so the negative effect of this is that people are exercising less and even losing their physical fitness. With this background, a better tool model for judgment and analysis for real-time human movement monitoring is required in the physical state monitoring and sports training field. Many traditional monitoring methods have been used for human movement monitoring. They mostly worked based on optics (Leber et al., 2018), thermal (Ge et al., 2019), resistive (Yamada et al., 2011), magnetic (Wang Y. et al., 2015), and chemical characteristics (Wiorek et al., 2020). Optical methods typically monitor signals by sensing changes in the intensity and frequency of light, which provides excellent accuracy and temporal resolution, but the fiber optic material is rigid and allows only small deformations. The capacitive method is to monitor the motion signal by detecting the change in capacitance. Although its sensitivity is very high, its structure is usually not very flexible. The resistance method is to monitor the human movement signal by investigating the change in resistivity, and it has high durability and fast response time, but it is usually not very flexible in structure either. The magnetic method is by sensing the change in the magnetic field, but it is generally not very stable. The chemical method monitors physiological data by sensingchanges in temperature, pH, etc., but it usually has a slow response rate. The electromagnetic method is to monitor the signal by electromagnetic induction; it has the advantages of long life, high efficiency at high frequency, high sensitivity, and high durability, but it also has some disadvantages such as high density, inflexibility, heaviness, and high cost. The electrostatic method is to monitor the signal by electrostatic induction and has the advantage of lightweight, but it also has the problem of low output and the need for pre-charging. The piezoelectric method is to sense the signal by piezoelectric effect and electrostatic induction; it is easy to make a fine structure, but its efficiency and output are relatively low. Most of the methods mentioned earlier have complex structures, usually require external power supplies, and are relatively costly. To solve these problems, prof. Zhonglin Wang and his co-workers proposed the concept of TENG in 2012 (Fan et al., 2012), which works based on the coupling effect of contact electrostatic and electrostatic induction (Wang, 2017). It has four different modes of operation (Wang S. et al., 2015), namely, the lateral sliding mode, vertical contact-separation mode, freestanding triboelectric-layer mode, and the single-electrode mode, respectively, which are displayed in Figure 1. (1) The lateral sliding mode is exhibited in Figure 1A. It starts from the same state of contact, with separation occurring in the in-plane direction by interlayer sliding. The relative sliding between these two surfaces generates triboelectric charges on both surfaces, which creates a lateral polarization along the sliding direction. In order to balance the electric field generated by the triboelectric charges, the inductive potential will drive the flow of electrons on the top and bottom electrodes. (2) The vertical contact-separation mode is shown in Figure 1B, where two triboelectric layers can come into contact with each other under the pressure of an external movement, resulting in oppositely charged surfaces. Subsequently, on release, a small gap in a vertical plane direction separates these two surfaces, creating a potential drop at both electrodes that drives the flow of electrons through the connected load. (3) The freestanding triboelectric-layer model is shown in Figure 1C, In this case, the freestanding triboelectric layers will alternately approach either of the two electrodes so that the induced potential difference will be reversed periodically. The AC output will drive an external load. (4) The single-electrode mode, shown in Figure 1D, changes the local electric field distribution as the top object approaches or leaves the electrode, causing an exchange of electrons between the bottom electrode and the reference electrode or ground, maintaining the potential balance between them. This is an area where mechanical energy is efficiently converted into electrical energy and signals using Maxwell’s displacement current as a driving force. Its most basic physical model is available in Maxwell’s set of equations (Wang, 2020) and will not be expanded upon this content here.
Through extensive research it has been shown that the TENG has the unique advantages of high efficiency (Wang Z. L. et al., 2015; Liu W. et al., 2019; Hinchet et al., 2019), simple construction (Chandrashekar et al., 2015; Wang Z. L. et al., 2015; Parida et al., 2019), high accuracy (Wang Z. et al., 2020), low cost (Fan et al., 2015), wide choice of materials (Chandrashekar et al., 2015; Pu et al., 2017b), light weight (Seung et al., 2015), high durability (Lee et al., 2013), being scalable (Pu et al., 2017b), requiring no external power supply (Cheng et al., 2017), being self-powered for tactile sensing (Meng et al., 2013), velocity (Tang et al., 2021), pressure (Lee et al., 2016), angle (Wang Z. et al., 2020), and dynamic motion (Pu et al., 2017a) sensing measurements, providing an excellent method of real-time monitoring for physical education and training. There are many types of sports, such as basketball, table tennis, athletics, swimming, fighting, and skiing, as shown in Table 1. Physical fitness monitoring includes the height and weight test, step test, lung function test, sprint, long-distance running, standing long jump, grip index, sit-ups, sit-and-reach test, and pull-ups, as shown in Table 2. These items currently suffer from various problems such as insensitive detection mechanisms, inaccurate data, and unscientific measurement methods. Due to the potential applications of TENGs in this area, this article reviews the use of TENGs in three areas: the tactical strategy in sport, individual sports training, and physical fitness testing and looks at the challenges and opportunities for TENG in the future.
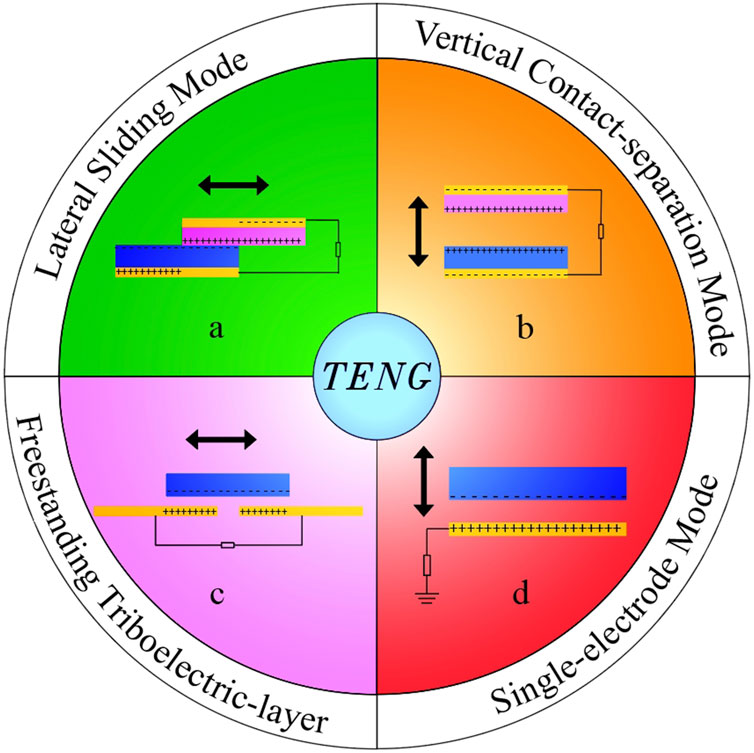
FIGURE 1. Working principal of the TENG: (A) lateral sliding mode; (B) vertical contact-separation mode; (C) freestanding triboelectric-layer mode; and (D) single-electrode mode.
2 TENG for Tactical Strategy in Sporting Competitions
Sporting competition is a very important characteristic for individuals, teams, and even countries. Sports competition requires the guidance of tactical strategies to improve the performance of athletes and provide impartial refereeing of referees. Different sports have different ways to collect data and contents, as shown in Table 1. Here, the sports are divided into six projects, which are basketball, table tennis, athletics, swimming, fighting, and skiing, respectively. During the basketball games, we can collect signals through the floor, basketball, and basketball shoes, which can monitor athletes’ movement data, basketball pressure on the floor, and the contact angle and power of the basketball to the rim. For table tennis, we can track signals through table tennis tables and rackets, which can judge the landing point of the ball and the strength of the blow. Athletics can use the finish floor to collect signals and determine the arrival time. In the sports of swimming, the signals can be picked up through the swimming pool and swimwear; then, the athlete’s swimming stance and speed can be monitored. During fighting sports, the signals can be collected through gloves and sandbags to monitor the strength of the athlete, while signals can be collected from tracks and skateboards to monitor the speed at different places during skiing games. In order to monitor the movement data during sports, different types of TENGs have been designed through different monitoring elements, which are described as follows.
In sports, position sensing is important, and the TENG has many applications in this area. To determine the landing position of a ping pong ball, Luo et al. developed an auto dynamic drop distribution statistics system and an edge ball judgment system (Luo et al., 2019), shown in Figure 2A, to provide training guidance and real-time assistance to athletes and referees. In order to determine the position of volleyball striking at the limb, Shi et al. invented a triboelectric nanogenerator (TENG)-based e-skin (Shi et al., 2021). As shown in Figure 2C, a volleyball sensing system based on a 2 × 3 e-skin array was developed, which can monitor the player’s position and angle of hitting the ball. The position of the athlete also needs to be monitored. Shi et al. (2020) demonstrated a smart floor monitoring system, as shown in Figure 2E. The system is based on deep learning for data analysis and can determine the athlete’s position, activity status, and identity information. There is another smart floor with a unique structure. He et al. (2017a) integrated the square-frame triboelectric nanogenerator (SF-TENG) into a standard wooden floor, as shown in Figure 2B. It can also monitor athletes and the basketball movement status.
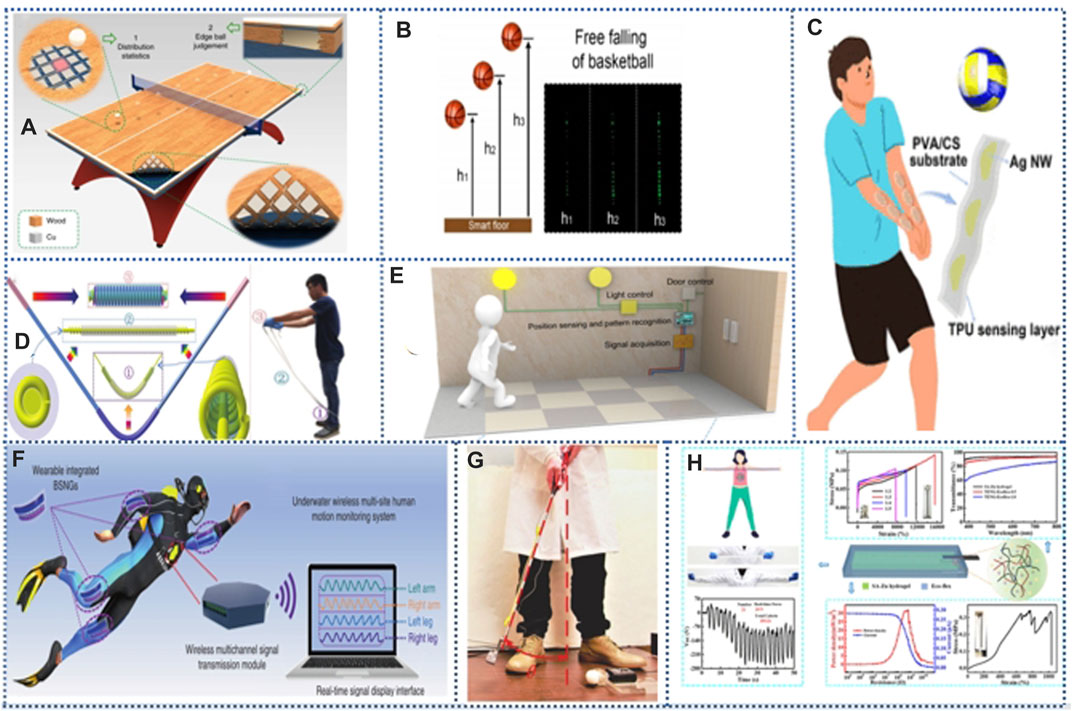
FIGURE 2. TENG for tactical strategy in sporting competitions. (A) Diagram of the intelligent wooden ping pong table. Reproduced with permission (Luo et al., 2019). Copyright 2019, Springer Nature. (B) Row of LEDs illuminated by free-falling basketball at different heights. Reproduced with permission (He et al., 2017a). Copyright 2017, American Chemical Society. (C) Diagram of the 2 × 3 integrated electronic skin arrays applied to the sport of volleyball. Reproduced with permission (Shi et al., 2021). Copyright 2021, American Chemical Society. (D) Schematic illustration of the self-counting skipping rope. Reproduced with permission (Dong et al., 2018). Copyright 2018, Wiley-VCH. (E) Conceptual diagram of the smart floor monitoring system. Reproduced with permission (Shi et al., 2020). Copyright 2020, The Author(s). (F) Schematic diagram of the application of a bionic stretchable nanogenerator (BSNG) in the underwater human motion monitoring system. Reproduced with permission (Zou et al., 2019). Copyright 2019, Springer Nature. (G) Application of MS for the swing angle measurement in golf. Reproduced with permission (Wu et al., 2019). Copyright 2019, Wiley-VCH. (H) Diagram of the practical application of the self-powered smart elastic belt system. Reproduced with permission (Sheng et al., 2021). Copyright 2021, American Chemical Society.
In cycling, the TENG also has some applications. Meng et al. (2015) proposed a self-powered rotational sensor (SPRS) for multitasking motion measurement, which has been used in real-time motion monitoring for bicycle wheels. For better data collection, Zhang et al. (2022) reported a wireless sensor system based on a triboelectric nanogenerator (TENG), which was applied to real-time monitoring of tire pressure and the speed of a bicycle. To judge cycling competitions fairly, Tang et al. (2021) proposed a wireless motion monitoring system based on the triboelectric nanogenerator (TENG). It can be used to monitor in real time the flow of athletes and bicycles entering the detection area, as well as their speed and direction of movement.
There is also the ability of TENGs to monitor the intensity level of a sporting event. Wu et al. (2019) proposed a cylindrical multifunctional sensor (MS), as shown in Figure 2G, which has a rotating magnetic mechanism with the ability to detect acceleration, force, and rotation parameters. It can be used to measure boxing acceleration and the golf club angle. To improve sensitivity, Peng et al. (2019) prepared a fabric-based triboelectric nanogenerator (FB-TENG) using melt-blown technology. It serves as a self-powered sensor with a very short response time to accurately collect pedestrian data and monitor boxing strength. To improve the efficiency of data collection, He et al. (2017b) described a square-grid triboelectric nanogenerator (SG-TENG) for collecting vibration energy and sensing impact. The SG-TENG is integrated with a table tennis paddle to obtain vibrational energy from hitting a table tennis ball with the paddle. The SG-TENG is integrated into a boxing glove and can be used in various combat sports, such as boxing and kickboxing, to monitor the boxing frequency and amplitude. The collected data help athletes monitor their condition and improve their skills.
The TENG has also been applied to the scoring system. Using silver-coated nylon yarn and silicone rubber, Dong et al. (2018) have designed a yarn-based triboelectric nanogenerator (TENG), as shown in Figure 2D, it has been used in self-counting jump ropes, gesture recognition, and the golf scoring system. To monitor the athlete’s limb stretch strength, Sheng et al. (2021) proposed a kind of TENG based on (SA-Zn) hydrogel. As shown in Figure 2H, energy can be obtained from the athletes’ movement. They also prepared a self-powered smart training belt for monitoring arm extension.
There are also specific sports in which the TENG can be applied. To help athletes with snow sports, Ahmed et al. (2019) presented the first snow-based triboelectric nanogenerator (snow-TENG), which can be used as a multifunctional sensor and energy harvester based on the snow-triboelectrification principle. It is very useful for snow-related movements. Similarly, to help athletes with underwater sports, an underwater biomimetic triboelectric nanogenerator was reported by Zou et al. (2019), as shown in Figure 2F, which mimics the structure of ion channels in the cell membrane of electric eels. It can be used for human swimming movement monitoring and submarine rescue.
These TENG-based motion sensors can make sports competitions scientific and fair and enable athletes to better develop their abilities. These works not only expand the application of automated systems to intelligent sports but also promotes the development of big data analysis in the intelligent sports industry.
3 TENG for Personal Sports Training
With the rapid development of science and technology, it is crucial for athletes to apply these emerging science and technology for physical exercise. Here, the TENG can be used as a human–machine interaction device and a mobile power source, applying the Internet of Things and artificial intelligence to athletes’ daily training and providing people with a scientific and convenient way to exercise. By detecting micro-movements of the skin around the corner of the eye, the TENG can assist athletes in the human–machine interaction. A TENG-based micromotion sensor was reported by Pu et al. (2017a) as shown in Figure 3A, which is fixed on a pair of glasses and applied to intelligent remote control systems and wireless hands-free typing systems, with the advantages of stability, simple operation, low cost, and ultra-high sensitivity. Similarly, Anaya et al. (2020) developed a novel triboelectric sensor (NEDTS). A micro-motion sensor has been developed for monitoring the skin around the corners of the eyes, which is integrated into a portable human–machine interface that can be used to remotely control instrumented devices.
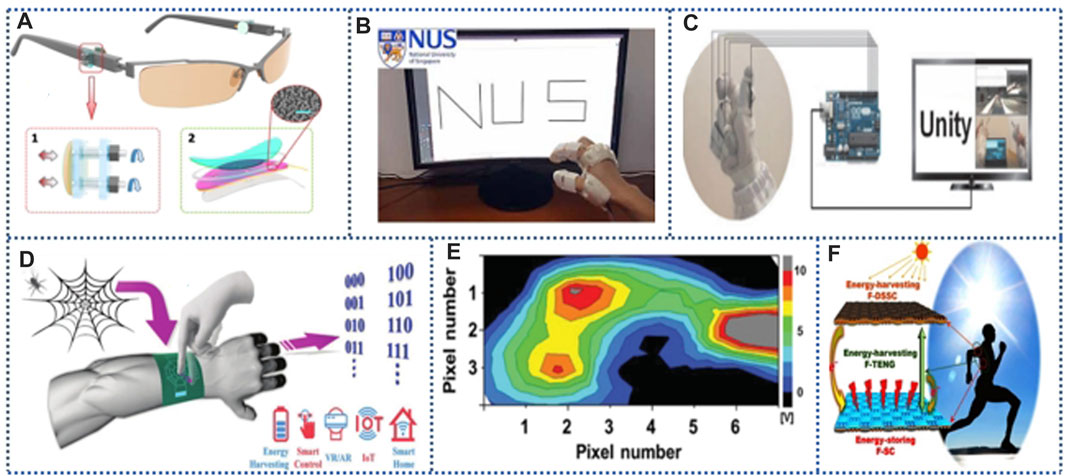
FIGURE 3. TENG for personal sports training. (A) Schematic diagram of the construction of the msTENG fitted to a pair of ordinary glasses. Reproduced with permission (Pu et al., 2017a). Copyright 2017, The Authors, published by AAAS. (B) Demonstrations of finger bending sensing as main functions of HMI. Reproduced with permission (Zhu et al., 2020). Copyright 2020, The Authors, published by AAAS. (C) Schematic diagram of the control system. Reproduced with permission (Wen et al., 2020). Copyright 2020, The Authors, published by Wiley-VCH Verlag GmbH and Co. KgaA, Weinheim. (D) Schematic representation of the BISNC interface and its spider-net-inspired structure. Reproduced with permission (Shi and Lee, 2019). Copyright 2019, The Authors, published by Wiley-VCH Verlag GmbH and Co. KgaA, Weinheim. (E) Diagram of a self-powered pressure sensor for detecting foot pressure. Reproduced with permission (Lee et al., 2016). Copyright 2016, Wiley-VCH. (F) Schematic representation of the self-charging power textile. Reproduced with permission (Rahman et al., 2020). Copyright 2016, The Authors.
In addition, the TENG can sense hand joint movements to help athletes train. Wen et al. (2020) investigated facile carbon nanotube and thermoplastic elastomer (CNT/TPE) coating methods, shown in Figure 3C. Then, a pair of TENG-based gloves can be made. The gloves have energy harvesting and human motion sensing capabilities. It can use the machine learning technology to complete various gesture recognition tasks, and the impact of sweat during operation is minimized. Similarly, Zhu et al. (2020) proposed a novel TENG-based finger flexion sensor, as shown in Figure 3B; integrating it into a glove allows for the human–machine interaction. In order to achieve more precise synchronization control, a TENG-based joint motion sensor (jmTQS) was designed by Pu et al. (2018). It had a manipulator synchronization system that uses human gestures instead of a handle to operate the manipulator, making the human–machine interface more natural and precise. In order to improve the flexibility of sensing, Shi and Lee (2019) proposed a flexible bio-inspired spider-net-coding (BISNC) interface. As shown in Figure 3D, the technology has various applications in intelligent sports, such as virtual reality, the Internet of Things, and human–computer interactions. For the sole purpose of training the athlete’s hand grip strength, Zhao et al. (2020) fabricated a TENG-based smart glove, which enables hand grip monitoring for athletes.
It is also possible for the TENG to be made into a smart band to assist athletes. Han et al. (2019) reported a TENG-based band, which can identify humans by detecting their muscle activity. The band allows quantitative detection of the walking distance, stride length, and speed. Similarly, Maharjan et al. (2018) demonstrated a novel TENG-based wearable motion sensor (WHEM-TENG), which can monitor the daily training of athletes.
There are also ways for the TENG to assist athletes in their training through their voice. Guo et al. (2018) designed a TENG-based auditory sensor (TAS), which can be used to build electronic hearing systems. Combined with intelligent machine equipment, TAS can perform high-quality speech recognition. To reduce the cost of speech recognition devices, Yuan et al. (2021) proposed a 3D-printed acoustic triboelectric nanogenerator (A- TENG). The speech signal is first converted into an electrical signal by A-TENG and then recognized and processed by a neural network for subsequent circuits, which can realize real-time speech recognition and show great potential in the field of intelligent sports.
In sports training, foot training is also critical. Lee et al. (2016) developed TENG-based pressure sensor H-TENGs, as shown in Figure 3E. It can be used as an active sensor to map the pressure generated by the athlete’s feet for training assistance. There are also some TENG-based smart shoes being researched. A review of TENG-based smart power generation shoes was conducted by Zou et al. (2020). Here, we will not go into details. These smart shoes can harvest energy and can also help athletes train by sensing the rhythm and intensity of their movements.
Energy harvesting can also be performed by the TENG to assist athletes in their training. Wang et al. (2017) developed a TENG-based energy-harvesting skin. It drives human–computer interaction equipment by collecting energy from athletes’ physical training. For carrying convenience, a highly miniaturized TENG (MFKI-HNG) was proposed by Rahman et al. (2020), which can effectively collect the vibration energy induced by human movement. Using a custom power management circuit, the athlete can make it a portable power source for electronics. For better energy harvesting, Wen et al. (2016) proposed a TENG-based hybrid self-charging system, shown in Figure 3F. It can harvest the energy of sunlight and sports at the same time and store them in one energy storage unit. It can be woven into textiles to create smart sportswear to assist athletes in their sports training. To improve wearing comfort for athletes, Ren et al. (2020) developed a TENG-based self-cleaning energy harvesting system. It can continuously collect a variety of energy and integrate with the human body, collecting energy without affecting the comfort of athletes.
There are still many sports aids based on the TENG, and it can be seen that they make the training of athletes more scientific and convenient.
4 TENG for the Physical Fitness Test
The physical fitness test is an effective means for athletes to monitor their physical condition. Regular physical fitness tests can prevent a number of potential physical diseases. The main test projects are shown in Table 2, which are the height and weight test, step test, lung function test, sprint, long-distance running, standing long jump, grip index, sit-ups, sit-and-reach test, and pull-ups. The height and weight test can test the level of human morphological development and body shape. The step test can monitor the function of the human cardiovascular system. The lung function test can monitor the breathing system function. The sprint test can monitor physical flexibility and harmonization. The long-distance running test can monitor physical endurance and the cardiovascular system. The standing long jump test can monitor the explosive power of lower limb muscles. The grip index test can monitor the relative strength of muscles. The sit-up test can monitor physical strength and endurance. The sit-and-reach test can monitor joint mobility and muscle flexibility, and the pull-ups can monitor the upper body strength. Different TENG sensors can be designed depending on how and what to test. Currently, a self-powered real-time sensor monitoring system based on the TENG has been applied to all aspects of physical fitness testing.
There are many applications for the TENG in joint and muscle rehabilitation. Wang et al. (2018) developed a TENG-based flexible sensor (KT-TENG). As shown in Figure 4H, the device can operate effectively during bending or stretching. The open-circuit voltage and short-circuit transfer charge showed good linearity with both tensile displacement and bending angle and could be used as a wearable self-powered sensor for real-time human motion monitoring, such as human gestures and knee flexion. To improve monitoring accuracy, Wang Z. et al. (2020) developed a TENG-based angle sensor (SPAS) that has been synthetically optimized to achieve a resolution of 2.03 nano radian. In addition, the SPAS has the advantage of being lightweight and thin. It can be installed in medical devices for personalized orthopedic rehabilitation. In a recent study, a TENG-based motion sensing system (MC-EH-HL) was developed by Gao et al. (2021) It has a unique structure, as shown in Figure 4F, and it can assist athletes in leg muscle monitoring and also lower limb rehabilitation training. For the treatment of joint trauma, Yang et al. (2021) prepared a multifunctional TENG (MF-TENG) with photothermal properties. As shown in Figure 4B, MF-TENG can be attached to different joints of the human body and can provide photothermal therapy under near-infrared laser irradiation to restore human joint motion. To assist patients with joint movements, Guo et al. (2021) invented a TENG-based crutch. It is equipped with a deep learning system and has advanced sensing capabilities, as shown in Figure 4C, providing a health monitoring platform for users with movement disorders.
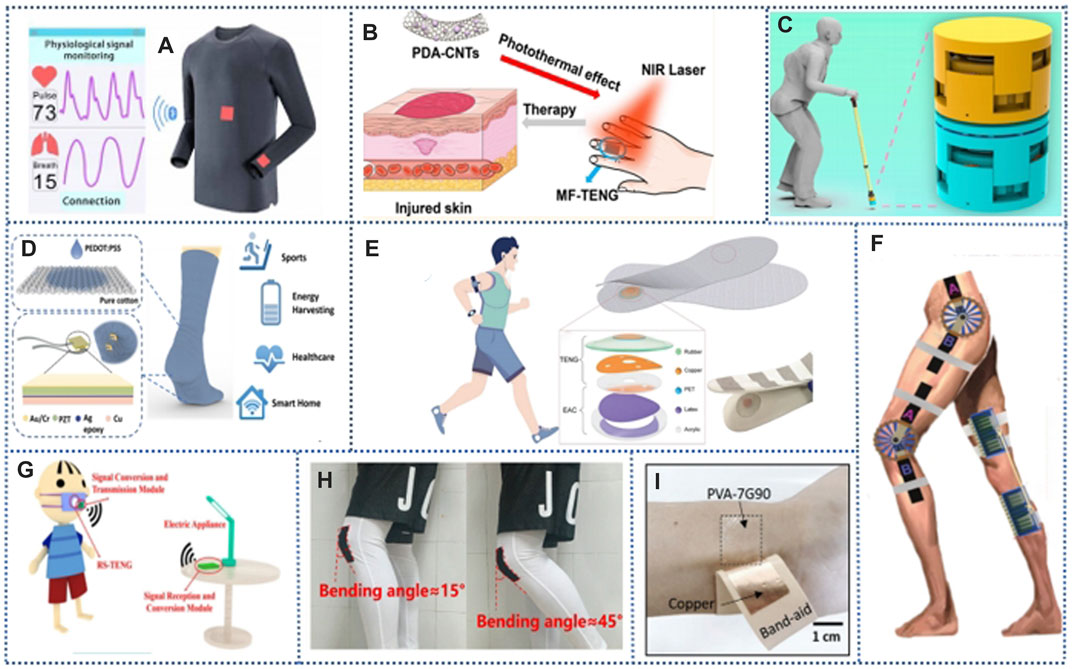
FIGURE 4. TENG for the physical fitness test. (A) To monitor respiratory and pulse signals in real time, two TATSAs are integrated into one shirt. Reproduced with permission (Fan et al., 2020). Copyright 2020, The Authors, published by AAAS. (B) Simulation diagram of the photothermal treatment. Reproduced with permission (Yang et al., 2021). Copyright 2021, American Chemical Society. (C) Multifunctional monitoring system enabled by the caregiving walking stick. Reproduced with permission (Guo et al., 2021). Copyright 2021, American Chemical Society. (D) Schematic representation of PEDOT. Reproduced with permission (Zhu et al., 2019). Copyright 2019, American Chemical Society. (E) Overview of the smart insole for gait monitoring. Reproduced with permission (Lin et al., 2018). Copyright 2018, Wiley-VCH. (F) Configuration of the MC–EH–HL system, which consists of two main components: R-TENG and S-PEG. Reproduced with permission (Gao et al., 2021). Copyright 2021, The Authors, Advanced Science published by Wiley-VCH GmbH. (G) Control circuit diagram of the smart facemask. Reproduced with permission (Lu et al., 2022). Copyright 2018, published by Elsevier Ltd. (H) Schematic diagram of the KT-TENG for real-time monitoring of knee joint movements. Reproduced with permission (Wang et al., 2018). Copyright 2018, The Authors. (I) Diagram of the PVA-7G90 film attached to the human wrist. Reproduced with permission (Wang R. et al., 2020). Copyright 2020, Wiley-VCH.
A number of applications are also available for the TENG in monitoring gait abnormalities. Lin et al. (2018) reported a TENG-based smart insole. As shown in Figure 4E, movement patterns such as jumping, stepping, walking, and running can be accurately monitored and can be used to monitor gait abnormalities and rehabilitation assessments. For improved comfort, Zhu et al. (2019) developed a TENG-based sock (-sock). It has better wearability, as shown in Figure 4C, and allows for walking pattern recognition and healthcare.
In addition, the TENG can monitor weak physiological movements. Chen et al. (2017) reported a TENG-based micromotion sensor. It can be attached to different parts of the athlete to monitor physiological signals in real time, such as blinking, pronunciation, arm movement, and pulse. Similarly, Lu et al. (2020) developed an ion-based TENG (PL-TENG), which attaches to the athlete’s face and neck to monitor fatigue and distraction.
In the field of sleep monitoring, the TENG has many applications. Zhou et al. (2020) developed a TENG-based textile, which can monitor all-round physiological parameters during the sleep of athletes. It can help improve sleep quality and physical fitness. To improve wearability, Fan et al. (2020) developed a TENG-based sensor array with high sensitivity and comfort, as shown in Figure 4A, further developing a long-term non-invasive health monitoring system for cardiovascular disease and sleep apnoea syndrome, making great progress in the quantitative analysis of certain chronic diseases. In order to improve the measurement sensitivity, Salauddin et al. developed a new MXene/Ecoflex nanocomposite with a fabric-based waterproof TENG (FW-TENG). Salauddin et al. (2020) invented a motion sensing device based on FW-TENG, which can be used as a sleep monitoring sensor for athletes. There are many more applications of the TENG for body health monitoring, and the aforementioned introduction shows that it has a great potential for development in this area.
The TENG can be used for cardiovascular monitoring. Wang R. et al. (2020) optimized the pva–gelatin composite film. As shown in Figure 4I, the prepared TENG devices made of the optimized pva–gelatin laminate film have a stable output. This wearable device can monitor an athlete’s pulse to prevent cardiovascular disease. Similarly, Park et al. (2021) have developed a novel TENG-based electronic skin which can also monitor the arterial pulse of the wrist. For better micro-pressure measurement, Liu Z. et al. (2019) developed a TENG-based pressure sensor, which can be mounted on the athlete’s chest and wrist for respiration and pulse monitoring. In order to better monitor in real time, Meng et al. (2020) developed a TENG-based sensor system (TS). The collected athlete’s pulse signal can be wirelessly transmitted to the application on the smartphone in time to display the athlete’s health data.
In addition, the TENG is also used for respiratory monitoring. Zhang et al. (2019) proposed a TENG-based respiration monitoring device, which can monitor athletes’ breathing status by sensing chest changes. To measure respiratory airflow, Wang et al. (2019) developed a TENG-based respiration sensor, which can convert respiratory airflow into electrical signals for detecting an athlete’s respiratory flow and respiratory rate. For ease of wear, Lu et al. (2022) designed a TENG-based respiratory sensor (RS-TENG), as shown in Figure 4G. Combining it with a mask and a circuit module can constitute an apnea alarm system so that an alarm can be issued in time after people stop breathing. In order to monitor blood oxygen levels, Chen et al. (2019) developed a TENG-based flexible blood oxygen monitoring system for the first time. Flexible blood oxygen and pulse detectors with no significant degradation in performance were obtained, providing a new method for sustainable monitoring of physiological parameters.
4.1 Summary and Outlook
Currently, sports competitions and body monitoring are moving toward intelligence. More and more sensors are appearing in this field. However, they share a common challenge that needs to be overcome: the measurement data are not accurate enough, the measurement methods are not scientific enough, and there are more interfering factors. For example, the sit-and-reach test is supposed to measure the flexibility of the subject’s lumbar joints and the flexibility of the muscles, but the current measurement method makes the length of the testers’ fingers, arms, and legs have a great influence on the measurement results. This article reviews the advantages of the TENG-based self-powered sensor, which is wearable, efficient, and has a wide choice of materials. However, the TENG also faces several opportunities and challenges.
1) Most of the current research on the TENG is still at the research stage. There is still a long way to go before it becomes a mature sensor. The main reason here is that most of the current research study has only characterized the potential of the TENG to be used as a sensor, and the accuracy as a sensor is not yet very high. The TENG can be designed as grids, to improve measurement sensitivity by increasing the density of the grids. For this, the highly sensitive self-powered angle sensor (SPAS) invented by Wang Z. et al. (2020) offers a way forward for accurate motion measurement of TENGs.
2) At present, the TENG, as a sports measurement device, still faces the problem of sweat soaking. By modifying the surface with hydrophobic chemical groups or generating surface nanostructures (Zhang et al., 2018), the hydrophobicity and self-cleaning properties of frictional electrical layers can be improved. Advanced encapsulation techniques (Wang, 2014) can also be developed to protect TENG devices from perspiration penetration. Consideration can also be given to designing modular and miniaturized wearable devices to solve these problems.
3) For sports, effective data collection and analysis are essential. Although some sports analysis techniques have been developed (Kutcher et al., 2013; Ahmadi et al., 2015), there are still limited ways to analyze sports data. The TENG has also developed some signal collection and analysis methods (Pu et al., 2017a; Wang Z. et al., 2020; Yin et al., 2020), but the accuracy and stability of the data still need to be improved, by integrating the data collection module, wireless transmission module, power management module, and signal processing module; it can collect data well in real time and solve some of the aforementioned problems.
There are many projects and requirements for sports and body monitoring, and this article provides an overview of different TENG sensing structures designed for different application conditions. For athletes’ sports training, the TENG can also be of great help in terms of human–machine interaction devices and energy supply. However, TENGs also face challenges such as poor sensing accuracy, imperfect signal processing, and sweat penetration, but what is certain is that along with the challenges there are also many opportunities and that TENGs combined with technologies such as cloud computing, big data, the Internet of Things, and artificial intelligence can become more intelligent and offer potential value for smart sport and body monitoring.
Author Contributions
JZ and ZY have contributed to data acquisition, data analysis, and drafting the article. These two authors have contributed equally to this work. XL has contributed to data analysis, interpretation of the data, and revising the article. All the authors have contributed to final proof reading and approval of the version for publication.
Funding
Financial supports provided by the Excellent Young Talents Fund Program of Higher Education Institutions of Anhui Province (Grant No. gxyg2017119), Anhui Provincial Quality Engineering Teaching and Research Key Projects (Grant No. 2021jyxm0754), the National Natural Science Foundation of China (Grant No.21606065), the Anhui Provincial Natural Science Foundation (Grant No. 1708085QE98), and the Fundamental Research Funds for the Central Universities (Grant No. PA2021GDGP0059) are gratefully acknowledged.
Conflict of Interest
The authors declare that the research was conducted in the absence of any commercial or financial relationships that could be construed as a potential conflict of interest.
Publisher’s Note
All claims expressed in this article are solely those of the authors and do not necessarily represent those of their affiliated organizations, or those of the publisher, the editors, and the reviewers. Any product that may be evaluated in this article, or claim that may be made by its manufacturer, is not guaranteed or endorsed by the publisher.
References
Ahmadi, A., Mitchell, E., Richter, C., Destelle, F., Gowing, M., O'Connor, N. E., et al. (2015). Toward Automatic Activity Classification and Movement Assessment during a Sports Training Session. IEEE Internet Things J. 2, 23–32. doi:10.1109/jiot.2014.2377238
Ahmed, A., Hassan, I., Mosa, I. M., Elsanadidy, E., Phadke, G. S., El-Kady, M. F., et al. (2019). All Printable Snow-Based Triboelectric Nanogenerator. Nano Energy 60, 17–25. doi:10.1016/j.nanoen.2019.03.032
Anaya, D. V., He, T., Lee, C., and Yuce, M. R. (2020). Self-Powered Eye Motion Sensor Based on Triboelectric Interaction and Near-Field Electrostatic Induction for Wearable Assistive Technologies. Nano Energy 72, 104675. doi:10.1016/j.nanoen.2020.104675
Chandrashekar, B. N., Deng, B., Smitha, A. S., Chen, Y., Tan, C., Zhang, H., et al. (2015). Roll-to-Roll Green Transfer of CVD Graphene onto Plastic for a Transparent and Flexible Triboelectric Nanogenerator. Adv. Mat. 27, 5210–5216. doi:10.1002/adma.201502560
Chen, H., Xu, Y., Zhang, J., Wu, W., and Song, G. (2019). Self-Powered Flexible Blood Oxygen Monitoring System Based on a Triboelectric Nanogenerator. Nanomaterials 9, 778. doi:10.3390/nano9050778
Chen, X., Parida, K., Wang, J., Xiong, J., Lin, M.-F., Shao, J., et al. (2017). A Stretchable and Transparent Nanocomposite Nanogenerator for Self-Powered Physiological Monitoring. ACS Appl. Mat. Interfaces 9, 42200–42209. doi:10.1021/acsami.7b13767
Cheng, X., Miao, L., Song, Y., Su, Z., Chen, H., Chen, X., et al. (2017). High Efficiency Power Management and Charge Boosting Strategy for a Triboelectric Nanogenerator. Nano Energy 38, 438–446. doi:10.1016/j.nanoen.2017.05.063
Dong, K., Deng, J., Ding, W., Wang, A. C., Wang, P., Cheng, C., et al. (2018). Versatile Core-Sheath Yarn for Sustainable Biomechanical Energy Harvesting and Real-Time Human-Interactive Sensing. Adv. Energy Mat. 8, 1801114. doi:10.1002/aenm.201801114
Fan, F.-R., Tian, Z.-Q., and Wang, Z. L. (2012). Flexible Triboelectric Generator. Nano Energy 1, 328–334. doi:10.1016/j.nanoen.2012.01.004
Fan, W., He, Q., Meng, K., Tan, X., Zhou, Z., Zhang, G., et al. (2020). Machine-Knitted Washable Sensor Array Textile for Precise Epidermal Physiological Signal Monitoring. Sci. Adv. 6 (11), eaay2840. doi:10.1126/sciadv.aay2840
Fan, X., Chen, J., Yang, J., Bai, P., Li, Z., and Wang, Z. L. (2015). Ultrathin, Rollable, Paper-Based Triboelectric Nanogenerator for Acoustic Energy Harvesting and Self-Powered Sound Recording. ACS Nano 9, 4236–4243. doi:10.1021/acsnano.5b00618
Gao, S., He, T., Zhang, Z., Ao, H., Jiang, H., and Lee, C. (2021). A Motion Capturing and Energy Harvesting Hybridized Lower‐Limb System for Rehabilitation and Sports Applications. Adv. Sci. 8, 2101834. doi:10.1002/advs.202101834
Ge, G., Lu, Y., Qu, X., Zhao, W., Ren, Y., Wang, W., et al. (2019). Muscle-Inspired Self-Healing Hydrogels for Strain and Temperature Sensor. ACS Nano 14, 218–228. doi:10.1021/acsnano.9b07874
Guo, H., Pu, X., Chen, J., Meng, Y., Yeh, M.-H., Liu, G., et al. (2018). A Highly Sensitive, Self-Powered Triboelectric Auditory Sensor for Social Robotics and Hearing Aids. Sci. Robot. 3 (20), eaat2516. doi:10.1126/scirobotics.aat2516
Guo, X., He, T., Zhang, Z., Luo, A., Wang, F., Ng, E. J., et al. (2021). Artificial Intelligence-Enabled Caregiving Walking Stick Powered by Ultra-low-frequency Human Motion. ACS Nano 15, 19054–19069. doi:10.1021/acsnano.1c04464
Han, Y., Yi, F., Jiang, C., Dai, K., Xu, Y., Wang, X., et al. (2019). Self-Powered Gait Pattern-Based Identity Recognition by a Soft and Stretchable Triboelectric Band. Nano Energy 56, 516–523. doi:10.1016/j.nanoen.2018.11.078
He, C., Zhu, W., Chen, B., Xu, L., Jiang, T., Han, C. B., et al. (2017a). Smart Floor with Integrated Triboelectric Nanogenerator as Energy Harvester and Motion Sensor. ACS Appl. Mat. Interfaces 9, 26126–26133. doi:10.1021/acsami.7b08526
He, C., Zhu, W., Gu, G. Q., Jiang, T., Xu, L., Chen, B. D., et al. (2017b). Integrative Square-Grid Triboelectric Nanogenerator as a Vibrational Energy Harvester and Impulsive Force Sensor. Nano Res. 11, 1157–1164. doi:10.1007/s12274-017-1824-8
Hinchet, R., Yoon, H.-J., Ryu, H., Kim, M.-K., Choi, E.-K., Kim, D.-S., et al. (2019). Transcutaneous Ultrasound Energy Harvesting Using Capacitive Triboelectric Technology. Science 365, 491–494. doi:10.1126/science.aan3997
Kutcher, J. S., McCrory, P., Davis, G., Ptito, A., Meeuwisse, W. H., and Broglio, S. P. (2013). What Evidence Exists for New Strategies or Technologies in the Diagnosis of Sports Concussion and Assessment of Recovery? Br. J. Sports Med. 47, 299–303. doi:10.1136/bjsports-2013-092257
Leber, A., Cholst, B., Sandt, J., Vogel, N., and Kolle, M. (2018). Stretchable Thermoplastic Elastomer Optical Fibers for Sensing of Extreme Deformations. Adv. Funct. Mat. 29, 1802629. doi:10.1002/adfm.201802629
Lee, J.-H., Lee, K. Y., Gupta, M. K., Kim, T. Y., Lee, D.-Y., Oh, J., et al. (2013). Highly Stretchable Piezoelectric-Pyroelectric Hybrid Nanogenerator. Adv. Mat. 26, 765–769. doi:10.1002/adma.201303570
Lee, K. Y., Yoon, H. J., Jiang, T., Wen, X., Seung, W., Kim, S. W., et al. (2016). Fully Packaged Self‐Powered Triboelectric Pressure Sensor Using Hemispheres‐Array. Adv. Energy Mat. 6, 1502566. doi:10.1002/aenm.201502566
Lin, Z., Wu, Z., Zhang, B., Wang, Y.-C., Guo, H., Liu, G., et al. (2018). A Triboelectric Nanogenerator-Based Smart Insole for Multifunctional Gait Monitoring. Adv. Mat. Technol. 4, 1800360. doi:10.1002/admt.201800360
Liu, W., Wang, Z., Wang, G., Liu, G., Chen, J., Pu, X., et al. (2019a). Integrated Charge Excitation Triboelectric Nanogenerator. Nat. Commun. 10, 1426. doi:10.1038/s41467-019-09464-8
Liu, Z., Zhao, Z., Zeng, X., Fu, X., and Hu, Y. (2019b). Expandable Microsphere-Based Triboelectric Nanogenerators as Ultrasensitive Pressure Sensors for Respiratory and Pulse Monitoring. Nano Energy 59, 295–301. doi:10.1016/j.nanoen.2019.02.057
Lu, Q., Chen, H., Zeng, Y., Xue, J., Cao, X., Wang, N., et al. (2022). Intelligent Facemask Based on Triboelectric Nanogenerator for Respiratory Monitoring. Nano Energy 91, 106612. doi:10.1016/j.nanoen.2021.106612
Lu, X., Zheng, L., Zhang, H., Wang, W., Wang, Z. L., and Sun, C. (2020). Stretchable, Transparent Triboelectric Nanogenerator as a Highly Sensitive Self-Powered Sensor for Driver Fatigue and Distraction Monitoring. Nano Energy 78, 105359. doi:10.1016/j.nanoen.2020.105359
Luo, J., Wang, Z., Xu, L., Wang, A. C., Han, K., Jiang, T., et al. (2019). Flexible and Durable Wood-Based Triboelectric Nanogenerators for Self-Powered Sensing in Athletic Big Data Analytics. Nat. Commun. 10, 5147. doi:10.1038/s41467-019-13166-6
Maharjan, P., Toyabur, R. M., and Park, J. Y. (2018). A Human Locomotion Inspired Hybrid Nanogenerator for Wrist-Wearable Electronic Device and Sensor Applications. Nano Energy 46, 383–395. doi:10.1016/j.nanoen.2018.02.033
Meng, B., Tang, W., Too, Z.-H., Zhang, X., Han, M., Liu, W., et al. (2013). A Transparent Single-Friction-Surface Triboelectric Generator and Self-Powered Touch Sensor. Energy Environ. Sci. 6, 3235. doi:10.1039/c3ee42311e
Meng, K., Zhao, S., Zhou, Y., Wu, Y., Zhang, S., He, Q., et al. (2020). A Wireless Textile-Based Sensor System for Self-Powered Personalized Health Care. Matter 2, 896–907. doi:10.1016/j.matt.2019.12.025
Meng, X. S., Li, H. Y., Zhu, G., and Wang, Z. L. (2015). Fully Enclosed Bearing-Structured Self-Powered Rotation Sensor Based on Electrification at Rolling Interfaces for Multi-Tasking Motion Measurement. Nano Energy 12, 606–611. doi:10.1016/j.nanoen.2015.01.015
Parida, K., Thangavel, G., Cai, G., Zhou, X., Park, S., Xiong, J., et al. (2019). Extremely Stretchable and Self-Healing Conductor Based on Thermoplastic Elastomer for All-Three-Dimensional Printed Triboelectric Nanogenerator. Nat. Commun. 10, 2158. doi:10.1038/s41467-019-10061-y
Park, J., Kim, D., and Kim, Y. T. (2021). “Triboelectric Nanogenerator Based E-Skin for Wearable Energy Harvesting and Pressure Sensing,” in 2021 IEEE 21st International Conference on Nanotechnology (NANO), Montreal, QC, 28–30 July 2021 (IEEE). doi:10.1109/nano51122.2021.9514293
Peng, F., Liu, D., Zhao, W., Zheng, G., Ji, Y., Dai, K., et al. (2019). Facile Fabrication of Triboelectric Nanogenerator Based on Low-Cost Thermoplastic Polymeric Fabrics for Large-Area Energy Harvesting and Self-Powered Sensing. Nano Energy 65, 104068. doi:10.1016/j.nanoen.2019.104068
Pu, X., Guo, H., Chen, J., Wang, X., Xi, Y., Hu, C., et al. (2017a). Eye Motion Triggered Self-Powered Mechnosensational Communication System Using Triboelectric Nanogenerator. Sci. Adv. 3, e1700694. doi:10.1126/sciadv.1700694
Pu, X., Liu, M., Chen, X., Sun, J., Du, C., Zhang, Y., et al. (2017b). Ultrastretchable, Transparent Triboelectric Nanogenerator as Electronic Skin for Biomechanical Energy Harvesting and Tactile Sensing. Sci. Adv. 3, e1700015. doi:10.1126/sciadv.1700015
Pu, X., Guo, H., Tang, Q., Chen, J., Feng, L., Liu, G., et al. (2018). Rotation Sensing and Gesture Control of a Robot Joint via Triboelectric Quantization Sensor. Nano Energy 54, 453–460. doi:10.1016/j.nanoen.2018.10.044
Rahman, M. T., Rana, S. S., Salauddin, M., Maharjan, P., Bhatta, T., Kim, H., et al. (2020). A Highly Miniaturized Freestanding Kinetic-Impact-Based Non-resonant Hybridized Electromagnetic-Triboelectric Nanogenerator for Human Induced Vibrations Harvesting. Appl. Energy 279, 115799. doi:10.1016/j.apenergy.2020.115799
Ren, Z., Zheng, Q., Wang, H., Guo, H., Miao, L., Wan, J., et al. (2020). Wearable and Self-Cleaning Hybrid Energy Harvesting System Based on Micro/Nanostructured Haze Film. Nano Energy 67, 104243. doi:10.1016/j.nanoen.2019.104243
Salauddin, M., Rana, S. M. S., Sharifuzzaman, M., Rahman, M. T., Park, C., Cho, H., et al. (2020). A Novel MXene/Ecoflex Nanocomposite‐Coated Fabric as a Highly Negative and Stable Friction Layer for High‐Output Triboelectric Nanogenerators. Adv. Energy Mater. 11, 2002832. doi:10.1002/aenm.202002832
Seung, W., Gupta, M. K., Lee, K. Y., Shin, K.-S., Lee, J.-H., Kim, T. Y., et al. (2015). Nanopatterned Textile-Based Wearable Triboelectric Nanogenerator. ACS Nano 9, 3501–3509. doi:10.1021/nn507221f
Sheng, F., Yi, J., Shen, S., Cheng, R., Ning, C., Ma, L., et al. (2021). Self-Powered Smart Arm Training Band Sensor Based on Extremely Stretchable Hydrogel Conductors. ACS Appl. Mat. Interfaces 13, 44868–44877. doi:10.1021/acsami.1c12378
Shi, Q., and Lee, C. (2019). Self‐Powered Bio‐Inspired Spider‐Net‐Coding Interface Using Single‐Electrode Triboelectric Nanogenerator. Adv. Sci. 6, 1900617. doi:10.1002/advs.201900617
Shi, Q., Zhang, Z., He, T., Sun, Z., Wang, B., Feng, Y., et al. (2020). Deep Learning Enabled Smart Mats as a Scalable Floor Monitoring System. Nat. Commun. 11, 4609. doi:10.1038/s41467-020-18471-z
Shi, Y., Wei, X., Wang, K., He, D., Yuan, Z., Xu, J., et al. (2021). Integrated All-Fiber Electronic Skin toward Self-Powered Sensing Sports Systems. ACS Appl. Mat. Interfaces 13, 50329–50337. doi:10.1021/acsami.1c13420
Tang, Y., Xuan, W., Zhang, C., Xu, L., Liu, F., Chen, J., et al. (2021). Fully Self-Powered Instantaneous Wireless Traffic Monitoring System Based on Triboelectric Nanogenerator and Magnetic Resonance Coupling. Nano Energy 89, 106429. doi:10.1016/j.nanoen.2021.106429
Wang, R., Mu, L., Bao, Y., Lin, H., Ji, T., Shi, Y., et al. (2020a). Holistically Engineered Polymer-Polymer and Polymer-Ion Interactions in Biocompatible Polyvinyl Alcohol Blends for High‐Performance Triboelectric Devices in Self‐Powered Wearable Cardiovascular Monitorings. Adv. Mat. 32, 2002878. doi:10.1002/adma.202002878
Wang, S., He, M., Weng, B., Gan, L., Zhao, Y., Li, N., et al. (2018). Stretchable and Wearable Triboelectric Nanogenerator Based on Kinesio Tape for Self-Powered Human Motion Sensing. Nanomaterials 8, 657. doi:10.3390/nano8090657
Wang, S., Lin, L., and Wang, Z. L. (2015a). Triboelectric Nanogenerators as Self-Powered Active Sensors. Nano Energy 11, 436–462. doi:10.1016/j.nanoen.2014.10.034
Wang, S., Tai, H., Liu, B., Duan, Z., Yuan, Z., Pan, H., et al. (2019). A Facile Respiration-Driven Triboelectric Nanogenerator for Multifunctional Respiratory Monitoring. Nano Energy 58, 312–321. doi:10.1016/j.nanoen.2019.01.042
Wang, X., Yin, Y., Yi, F., Dai, K., Niu, S., Han, Y., et al. (2017). Bioinspired Stretchable Triboelectric Nanogenerator as Energy-Harvesting Skin for Self-Powered Electronics. Nano Energy 39, 429–436. doi:10.1016/j.nanoen.2017.07.022
Wang, Y., Chu, J., Zhang, R., Wang, L., and Wang, Z. (2015b). A Novel Autonomous Real-Time Position Method Based on Polarized Light and Geomagnetic Field. Sci. Rep. 5, 9725. doi:10.1038/srep09725
Wang, Z., An, J., Nie, J., Luo, J., Shao, J., Jiang, T., et al. (2020b). A Self‐Powered Angle Sensor at Nanoradian‐Resolution for Robotic Arms and Personalized Medicare. Adv. Mat. 32, 2001466. doi:10.1002/adma.202001466
Wang, Z. L., Chen, J., and Lin, L. (2015c). Progress in Triboelectric Nanogenerators as a New Energy Technology and Self-Powered Sensors. Energy Environ. Sci. 8, 2250–2282. doi:10.1039/c5ee01532d
Wang, Z. L. (2017). On Maxwell's Displacement Current for Energy and Sensors: the Origin of Nanogenerators. Mater. Today 20, 74–82. doi:10.1016/j.mattod.2016.12.001
Wang, Z. L. (2020). On the First Principle Theory of Nanogenerators from Maxwell's Equations. Nano Energy 68, 104272. doi:10.1016/j.nanoen.2019.104272
Wang, Z. L. (2014). Triboelectric Nanogenerators as New Energy Technology and Self-Powered Sensors - Principles, Problems and Perspectives. Faraday Discuss. 176, 447–458. doi:10.1039/c4fd00159a
Wen, F., Sun, Z., He, T., Shi, Q., Zhu, M., Zhang, Z., et al. (2020). Machine Learning Glove Using Self‐Powered Conductive Superhydrophobic Triboelectric Textile for Gesture Recognition in VR/AR Applications. Adv. Sci. 7, 2000261. doi:10.1002/advs.202000261
Wen, Z., Yeh, M. H., Guo, H., Wang, J., Zi, Y., Xu, W., et al. (2016). Self-Powered Textile for Wearable Electronics by Hybridizing Fiber-Shaped Nanogenerators, Solar Cells, and Supercapacitors. Sci. Adv. 2, e1600097. doi:10.1126/sciadv.1600097
Wiorek, A., Parrilla, M., Cuartero, M., and Crespo, G. A. (2020). Epidermal Patch with Glucose Biosensor: pH and Temperature Correction toward More Accurate Sweat Analysis during Sport Practice. Anal. Chem. 92, 10153–10161. doi:10.1021/acs.analchem.0c02211
Wu, Z., Zhang, B., Zou, H., Lin, Z., Liu, G., and Wang, Z. L. (2019). Multifunctional Sensor Based on Translational‐Rotary Triboelectric Nanogenerator. Adv. Energy Mat. 9, 1901124. doi:10.1002/aenm.201901124
Yamada, T., Hayamizu, Y., Yamamoto, Y., Yomogida, Y., Izadi-Najafabadi, A., Futaba, D. N., et al. (2011). A Stretchable Carbon Nanotube Strain Sensor for Human-Motion Detection. Nat. Nanotech 6, 296–301. doi:10.1038/nnano.2011.36
Yang, D., Ni, Y., Kong, X., Li, S., Chen, X., Zhang, L., et al. (2021). Self-Healing and Elastic Triboelectric Nanogenerators for Muscle Motion Monitoring and Photothermal Treatment. ACS Nano 15, 14653–14661. doi:10.1021/acsnano.1c04384
Yin, X., Liu, D., Zhou, L., Li, X., Xu, G., Liu, L., et al. (2020). A Motion Vector Sensor via Direct‐Current Triboelectric Nanogenerator. Adv. Funct. Mater. 30, 2002547. doi:10.1002/adfm.202002547
Yuan, M., Li, C., Liu, H., Xu, Q., and Xie, Y. (2021). A 3d-Printed Acoustic Triboelectric Nanogenerator for Quarter-Wavelength Acoustic Energy Harvesting and Self-Powered Edge Sensing. Nano Energy 85, 105962. doi:10.1016/j.nanoen.2021.105962
Zhang, C., Chen, J., Xuan, W., Huang, S., Shi, L., Cao, Z., et al. (2022). Triboelectric Nanogenerator-Enabled Fully Self-Powered Instantaneous Wireless Sensor Systems. Nano Energy 92, 106770. doi:10.1016/j.nanoen.2021.106770
Zhang, H., Zhang, J., Hu, Z., Quan, L., Shi, L., Chen, J., et al. (2019). Waist-Wearable Wireless Respiration Sensor Based on Triboelectric Effect. Nano Energy 59, 75–83. doi:10.1016/j.nanoen.2019.01.063
Zhang, Q., Liang, Q., Liao, Q., Ma, M., Gao, F., Zhao, X., et al. (2018). An Amphiphobic Hydraulic Triboelectric Nanogenerator for a Self-Cleaning and Self-Charging Power System. Adv. Funct. Mat. 28, 1803117. doi:10.1002/adfm.201803117
Zhao, Z., Huang, Q., Yan, C., Liu, Y., Zeng, X., Wei, X., et al. (2020). Machine-Washable and Breathable Pressure Sensors Based on Triboelectric Nanogenerators Enabled by Textile Technologies. Nano Energy 70, 104528. doi:10.1016/j.nanoen.2020.104528
Zhou, Z., Padgett, S., Cai, Z., Conta, G., Wu, Y., He, Q., et al. (2020). Single-Layered Ultra-Soft Washable Smart Textiles for All-Around Ballistocardiograph, Respiration, and Posture Monitoring during Sleep. Biosens. Bioelectron. 155, 112064. doi:10.1016/j.bios.2020.112064
Zhu, M., Sun, Z., Zhang, Z., Shi, Q., He, T., Liu, H., et al. (2020). Haptic-Feedback Smart Glove as a Creative Human-Machine Interface (HMI) for Virtual/augmented Reality Applications. Sci. Adv. 6, eaaz8693. doi:10.1126/sciadv.aaz8693
Zhu, M., Shi, Q., He, T., Yi, Z., Ma, Y., Yang, B., et al. (2019). Self-Powered and Self-Functional Cotton Sock Using Piezoelectric and Triboelectric Hybrid Mechanism for Healthcare and Sports Monitoring. ACS Nano 13 (2), 1940–1952. doi:10.1021/acsnano.8b08329
Zou, Y., Libanori, A., Xu, J., Nashalian, A., and Chen, J. (2020). Triboelectric Nanogenerator Enabled Smart Shoes for Wearable Electricity Generation. Research 2020, 7158953. doi:10.34133/2020/7158953
Keywords: sports, TENG, physical fitness, human–machine interactive, energy harvesting
Citation: Zhang J, Yang Z and Liang X (2022) Development and Prospects of Triboelectric Nanogenerators in Sports and Physical State Monitoring. Front. Mater. 9:902499. doi: 10.3389/fmats.2022.902499
Received: 23 March 2022; Accepted: 21 April 2022;
Published: 27 May 2022.
Edited by:
Wenai Shen, Huazhong University of Science and Technology, ChinaReviewed by:
Xinglong Gong, University of Science and Technology of China, ChinaXinyu Xue, Northeastern University, China
Dan Li, Zhengzhou University, China
Copyright © 2022 Zhang, Yang and Liang . This is an open-access article distributed under the terms of the Creative Commons Attribution License (CC BY). The use, distribution or reproduction in other forums is permitted, provided the original author(s) and the copyright owner(s) are credited and that the original publication in this journal is cited, in accordance with accepted academic practice. No use, distribution or reproduction is permitted which does not comply with these terms.
*Correspondence: Xin Liang, x.liang@hfuu.edu.cn
†These authors have contributed equally to this work and share first authorship