- 1Department of Fire Engineering, University of Zilina, Zilina, Slovakia
- 2Department of Crisis Management, University of Zilina, Zilina, Slovakia
- 3Department of Wood and Paper Science and Technology, College of Agriculture and Natural Resources, University of Tehran, Tehran, Iran
Wood-based composite materials represent a major fire safety risk. The application of water-based fire retardants (FR) can improve their fire performance. However, it is important to investigate the performance and stability of this protective system over time, as the ageing of materials and their exposure to climatic conditions can change their properties. The aim of this research work was to evaluate the effect of long-term aging on the combustibility of low-density insulation fibreboards (IFs) treated with a water-soluble flame retardant (FR) based on inorganic salts. Both natural and accelerated aging simulations were conducted. The accelerated aging simulation was performed using climate chambers. The FR efficiency was evaluated by the mass loss rate of the tested specimen. As expected, the FR formulation used significantly enhanced the fire properties of the boards, reaching maximum efficiency 6 months after its application onto the fibreboards. Markedly, after 4 years the efficiency of the investigated FR additive was considerably decreased with weight loss values comparable with the values of the untreated samples. Markedly, besides the apparent protection of wood fibers, the retardant did not prevent the gradual increase in mass loss rate of samples, it just slowed down the process. The main result of the study is to determine the effectiveness of the investigated FR on IFs during their natural ageing. The development of accurate datasets on the thermal behaviour of IFs is critical for their proper utilisation in building and construction applications.
Introduction
The use of wood and wood-based composites in a wide range of applications, including building and construction, is limited by numerous fire safety requirements, imposed throughout Europe, related to its flammability and spread of fire characteristics (Ayrilmiş, 2007; Sydor and Wieloch, 2009; Bekhta et al., 2016; Bryn et al., 2016; Cai et al., 2016; Antov et al., 2019; Savov and Antov, 2020). Accidental fires of wood-based composites represent a major safety risk, making it essential to have a proper understanding of their fire behaviour.
In Europe, construction products and building elements are classified regarding their fire resistance into the following seven classes: A1, A2, B, C, D, E and F (EN 13501-1, 2019). In recent years, the insulation wooden fiberboards (IFs) are one of the most commonly used natural thermal insulation materials in civil engineering. Most often IF are used for insulation of new buildings, wooden buildings, low-energy houses and also for additional insulation of older buildings. Regarding to the intended applications of IFs, always their fire resistance, heat insulation, and toxic emissions need to be considered (Segovia et al., 2020). IFs have fairly low emissions (of the formaldehyde and the total volatile organic com-pounds) with good insulation characteristics, but their fire resistance must be enhanced to fulfil standard requirements when used in the building sector. The IFs are usually classified in classes E or F, i.e., easily flammable materials, mainly due to their low density (≈200 kg m−3) and content of highly flammable plant fibres (Reinprecht, 2016).
Fire behaviour of wood-based composites has been a major concern of both academia and industry, and many studies have been focused on evaluation and investigation of the development and application of different means to enhance their fire resistance. A number of studies have investigated the fire resistance properties of IFs. For example, a study (Tang et al., 2017) investigates the fire behaviour of standard fibreboard and high-density fibreboard using DTG and TGA methods. The results indicate that ultra-high-density fibreboards have better fire performance. The fire and smouldering behaviour of fibreboards investigated by (Madyaratri et al., 2022). (Kadlicova et al., 2017) monitored weight loss in the heat sources by delaying the time depending on the heat sources and the type of fibreboard. Martinka et al., 2021 describe the insulation behaviour of wood-based materials during thermal loading by thermogravimetric analysis and by calculating the activation energy.
Two different approaches have been proposed to increase the fire resistance of IFs: I.) use of appropriate binders (Bryn et al., 2016), and II.) addition of fire retarding chemicals during the production process (Östman, 1984; Ayrilmiş, 2007; Chen et al., 2016; Wu et al., 2017). These specific fire retarding compounds, applied either in liquid or dry state, and commonly known as flame retardants (FRs), are used to treat solid wood, fibers or particles to reduce their flammability (Bekhta et al., 2016; Bryn et al., 2016; Igaz et al., 2017; Mantanis et al., 2018; Martinka et al., 2018; Němec et al., 2019). FRs enhance the formation of increased char layer on the surface of wood-based panels and reduce the amount of combustible volatile vapours (Rowel, 2012). In general, fire resistant wood-based composites are manufactured by treatments with nitrogen-, boron-, phosphorus-, zinc-, and aluminium-containing chemicals (Jieyu et al., 1994; Pizzi and Mittal, 2003; Mazela and Broda, 2015; Lin C.-F. et al., 2020; Mantanis et al., 2020). In the production of fibreboards, FRs are added to the fibres in the blowline as aqueous solutions or suspensions, having 50–60% active solids content (Mantanis, 2002; Yotov et al., 2015; Wang et al., 2016; Mantanis et al., 2018).
In the preliminary study, the post treatment of IFs with a commercial water-based FR showed the ability to improve their reaction-to-fire performance from the E to B according to the Euroclass system (EN 13501-1, 2019). Post treatment of IFs with a FR formulation either through immersion or spray applications provides an envelope protection. In this system, the products remain at the surface where they are most needed to form a protective barrier against fire. No previous studies have examined the performance and stability of this protection system over time.
Therefore, the aim of this research work was to evaluate the effect of long-term aging on the fire performance of low-density IFs treated with a water-soluble FR composed of 20% ammonium hydrogenphosphate and 10% ammonium sulfate.
Materials and Methods
Specimens were divided into five groups, which were tested over a 6-months time span. A control group of untreated specimens was also tested to provide baseline data.
60-mm thick insulation wooden fibreboard (IF) (Steico protect, 2020) made out of three layers were chosen for this study (Figure 1A). The dimensions of each specimen were 90 × 80 × 60 mm (Length × Width × Thickness) and the average volumetric mass density was 170 kg m−3.
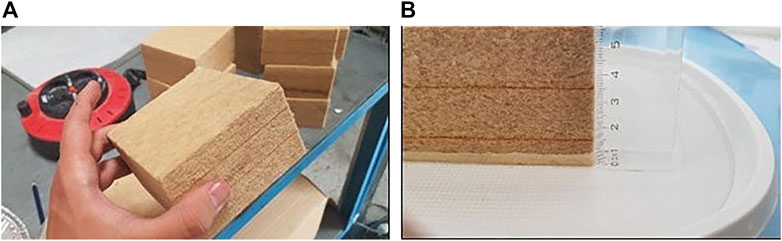
FIGURE 1. Insulation wooden fibreboard (IF). (A) The IFs specimens used for conducting the experiments; (B) Impregnation of samples in FR solution.
Specimens were divided into six groups, A/B/C/D/E/V where groups A-E corresponded to the aging of each group, with A being tested soon after application of the flame retardant and E being tested after 2 years of natural aging (Table 1). Along with these, a group of untreated specimens designated as V, was tested to provide baseline data for further comparison. Each group contained 10 specimens to ensure higher accuracy of results.
A two-year-long simulation of natural aging was conducted to procure indications and estimate predictions of the changes in fire resistance of coated low-density fiberboards. The simulation was carried out in conditions of real exterior construction.
A water-based FR, i.e., a solution of inorganic salts in water (with a composition of 20% ammonium hydrogenphosphate and 10% ammonium sulfate), was used for treating the IFs. It is described as a transparent liquid with a light urea odor, completely miscible with water, with a density up to 1,180–1,200 kg m−3. Just like fibreboards, FR is fully degradable in nature, neither causing toxic contamination of the soil nor any undesirable ecological consequences in general. The FR can be combined with fungicide and insecticide agents when applied onto wooden materials (Lowden and Hull, 2013; Lee et al., 2019; Lin C.-f. et al., 2020).
Each specimen was soaked to a depth of 1 cm into 250 ml of the FR. The impregnation process took 15 min and is displayed in Figure 1B. The specimens that did not undergo the aging simulation were not tested immediately after the FR application, but 14 days after application.
Reaction to fire was measured using mass loss of the specimens during the test. The surface of specimens was exposed to a propane-butane torch with a 25 mm flame at a 90° angle (Figure 2A). Mass loss was automatically monitored in 15 s intervals for 10 min using BalanceLink software (Mettler Toledo, Barcelona, Spain). Specimens were exposed to the flame only for the first 2 min of the experiment. Continuity of combustion was observed for the remaining 8 min.
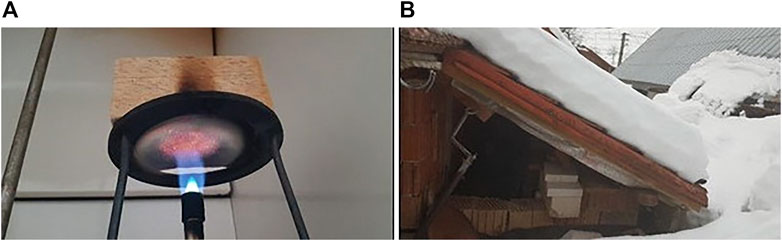
FIGURE 2. (A) Fiberboard specimen exposed to propane-butane torch; (B) Natural aging simulation site.
The simulation of natural aging was carried out in a rural area in northwest Slovakia (Púchov district) in a north-facing, roof-protected site which is part of a multigenerational house. This site provided protection from direct sunlight, rain, snow and strong wind. The main parameters affecting specimens’ aging were air t emperature and humidity (Figure 2B). Specimens were placed with gaps between them in order to ensure even distribution of airflow for each sample. The natural aging simulation lasted 2 years until the last group of specimens (E) was tested.
Results
The results of the experiments were subjected to a statistical analysis, which consisted of three parts: (1) comparison of the differences between samples (analysis of difference) and prediction of the aging material’s ability to resist the effects of combustion (mass loss rate change in the future); (2) correlation analysis of selected parameters among samples (analysis of dependence); (3) prediction of the burning rate development in samples over 2 years of age.
Analysis of Difference
Firstly, we investigated whether there were significant differences between the samples with respect to each selected variable individually (mass loss over time and mass loss rate over time). This analysis was made based on the assumption that if, after a certain period of time, mass loss and mass loss rate of the material does not change substantially for the samples, it can be assumed that this will not change substantially even later on. The confirmation of this assumption will help us to predict the development of the flame-resistance capability of the material and to establish an appropriate regression function for the prediction of the burning development.
For this reason, we have made a step-by-step comparison of the individual samples. The comparison was carried out primarily using statistical testing (paired t-test) and supplemented by graphical outputs to compare the difference in mass loss and the mass loss rate the samples (Figure 3A, Figure 3B). A paired t-test is generally used to compare two groups where each entity is measured twice, including repeated-measures designs (in our case, the same sample of different ages), resulting in pairs of observation. Paired t-tests were performed separately for the parameter “mass loss” and separately for “mass loss rate” for all sample combinations (time-linked: A-B, B-C, C-D, D-E, E-V, A-V).
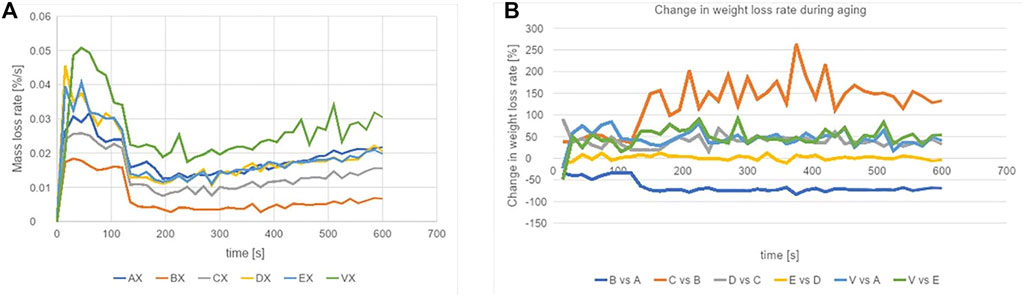
FIGURE 3. (A) Comparison of mass loss rates between the individual samples; (B) Change in mass loss rate of samples of different ages.
As part of the statistical testing, we tested an alternative hypothesis (H1): there is a significant difference between the samples (during aging) against the zero hypothesis (H0): there is no statistically significant difference between the samples.
The t-test results showed that there were statistically significant differences between almost all samples (at significance level p < 0.01) in terms of mass loss as well as mass loss rate. Sample A differed significantly from sample B in terms of mass loss: p = 7.30E-28; sample B differed significantly from sample C: p = 5.61E-23; sample C differed significantly from sample D: p = 9.71E-13; sample E differed significantly from sample V: p = 5.83E-13; sample A differed significantly from sample V: p = 1.03E-15. However, statistically significant differences between D and E samples were not confirmed (p = 0.85) indicating their statistical similarity (Table 2).
A graphical representation of the comparison of samples in terms of total mass loss and relative change in mass loss is shown in Figure 4A and Figure 4B, respectively. To note, after almost 24 months (2 years, or between samples D and E), there was already a slight change in mass loss, indicating that D and E samples have similar characteristics in this respect. Minor deviations can be seen in the initialization phase—up to 180 s, then development stabilizes. Major differences in this phase are obvious in other comparisons.
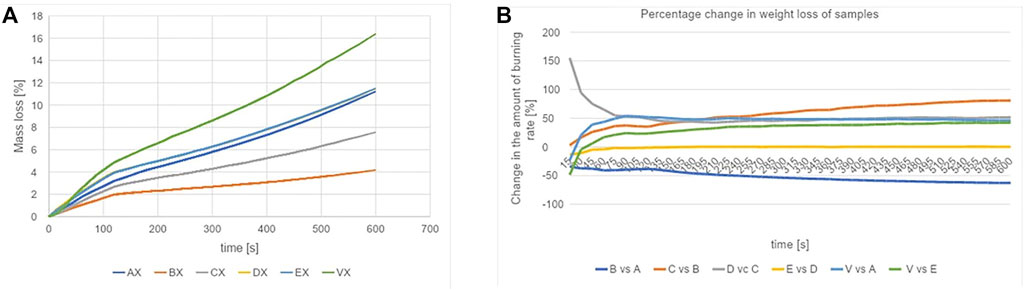
FIGURE 4. (A) Comparison of mass loss between individual samples; (B) Percentage change in mass loss in samples.
This is proven by the other charts comparing the change in mass loss rate during aging (Figure 3A and Figure 3B). As in previous charts, it is clear that after 24 months, the changes between samples were minimal because the percentage changes oscillate around zero.
Overall, Figure 5A summarizes the difference in samples. It clearly shows that sample B (6 months after impregnation) had the best fire properties. In the course of the following year, these properties deteriorated (up to 18 months) and then began to stabilize. The 1.5 and 2-year-old samples had very similar characteristics to the sample which was impregnated and immediately subjected to the experiment (sample A—0 months). It is therefore clear that after 6 months, the impregnating properties deteriorate and mass loss during burning increases for samples subjected to the experiment.
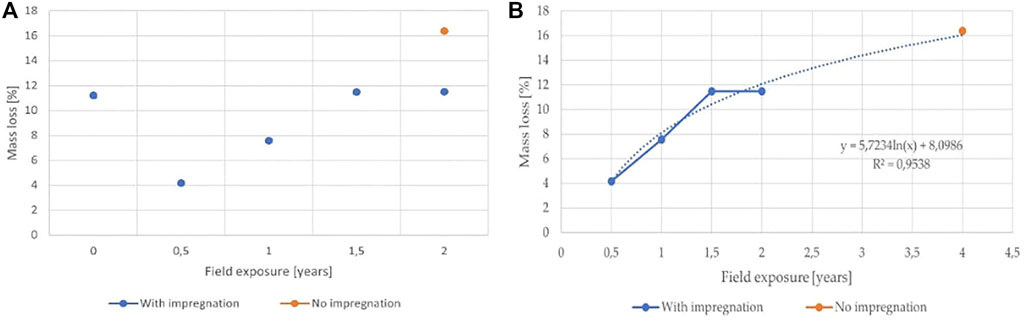
FIGURE 5. (A) Mass loss over field exposure (all samples); (B) Prediction of the development of mass loss in samples over 2 years of age.
After 6 months, the development of burning can be predicted for the samples older than the observation period, but the prediction is a bit distorted (Figure 5B). If sample A is included in the prediction model, then the time period mentioned above, showing positive features of the FR (between samples A and B), would fundamentally affect the entire forecast. For a more accurate forecast, it is appropriate to follow a longer period of time, which was not possible in this case as the experiment was part of a doctoral project. The forecast was carried out using a logarithmic function, which, with its increasing course and the coefficient of reliability, corresponds most logically to the possible development of older samples.
After 4 years, with the reliability of the forecast (R2 = 0.95), it can be claimed that the effects of the retarder will disappear (mass loss = 16.03%).
In addition, the actual duration of the loss of efficiency of the FR may be significantly longer, as there is an increase in the amount of material burned by only 0.024% between the last E (11.497%) and D (11.473%) samples. If such a low pace of deterioration is maintained, the level of the V sample (16.387% of the mass loss) would be reached by the fire-treated sample after approximately 104 years (calculated as linear development of the amount of material burnt from sample D (11.473%) to sample V (16.387%)).
Analysis of Dependence
For an assessment of the relationship between the time (independent variable) and a) mass loss and b) mass loss rate (dependent variables) the results of experiments were subjected to the dependence analysis (Table 3). Pearson’s correlation coefficients were used to explore the relationships between (1) the dependent variables “mass loss” and independent variable “time” and (2) the dependent variable “mass loss rate” and independent variable “time”.
It is clear that there is a linear dependence between time and mass loss (high correlation coefficient). Dependence rate between time and mass lose rate is lower what means that there are also other features that affect it.
Prediction of the Burning Rate Development in Samples Over 2 years of Age
We developed the model using the method of the smallest squares using linear regression. The model was divided into two phases, the initiation phase and the spontaneous burning phase. An analysis of the entire burning process found that there were significant differences between these phases, which is clearly demonstrated in Figure 6. In view of the burning forecast, the spontaneous burning phase is more important for the purposes of this article, as the initiation phase was limited to 2 minutes during which the samples were exposed to direct flame.
The differences were caused by a fundamental change in the course of the experiment, when the sample was put aside from the flame source and thus entered the spontaneous burning phase. In view of these differences, the process of burning was divided into two main parts which were evaluated separately. The division of the experiment into these two phases results in more precise and reliable predictions that would influence each other without making them separate. The burner was put away after 120 s of the experiment, but the boundary for the separation phase had been set at 150 s. During the intervening 30 s, spontaneous burning should stabilize as the effect of the flame on the burning rate of the sample continued for a short period even after it was shut down.
Initiation phase: The burning speed immediately rises after exposure to a flame and is highest in the first tens of seconds. With certain variations, it decreases linearly (R2 = 0.86, p < 0.01) almost until the end of the third minute (Figure 6).
Spontaneous burning phase: the correlation between the burning rate and the time of burning is shown in Figure 7. The parameters of models were calculated using Excel software, after the data from the experiments were inserted.
The resulting model equation is stated in the formula 1.
Mass loss rate = 0.0003; Burning Time + 0,0113.
The linear dependence between these variables (r = 0.95; p < 0.01) allows the development of a dependent variable to be anticipated with sufficient reliability (R2 = 0.91, p < 0.01). A reliability R2 = 0.91 means that 90.95% of the variability of the explained variable is explained by the variability of the explanatory variable. The remaining 9.05% variability is influenced by the variability of other factors not considered in the model (in (1) this is represented by “u”). Thus, the mass loss rate will increase linearly. Figure 8 also includes a development forecast for another 2 min of burning at a confidence level of 95%, which are seen as the lower and upper confidence limits.
The model can be used for prognostic purposes when the test conditions are met and we have therefore started testing the model. The model as a whole (Fisher F test), model parameter (t-test), autocorrelation and heteroscedasticity were tested. Results of the tests are shown in the Table 4 below.
The results indicate that the model passed all tests successfully and is applicable for prognostic purposes at a significance level of α = 0.05. Testing the coefficient of determination by Fisher’s distribution showed that the value of the model is very high. Parameter b1 has an error rate of only p = 1.43E-12, which does not affect the model at the selected significance level. The Durban-Watt test shows that the autocorrelation of residues does not occur in the model. Based on the heteroscedasticity test (Goldfeld-Quandt test), we can declare that heteroscedasticity is present in the model, i.e. there is a constant dispersion of residues around the regression line.
Discussion
One of the main drawbacks for the use of wood-based composites in the construction industry and other structural applications is their inherent flammability and combustibility. Fibrous lignocellulose-based materials, classified as environmentally friendly and economically advantageous, are preferred. The fact that they belong to fire class E puts them at a disadvantage and it is debatable to what extent they guarantee meeting high fire protection requirements. It is logical that the issue of fire safety of these materials is given great attention. In the construction industry, this applies to the assessment of both European (White, 2009; Makovická Osvaldová and Osvald, 2013; Makovicka Osvaldova et al., 2020) and tropical wood species and their modifications (Winandy, 2001). The processing of wood into large-scale materials also requires attention. Many large-scale materials, even the wood-based ones, have their own burning specificities depending on their modified density and volume-to-surface ratio (Osvald et al., 2016).
A wide variety of FR compounds are commonly used, enabling wood-based composites with poor fire characteristics to fulfill the required fire performance criteria, widening their range of potential applications. Each large-scale material structure allows for a different application of retardant (Lee et al., 2019), adhesive mixture (plywood), or veneer impregnation. In the case of the chipboard and oriented strand board, the application of a solid FR to the mixture before pressing is also possible. In this case, fibreboards are quite limited to surface treatment by painting, spraying, or dipping. Based on these limited options for our fibreboard modification, the focus is on the modifications described in the methodology of the article.
The results of the present study showed that the peak efficiency of the FR occurred 6 months after its application (group B) and not immediately after its application (group A), which makes it somewhat more difficult to forecast other development due to aging. Because the experimental intervals are set at every 6 months, a more detailed experiment with smaller intervals should be carried out to more precisely determine the peak of efficacy. However, the peak is not as significant as the actual development of efficiency due to aging, as these results indicate. A downward trend in the efficiency of the FR with a gradual aging of the samples can be observed, except for groups A and B, where significant improvements can be seen.
Continuous smoldering with an increasing burning rate is a phenomenon that is caused by low density and high airiness of samples that practically completely burn away, which is not the case, for example, with wooden plywood which (under the same conditions) slows the burning rate until self-extinguishing occurs. This is a fundamental difference in the burning process between fiberboards and other wood-based materials.
The explanatory variable mentioned in Eq. 1 is not the only one that affects the mass loss rate, but it was the main indicator observed from the point of view of experiments. Other factors on which the “y” depends include, for example, surface defects such as chipped material particles or fraying of otherwise pressed fibers of the test surface. In the case of fiberboards, the occurrence of bigger wood fibers that were not finely crushed during the manufacturing process also influences the mass loss rate. These are factors that affect the mass loss rate, but they are difficult to integrate into the formation of prediction models.
In conclusion, just a few words on the test methodology. Each test method simulates more or less real fire conditions. It is also important which type of material is burning. If we were to evaluate everything using just one method, we might not evaluate every material properly. Therefore, we modified our test method so that its evaluation takes into consideration the fire performance in fiberboard materials (Osvald et al., 2016).
Conclusion
The first and fundamental conclusion of this research is that the FR used in the experiment had a significant impact on the resistance of fibreboards to heat, as demonstrated by the reduction in mass loss on the samples where it had been applied.
The primary objective of the experiments was to assess the ability of fibreboard samples to maintain improved fire resistance through the use of a flame retardant over time. However, as the data showed, evaluating this property is more problematic than expected. The predictions of future development are complicated by the sudden improvement in the resistance of the fiberboard after 6 months of simulation, as demonstrated by a significant reduction in mass loss. If we take the model into consideration (Figure 8), we can conclude that within 4 years the retardant will lose its efficiency, and the mass losses become similar to that of the samples without flame retardant, which would mean there would be no point in using it in real conditions in buildings. However, if the sudden improvement in efficiency mentioned above is only a local extreme and the efficiency then stabilizes after a year and a half, the flame retardant can perform its function for an incomparably long period of time. The authors tend to be inclined to the latter of these options, because of the significant stabilization of efficacy in the D and E sample groups, where there are negligible differences in results that still give very similar values compared to the A sample group. However, in order to confirm these assumptions, it would be necessary to procure data from a longer period of natural simulation, which was not possible for the reason mentioned above. It would also be unreliable to build a model based on data from the D and E sample groups.
As the experimental results demonstrated, the investigated FR reached its peak of efficiency 6 months after its application onto the fibreboards. However, we should take into account that six-month intervals are a relatively long period of time; measurements should be made in shorter time intervals to determine the peak efficiency more accurately. Markedly, besides the apparent protection of wood fibers, the retardant did not prevent the gradual increase in mass loss rate of samples, it just slowed down the process. Conclusively, the results obtained contribute to better understanding and predicting the fire behaviour of wood-based composites (fibreboards) used as insulation materials in building applications.
Data Availability Statement
The raw data supporting the conclusions of this article will be made available by the authors, without undue reservation.
Author Contributions
Conceptualization- LO, PM, and JJ; methodology- JJ; software- MT; validation- LO, PM, JJ; formal analysis, investigation, resources, data curation- LO and PM; writing–original draft preparation- JJ; writing–review and editing- LO, KK; visualization- DE, MT; supervision- LO. All authors have read and agreed to the published version of the manuscript.
Funding
This work was supported by Grant System of University of Zilina No. 1/2021 (13859). This article was funded by the Grant System of University of Zilina of the project: Experimental Determination of Fire-technical Parameters of Alternative Building Materials and Evaluation of its Fire Safety. Project No. 16961.
Conflict of Interest
The authors declare that the research was conducted in the absence of any commercial or financial relationships that could be construed as a potential conflict of interest.
Publisher’s Note
All claims expressed in this article are solely those of the authors and do not necessarily represent those of their affiliated organizations, or those of the publisher, the editors and the reviewers. Any product that may be evaluated in this article, or claim that may be made by its manufacturer, is not guaranteed or endorsed by the publisher.
References
Antov, P., Savov, V., and Neykov, N. (2019). Possibilities for Manufacturing Insulation Boards with Participation of Recycled Lignocellulosic Fibres. Manag. Sustain. Dev. 75, 72–76. doi:10.13140/RG.2.2.34391.11680
Ayrilmis, N. (2007). Effect of Fire Retardants on Internal Bond Strength and Bond Durability of Structural Fiberboard. Building Environ. 42 (3), 1200–1206. doi:10.1016/j.buildenv.2005.11.017
Bekhta, P., Bryn, O., Sedliačik, J., and Novák, I. (2016). Effect of Different Fire Retardants on Birch Plywood Properties. Acta Fac. Xylologiae 58 (1), 59–66. doi:10.17423/afx.2016.58.1.07
Bryn, O., Bekhta, P., Sedliačik, J., Forosz, V., and Galysh, V. (2016). The Effect of Diffusive Impregnation of Birch Veneers with Fire Retardant on Plywood Properties. BioRes 11 (4), 9112–9125. doi:10.15376/biores.11.4.9112-9125
Cai, L., Zhuang, B., Huang, D., Wang, W., Niu, M., Xie, Y., et al. (2016). Ultra-low Density Fibreboard with Improved Fire Retardance and thermal Stability Using a Novel Fire-Resistant Adhesive. BioRes 11 (2), 5215–5229. doi:10.15376/biores.11.2.5215-5229
Chen, T., Liu, J., Wu, Z., Wang, W., Niu, M., Wang, X., et al. (2016). Evaluating the Effectiveness of Complex Fire-Retardants on the Fire Properties of Ultra-low Density Fiberboard (ULDF). BioRes 11 (1), 1796–1807. doi:10.15376/BIORES.11.1.1796-1807
EN 13501-1 (2019). Fire Classification of Construction Products and Building Elements - Part 1: Classification Using Data from Reaction to Fire Tests. Brussels Belgium: European Committee for Standardization.
Igaz, R., Krišťák, Ľ., Ružiak, I., Gajtanska, M., and Kučerka, M. (2017). Thermophysical Properties of OSB Boards versus Equilibrium Moisture Content. BioRes 12 (4), 8106–8118. doi:10.15376/biores.12.4.8106-8118
Jieyu, L., Yinghai, C., and Caiying, S. (1994). Flame Retardant of Guanidine Condensed Phosphate (GCP) for wood. J. Northeast. For. Univ. 5, 58–63. doi:10.1007/bf02842974
Kadlicová, P., Gašpercová, S., and Osvaldová, L. M. (2017). Monitoring of Weight Loss of Fibreboard during Influence of Flame. Proced. Eng. 192, 393–398. doi:10.1016/j.proeng.2017.06.068
Lee, M., Lee, S. M., Kang, E. C., and Son, D. (2019). Combustibility and Characteristics of wood-fiber Insulation Boards Prepared with Four Different Adhesives. BioResources 14 (3), 6316–6330. doi:10.15376/biores.14.3.6316-6330
Lin, C.-f., Karlsson, O., Mantanis, G. I., Jones, D., and Sandberg, D. (2020a). “Fire Retardancy and Leaching Resistance of pine wood Impregnated with Melamine Formaldehyde Resin In-Situ with Guanyl-Urea Phosphate/boric Acid,” in 9th International Conference on Wood & Fire Safety (WFS 2020), High Tatras - Štrbské Pleso, Slovakia, 2-3 November, 2020 (Cham: Springer), 83–89. doi:10.1007/978-3-030-41235-7_12
Lin, C.-F., Karlsson, O., Mantanis, G. I., and Sandberg, D. (2020b). Fire Performance and Leach Resistance of pine wood Impregnated with Guanyl-Urea Phosphate/boric Acid and a Melamine-Formaldehyde Resin. Eur. J. Wood Prod. 78 (1), 107–111. doi:10.1007/s00107-019-01483-y
Lowden, L., and Hull, T. (2013). Flammability Behaviour of wood and a Review of the Methods for its Reduction. Fire Sci. Rev. 2, 4. doi:10.1186/2193-0414-2-4
Madyaratri, E. W., Ridho, M. R., Aristri, M. A., Lubis, M. A. R., Iswanto, A. H., Nawawi, D. S., et al. (2022). Recent Advances in the Development of Fire-Resistant Biocomposites-A Review. Polymers 14, 362. doi:10.3390/polym14030362
Makovicka Osvaldova, L., Kadlicova, P., and Rychly, J. (2020). Fire Characteristics of Selected Tropical Woods without and with Fire Retardant. Coatings 10, 527. doi:10.3390/COATINGS10060527
Makovická Osvaldová, L., and Osvald, A. (2013). Flame Retardation of Wood. Adv. Mat. Res. 693, 1331–1334. doi:10.4028/www.scientific.net/AMR.690-693.1331
Mantanis, G. (2002). Aqueous Fire Retardant. International Patent WO 02/102926 A1. Geneva: World Intellectual Property Organisation, 1–13.
Mantanis, G. I., Athanassiadou, E. T., Barbu, M. C., and Wijnendaele, K. (2018). Adhesive Systems Used in the European Particleboard, MDF and OSB Industries. Wood Mater. Sci. Eng. 13 (2), 104–116. doi:10.1080/17480272.2017.1396622
Mantanis, G. I., Martinka, J., Lykidis, C., and Ševčík, L. (2020). Technological Properties and Fire Performance of Medium Density Fibreboard (MDF) Treated with Selected Polyphosphate-Based Fire Retardants. Wood Mater. Sci. Eng. 15 (5), 303–311. doi:10.1080/17480272.2019.1596159
Martinka, J., Mantanis, G. I., Lykidis, C., Antov, P., and Rantuch, P. (2021). The Effect of Partial Substitution of Polyphosphates by Aluminium Hydroxide and Borates on the Technological and Fire Properties of Medium Density Fibreboard. Wood Mater. Sci. Eng., 1–7. doi:10.1080/17480272.2021.1933175
Martinka, J., Martinka, F., Rantuch, P., Hrušovský, I., Blinová, L., and Balog, K. (2018). Calorific Value and Fire Risk of Selected Fast-Growing wood Species. J. Therm. Anal. Calorim. 131, 899–906. doi:10.1007/s10973-017-6660-2
Mazela, B., and Broda, M. (2015). “Natural Polymer-Based Flame Retardants for wood and wood Products,” in Proceedings of the 11th Conference of the Northern European Network for Wood Sciences and Engineering 2015, September 14-15, 2015 (Poland: Poznan), 146–155. doi:10.1533/9781855737464.293
Němec, M., Igaz, R., Gergeľ, T., Danihelová, A., Ondrejka, V., Krišťák, Ľ., et al. (2019). Acoustic and Thermophysical Properties of Insulation Materials Based on wood Wool. Akustika 33, 115–123.
Östman, B. A.-L. (1984). Fire Retardant wood-fiber Insulating Board. J. Fire Sci. 2 (6), 454–467. doi:10.1177/073490418400200605
Osvald, A., Makovická Osvaldová, L., Mitrenga, P., Dušková Smrčková, M., and Chmelíková, D. (2016). Non-Normalized Evaluation Methods of Retarding Effects of Fire Retardants for Wood. Kem 688, 190–194. doi:10.4028/www.scientific.net/kem.688.190
Rowel, R. (2012). Handbook of Wood Chemistry and Wood Composites. USA: CRC Press, Taylor & Francis Group.
Savov, V., and Antov, P. (2020). Engineering the Properties of Eco-Friendly Medium Density Fibreboards Bonded with Lignosulfonate Adhesive. Drv. Ind. (Online) 71 (2), 157–162. doi:10.5552/drvind.2020.1968
Segovia, F., Blanchet, P., Auclair, N., and Essoua Essoua, G. (2020). Thermo-mechanical Properties of a wood Fiber Insulation Board Using a Bio-Based Adhesive as a Binder. Buildings 10 (9), 152. doi:10.3390/buildings10090152
Steico protect (2020). AvaliableAt: https://web.steico.com/sk/produkty/izolace-drevo/steicoprotect/prehled/(Accessed on September 14, 2020).
Sydor, M., and Wieloch, G. (2009). Construction Properties of wood Taken into Consideration in Engenering Practice. Drewno 52 (181), 63–73.
Tang, Q., Fang, L., and Guo, W. (2017). Investigation into Mechanical, thermal, Flame-Retardant Properties of wood Fiber Reinforced Ultra-high-density Fiberboards. BioRes 12 (3), 6749–6762. doi:10.15376/biores.12.3.6749-6762
Wang, J., Wang, F., Gao, Z., Zheng, M., and Sun, J. (2016). Flame Retardant Medium-Density Fiberboard with Expanded Vermiculite. BioResources 11 (3), 6940–6947. doi:10.15376/biores.11.3.6940-6947
White, R. H. (2009). “Accelerated Weathering of Fire-Retardant-Treated wood for Fire Testing,” in Proceedings of the 20th annual Conference on Recent Advances in Flame Retardancy of Polymeric Materials, Wellesley, MA, June 1-3, 2009 (Stamford, Connecticut: BCC Research), 246–256.
Winandy, J. E. (2001). Thermal Degradation of Fire-Retardant -treated wood: Predicting Residual Service Life. For. Prod. J. 51 (2), 47–54.
Wu, Z., Huang, D., Wang, W., Chen, T., Lin, M., Xie, Y., et al. (2017). Optimization for Fire Performance of Ultra-low Density Fiberboards Using Response Surface Methodology. BioResources 12 (2), 3790–3800. doi:10.15376/biores.12.2.3790-3800
Keywords: insulation fibreboards, wood, aging, fire resistance, fire retardancy
Citation: Osvaldová LM, Mitrenga P, Jancík J, Titko M, Efhamisisi D and Košútová K (2022) Fire Behaviour of Treated Insulation Fibreboards and Predictions of its Future Development Based on Natural Aging Simulation. Front. Mater. 9:891167. doi: 10.3389/fmats.2022.891167
Received: 07 March 2022; Accepted: 21 March 2022;
Published: 06 April 2022.
Edited by:
Petar Antov, University of Forestry, BulgariaReviewed by:
Maciej Sydor, Poznan University of Life Sciences, PolandViktor Savov, University of Forestry, Bulgaria
Jozef Martinka, Slovak University of Technology in Bratislava, Slovakia
Copyright © 2022 Osvaldová, Mitrenga, Jancík, Titko, Efhamisisi and Košútová. This is an open-access article distributed under the terms of the Creative Commons Attribution License (CC BY). The use, distribution or reproduction in other forums is permitted, provided the original author(s) and the copyright owner(s) are credited and that the original publication in this journal is cited, in accordance with accepted academic practice. No use, distribution or reproduction is permitted which does not comply with these terms.
*Correspondence: Linda Makovická Osvaldová, bGluZGEubWFrb3ZpY2thQHVuaXphLnNr